ABSTRACT
The purpose of this study was to determine the effect of different subjects from Lactiplantibacillus plantarum SCS5 (L. plantarum SCS5) on type 2 diabetes by modulating oxidative stress levels.The normal group (NG) and the model group (MG) of mice were fed normally, while the three test groups (LP1,LP2,LP3) received 10 ml/kg (body weight) of the L. plantarum SCS5 suspension, intracellular material, or heat-killed intracellular material daily for 10 weeks. The weight loss and the increase in blood glucose were alleviated in the LP2 and LP3 groups. Insulin, catalase, superoxide dismutase, and glutathione peroxidase significantly increased in the LP3 group of mice compared with the MG group. Additionally, the pathological sections showed that the lesions in the LP3 group were less severe than those in the MG group. These findings indicate that the heat-killed intracellular L. plantarum SCS5 material was most effective in alleviating oxidative stress in type 2 diabetic mice.
1. Introduction
The prevalence of type 2 diabetes (T2D) has reached epidemic proportions, with an estimated 400 million people affected worldwide (Zimmet et al., Citation2016). The continual rise in the incidence of T2D has led to a significant increase in the prevalence of diabetics over the past few decades (Lovic et al., Citation2020). T2D complications are highly common, as more than 50% of diabetic patients die from cardiovascular disease, and about 10% die from renal failure (Susan van et al., Citation2010). Oxidative stress may be a significant factor in the onset and progression of T2D, according to increasing evidence (Forbes et al., Citation2008). Chronic hyperglycemia in diabetic patients leads to excess production of reactive oxygen species (ROS) by enhancing mitochondrial oxygen consumption, disrupting mitochondrial function, and activating nicotinamide adenine phosphate oxidase. These events lead to oxidative stress when ROS production increases or endogenous antioxidant activity decreases, which, in turn, causes β-cell dysfunction and islet resistance (Zhang et al., Citation2020). Islet β-cells play an important role in the pancreas of T2D patients, and islet β-cell dysfunction and insulin resistance have serious consequences for recovery and treatment of diabetes (Ayepola et al., Citation2014).
Many studies have shown the significant role of lactic acid bacteria in improving oxidative stress. Lactiplantibacillus plantarum JLAU103 (L. plantarum JLAU103) protected against oxidative stress by activating antioxidation enzymes and Nrf2/Keap1 pathways (J. Wang et al., Citation2022). L. plantarum P101 can alleviate liver injury by modulating the Nrf2/HO-1 antioxidant pathway (S. Liu et al., Citation2023). Studies on L. plantarum CCFM8861 have also shown that treatment with it effectively reduces oxidative stress in the mouse brain (Yu et al., Citation2021). Taken together, these results suggest that Lactiplantibacillus improves the level of oxidative stress.
In recent times, numerous researchers have delved into the exploration of L. plantarum to improve oxidative stress in T2D. L. plantarum SS18–5 effectively controls the body weights of rats to reduce fasting glucose and enhance lipid peroxidation to improve T2D (Yang et al., Citation2021).An extract of L. plantarum TWK10-fermented soymilk enhances antioxidant enzyme activity, which, in turn, modulates factors related to cognitive function in T2D rats (T. H. Liu et al., Citation2020). L. plantarum JLAU103 exopolysaccharide (EPS103) protects against brain neurological damage in T2D mice by alleviating oxidative stress (Qi et al., Citation2022). In a prior study conducted within our group, investigating a different strain, L. plantarum SCS3 intriguingly revealed the potential of its boiled intracellular contents to proficiently regulate oxidative stress in mice with T2D (Meng, Citation2022b). However, these studies studied serum oxidative indicators while few studies have explored the effect of L. plantarum on diabetes-related symptoms by examining its effect on pancreatic oxidative stress. Research shows that relatively low levels of antioxidant enzymes, such as superoxide dismutase (SOD) and glutathione peroxidase (GPx), as well as serum levels of catalase (CAT) in pancreatic β-cells increase the likelihood of damage from ROS producing oxidative stress, which, in turn, reduces glucose-stimulated insulin (INS) secretion and aggravates diabetes (Robertson & Harmon, Citation2006). In the present study, we observed the effects of L. plantarum SCS5 and its intracellular material vs. heat-killed intracellular material on oxidative stress in streptozocin (STZ)-induced T2D mice by observing the changes in blood glucose-related indicators, malondialdehyde (MDA) in serum, and antioxidant levels in the pancreas. We hope to elucidate the ability of L. plantarum SCS5 to alleviate diabetic symptoms by regulating pancreatic oxidative stress in mice and to provide theoretical support and data for further studies on the mechanism of L. plantarum SCS5 to alleviate oxidative stress in T2D.
2. Materials and methods
2.1. Cultivation and treatment of L. plantarum SCS5
The bacteria were isolated from Sichuan fermented sausages in China, inoculated in sterilized Man, Rogosa, and Sharpe (MRS) broth medium (Hangzhou Bess Biotechnology Co., Ltd., Hangzhou, China), and incubated at 37°C for 19 h in a constant temperature incubator.
Then, the cultured strains were divided into three parts for centrifugation (8,000 rpm, 4°C, 5 min). The first LP1 part was a resuspension that was gavaged using a 0.1 mol/L sterile phosphate-buffered saline (PBS) resuspension (suspension: PBS = 1:10). The second LP2 part was added to the cell lysate (Beyoncé Biotechnology Co., Ltd.) and placed at 4°C for 1 h. The supernatant (suspension: PBS = 1:10) was collected and gavaged. The LP3 part was obtained from the supernatant in a centrifuge tube and placed in a water bath at 100°C for 30 min to ensure inactivation of the protein (suspension: PBS = 1:10).
