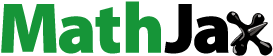
ABSTRACT
Mutations are the result of effects produced by mutagenic agents, which may be responsible for the generation of chronic-degenerative diseases such as cancer. The aim of this work was to detect and characterize compounds with antimutagenic activity in white shrimp (Litopenaeus vannamei) cephalothorax. A serial extraction was carried out where different organic solvents, and its biological activity was evaluated. The antioxidant capacity was evaluated by DPPH and ABTS assay, where the methanol and water-soluble extracts had the highest antioxidant capacity. Antimutagenicity was evaluated by the Ames test, where the methanol-soluble extract showed the highest antimutagenic activity. The methanolic extract was fractionated by open column chromatography, the fractions obtained were determined antioxidant and antimutagenic activity and the ability to protect DNA from oxidative damage. Fraction H27 provided DNA the highest protection against the damage caused by H2O2. Partial chemical-characterization of fraction H27 suggests the presence of aromatic, hydroxylated, unsaturated compounds.
Introduction
Cancer is a process by which cells grow and spread without control. Cancer is placed as the second cause of death in the world (World Health Organization, Citation2020). Oxidative stress is responsible for various diseases such as diabetes, atherosclerosis, metabolic disorders, vascular diseases, and cancer (Guerra Citation2001). When imbalances occur in the production of reactive oxygen species, free radicals are derived, which cause damage at the cellular level (García Romo et al., Citation2020) and harmful effects in molecules such as proteins, lipids, and nucleic acids take place (Pizzino et al., Citation2017).
The generation of changes in the DNA sequence of an organism is known as mutagenicity, which also is associated with metabolic problems in cellular systems causing morbidity and mortality in organisms. For this reason, it is important to search for components that can protect DNA from damage caused by mutagenic agents (Uribe-Yunda et al., Citation2012).
Chemoprevention is a strategy that has been considered for the benefits they present when treating diseases such as cancer. These compounds can be found naturally and synthetically, being able to prevent the different processes that damage cells, avoiding the early appearance of chronic-degenerative diseases (Steward et al., Citation2013).
The use of antioxidants of natural origin has been gaining attention lately, since they represent a healthy alternative to synthetic antioxidants; the latter have been reported as either toxicological or carcinogenic agents (Mitterer et al., Citation2021). Several studies have focused on the antioxidant effect present in terrestrial organisms, extracts from plants such as Secamone afzelii (Sinan et al., Citation2023), fruits such as pepper where their nutraceutical potential is highlighted (Della Valle et al., Citation2020) and peptides present in spinach species with anti-inflammatory and antinociceptive antioxidant activity (Maricianno Lorenza et al., 2023). However, the products of marine origin have been of great interest to scientists, due to the enormous number of biological activities they may exert (García Romo et al., Citation2020).
Previous studies have shown that white shrimp has antiproliferative, antioxidant and antimutagenic activity (Lopez-Saiz et al., Citation2016). However, studies that have reported antimutagenic activity in white shrimp (Litopenaeus vannamei) have been carried out in the compounds found in the muscle (Wilson-Sanchez et al., Citation2010). Antioxidant compounds can intervene in mutagenic processes, mainly preventing the oxidation of nitrogenous bases, therefore antioxidants have the potential to be antimutagenic (Renner et al., Citation1988). Based on the above, the aim of this study was to detect and characterize compounds with antimutagenic activity in white shrimp (Litopenaeus vannamei) cephalothorax.
Materials and methods
Raw material
White shrimp (Litopenaeus vannamei) specimens were obtained from a local shrimp processing company in Hermosillo, Sonora, Mexico, from which the cephalothorax was obtained and stored in polyethylene bags at −20°C until used.
Extraction
The extraction of bioactive compounds was carried out according to Osuna Ruiz et al. (Citation2016). A serial extraction using hexane, acetone, methanol, and water soluble. Firstly, 50 g of cephalothorax were homogenized in a food processor with 150 mL of hexane (1:3 w/v), kept under constant stirring for 24 h and protected from light, at a maximum temperature of 25°C; samples were filtered under vacuum with a Buchner funnel using filter paper (Whatman No. 1). After the solvent was recovered by evaporation, the hexane-soluble extract (HE) was obtained, and the solid was macerated in the same way, with the different solvents serially obtaining the acetone (AE), methanol (ME) and water- (WE) soluble extracts. Extracts were concentrated using a rotary evaporator (Yamato, RE300) under negative pressure at 40°C and carried to dryness under a N2 stream.
