ABSTRACT
The fortification of buckwheat (Fagopyrum esculentum Moench) with rheological characteristics, nutritional properties, and formation of contaminants in wheat flour-based flaky rolls was studied. Higher content of buckwheat flour increased the antioxidant capacity, and phenolic, protein, fiber, rutin, and quercetin contents, leading to the development of a nutritionally improved product. The contents of the process contaminants, for example, the acrylamide, hydroxymethylfurfural (HMF), and furfural ranged from 147.25–262.19 μg/kg, 19.17–73.56 mg/kg, and 1.34–4.55 mg/kg, respectively, when buckwheat was added in the range of 0–30%. Parallelly, the production levels of methylglyoxal (MGO) and glyoxal (GO) were 4.09–23.55 and 0.69–2.05 mg/kg in the buckwheat substitution range of 0–30%. Shortly, even though incorporating 10% buckwheat flour had the best sensory scores, 20% was the most suitable substitution rate in terms of controlling the contaminants produced during processing while enhancing the nutritional properties of flaky rolls.
1. Introduction
Cereals are a critical part of global food culture. Cereals are a food category or that offers significant nutritional benefits, good health, and a positive impact on the body (Nayik et al., Citation2023). Common buckwheat (Fagopyrum esculentum) and tartary buckwheat (Fagopyrum tataricum) are widely used because of their high agricultural value. These pseudocereals are generally acknowledged as good sources of nutritional and valuable compounds such as proteins, lipids, dietary fiber, and minerals (Bastida et al., Citation2015). Buckwheat proteins mainly consist of albumin and globulin, with some prolamin and gluten (Zhu, Citation2021). The high biological availability of these proteins is because of their perfectly proportioned amino acid composition and richness in lysine and arginine residues. In addition, buckwheat peptides have great potential as antimicrobial, antihypertensive, antidiabetic, and antiaging agents owing to their health-promoting properties (Graziano et al., Citation2022; Zhu, Citation2021).
Development of functional foods is an interesting topic in the food industry. However, understanding the effects of processing is crucial for optimizing the conditions and obtaining functional foods with superior bioactive compounds (Bastida et al., Citation2015). Buckwheat has attracted attention as a promising functional food owing to its functionalities and contents, such as proteins, flavonoids, phytosterols, and fibers (Graziano et al., Citation2022). Many functional buckwheat-derived products include buckwheat-improved confectioneries, energy bars, noodles, tea, tarhana, sprouts, and buckwheat honey (Bilgiçli, Citation2009; Deng et al., Citation2018; Guo et al., Citation2017; Kaur et al., Citation2015; Kim et al., Citation2004; Kowalski et al., Citation2022; Ma et al., Citation2013). Buckwheat noodles presented a good antioxidant capacity which evaluated by the ferric reducing antioxidant power, DPPH free radical scavenging activity, and ABTS•+ scavenging assay due to its greater level of total phenolics (182.65–221.27 mg GA eq./100 g d.w.) and flavonoids (183.33–218.69 mg rutin eq./100 g d.w.) (Ma et al., Citation2013). A previous study demonstrated that buckwheat honey has better cellular antioxidant and antibacterial activities against Staphylococcus aureus and Pseudomonas aeruginosa than manuka honey (Deng et al., Citation2018). Buckwheat is an excellent food ingredient with a high nutritional value.
Incorporating specific ingredients to formulate certain foods may be advantageous from a nutritional and health perspective. However, some final products may have negative features or attributes. A previous study reported that biscuit samples containing 95:5 wheat: velvet bean (Mucuna pruriens) seed flour had the highest amino acid contents for methionine (4.68 mg/100 g) and lysine (6.69 mg/100 g). Nevertheless, this flour has an adverse impact on the sensory qualities of biscuits (Ezegbe et al., Citation2023). Incorporating chia (Salvia hispanica L.) in a formulation of wheat-based biscuits enhances the antioxidant capacity, as well as phenolic compounds, proteins, fibers, and polyunsaturated fatty acid contents of the final product. Despite the increase in nutritional quality, acrylamide, hydroxymethylfurfural (HMF), and furfural contents also increase (Mesías et al., Citation2016). In addition, a previous study showed that substituting black rice (Oryza sativa L.) flour in flaky rolls also increased the content of asparagine, acrylamide, HMF, furfural, methylglyoxal (MGO), and glyoxal (GO) in flaky rolls, even though adding black rice flour to flaky rolls effectively increased crude protein, total dietary fiber (TDF), soluble dietary fiber (SDF), and insoluble dietary fiber (IDF) content (Lu et al., Citation2022). Thus, although nutritional properties can be enhanced by incorporating certain ingredients into the final product, the increase in contaminant content and the extent of the negative effects should be carefully considered in terms of risk and benefit factors.
Egg is a ubiquitous ingredient in many commercially available foods; however, owing to IgE-mediated egg allergy and dietary restrictions, such as a lacto-ovo-vegetarian diet or vegan, investigation of egg-free products is challenging and a novelty to the food manufacturer (Csurka et al., Citation2022). Optimizing recipes and technological process parameters, as well as characterizing the final product in terms of sensory acceptance and probable functional properties, is vital for obtaining different buckwheat-based food products with health-promoting components (fiber, minerals, protein, and bioactive compounds). In this study, traditional egg biscuit – flaky rolls, known as Phoenix egg rolls in Macau and egg biscuits in Southeast Asian countries, were incorporated with buckwheat flour and rice protein powder as egg replacements to produce egg-free buckwheat-substituted flaky rolls. The effects of buckwheat flour supplementation on the rheological characteristics, nutritional properties, and production of process contaminants in flaky rolls were investigated.
2. Materials and methods
2.1. Materials
Buckwheat (Fagopyrum esculentum) was cultivated (2020) at a local farm in the Erlin Township, Changhua County, Taiwan. Buckwheat was milled and passed through a 120-µm sieve and stored in an air-tight aluminum container before further analysis. The analysis of moisture (Association of Official Agricultural [AOAC] Official Method 925.10), crude protein (AOAC Official Method AOAC 920.87), and ash content (AOAC Official Method AOAC 923.03) of the flour followed the AOAC method (1995). The moisture, crude protein, and ash contents of buckwheat flour was 11.17%, 12.38%, and 2.14%, respectively.