2.2. Animal experiment
This study was approved by the Animal Ethics Committee of Chengdu University of Traditional Chinese Medicine (2019–2020) and complied with the 2010/63/EU Directive (European Parliament and Council of the European Union, Citation2010). The experimental mice were 6-week-old Kunming male mice without a specific pathogen from Sichuan Dashuo Biotechnology Co., Ltd. (Sichuan, China) They were fed for 1 week and each group of 15 was divided into the normal group (NG), the model group (MG), the L. plantarum SCS5 suspension group (LP1), the L. plantarum SCS5 intracellular materials group (LP2), and the high-temperature treated L. plantarum SCS5 heat-killed intracellular materials group (LP3). All mice, except the NG mice, were given 0.2 mL of 50 mg/kg (body weight) STZ during the second week by intraperitoneal injection for 5 days (Y. Wang et al., Citation2018), and their blood glucose levels were measured after 1 week of adaptation. The diabetic mouse model was successfully established with fasting glucose levels ≥7.0 mmol/L and 2-h oral glucose tolerance test (OGTT) glucose levels ≥11.1 mmol/L (Society, Citation2021). The NG mice were fed normally during intragastric administration, and the LP1, LP2, and LP3 mice were administered 10 mL/kg (body weight) of L. plantarum SCS5 suspension, intracellular materials, and heat-killed intracellular materials every day, respectively, for 10 weeks. The entire experiment lasted 13 weeks ().
Table 1. The flowchart of the experimental protocol. NG, normal group; MG, model group; LP1, gavage 10 mL/kg (body weight) of L. plantarum SCS5 suspension; LP2, gavage 10 mL/kg (body weight) of L. plantarum SCS5 intracellular material; LP3, gavage 10 mL/kg (body weight) of L. plantarum SCS5 heat-killed intracellular material; STZ, intraperitoneal injection of 50 mg/kg (body weight) STZ for 5 days.
2.3. Measurement of diabetes-related indicators
2.3.1. Weight monitoring
Body weight was measured after 12 h of fasting and then measured once per week for 13 weeks from the beginning of the experiment.
2.3.2. Monitoring the variations in blood glucose
Fasting blood glucose (FBG) was measured after 12 h of fasting and measured once per week for 13 consecutive weeks from the beginning of the experiment.
2.3.3. Test for oral glucose tolerance
The OGTT was performed after successful modeling was achieved on week 13. The mice were fasted for 12 h but drank water. Blood glucose was measured at 0, 15, 30, 60, 90, and 120 min after gavage with 2.0 g/kg (body weight) of glucose, respectively.
2.3.4. Insulin and glycosylated hemoglobin parameters
The serum levels of glycosylated hemoglobin (HbA1c) and INS in the pancreatic tissue of the mice were measured with enzyme-linked immunosorbent assay (ELISA) kits during week 13 (Shanghai Enzyme Linked Biotechnology Co., Ltd., Shanghai, China).
2.4. Oxidative indicators and pathological sectioning
2.4.1. MDA analysis
Orbital blood was obtained from the mice at the end of the experiment on week 13. The blood was centrifuged, and the supernatant was collected as the serum. The serum MDA levels in the mice were determined with an ELISA kit. (Shanghai Enzyme Linked Biotechnology). In summary, the 96-well plate is organized with blank, standard, and sample wells. Blank wells exclude test samples and enzyme reagents, while standard wells contain varying standard concentrations. Sample dilution is added to sample wells, followed by the test sample. Incubation occurs for 60 minutes at 37°C under light-protected sealing, followed by a 5-time wash. Enzyme reagent is added, with another 60-minute light-protected incubation and 5 washes. Color developers A and B are gently shaken into each well and incubated at 37°C for 15 minutes. Termination solution is added, and OD value is measured at 450 nm. All tests are duplicated under accepted conditions.
2.4.2. Pancreatic antioxidant analysis and pathological sections
Upon the conclusion of the experiment, the mice underwent dissection. The pancreas was meticulously removed from the freshly dissected mice in a sterile environment. It was then cleansed using cold 0.85% saline solution and subsequently weighed. Specific pancreatic tissues were scrutinized to ascertain the levels of SOD, CAT, GPx, glutathione (GSH), and heme oxygenase-1 (HO-1) by utilizing ELISA kits (Shanghai Enzyme Linked Biotechnology). The measurement method was consistent with that described in section 2.3.4. The remaining portion of pancreatic tissue was soaked in a 10% neutral formalin fixative solution and transferred to Chengdu Lilai Biotechnology Co., Ltd. (Pathology Department of Chengdu Lilai Biomedical Experimental Center) for detection. Hematoxylin-eosin-stained tissues were used to detect pancreatic pathology with a microscope.
2.5. Statistical analysis
The results are expressed as mean ± standard deviation. The mouse body weights, blood glucose, INS content, HbA1c, MDA, and antioxidant levels were analyzed using SPSS 27.0 software (IBM SPSS, Inc., Armonk, NY, U.S.A.). Data conforming to a normal distribution were analyzed using one-way analysis of variance, and data that did not conform to a normal distribution were subjected to nonparametric tests. P-value < .05 was considered significant.
3. Results
3.1. Regulation of body weight by L. plantarum SCS5
As shown in , body weight tended to decrease in all groups of mice after week 4, except in the NG group, suggesting that STZ-induced diabetes is associated with weight loss. The body weights of the mice in the LP2 group began to increase at the beginning of week 9 (gavage week 6) and stabilized by the end of the experiment. The body weights of mice in the LP3 group increased by week 13 (gavage week 10). These results indicate that the L. plantarum SCS5 intracellular material and heat-killed intracellular material alleviated weight loss in T2D mice.