The extracts were re-suspended in the corresponding extraction solvent, bringing them to known concentrations and stored at 4°C until further use.
Antioxidant activity
DPPH 2,2-difenil-1-picrilhidracilo
The methodology reported by Brand Williams and Cuvelier (Citation1995) was followed with a few modifications. Briefly, the extracts were prepared in seral dilutions (500, 250, 125, 62.5, 31.25 µg/mL). A 100 µL sample was mixed with 900 µL of DPPH solution (1.52 mM), incubated for 30 min and then the absorbance read at 540 nm in a spectrophotometer (BIOMATE 3S, UV-Visible Spectrophotometer). The inhibition percentage was calculated according to the formula 1.
Where:
Abs X: Absorbance treatment
Abs Control: Absorbance control
C-: Negative control
The inhibition percentage was transformed into trolox equivalents, based on the absorbance data obtained for a curve of different concentrations of this commercial antioxidant, using the formula 2.
Where:
x: Trolox equivalents
y: Percentage of inhibition
ABTS 2,2´-azinobis (3-etilbenzotiazolinal 6-sulfonate)
The methodology described by Rea et al. (Citation1999) was used with a few modifications. The extracts were prepared in serial dilutions (500, 250, 125, 62.5, 31.25 µg/mL). A 100 µL aliquot of the sample was mixed with 900 µL of ABTS (7 mM), incubated for 30 min and the absorbance read at 734 nm in a spectrophotometer (BIOMATE 3S, UV-Visible Spectrophotometer). The percentage of inhibition was calculated according to the formula 1.
The inhibition percentage was transformed into trolox equivalents, based on the absorbance data obtained for a curve of different concentrations of this commercial antioxidant, using the formula 3.
Where:
x: Trolox equivalents
y: Percentage of inhibition
Antimutagenic activity
To evaluate the antimutagenic activity of shrimp cephalothorax extracts, the method reported by Maron and Ames (Citation1983) was used. Top agar (2 mL) supplemented with a biotin and histidine solution, 100 µL of Salmonella typhimurium TA100 strain culture, 100 µL of shrimp cephalothorax extract solution (500, 250, 125, 62.5, or 31.25 µg/mL) and 0.1 mg/plate of sodium azide mutagen (AzS) as control mutagen, were combined in a test tube and poured onto minimal glucose agar plates. Negative (spontaneous revertants) and positive (AzS without stimulus) controls were used. Plates were incubated at 37°C for 48 h, and the number of revertant colonies per plate was counted. The inhibition percentage was calculated using the formula 4.
Where:
SR: Spontaneous Revertants
Open column chromatography
To isolate bioactive compounds from the extract with the highest antimutagenic activity, a fractionation process using open a silica gel (2.5 cm × 74 cm using 70–230 mesh Sigma-Adrich) column chromatography was carried. The elution was performed using different polarities solvents (acetone, methanol, and water). The eluates obtained were subjected to thin-layer chromatography (TLC) and revealed using UV light to identify those fractions sharing similar signals were combined into a single fraction. The mobile phase for TLC was that used for the open column chromatography.
Comet assay (single-cell gel electrophoresis)
Single gel electrophoresis or comet assay is a standard technique for the detection of DNA damage at the single-cell level and can be used to evaluate the protective effect that bioactive fractions may provide on DNA against oxidative damage. This assay was performed as described by Olive et al. (Citation1990), Singh et al. (Citation1998), Yañez et al. (Citation2003) with modifications made for the analysis of antigenotoxic activity.
Vials containing 940 µL of DMEM medium (Dulbecco’s Modified Eagle Medium) were prepared to place 20 µL of whole blood. A 20 µL aliquot of the extract, at different concentrations (100, 50 or 25 µg/mL) was added to the vials, mixed and allowed to incubate for 1 h at 37°C. Subsequently, 20 µL of 10 mM H2O2 solution was added, mixed and incubated at room temperature for 10 min. The mixture was centrifuged at 2500 rpm for 30 s (Galaxy 13 microcentrifuge, VWR; max vel 10,000 rpm/7,200× g) and the supernatant was removed. A 225 µL aliquot of low melting point agarose solution (LMPA) (2.57 mg/mL) was added and mixed. A 75 µL volume of the LMPA-blood mixture was taken and placed in the center of a pre-coated slide with a layer of 0.5% regular agarose. A coverslip was placed over the mixture and left in the refrigerator for 10 min. After this, the coverslip was removed and 75 µL of LMPA were added, a coverslip was placed and left refrigerated for 10 min. Slides were removed and placed in Coplin cups containing cold lysis solution (10 mMTris-HCl, 2.5 M NaCl, and 0.1 M Na EDTA, pH 10). Coplin cups were kept refrigerated until electrophoresis.