Wheat flour used in this study was supplied by Chi-Fa Enterprise (Taichung, Taiwan). The moisture, crude protein, and ash content of the wheat flour were 13.46%, 11.39%, and 0.31%, respectively. Rice protein powder (67% protein, dry weight) was purchased from Vedan Enterprise (Taichung, Taiwan). The rice protein contained 4.75%, 8.63%, and 7.0% moisture, fat, and carbohydrates, respectively. Other basic baking ingredients such as margarine, sugar powder, corn flour, lecithin, and salt were purchased from a local market in Yuanlin City, Changhua County, Taiwan. All chemicals used in this study were purchased from Sigma-Aldrich. (St. Louis, MO, U.S.A.) and Merck (Darmstadt, Germany). All chemicals used was American Chemical Society certified analytical grade.
2.2. Flaky rolls preparation
Flaky rolls were prepared by designing three different wheat/buckwheat flour groups with ratios of 100:0 (control, W), 90:10 (10 BW), 80:20 (20 BW), and 70:30 (30 BW), as indicated in . Margarine and sugar powders were mixed (Electrolux EHM3407, Electrolux Thailand Co Ltd, Bangkok, Thailand) until they were fluffy. Next, wheat flour, buckwheat flour, rice protein, corn flour, lecithin, salt, and deionized water were added to the mixture. All ingredients were mixed to form a smooth-flowing batter. The pasting and rheological characteristics of the dough were directly analyzed. For the flaky roll preparation, the circular metal pan was preheated to 150°C, 15 g of the batter was spread on the pan, the lid was closed, and the pan was baked for 30 s. The flaky roll was folded by one quarter on each side and then rolled around a flat rod to form a flat-shaped flaky roll.
Table 1. Flaky egg-free roll formulation fortified with rice protein and different proportions of buckwheat flour.
2.3. Pasting properties
A Rapid-Visco Analyzer (Model RVA-3D, Newport Scientific, Sydney, Australia) was used to analyze the pasting properties of the flour samples in triplicate. A flour sample (3 g) and distilled water with a flour: water ratio of 1:7 were prepared in the test canister prior to entering the heating-cooling cycle. The starch slurry was equilibrated at 50°C for 1 min at the beginning, heated to 95°C at a rate of 5°C/min, and held for 5 min. Next, the mixture was cooled to 50°C (5°C/min) and the temperature was maintained at 50°C for 2 min. The pasting profiles of the samples, including the peak viscosity (BU), breakdown (BU), setback (BU), and final viscosity (BU), were tabulated from the viscoamylograph of the Rapid Visco Analyzer curves.
2.4. Farinograph measurements
The farinographic mixing properties of dough, which corresponded to the standard method of the American Association for Clinical Chemistry (AACC) 54–21 (2000), were measured using a Brabender farinograph (Brabender farinograph-E, Brabender, Duisburg, Germany). Wheat flour was substituted with different quantities of buckwheat flour at 0%, 10%, 20%, and 30%, and was formulated and prepared by weighing the formulated flour at 100 g and mixing evenly for 5 min. Next, water was added to 500 Brabender units (BU) line and the graphs of the farinograph were agitated to dramatically change and prolong the time to 12 min. The water absorption (%), dough development time (min), breakdown time (min), stability (min), and peak time (min) were recorded. Water absorption (%) was defined as the percentage of water absorbed by the dough to the 500 BU line. Development time is the amount of time required to mix dough for optimum development. Breakdown time was defined as the time to the nearest half minute from the start of the mixing until there was a decline of 30 Brabender Units from the peak point. Stability (min) was defined as the changes between arrival and departure times. The peak time (min) is expressed as the time required to reach the peak of the curved band.
2.5. Extensograph measurements
The extensographic characteristics of formulated dough samples were evaluated by using the Brabender extensograph (Brabender extensograph-E, Brabender, Duisburg, Germany) which referred to the standard method of the AACC 54–10 (2000). For farinographic analysis, the samples were divided into two parts (150 g each). The sample was then passed through the balling and mold units of the extensograph. The sample was stretched after 45 min of rest in a fermentation cabinet. After fermentation, balling and molding were performed. The test was performed in triplicates. The results are expressed as the maximum resistance to extension (BU). Extensibility (cm) was defined as the distance traveled by the recorder paper from the moment the hook touched the test piece until it ruptured the test piece.
2.6. Chemical composition analysis
The nutritional and proximate composition of flaky rolls with buckwheat flour, including moisture, total sugar, crude protein, crude lipid, total dietary fiber (TDF), soluble dietary fiber (SDF), insoluble dietary fiber (IDF), ash, free sugar, and cholesterol content, were examined in independent three replicates and correlated with the official methods of AOAC 935.39. The moisture content was studied using the hot-air oven method; ash content was evaluated using the method of incinerating samples in a muffle furnace at 600°C; crude protein content was measured using the Kjeldahl method with conversion factor of 5.25, which is suitable for buckwheat; fat content was analyzed using the acid hydrolysis method; cholesterol content was verified using the digitonin method, and the fiber content was calculated using the enzymatic – gravimetric method.
2.7. Characteristics and texture analysis
The length (cm), width (cm), and thickness (cm) of the buckwheat flaky roll were measured using a Vernier caliper. The textural properties of hardness (N), fracturability (mm), springiness (mm), cohesiveness, and adhesiveness (N.S) were measured using a 3-point bend rig (HDP/3 PB) set up with a 5-kg load cell and a heavy-duty platform of the Brookfield CT3 Texture Analyzer (New York, U.S.A.). The test speed was set at 3.0 mm/s and the trigger force was set automatically at 50 g. The hardness value appeared as the maximum force applied, whereas the fracturability value represented the distance at the point of break.
2.8. Color assessment
The color of the flaky roll samples was evaluated using a Color Meter model ZE-2000 (Nippon Denshku Industries, Tokyo, Japan). The L* value was used as the lightness measurement, a* value representing the greenness-redness, and b* value considered as blueness-yellowness were examined to verify the incorporation of buckwheat flour into the quality (color) changes. A standard black and white ceramic tile was used to calibrate the instrument before measurement. Color measurements were performed at room temperature in triplicate.
2.9. Sensory evaluation
A total of 31 panelists, including 16 females and 15 males (aged from 20–30 years) from the Department of Food and Nutrition, Providence University, were randomly selected to participate in the sensory evaluation session. The sensory test was conducted in a standard sensory laboratory at room temperature and strictly complied with the GB/T 20,980–2007 and ISO 4121 criteria to assess the organoleptic characteristics of the flaky roll supplemented with buckwheat flour in terms of appearance, aroma, taste, texture, and total acceptability. All the panelists needed to attend the related tutorial and training classes for at least 6 h before carrying out the sensory test so that they could professionally identify and comprehend the rating scales of each attribute. Flaky roll samples were cut to a size of 5.0 cm2 and randomly placed on white disposable polyform plates, which labeled with a random 3-digit number. All the samples were freshly prepared and covered with food wrap until testing. The flaky rolls were assessed by quantitative descriptive analysis involving a 6-point interval scale with scores from 0 to 5 for each attribute, with 0 indicating no value and 5 indicating extremely strong values.