Figure 1. Body weight changes of mice between different groups. Normal group (NG), STZ treatment group (MG); STZ + L. plantarum SCS5 suspension group (LP1); STZ + L. plantarum SCS5 intracellular material group (LP2); STZ + L. plantarum SCS5 heat-killed intracellular material group (LP3). *Values compared with MG mice are significantly different at p < .05.
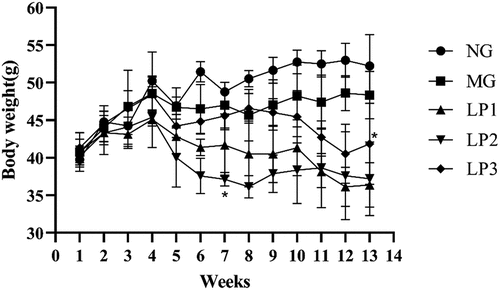
3.2. Regulation of fasting blood glucose (FBG) by L. plantarum SCS5
As shown in , FBG increased in all groups, except the NG group after week 3. This result suggests that the blood glucose of the mice injected with STZ was adversely affected, which may have occurred due to the destruction of pancreatic islet β-cells caused by STZ (Furman, Citation2021). The FBG of mice in the LP3 group decreased beginning in week 4 (gavage week 1) and fell to a normal level of 5.33 mmol/L by week 12. The FBG of mice in the LP2 group decreased on week 11 (gavage week 8). These results indicate that the L. plantarum SCS5 intracellular material and heat-killed intracellular material alleviated the increased blood glucose levels in T2D mice.
Figure 2. Changes in fasting blood glucose level. Normal group (NG); STZ treatment group (MG); STZ + L. plantarum SCS5 suspension group (LP1); STZ + L. plantarum SCS5 intracellular material group (LP2); STZ + L. plantarum SCS5 heat-killed intracellular material group (LP3). *Values compared with other groups mice are significantly different at p < .05.
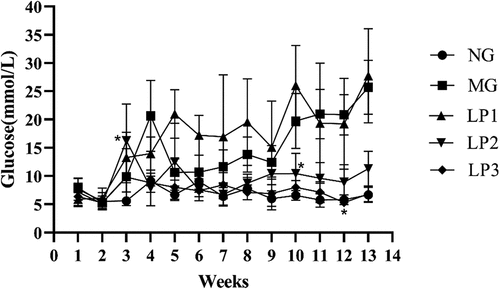
3.3. Regulation of oral glucose tolerance by L. plantarum SCS5
Blood glucose increased in all groups of mice after the glucose infusion, and the blood glucose levels in all groups were higher than those in the NG group at 15 min. No significant decrease was detected (; p > .05). The area under the curve (AUC) values of the MG, LP1, LP2, and LP3 groups were significantly different (). This result indicates that STZ reduced glucose tolerance in mice. As shown in , the blood glucose levels of the mice after 10 weeks of gavage, except those in the MG and LP1 groups, decreased from 30 min onwards. Among them, the decrease in the blood glucose level in the LP3 group was higher than that in the LP2 group, and it decreased to 10.25 mmol/L at 120 min. As shown in , the AUC values of the LP2 and LP3 groups were significantly different from those of the MG and NG groups (p < .05), but their AUC values were closer to that of the NG group. These results suggest that the L. plantarum SCS5, intracellular material, and heat-killed intracellular material improved glucose tolerance in hyperglycemic mice.
Figure 3. Changes in blood glucose levels and oral glucose tolerance. a,b before the experiment, c,d at the end of the experiment, oral glucose tolerance of experimental mice after 13 weeks of administration of L. plantarum SCS5, and blood glucose levels measured at 0, 30, 60, 90 and 120 min respectively. At the same time, the area under the curve of the drawn curve is calculated. Normal group (NG); STZ treatment group (MG); STZ + L. plantarum SCS5 suspension group (LP1); STZ + L. plantarum SCS5 intracellular material group (LP2); STZ + L. plantarum SCS5 heat-killed intracellular material group (LP3). *Values compared with NG mice are significantly different at p < .05. #Values compared with MG mice are significantly different at p < .05.
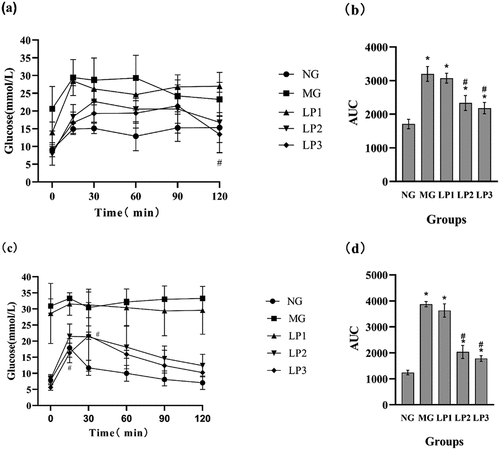
3.4. Modulation of serum HbA1c by L. plantarum SCS5
According to , the serum HbA1c levels of mice in the LP1, LP2, and LP3 groups decreased compared with that in the MG group. The LP1 group had the lowest HbA1c level of 476.25 nmol/L, suggesting that the L. plantarum SCS5 suspension reduced the serum HbA1c level in T2DM mice.