2.10. Total phenolic content (TPC) analysis
Phenolic compounds in foods play a vital role as a health protecting factor, hence it is important to determine the TPC contain in the food (Chan et al., Citation2020). The TPC of the samples was determined by using the Folin-Ciocalteu method, with gallic acid act as the standard compounds and double-distilled water (ddH2O) was used as the blank. First, the test sample (10 g) was extracted with 200 mL 80% methanol, followed by filtration and evaporation to dryness using a rotary evaporator at 40°C. Next, 1 mg/mL of methanolic extractant was mixed with Folin – Ciocalteu phenol reagent (0.5 mL) and 5 mL of 5% sodium carbonate (Na2CO3) solution to form the reaction mixture. The reaction mixtures were diluted with distilled water to 25 mL and allowed to stand for 60 min. The absorbance of the blank was measured at 750 nm wavelength. TPC was calculated by comparison with a standard curve of gallic acid, and the results were expressed as milligram gallic acid equivalent (GAE) per gram dry weight (mg GAE g−1 DW).
2.11. Rutin and quercetin content analysis
Quercetin and rutin contents were assayed by HPLC (SPD – M10A VP Shimadzu, LC–8A pump, Japan) using a Phenomenex C18 column (250 mm × 4.6 mm, 5.0 μm), as previously described (Qin et al., Citation2010; Sun et al., Citation2018; Tien et al., Citation2018). Methanol (solvent A) and Milli-Q water with phosphoric acid (solvent B; pH 3.0) were used for HPLC. The column temperature was set to 35°C at a flow rate of 1.0 mL/min, and quercetin and rutin were detected at 290 nm and separated using a linear gradient elution program. The gradient program was as follows: initial 10% B; 0–5 min, 10–45% B; 5–10 min, 45% B; 10–30 min, 65% A, 30 min 10% B. The results are expressed as milligrams of individual standards in 1 kg of sample (mg/kg DW).
2.12. Total antioxidant capacity by DPPH
The free radical scavenging activity of the sample and standard was assessed based on the free radical scavenging effect of the stable DPPH free radical activity. Gallic acid was used as the standard solution. DPPH was diluted with methanol to a concentration of 0.1 mM. Two mL of 0.1 mM DPPH was mixed with 1 mL of the sample solution or standard solution. The reaction mixtures were kept in the dark for 30 min, and the absorbance was measured using a spectrophotometer (SH-U830 Vis-Spectrophotometer, Shishin Technology Co., Ltd., Taipei, Taiwan) at a wavelength of 518 nm. The analysis was performed in triplicate.
2.13. Total antioxidant capacity by the oxygen radical antioxidant capacity (ORAC)
ORAC analysis using fluorescein (FL) as the fluorescent probe was performed as previously described with some modifications (Lu et al., Citation2022). The 96-well microplates were used in this study, and the absorbance was read at wavelengths of 485 nm for excitation and 530 nm for emission. The reaction was performed at 37°C, initiated by the thermal decomposition of 2,2-azobis (2-amidinopropane) dihydrochloride (AAPH) in 75 mM phosphate buffer (pH 7.0). To each well of the 96 -wells was added, in 50 μL of 78 nM FL and 50 μL of sample, which was phosphate buffer, 20 μM Trolox was used as the standard, and 25 μL of 221 mM AAPH was added. Before adding AAPH, the plates were heated at 37°C for 15 min to avoid variations in measurement among wells due to the low conductivity of the 96-well plates. Fluorescence was recorded as the relative fluorescence intensity (FI%) every 5 min until the fluorescence value was less than 5% of the initial reading. Each analysis and measurement was performed in triplicate.
2.14. Determination of acrylamide
A ground flaky roll sample (1 g) was extracted with 20 mL of 10 mM formic acid in 20 mL distilled water and vortexed for 5 min. The supernatant collected from the extraction was run through a preconditioned Oasis MCX solid-phase extraction (SPE) cartridge before the pure extract was investigated using LC-MS/MS. The acrylamide content of the test samples was studied using a Waters Acquity H Class UPLC system (Millford, MA, U.S.A.) equipped with a TQ detector with electrospray ionization operated in positive mode. Chromatographic separations were performed at a flow rate of 0.5 mL/min on a Thermo Scientific Hypercarb column (100 × 2.1 mm × 3 µm) with formic acid solution as the mobile phase for 15 min of isocratic elution. The column was equilibrated at 50°C, whereas the electrospray source had a capillary voltage of 2.00 kV, cone voltage of 23 V, extractor voltage of 4 V, source temperature of 120°C, desolvation temperature of 400°C, and desolvation gas (nitrogen) flow of 900 L/h. Calibration curves were constructed with quantifications ranging from 1 to 40 ng/mL (1, 2, 5, 10, 20, and 40 ng/mL). The results are expressed as μg/kg.
2.15. Determination of asparagine
The sample extraction method was the same as that described in Section 2.14. Carrez I and II solutions were added to the extraction mixture and centrifuged at 15,000 × g for 15 min. The supernatant was further diluted with an equal volume of acetonitrile and centrifuged (15,000 × g for 15 min). The supernatant was filtered by using a 0.45 µm nylon filter and collected in a vial for further analysis. A Waters Acquity UPLC system coupled with a triple quadrupole detector was used to determine the free asparagine content of the samples. Thermo Scientific Syncronis HILIC column (100 × 2.1 mm × 1.7 µm) was used with a gradient mixture of Solvent A, which is 5 mM ammonium formate in water with formic acid, and Solvent B which is prepared by 5 mM ammonium formate in water: acetonitrile (v/v 1:9) with formic acid, as the mobile phase. Elution was carried out at 40°C with a flow of 0.7 mL/min, using the following gradients: at the beginning of the analysis, the mobile phase gradient was conditioned with 0% Solvent A (100% Solvent B), then steadily increased in Solvent A 80% (20% Solvent B) for 8 min, held for 5 min, then dropped steadily to the initial conditions (0% Solvent A, 100% Solvent B) for 2 min and detained for 10 min. The condition for the electrospray source was with the following parameters: capillary voltage (3.5 kV); cone voltage (20 V); extractor voltage (3 V); source temperature (120°C); desolvation temperature (370°C), and desolvation gas (nitrogen) with flow of 900 L/h. Calibration curves using theanine as a standard were prepared at concentrations from 0.05 to 3.0 mg/L. The final results are expressed as mg/kg.
2.16. Determination of hydroxymethylfurfural (HMF) and furfural
The ground sample (500 mg) was immersed in 5 mL deionized water, 0.25 mL potassium ferrocyanide (15% w/v), and 0.25 mL zinc acetate (30% w/v) solutions in a centrifuge tube. The mixture was centrifuged at 15,000 × g for 15 min at 5°C. The collected supernatant was filtered through a 0.45-µm syringe filter prior to HPLC (Shimadzu, Kyoto, Japan) analysis. The analysis was performed on a Synergy 4 µm Hydro-RP 80A (250 × 4.6 mm Phenomenex) column with a mobile phase of a mixture of acetonitrile and water (5% v/v) at a flow rate of 1 mL/min under isocratic conditions. The UV detector was set at a wavelength of 280 nm, and HMF was quantified using the external standard method within a concentration range of 0.025–75 mg/L. All analyses were performed in triplicate, and the results were expressed as mg/kg samples.
2.17. Determination of di-carbonyl compounds, glyoxal (GO) and methylglyoxal (MGO)
A reverse-phase high-performance liquid chromatography (RP-HPLC) procedure furnished withDAD detector at a wavelength of 315 nm was used to analyze the GO and MGO contents, which corresponded to the quinoxaline (Q) and 2-methylquinoxaline (2-MQ) contents. An LC system (Kyoto, Japan) equipped with an ACEC18 column (5 μm, 250 × 4.6 mm, Advanced Chromatography Technologies, Aberdeen, UK), low-pressure gradient former, pump and DAD detector was utilized, and a mixture of 0.5% (v/v) acetic acid in water and methanol (40:60 v/v) was used to carry out the isocratic elution. The Q and 2-MQ standard solutions were prepared in ultrapure water at concentration of 0.16–5.0 mg/mL.
2.18. Statistical analysis
Experimental data are presented as the mean ± standard deviation (SD) of independent triplicate experiments. All data were analyzed using one-way analysis of variance (ANOVA) and Tukey’s test to verify the significant differences of the data at P < .05. The Microcal Origin program version 2021 (Origin Lab Corp., Northampton, MA) was used.
3. Results and discussion
3.1. Rheological characteristics and pasting properties of dough
provides information about the rheological characteristics (farinographic and extensographic characteristics) and pasting properties of the dough samples with various proportions of buckwheat flour. shows the farinograph characteristics of sample dough containing different proportions of buckwheat flour. Farinographics were used to assess the baking quality and performance of the wheat flour dough. Farinographics record the resistance to deformation or consistency of dough mixed with flour and water. Farinographics assesses the effect of flour on dough handling properties and establishes quality control measures to appropriately handle wheat crop changes. The water absorption (%) of the dough upsurged from 65.29% ± 4.53% (W) to 80.63% ± 4.21% (30 BW) when buckwheat was incorporated from 0% to 30%. Dough development time (min) increased from W (1.87 ± 0.32 min) to 20BW (2.52 ± 0.09 min), but decreased at 30BW (2.42 ± 0.11 min). By contrast, the breakdown time (min), stability (min), and peak time (min) decreased sharply from W to 30 BW. Water absorption describes the amount of water added to balance the farinograph curve at 500 BU and is expressed as a percentage of flour. The water absorption rate increased when buckwheat flour was substituted for wheat flour. This may be because the high fiber content (TDF, SDF, and IDF content) of the flaky rolls increased from W to 30 BW when the proportion of added buckwheat increased (). A higher buckwheat flour content decreased the time (min) in which the dough maintained its standard consistency during kneading (stability time). The farinograph stability time is correlated with the strength of the flour, and short stability times are more applicable for biscuit products because of shorter mixing times. In short, substituting buckwheat flour led to a significant change in dough farinographic parameters, causing the water absorption rate and dough development time to increase, whereas the breakdown time, peak time, and stability time declined.
Figure 1. Effect of flaky rolls fortified with different proportions of buckwheat flour on farinograph characteristics: (a) control (W), (b) 10 BW, (c) 20 BW, and (d) 30 BW.
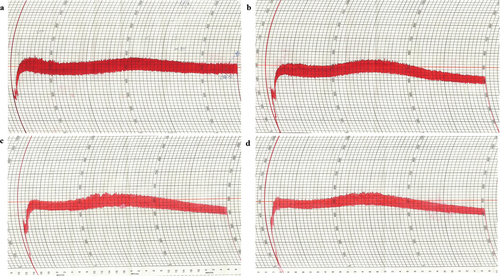
Table 2. Rheological characteristics and pasting properties of wheat dough with different proportions of buckwheat flour.
Table 3. Nutritional composition (based on dry weight) of flaky rolls incorporated with different proportions of buckwheat flour.
An extensograph is important for measuring the quality of flour and the stretching behavior of dough. Extensional properties, which determine dough expansion during proofing and baking, directly affect the final product’s volume and texture quality. The maximum resistance to extension (BU) of the extensographic properties increased from W to 30 BW from 45 to 135 min. The 10BW and 20BW samples increased from 45 min to 90 min and then declined at 135 min. Incorporating buckwheat flour lowered the extensibility (cm) of the 10BW, 20BW, and 30 BW samples from 45 to 135 min. However, the extensibility of the W sample also showed a downward trend from 45 to 90 min but increased at 135 min.
The pasting properties were determined using a Rapid Visco Analyzer, a rotational (stirring) rheometer that measures the apparent viscosity of starch-containing suspensions and flour/water mixtures under variable and controlled heating (cooking), cooling, and shear stress conditions. In this study, the incorporation of buckwheat flour decreased peak viscosity, breakdown, and setback values. However, the final viscosity fluctuated. The peak viscosity (highest viscosity reached during pasting) decreased from 858.27 ± 25.93 BU (sample W) to 765.01 ± 19.45 (10BW), 743.75 ± 22.24 BU (20BW), and 657.75 ± 19.29 BU (30BW). The final viscosity, which represent the viscosity at the end of the pasting cycle, varied from 1192.45 ± 25.13 BU (sample W) to 1055.96 ± 27.25 BU (10BW), 1066.82 ± 33.29 BU (20BW), and 1007.31 ± 21.29 BU (30BW). The peak viscosities of the tested formulations were significantly different from each other. The peak viscosity of the composite flour decreased with increasing buckwheat flour contents, which may have been due to the higher protein and fiber content in the flaky flour (). A previous study concluded that the complex components of buckwheat flour, such as proteins, fibers, pectin, hemicellulose, cellulose, and rutin, along with the interactions between these components, may disturb their viscosity characteristics (Sun et al., Citation2018). Furthermore, starch is the main constituent of buckwheat flour; therefore, the pasting properties of flour are affected by its starch fraction (Sun et al., Citation2018).