Figure 4. HbA1c level in serum of experimental mice. HbA1c level of experimental mice given L. plantarum SCS5 at week 13. Normal group (NG); STZ treatment group (MG); STZ + L. plantarum SCS5 suspension group (LP1); STZ + L. plantarum SCS5 intracellular material group (LP2); STZ + L. plantarum SCS5 heat-killed intracellular material group (LP3). a,bValues in the same column with different superscript letters significantly differ at p < .05.
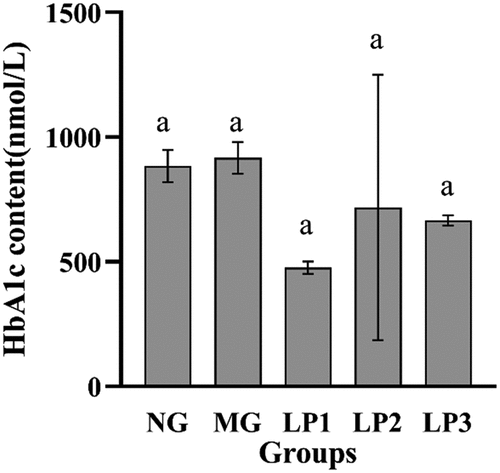
3.5. Modulation of pancreatic INS by L. plantarum SCS5
According to , the serum INS levels of mice in the LP1 and LP2 groups did not change significantly (p > .05), compared with that in the MG group, but the INS level was significantly higher in the LP3 group (16.90 mIU/L). These results suggest that heat-killed L. plantarum SCS5 intracellular material increased INS secretion.
Figure 5. Change of INS levels in pancreas of mice during the experiments. INS level of experimental mice given L. plantarum SCS5 at week 13. Normal group (NG); STZ treatment group (MG); STZ + L. plantarum SCS5 suspension group (LP1); STZ + L. plantarum SCS5 intracellular material group (LP2); STZ + L. plantarum SCS5 intracellular heat-killed material group (LP3). a,bValues in the same column with different superscript letters significantly differ at p < .05.
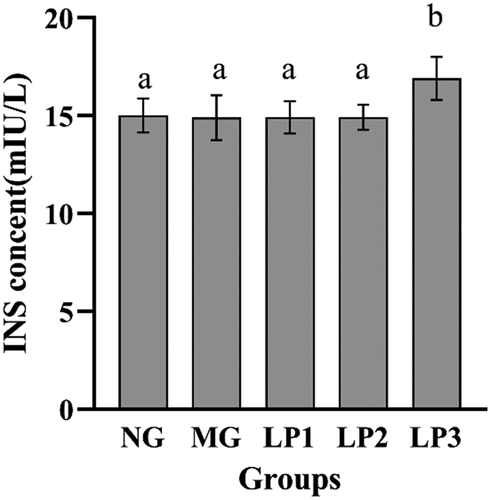
3.6. Modulation of serum MDA by L. plantarum SCS5
As shown in , MDA content in the MG group increased compared to that in the NG group, possibly because of the destruction of pancreatic β-cells caused by STZ, which produces INS resistance manifested as hyperglycemia leading to increased lipid peroxidation (Sasson, Citation2017). The MDA levels in the mice from the LP1, LP2, and LP3 groups decreased after 10 weeks of gavage, compared to the MG group. The LP2 group decreased to 4.26 nmol/L, while the LP1 group had the lowest level at 4.24 nmol/L. These results indicate that the L. plantarum SCS5 suspension and intracellular material effectively removed MDA from diabetic mice.
Figure 6. MDA level in serum of experimental mice. MDA level of experimental mice given L. plantarum SCS5 at week 13. Normal group (NG); STZ treatment group (MG); STZ + L. plantarum SCS5 suspension group (LP1); STZ + L. plantarum SCS5 intracellular material group (LP2); STZ + L. plantarum SCS5 heat-killed intracellular material group (LP3). a,bValues in the same column with different superscript letters significantly differ at p < .05.
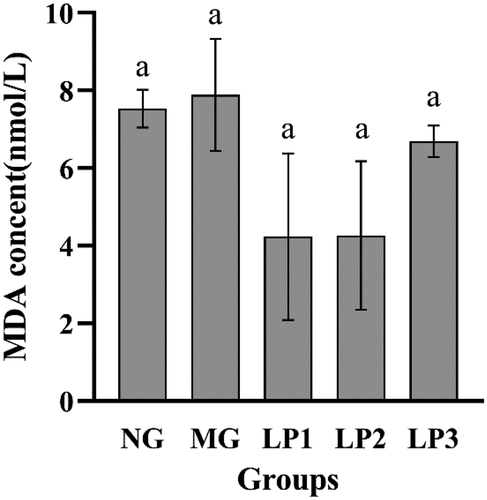
3.7. Effects of L. plantarum SCS5 on antioxidants in pancreas
As shown in , the levels of SOD (124.96 IU/mL), CAT (74.70 IU/mL), GPx (0.54 IU/mL), and GSH (206.993 ng/L) increased in the LP3 group after 10 weeks of gavage compared to those in the MG group, while except for HO-1 the LP2 group had lower antioxidant levels than the LP3 group, and the LP1 group had the highest HO-1 level at 1,138.71IU/L. The levels of SOD, CAT, and GPx were significantly higher in the LP3 group than in the MG group (P < 0.05). These findings demonstrate that key antioxidant levels varied in the pancreas of hyperglycemic mice. The L. plantarum SCS5 heat-killed intracellular material boosted antioxidant activity.
Figure 7. SOD (a),CAT (b),GPx (c),HO-1 (d),GSH (e) levels in pancreas of mice. Normal group (NG); STZ treatment group (MG); STZ + L. plantarum SCS5 suspension group (LP1); STZ + L. plantarum SCS5 intracellular material group (LP2); STZ + L. plantarum SCS5 heat-killed intracellular material group (LP3). a,bValues in the same column with different superscript letters significantly differ at p < .05.