3.2. Nutritional composition analysis
describes the nutritional composition of the flaky rolls incorporated with various proportions of buckwheat flour. The moisture content of the flaky rolls increased as more buckwheat flour was substituted, with a value from 2.85 to 4.03 g/100 g. The crude protein also increased as more buckwheat flour was added, and 30BW had the highest value (25.44 ± 1.68 g/100 g) and the control sample (W) had the lowest value (20.38 ± 0.57 g/100 g). Previous studies have shown that incorporating rice protein into flaky rolls effectively boosts the protein content (Chan et al., Citation2020; Lu et al., Citation2022). Plant-based proteins have been developed to address consumer demand and sustainability of future food supplies, and the market has grown exponentially (Wen et al., Citation2020). Rice protein isolate is a hypoallergenic protein that is frequently used in infant formulas and diets for patients with allergies (Amagliani et al., Citation2017). A related study reported that the protein content of buckwheat flour is 13.9 g/100 g d.w., which is superior than that of maize flour (8.3 g/100 g d.w.) and rice flour (6.9 g/100 g d.w.) (De Arcangelis et al., Citation2020). In addition, buckwheat contains proteins with a balanced amino acid composition and is distinguished by a high essential amino acid content, such as lysine, which is a rare amino acid in common cereals such as wheat, maize, and rice (Abdel-Aal & Wood, Citation2004). Therefore, incorporating buckwheat flour and adding rice protein significantly increased the protein content of the flaky rolls.
Incorporating buckwheat flour lowered the crude lipid content of flaky rolls. The crude lipid contents in the buckwheat flaky rolls ranged from 11.03 ± 0.25–12.03 ± 0.05 g/100 g. A previous study revealed that six fatty acids – palmitic (C16:0), stearic (C18:0), oleic (C18:1), linoleic (C18:2), linolenic (C18:3), and gondoic (C20:1) – were the most prevalent among the 15 fatty acids found in the three buckwheat cultivars (Tien et al., Citation2018). Furthermore, common buckwheat had the highest percentage of oleic acid (43.94%), followed by tartary buckwheat (39.49% oleic acid), and tetraploid tartary buckwheat (35.65% oleic acid) (Tien et al., Citation2018). The 30BW flaky rolls showed the highest TDF, SDF, and IDF. TDF, SDF, and IDF increased markedly with the addition of buckwheat, which contains a high amount of fiber. Dietary fiber is the principal bioactive constituent and comprises approximately 40% buckwheat bran, including IDF and SDF (Rachman et al., Citation2020). Raising the content of buckwheat flour in the flaky rolls caused a significant difference in ash content from 1.57 ± 0.09 g/100 g (W) to 1.87 ± 0.14 g/100 g (30BW). A related study reported that the flour of common buckwheat cultivars contains 1.33%- 3.09% ash (Qin et al., Citation2010).
In short, substituting buckwheat flour effectively reduced the total sugar and crude lipid content, whereas crude protein, TDF, SDF, and IDF increased in the flaky rolls.
3.3. Dimensional quality and textural analysis
provides information on the characterization, textural properties, and color analysis of the flaky rolls substituted with various proportions of buckwheat flour. The appearance of buckwheat-substituted flaky rolls are shown in . A positive correlation was detected between farinographic stability time and volume of flaky rolls. The lower the stability time (), the smaller is the volume of the flaky roll (). Thus, incorporating buckwheat flour significantly changed the textural properties of the flaky rolls compared to the control sample, but only a slight difference was observed among the 10BW, 20BW, and 30 BW groups. Substituting buckwheat flour lowered the fracturability, springiness, and adhesiveness of the flaky rolls. Nevertheless, substituting buckwheat flour increased its hardness and cohesiveness. The high dietary fiber content of buckwheat competes with water to enfold the starch granules distributed in the gluten network, thus prohibiting the protein molecules from being crisscrossed and disturbing the formation of the spatial network (Jia et al., Citation2020). The composite matrix of protein aggregates, lipids, and sugars fixed within ungelatinized starch granules affects the hardness of flaky rolls. If the hardness of a flaky roll is too high, it will affect the taste and cause biscuits to be soggy. However, if the hardness of the flaky roll is too low, it breaks easily (Lu et al., Citation2022). Cohesiveness describes the internal binding force required to form a sample, and reveals the strength of the interaction between molecules within a sample or between various structural elements. Cohesiveness also corresponds to the ability of a sample to resist damage and maintain integrity. The cohesiveness of the flaky rolls increased when buckwheat flour was substituted, resulting in crispy flaky rolls that were easily crushed into pieces, and could be transported and stored conveniently. A related study indicated that using isolated Douglas dietary fiber (DDF) in supplemented biscuits showed that increasing the DDF in flour affected the physical characteristics of the biscuits by increasing the hardness and decreasing the diameter, thickness, and spread ratio (Aboshora et al., Citation2019). The color development of the flaky rolls depended on the sugar and protein content of the raw flour. Sample 30 BW, which contained higher amounts of protein and sugar (), had a darker yellow appearance, as observed in . Color is one of the most crucial sensory attributes of food and its appearance influences consumers’ decisions. Color development in protein-rich biscuits depends mainly on the Maillard reaction, and caramelization occurs when the protein reacts with sugars during baking (Pokharel et al., Citation2023). Additionally, the dextrinization of starch in wheat flour may be responsible for color development. Therefore, the results of the buckwheat-substituted flaky rolls corresponded with those of Mieszkowska and Marzec (Mieszkowska & Marzec, Citation2016), showing that color is critically affected by polydextrose and protein-rich ingredients owing to the progression of the Maillard reaction, leading to deterioration in lightness (L* value) and increased redness (a* value).
Table 4. Characterization and textural properties of flaky rolls containing different proportions of buckwheat flour.