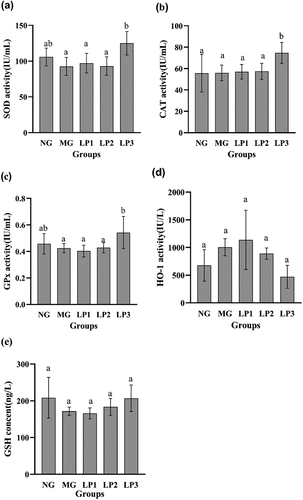
3.8. Pathological section analysis of the pancreatic tissue
As shown in , the pancreatic tissue structure of the mice in the NG group was normal, while islet cells in the MG group were necrotic, cell nuclei were consolidated, and the cytoplasm was vacuolated. The lesions in the LP1 group were relatively severe compared with those in the MG group, with different degrees of degeneration and necrosis of the glandular vesicle epithelial cells. A small number of glandular cells invaded the islets in the LP2 group, and the islet cells were necrotic. However, the extent of pancreatic tissue lesions in the LP3 group was relatively mild, with only a small amount of islet cell necrosis, and no significant lesions were observed. These results show that L. plantarum SCS5 heat-killed intracellular material significantly ameliorated the pathological changes, such as islet cell proliferation and necrosis, in hyperglycemic mice.
4. Discussion
The key cause for the development of T2D is defective INS secretion from pancreatic β-cells, which do not secrete sufficient INS to maintain normal glucose homeostasis, and insulin-sensitive tissues are unable to respond to the INS (Roden & Shulman, Citation2019). Hyperglycemia and INS resistance develop as the disease progresses (Galicia-Garcia et al., Citation2020). The results of this study show that L. plantarum SCS5 improved weight loss in diabetic mice and had a modulating effect on FBG, OGTT, and HbA1c. The best effects were observed with the heat-killed L. plantarum SCS5 intracellular material. Our previous study reported positive effects of heat-killed L. plantarum SCS4 intracellular material on weight loss in mice (Meng et al., Citation2022a) and that L. plantarum Y15 regulates gene expression related to inflammatory and INS signaling pathways by reducing pro-inflammatory cytokines and improving diabetes-related biochemical indicators, such as glucose tolerance and FBG levels (Y. Liu et al., Citation2022). We then measured INS in mouse islet cells and observed its relationship with glucose tolerance and found that the L. plantarum SCS5 heat-killed intracellular material had the best modulating effect on INS deficiency. However, the L. plantarum SCS5 heat-killed intracellular material increased pancreatic INS, which was inconsistent with our previous finding that L. plantarum SCS3 heat-killed intracellular material lowers serum INS (Meng, Citation2022b). This may be due to the different phases of INS secretion during the early stages of diabetes. We hypothesized that the L. plantarum SCS3 heat-killed intracellular material group may have been in the first phase of reduced INS secretion. Our experiment was conducted during the second phase, which revealed increased INS secretion due to the increase in blood glucose caused by the first phase (Del Prato & Tiengo, Citation2001).
Oxidative stress is a risk factor for the development and progression of T2D. MDA is cytotoxic and causes cross-linking and polymerization of proteins, nucleic acids, and other macromolecules when produced in large quantities, which disrupts the structure and function of cell membranes (H. Liu et al., Citation2015). The MDA level is positively correlated with oxidative stress and lipid peroxidation and indirectly reflects the severity of the free radical attack (Gorski et al., Citation2003). The current study found an increase in serum MDA levels in mice after the STZ injections and a significant decrease in serum MDA levels with the L. plantarum SCS5 suspension after gavage week 10, suggesting that the L. plantarum SCS5 suspension reduced lipid peroxidation in the mice. Our other study revealed that the MDA level was higher in the gavage heat-killed L. plantarum SCS3 group than in the MG group (Meng, Citation2022b), which may be due to different different treated L. plantarum subjects used. We hypothesized that particularly active substances may be present in the L. plantarum SCS5 suspension compared to the heat-killed L. plantarum SCS3 intracellular material, which prevented the generation of new free radicals, eliminated existing free radicals, and promoted the elimination of these free radical metabolites. MDA is excreted as a free radical metabolite.
The development of T2D is associated with antioxidants in the body, and the level of cellular antioxidant enzyme activity indirectly reflects the body’s ability to scavenge oxygen free radicals (Fink & Scandalios, Citation2002; Warner et al., Citation2004). CAT activity increases linearly with the effective concentration of H202, whereas GPx scavenges H202 and transforms MDA into normal fatty acids, which reduces the membrane damage brought on by lipid peroxidation chain events. There is a synergistic effect between SOD, GPx, and CAT. The Cu2+ in SOD is reduced by the eliminate H202 to protect SOD. Similarly, O2- inactivates CAT and GPx, while SOD protects these two enzymes (Herbette et al., Citation2003). HO-1 is a phase II detoxification enzyme that triggers a subordinate signaling pathway with protective effects against oxidative stress (An et al., Citation2021). This study showed that the L. plantarum SCS5 suspension had a positive effect on the HO-1 level, while the heat-killed L. plantarum SCS5 intracellular material had no effect on HO-1. As HO-1 expression is primarily regulated at the transcriptional level, transcription factors bind to the HO-1 gene promoter region and participate in transcriptional activity, such as heat-shock factor, activator protein-1, and nuclear factor-κB (Alam & Cook, Citation2007). Therefore, we hypothesized that the L. plantarum SCS5 suspension may activate multiple transcription factors and stimulate HO-1 to maintain cellular homeostasis under oxidative stress conditions. However, the heat-killed L. plantarum SCS5 intracellular material significantly increased SOD, GPx, and CAT activities in the pancreas. The heat-killed L. plantarum SCS5 intracellular material may have allowed Nrf2 to encode phase II detoxifying and antioxidant enzymes by increasing Nrf2 signaling, which acts synergistically through SOD, GPx, and CAT to scavenge free radicals and reduce oxidative stress in T2D mice (H. Li et al., Citation2020). The ability to increase antioxidant enzyme levels has been reported in studies on L. plantarum KSFY06 (Li et al., Citation2019a). L. plantarum NA136 treatment results in increased CAT expression, which, in turn, triggers the translocation of Nrf2 to the nucleus, thereby regulating cellular antioxidant enzymes, including SOD and HO-1 (Y. Wang et al., Citation2018). Based on these results, the heat-killed L. plantarum SCS5 intracellular material significantly increased antioxidant activities in the pancreas. Interestingly, the pancreatic tissue structure of the NG group was relatively clear, with islet cell clusters of different sizes and clear borders and no obvious necrosis. Some tissues in the MG, LP1, LP2, and LP3 groups showed different degrees of invasion of the exocrine part of the alveolar cells into the islets, different numbers of islet cells, invasion of islet cells into the islets, and a small amount of islet cell necrosis. However, the extent of the pancreatic tissue lesions in the LP3 group was relatively mild. This result suggests that the L. plantarum SCS5 heat-killed intracellular material protected islet cells by alleviating oxidative stress.
Overall, oxidative stress is thought to play an important role in the pathogenesis of T2D (Herlein et al., Citation2010). However, probiotics improve symptoms caused by oxidative stress. In this study, the L. plantarum SCS5 heat-killed intracellular material modulated oxidative stress by increasing the activities of antioxidant enzymes and substances (SOD, GPx, CAT, and GSH) in the pancreas of mice and ameliorated hyperglycemia and weight loss. One of our studies also suggested that L. plantarum SCS4 heat-killed intracellular material improves hyperglycemia and weight loss by regulating key antioxidant enzyme activities (Meng et al., Citation2022a). As the adverse effects of prolonged exposure to high glucose levels on β-cells are related to oxidative stress, we hypothesized that oxidative stress can be prevented by increasing antioxidant contents using the L. plantarum SCS5 heat-killed intracellular material, leading to the alleviation of diabetic symptoms and ameliorating the adverse changes in β-cells (Gao et al., Citation2021). In addition, the L. plantarum SCS5 suspension regulated oxidative stress in the body by reducing the MDA level, resulting in the alleviation of weight loss in the diabetic mice. This may have occurred because the L. plantarum SCS5 suspension restored the intestinal flora and intestinal barrier function in mice and further affected Nrf2/Keap1 pathway-related protein expression to attenuate oxidative stress in mice (Maritim et al., Citation2003).
5. Conclusions
This study shows that the L. plantarum SCS5 heat-killed intracellular material improved the symptoms associated with T2D by increasing the levels of key antioxidant enzymes and substances as well as INS in the pancreas of T2D mice and reducing the levels of MDA and HbA1c in serum. The antioxidants are essential for the L. plantarum SCS5 heat-killed intracellular material but their mechanisms of action, remain unknown. Research shows that substances, such as lipopolysaccharides and peptidoglycans, may be present in heat-killed L. plantarum (Taverniti & Guglielmetti, Citation2011). L. plantarum activates the Keap1-Nrf2-ARE pathway, which plays an important role in protecting cells from oxidative stress (Li et al., Citation2019b). The goal of our future research is to determine the key substances that play a role in the L. plantarum SCS5 heat-killed intracellular material. We will determine whether they bind to Keap1 in the form of small molecular peptides, which induce Nrf2 translocation to the nucleus, and increase the expression levels of antioxidant substances in pancreatic β-cells by activating the Keap1-Nrf2-ARE signaling pathway, thereby reducing oxidative damage in vivo and preventing T2D.
Authors’ contributions
Xiao Meng contributed to the experimental study design and completed the experimental study and manuscript. Siheng Ren contributed to the experimental study and wrote the manuscript. Yongkun Hu, Ran Ran and Weixi Ge contributed to the experimental study. Ying Zhang performed quality control on the experimental results. Lishi Jiang and Shukun Liu performed the quality control of the paper. Xiao Meng and Siheng Ren contributed equally to the article.
Disclosure statement
No potential conflict of interest was reported by the author(s).
Data availability statement
The datasets generated for this study are available upon request to the corresponding author.
References
- Alam, J., & Cook, J. L. (2007). How many transcription factors does it take to turn on the heme oxygenase-1 gene? American Journal of Respiratory Cell and Molecular Biology, 36(2), 166–174. https://doi.org/10.1165/rcmb.2006-0340TR
- An, R., Li, D., Dong, Y., She, Q., Zhou, T., Nie, X., Pan, R., & Deng, Y. (2021). Methylcobalamin protects melanocytes from H2O2-induced oxidative stress by activating the Nrf2/HO-1 Pathway. Drug Design, Development and Therapy, 15, 4837–4848. https://doi.org/10.2147/dddt.S336066
- Ayepola, O. R., Brooks, N. L., & Oguntibeju, O. O. (2014). Oxidative stress and diabetic complications: The role of antioxidant vitamins and flavonoids. Antioxidant-Antidiabetic Agents and Human Health https://doi.org/10.5772/57282
- Del Prato, S., & Tiengo, A. (2001). The importance of first-phase insulin secretion: Implications for the therapy of type 2 diabetes mellitus. Diabetes/Metabolism Research and Reviews, 17(3), 164–174. https://doi.org/10.1002/dmrr.198
- European Parliament and Council of the European Union. (2010). Directive 2010/63/EU of the European parliament and of the council on the protection of animals used for scientific purposes. Council of Europe.