3.4. Sensory evaluation
The sensory evaluation of the buckwheat-fortified flaky rolls is shown in . The organoleptic characteristics of the flaky rolls substituted with buckwheat flour were investigated by 31 trained panelists. Sample 10BW had the highest overall acceptability scores for appearance, aroma, and taste. No significant difference in the mean texture score (4.29 ± 0.33) was observed with the control sample (4.21 ± 0.32). Sample 30BW contained a higher amount of fiber (), which presented a higher hardness () and could affect mouthfeel and impact overall acceptance. Thus, a suitable amount of buckwheat for incorporation into flour should be considered in future product development and marketing.
3.5 Total phenolic content, rutin, and quercetin contents, and in vitro antioxidant capacity
Buckwheat contains high amounts of phenolic compounds, which contribute to its antioxidant, anti-inflammatory, and anti-hypertensive properties (Zhu, Citation2021). summarized the in vitro antioxidant capacity of the flaky rolls fortified with various proportions of buckwheat flour: (a) total phenolic content (TPC), (b) rutin content, (c) quercetin content, (d) DPPH scavenging activity, and (e) ORAC assay. TPC, rutin, quercetin, DPPH and ORAC activity in the W, 10, 20, and 30BW groups increased markedly from 17.58 ± 0.68 to 82.54 ± 1.23 mg GAE/100 g, 0.01 ± 0.00 to 0.78 ± 0.14 mg/kg, 0.02 ± 0.00 to 0.69 ± 0.15 mg/kg, 7.98 ± 0.15 to 9.96 ± 0.25 µmol TEAC/g, and 57.79 ± 1.17 to 139.25 ± 14.62 µmol TEAC/g, respectively. The addition of buckwheat flour increased TPC, rutin, and quercetin contents, as well as antioxidant activities. These data indicate a strong relationship between the antioxidant capacity and TPC of buckwheat. Moreover, rutin and quercetin in buckwheat contribute to the antioxidant capacity of flaky rolls. A previous study reported that the high antioxidant capacity of buckwheat is associated with high polyphenol content, particularly the high content of flavonoids, such as rutin, quercetin, and phenolic compounds (Tien et al., Citation2018; Van Hung & Morita, Citation2008).
Table 5. In vitro antioxidant capacity of flaky rolls fortified with different amount of buckwheat flour.
Flavonoids and their glycosides are a major class of secondary metabolites in plant. They are widely distributed natural compounds of special interest because of their antioxidant characteristics and potential to prevent fatigue, diabetes mellitus, oxidative stress, and Parkinson’s disease (Kreft, Citation2016; Zhang et al., Citation2017). Flavonoids are plant-derived phenolic compounds. Quercetin is an aglycone formed after enzymatic degradation of rutin by rutinosidase (Gullon et al., Citation2017; Kawabata et al., Citation2015). Quercetin from the degradation of rutin in buckwheat grains partially, inhibits the glucosidase in the intestine, which is able to maintain normal blood glucose levels (Kawabata et al., Citation2015). Moreover, a previous study also revealed that rutin exhibited antiviral effects against the Sars-CoV-2 virus (Luthar et al., Citation2020). In short, the consumption of rutin-rich-buckwheat-fortified flaky rolls may be a beneficial approach for the enhancement of human nutrition, because of the known protective health effects of rutin.
3.6 Free asparagine, acrylamide, HMF, furfural, methylglyoxal, and glyoxal in the flaky rolls
shows the acrylamide, asparagine, HMF, furfural, methylglyoxal, and glyoxal contents in flaky rolls supplemented with different proportions of buckwheat flour. The acrylamide content increased from 147.25 ± 11.26 (W) to 167.83 ± 21.25 (10 BW), and from 229.19 ± 32.19 (20 BW) to 262.19 ± 36.41 (30 BW) µg/kg as buckwheat content increased. The acrylamide precursors and the asparagine content increased from 355.12 ± 17.25 to 516.89 ± 33.68 mg/kg as high concentration of buckwheat flour was added. Food processing processes that involve high temperatures cause the Maillard reaction to occur. The most common Maillard route by which acrylamide is formed is consider to be a heated reaction with asparagine and α-hydroxycarbonyl compounds, such as the reducing sugars (Mesías et al., Citation2019). Acrylamide is a carcinogen, and in 2018, the European Union (EU) legislation announced the benchmark level (BML) in biscuits and cookies to be below 350 parts per billion (ppb) (Mesías et al., Citation2019; Mihai et al., Citation2020). Acrylamide levels beyond the EU have been subject to this benchmarking system since 2018, when manufacturers are tasked with aiming for acrylamide levels as low as reasonably achievable. If the BML is over the limit, the producer must audit and revise their mitigation measures to lower the level (Mesías et al., Citation2019; Mihai et al., Citation2020). The higher the asparagine content, the higher the amount of acrylamide formed in the flaky rolls substituted with buckwheat flour. The appearance of acrylamide in biscuits prepared from different wholegrain flours depends on the free asparagine content and the baking conditions (Žilić et al., Citation2020). Hence, selecting the proper processing procedure and cereal species with low levels of acrylamide precursors can reduce the acrylamide content in the final product. Similar results were observed for a food industry method for reducing the acrylamide content in the final product (Žilić et al., Citation2020).
Table 6. Acrylamide, asparagine, HMF, furfural, methylglyoxal, and glyoxal contents in flaky rolls incorporated with different proportions of buckwheat flour.
Same results were observed for HMF, furfural, methylglyoxal, and glyoxal contents. HMF content increased rapidly from 19.17 ± 2.16 (W) to 73.56 ± 4.11 (30BW) mg/kg as the proportion of buckwheat flour increased. Furfural (furaldehyde) content increased from 10BW (2.63 ± 0.32 mg/kg) to 20BW (3.17 ± 0.19 mg/kg), and 30BW (4.55 ± 0.09 mg/kg) compared to the control (1.34 ± 0.02 mg/kg). These results correspond to those of a previous study in which wheat-based biscuits were substituted with different quantities of chia flour (Mesías et al., Citation2016). Chia flour added to wheat-based biscuits not only boosted the nutritional properties but also increased acrylamide, HMF, and furfural in chia-enriched biscuits (Mesías et al., Citation2016). However, the acrylamide, HMF, and furfural contents of buckwheat-substituted flaky rolls were lower than those of the chia-enriched biscuits, in which the acrylamide, HMF, and furfural contents were 151–1,188 μg/kg, 22.8–71.4 mg/kg, and 1.3–5.6 mg/kg, respectively (Mesías et al., Citation2016). Buckwheat-substituted flaky rolls also demonstrated higher levels of GO and MGO than the control. MGO content ranged from 4.09 ± 0.52 to 23.55 ± 3.38 mg/kg, while GO increased from 0.69 ± 0.02 to 2.05 ± 0.4 mg/kg. These reactive carbonyl compounds are produced by the degradation of sugars during caramelization and lipid oxidation (Lu et al., Citation2022; Mesías et al., Citation2016). Previous related studies have also pointed out that di-carbonyl compounds are primarily subjected to the formation of toxic Maillard reaction products (EFSA, Citation2015). Hence, the appropriate amount of buckwheat flour should be carefully contemplated when designing new buckwheat-based flaky roll formulations.