- Fink, R. C., & Scandalios, J. G. (2002). Molecular evolution and structure–function relationships of the superoxide dismutase gene families in angiosperms and their relationship to other eukaryotic and prokaryotic superoxide dismutases. Archives of Biochemistry and Biophysics, 399(1), 19–36. https://doi.org/10.1006/abbi.2001.2739
- Forbes, J. M., Coughlan, M. T., & Cooper, M. E. (2008). Oxidative stress as a major culprit in kidney disease in diabetes. Diabetes, 57(6), 1446–1454. https://doi.org/10.2337/db08-0057
- Furman, B. L. (2021). Streptozotocin‐induced diabetic models in mice and rats. Current Protocols, 1(4). https://doi.org/10.1002/cpz1.78
- Galicia-Garcia, U., Benito-Vicente, A., Jebari, S., Larrea-Sebal, A., Siddiqi, H., Uribe, K. B., Ostolaza, H., & Martín, C. (2020). Pathophysiology of type 2 diabetes mellitus. International Journal of Molecular Sciences, 21(17), 6275. https://doi.org/10.3390/ijms21176275
- Gao, Y., Liu, Y., Ma, F., Sun, M., Song, Y., Xu, D., Mu, G., & Tuo, Y. (2021). Lactobacillus plantarum Y44 alleviates oxidative stress by regulating gut microbiota and colonic barrier function in Balb/C mice with subcutaneous d-galactose injection. Food & Function, 12(1), 373–386. https://doi.org/10.1039/d0fo02794d
- Gorski, J. A., Balogh, S. A., Wehner, J. M., & Jones, K. R. (2003). Learning deficits in forebrain-restricted brain-derived neurotrophic factor mutant mice. Neuroscience, 121(2), 341–354. https://doi.org/10.1016/s0306-4522(03)00426-3
- Herbette, S., Lenne, C., Labrouhe, D. T., Drevet, J. R., & Roeckel-Drevet, P. (2003). Transcripts of sunflower antioxidant scavengers of the SOD and GPX families accumulate differentially in response to downy mildew infection, phytohormones, reactive oxygen species, nitric oxide, protein kinase and phosphatase inhibitors. Physiologia Plantarum, 119(3), 418–428. https://doi.org/10.1034/j.1399-3054.2003.00186.x
- Herlein, J. A., Fink, B. D., & Sivitz, W. I. (2010). Superoxide production by mitochondria of insulin-sensitive tissues: Mechanistic differences and effect of early diabetes. Metabolism: Clinical and Experimental, 59(2), 247–257. https://doi.org/10.1016/j.metabol.2009.07.021
- Li, F., Huang, G., Tan, F., Yi, R., Zhou, X., Mu, J., & Zhao, X. (2019a). Lactobacillus plantarum KSFY06 on d‐galactose‐induced oxidation and aging in Kunming mice. Food Science & Nutrition, 8(1), 379–389. https://doi.org/10.1002/fsn3.1318
- Li, M., Liu, X., Liu, Y., Wang, Y., Yi, C., Tian, J., Liu, K., Chu, J., & Li, M. (2019b). Protective effect of lactobacillus plantarum on alcoholic liver injury and regulating of keap-Nrf2-ARE signaling pathway in zebrafish larvae. Plos One, 14(9), e0222339. https://doi.org/10.1371/journal.pone.0222339
- Li, H., Shi, J., Zhao, L., Guan, J., Liu, F., Huo, G., & Li, B. (2020). Lactobacillus plantarum KLDS1.0344 and Lactobacillus acidophilus KLDS1.0901 mixture prevents chronic alcoholic liver injury in mice by protecting the intestinal barrier and regulating gut microbiota and liver-related pathways. Journal of Agricultural and Food Chemistry, 69(1), 183–197. https://doi.org/10.1021/acs.jafc.0c06346
- Liu, T. H., Lin, W. J., Cheng, M. C., & Tsai, T. Y. (2020). Lactobacillus plantarum TWK10‐fermented soymilk improves cognitive function in type 2 diabetic rats. Journal of the Science of Food and Agriculture, 100(14), 5152–5161. https://doi.org/10.1002/jsfa.10564
- Liu, S., Zhao, Y., Xu, X., Wang, M., Tao, X., & Xu, H. (2023). Lactiplantibacillus plantarumP101 alleviates alcoholic liver injury by modulating the Nrf2/HO-1 pathway in mice. Journal of Applied Microbiology, 134(1). https://doi.org/10.1093/jambio/lxac032
- Liu, H., Zhao, M., Yang, S., Gong, D.-R., Chen, D.-Z., & Du, D.-Y. (2015). (2R,3S)-pinobanksin-3-cinnamate improves cognition and reduces oxidative stress in rats with vascular dementia. Journal of Natural Medicines, 69(3), 358–365. https://doi.org/10.1007/s11418-015-0901-0
- Liu, Y., Zheng, S., Cui, J., Guo, T., & Zhang, J. (2022). Lactiplantibacillus plantarum Y15 alleviate type 2 diabetes in mice via modulating gut microbiota and regulating NF-κB and insulin signaling pathway. Brazilian Journal of Microbiology, 53(2), 935–945. https://doi.org/10.1007/s42770-022-00686-5
- Lovic, D., Piperidou, A., Zografou, I., Grassos, H., Pittaras, A., & Manolis, A. (2020). The growing epidemic of diabetes mellitus. Current Vascular Pharmacology, 18(2), 104–109. https://doi.org/10.2174/1570161117666190405165911
- Maritim, A. C., Sanders, R. A., & Watkins, J. B. (2003). Diabetes, oxidative stress, and antioxidants: A review. Journal of Biochemical and Molecular Toxicology, 17(1), 24–38. https://doi.org/10.1002/jbt.10058
- Meng, X., Chen, X.-Z., Sun, J.-Y., Zhang, Y., Jiang, L.-S., & Wang, J. (2022a). Exploring the oxidative stress Regulation of mice with hyperglycemia by Lactiplantibacillus plantarum SCS4. Current Microbiology, 79(11). https://doi.org/10.1007/s00284-022-03008-y
- Meng, X., Liu, S., Liu, Q., Zhang, Y., Jing, L., Huang, X., Sun, J., & Ye, L. (2022b). Anti-oxidative stress properties by Lactiplantibacillus plantarum SCS3 in streptozotocin-induced diabetic mice. CyTA - Journal of Food, 20(1), 297–304. https://doi.org/10.1080/19476337.2022.2136759
- Qi, Y., Wang, D., Fang, L., Liu, X., Liu, C., Zhao, F., Wu, D., Wang, X., Wang, J., & Min, W. (2022). Hypoglycemic effect of exopolysaccharide from Lactiplantibacillus plantarum JLAU103 on streptozotocin and high-fat diet-induced type 2 diabetic mice. Foods, 11(22), 3571. https://doi.org/10.3390/foods11223571
- Robertson, R. P., & Harmon, J. S. (2006). Diabetes, glucose toxicity, and oxidative stress: A case of double jeopardy for the pancreatic islet β cell. Free Radical Biology and Medicine, 41(2), 177–184. https://doi.org/10.1016/j.freeradbiomed.2005.04.030
- Roden, M., & Shulman, G. I. (2019). The integrative biology of type 2 diabetes. Nature, 576(7785), 51–60. https://doi.org/10.1038/s41586-019-1797-8
- Sasson, S. (2017). Nutrient overload, lipid peroxidation and pancreatic beta cell function. Free Radical Biology and Medicine, 111, 102–109. https://doi.org/10.1016/j.freeradbiomed.2016.09.003
- Society, C. D. (2021). Guidelines for the prevention and treatment of type 2 diabetes in China (2020 edition). International Journal of Endocrinology and Metabolism, 41, 482–548. https://doi.org/10.3760/cma.j.cn115791-20210221-00095
- Susan van, D., Beulens, J. W. J., Yvonne, T., van der, S., Grobbee, D. E., & Nealb, B. (2010). The global burden of diabetes and its complications: An emerging pandemic. European Journal of Cardiovascular Prevention & Rehabilitation, 17(1_suppl), s3–s8. https://doi.org/10.1097/01.hjr.0000368191.86614.5a
- Taverniti, V., & Guglielmetti, S. (2011). The immunomodulatory properties of probiotic microorganisms beyond their viability (ghost probiotics: Proposal of paraprobiotic concept). Genes & Nutrition, 6(3), 261–274. https://doi.org/10.1007/s12263-011-0218-x
- Wang, J., Li, M., Gao, Y., Li, H., Fang, L., Liu, C., Liu, X., & Min, W. (2022). Effects of exopolysaccharides from Lactiplantibacillus plantarum JLAU103 on intestinal immune response, oxidative stress, and microbial communities in cyclophosphamide-induced immunosuppressed mice. Journal of Agricultural and Food Chemistry, 70(7), 2197–2210. https://doi.org/10.1021/acs.jafc.1c06502
- Wang, Y., Li, M., & Ni, Z. (2018). Primary study on the hypoglycemic mechanism of 5rolGLP-HV in STZ-induced type 2 diabetes mellitus mice. Journal of Biosciences, 43(5), 921–929. https://doi.org/10.1007/s12038-018-9809-7
- Warner, D. S., Sheng, H., & Batinić-Haberle, I. (2004). Oxidants, antioxidants and the ischemic brain. Journal of Experimental Biology, 207(18), 3221–3231. https://doi.org/10.1242/jeb.01022
- Yang, F., Wang, J., Zhang, H., Xie, Y., Jin, J., Liu, H., Pang, X., & Hao, H. (2021). Hypoglycemic effects of space‐induced Lactobacillus plantarum SS18‐5 on type 2 diabetes in a rat model. Journal of Food Biochemistry, 45(9). https://doi.org/10.1111/jfbc.13899
- Yu, L., Zhang, L., Duan, H., Zhao, R., Xiao, Y., Guo, M., Zhao, J., Zhang, H., Chen, W., & Tian, F. (2021). The protection of Lactiplantibacillus plantarum CCFM8661 against benzopyrene-induced toxicity via regulation of the gut microbiota. Frontiers in Immunology, 12, 736129. https://doi.org/10.3389/fimmu.2021.736129
- Zhang, P., Li, T., Wu, X., Nice, E. C., Huang, C., & Zhang, Y. (2020). Oxidative stress and diabetes: Antioxidative strategies. Frontiers of Medicine, 14(5), 583–600. https://doi.org/10.1007/s11684-019-0729-1
- Zimmet, P., Alberti, K. G., Magliano, D. J., & Bennett, P. H. (2016). Diabetes mellitus statistics on prevalence and mortality: Facts and fallacies. Nature Reviews Endocrinology, 12(10), 616–622. https://doi.org/10.1038/nrendo.2016.105