4. Conclusion
The current study provides a practical solution for eliminating eggs from flaky roll recipes to minimize health-related problems of eggs, such as allergies, and to promote bakery products, excluding eggs as an ingredient. The flaky rolls made with buckwheat flour and RP as egg replacement had equally good textural, dimensional, and sensorial properties of flaky rolls compared to those of the control. Dough behavior during online processing indicates the excellent machinability of buckwheat flour. The results obtained from the farinographic, extensographic, and pasting properties, in addition to the nutritional assay, sensory evaluation, and texture analysis, demonstrated that the quality of buckwheat flaky rolls was superior to that of the control when comparing certain parameters. We conclude that incorporating buckwheat flour and RP as egg replacers in a flaky roll recipe enhances the rheological, nutritional, functional, and textural properties of the product. The results indicated that 20% buckwheat flour was the most suitable substitution.
Acknowledgments
The authors thank Min-Cher Food Company for providing the machine for flaky egg roll production.
Disclosure statement
No potential conflict of interest was reported by the author(s).
Additional information
Funding
References
- Nayik, G. A., Tufail, T., Anjum, F. M., & Javed Ansari, M. (2023). Cereal grains: Composition, nutritional attributes, and potential applications (1st ed.). CRC Press. https://doi.org/10.1201/9781003252023
- Abdel-Aal, E., & Wood, P. (2004). Specialty grains for food and feed. American Association of Cereal Chemists.
- Aboshora, W., Yu, J., Omar, K. A., Li, Y., Hassanin, H. A., Navicha, W. B., & Zhang, L. (2019). Preparation of doum fruit (Hyphaene thebaica) dietary fiber supplemented biscuits: Influence on dough characteristics, biscuits quality, nutritional profile and antioxidant properties. Journal of Food Science and Technology, 56(3), 1328–1336. https://doi.org/10.1007/s13197-019-03605-z
- Amagliani, L., O’Regan, J., Kelly, A. L., & O’Mahony, J. A. (2017). The composition, extraction, functionality and applications of rice proteins: A review. Trends in Food Science & Technology, 64, 1–12. https://doi.org/10.1016/j.tifs.2017.01.008
- Bastida, J. A., Piskula, M., & Zieliński, H. (2015). Recent advances in processing and development of buckwheat derived bakery and non-bakery products – a review. Polish Journal of Food and Nutrition Sciences, 65(1), 9–20. https://doi.org/10.1515/pjfns-2015-0005
- Bilgiçli, N. (2009). Effect of buckwheat flour on chemical and functional properties of tarhana. LWT-Food Science and Technology, 42(2), 514–518. https://doi.org/10.1016/j.lwt.2008.09.006
- Chan, Y.-J., Lu, W.-C., Lin, H.-Y., Wu, Z.-R., Liou, C.-W., & Li, P.-H. (2020). Effect of rice protein hydrolysates as an egg replacement on the physicochemical properties of flaky egg rolls. Foods, 9(2), 245. https://doi.org/10.3390/foods9020245
- Csurka, T., Varga-Tóth, A., Kühn, D., Hitka, G., Badak-Kerti, K., Alpár, B., Surányi, J., Friedrich, L. F., & Pásztor-Huszár, K. (2022). Comparison of techno-functional and sensory properties of sponge cakes made with egg powder and different quality of powdered blood products for substituting egg allergen and developing functional food. Frontiers in Nutrition, 9, 979594. https://doi.org/10.3389/fnut.2022.979594
- De Arcangelis, E., Cuomo, F., Trivisonno, M. C., Marconi, E., & Messia, M. C. (2020). Gelatinization and pasta making conditions for buckwheat gluten-free pasta. Journal of Cereal Science, 95, 103073. https://doi.org/10.1016/j.jcs.2020.103073
- Deng, J., Liu, R., Lu, Q., Hao, P., Xu, A., Zhang, J., & Tan, J. (2018). Biochemical properties, antibacterial and cellular antioxidant activities of buckwheat honey in comparison to manuka honey. Food Chemistry, 252, 243–249. https://doi.org/10.1016/j.foodchem.2018.01.115
- EFSA, E. (2015). Scientific opinion on acrylamide in food-EFSA panel on contaminants in the food chain (CONTAM). The EFSA Journal, 13(16), 4104.
- Ezegbe, C. C., Onyeka, J. U., & Nkhata, S. G. (2023). Physicochemical, amino acid profile and sensory qualities of biscuit produced from a blend of wheat and velvet bean (mucuna pruriens) flour. Heliyon, 9(4), e15045. https://doi.org/10.1016/j.heliyon.2023.e15045
- Graziano, S., Agrimonti, C., Marmiroli, N., & Gullì, M. (2022). Utilisation and limitations of pseudocereals (quinoa, amaranth, and buckwheat) in food production: A review. Trends in Food Science & Technology, 125, 154–165. https://doi.org/10.1016/j.tifs.2022.04.007
- Gullon, B., Lú-Chau, T. A., Moreira, M. T., Lema, J. M., & Eibes, G. (2017). Rutin: A review on extraction, identification and purification methods, biological activities and approaches to enhance its bioavailability. Trends in Food Science & Technology, 67, 220–235. https://doi.org/10.1016/j.tifs.2017.07.008
- Guo, H., Yang, X., Zhou, H., Luo, X., Qin, P., Li, J., & Ren, G. (2017). Comparison of nutritional composition, aroma compounds, and biological activities of two kinds of tartary buckwheat tea. Journal of Food Science, 82(7), 1735–1741. https://doi.org/10.1111/1750-3841.13772
- Jia, M., Yu, Q., Chen, J., He, Z., Chen, Y., Xie, J., Nie, S., & Xie, M. (2020). Physical quality and in vitro starch digestibility of biscuits as affected by addition of soluble dietary fiber from defatted rice bran. Food Hydrocolloids, 99, 105349. https://doi.org/10.1016/j.foodhyd.2019.105349
- Kaur, M., Sandhu, K. S., Arora, A., & Sharma, A. (2015). Gluten free biscuits prepared from buckwheat flour by incorporation of various gums: Physicochemical and sensory properties. LWT-Food Science and Technology, 62(1), 628–632. https://doi.org/10.1016/j.lwt.2014.02.039
- Kawabata, K., Mukai, R., & Ishisaka, A. (2015). Quercetin and related polyphenols: New insights and implications for their bioactivity and bioavailability. Food & Function, 6(5), 1399–1417. https://doi.org/10.1039/C4FO01178C
- Kim, S.-L., Kim, S.-K., & Park, C.-H. (2004). Introduction and nutritional evaluation of buckwheat sprouts as a new vegetable. Food Research International, 37(4), 319–327. https://doi.org/10.1016/j.foodres.2003.12.008
- Kowalski, S., Mikulec, A., Mickowska, B., & Buksa, K. (2022). Nutritional properties and amino acid profile of buckwheat bread. Journal of Food Science and Technology, 59(8), 3020–3030. https://doi.org/10.1007/s13197-022-05518-w
- Kreft, M. (2016). Buckwheat phenolic metabolites in health and disease. Nutrition Research Reviews, 29(1), 30–39. https://doi.org/10.1017/S0954422415000190
- Lu, W.-C., Cheng, Y.-T., Chan, Y.-J., & Li, P.-H. (2022). Food safety assessments of acrylamide formation and characterizations of flaky rolls enriched with black rice (Oryza sativa). Frontiers in Nutrition, 9, 1027800. https://doi.org/10.3389/fnut.2022.1027800
- Luthar, Z., Germ, M., Likar, M., Golob, A., Vogel-Mikuš, K., Pongrac, P., Kušar, A., Pravst, I., & Kreft, I. (2020). Breeding buckwheat for increased levels of rutin, quercetin and other bioactive compounds with potential antiviral effects. Plants (Basel), 9(12), 1638. https://doi.org/10.3390/plants9121638
- Ma, Y. J., Guo, X. D., Liu, H., Xu, B. N., & Wang, M. (2013). Cooking, textural, sensorial, and antioxidant properties of common and tartary buckwheat noodles. Food Science and Biotechnology, 22(1), 153–159. https://doi.org/10.1007/s10068-013-0021-0
- Mesías, M., Holgado, F., Márquez-Ruiz, G., & Morales, F. J. (2016). Risk/benefit considerations of a new formulation of wheat-based biscuit supplemented with different amounts of chia flour. LWT, 73, 528–535. https://doi.org/10.1016/j.lwt.2016.06.056
- Mesías, M., Morales, F. J., & Delgado-Andrade, C. (2019). Acrylamide in biscuits commercialised in Spain: A view of the Spanish market from 2007 to 2019. Food & Function, 10(10), 6624–6632. https://doi.org/10.1039/C9FO01554J
- Mieszkowska, A., & Marzec, A. (2016). Effect of polydextrose and inulin on texture and consumer preference of short-dough biscuits with chickpea flour. LWT, 73, 60–66. https://doi.org/10.1016/j.lwt.2016.05.036
- Mihai, A. L., Negoiţă, M., & Horneţ, G.-A. (2020). Assessment of the acrylamide level of cereal-based products from Romania market in accordance with commission regulation (EU) 2017/2158. The Annals of the University Dunarea de Jos of Galati Fascicle VI-Food Technology, 44(1), 104–117.
- Pokharel, A., Dangal, A., Karki, S., Lamichhane, S., Timsina, P., & Bohara, A. (2023). Study on the effect of different treatments on soy flour, and quality and sensory evaluation of prepared biscuits incorporated with oats and soy flour. Legume Science, e181. https://doi.org/10.1002/leg3.181
- Qin, P., Wang, Q., Shan, F., Hou, Z., & Ren, G. (2010). Nutritional composition and flavonoids content of flour from different buckwheat cultivars. International Journal of Food Science & Technology, 45(5), 951–958. https://doi.org/10.1111/j.1365-2621.2010.02231.x
- Rachman, A., Chen, L., Brennan, M., & Brennan, C. (2020). Effects of addition of buckwheat bran on physicochemical, pasting properties and starch digestion of buckwheat gels. European Food Research and Technology, 246(10), 2111–2117. https://doi.org/10.1007/s00217-020-03560-6
- Sun, X., Li, W., Hu, Y., Zhou, X., Ji, M., Yu, D., Fujita, K., Tatsumi, E., & Luan, G. (2018). Comparison of pregelatinization methods on physicochemical, functional and structural properties of tartary buckwheat flour and noodle quality. Journal of Cereal Science, 80, 63–71. https://doi.org/10.1016/j.jcs.2018.01.016
- Tien, N. N. T., Trinh, L. N. D., Inoue, N., Morita, N., & Hung, P. V. (2018). Nutritional composition, bioactive compounds, and diabetic enzyme inhibition capacity of three varieties of buckwheat in Japan. Cereal Chemistry, 95(5), 615–624. https://doi.org/10.1002/cche.10069
- Van Hung, P., & Morita, N. (2008). Distribution of phenolic compounds in the graded flours milled from whole buckwheat grains and their antioxidant capacities. Food Chemistry, 109(2), 325–331. https://doi.org/10.1016/j.foodchem.2007.12.060
- Wen, C., Zhang, J., Zhang, H., Duan, Y., & Ma, H. (2020). Plant protein-derived antioxidant peptides: Isolation, identification, mechanism of action and application in food systems: A review. Trends in Food Science & Technology, 105, 308–322. https://doi.org/10.1016/j.tifs.2020.09.019
- Zhang, C., Zhang, R., Li, Y. M., Liang, N., Zhao, Y., Zhu, H., He, Z., Liu, J., Hao, W., Jiao, R., Ma, K. Y., & Chen, Z.-Y. (2017). Cholesterol-lowering activity of tartary buckwheat protein. Journal of Agricultural and Food Chemistry, 65(9), 1900–1906. https://doi.org/10.1021/acs.jafc.7b00066
- Zhu, F. (2021). Buckwheat proteins and peptides: Biological functions and food applications. Trends in Food Science & Technology, 110, 155–167. https://doi.org/10.1016/j.tifs.2021.01.081
- Žilić, S., Aktağ, I. G., Dodig, D., Filipović, M., & Gökmen, V. (2020). Acrylamide formation in biscuits made of different wholegrain flours depending on their free asparagine content and baking conditions. Food Research International, 132, 109109. https://doi.org/10.1016/j.foodres.2020.109109