The samples were then placed in a dark electrophoresis chamber which contained a cold alkaline buffer (300 mM NaOH, 1 nM EDTA, pH 13). The samples were allowed to stand for 20 min. Electrophoresis conditions were 25 mV, 300 mA, for 20 min. Once the electrophoresis was finished, the slides were removed and washed three times with neutralization buffer (0.4 mM Tris buffer at pH 7.5) for 5 min per wash. Finally, the slides were washed twice with anhydrous absolute ethyl alcohol for 5 min per wash. Excess of alcohol was removed, and slides were left to dry, stained with 60 µL of ethidium bromide and a coverslip was placed. An epifluorescence microscope equipped with an excitation filter of 515–560 nm and a barrier filter of 590 nm was used to visualize the comets. The comet image magnification was 200× . Olive tail moment was measured using the Image Analysis System (IAS-Delta Sistemi, Roma, Italy) software. Cell viability was determined by trypan blue dye exclusion and was always above 95%. The DNA damage produced was reported as the Olive Tail Moment (Olive et al., Citation1990).
Spectroscopic methods
UV-Visible spectroscopy
The sample was dissolved in DMSO and placed in a quartz cell where it was scanned using a spectrophotometer (BIOMATE 3S, UV-Visible Spectrophotometer) in the wavelength range 190–1100 nm.
13C and 1H-NMR analysis
The chemical-structural characterization of the compounds with antimutagenic activity was carried out by means of carbon and proton magnetic resonance (13C- and1H-NMR), using an Agilent Technologies equipment at 400 MHz. The sample was dissolved in CDCl3 (Sigma-Aldrich, Saint Louis, MI, U.S.A.) at 20 mg/mL and tetramethylsilane (TMS) was used as internal standard; samples were placed in 5 mm diameter ultraprecision NMR tubes. Chemical changes were recorded in ppm.
Results and discussion
Extraction
From the serial extraction, 4 crude extracts were obtained from the head of the white shrimp (L. vannamei): hexanic (HE) with an intense orange color, acetonic (AE) with an orange color, methanolic (ME) with a yellow color and the water-soluble (WE) that presented an opaque white color. From the serial extraction with hexane, acetone, methanol and water-soluble, yields were obtained 6.3% ± 1.8 to (hexane), 4.0 ± 1.6 (acetone) 5.8% ± 3.5 (methanol) 4.9% ± 0.9 (water-soluble), obtaining a higher yield for the hexane extract. The physical characteristics represent the difference in the composition of the compounds present in each of the extracts.
Antioxidant activity DPPH
The extracts showed significant differences with respect to their capability of donating hydrogens (), being the methanolic extract, at test concentrations of 500, 250 and 125 µg/mL and the aqueous extract, at test concentration of 500 µg/µL, those where greater amounts of trolox equivalents were recorded, obtaining the highest value for the aqueous extract (304.84 µL TE/g). A dose-dependent reduction of the DPPH radical was observed for the methanolic extract, even at low concentrations. DPPH is a free radical that is inhibited by the donation of hydrogen atoms by an antioxidant; therefore, these results suggest that these extracts exhibit the capability of donating protons by which may stabilize free radicals.
Table 1. Antioxidant activity of crude extracts of white shrimp cephalothorax by DPPH and ABTS assay.
Antioxidant activity ABTS
The extracts with different polarity showed significant differences (p ≤ 0.05) with respect to their capability to donate electrons, as assessed by the ABTS method (). The aqueous (500, 250 and 125 µg/mL), the methanolic (500, 250 µg/mL), and the hexanic (500 µg/mL) extracts had the greatest amounts of trolox equivalents, obtaining the highest value for the aqueous extract (1962.97 µL TE/g).
These results suggest that the compounds present in the extracts obtained from the cephalothorax of white shrimp (L. vannamei) can donate electrons which may be transferred to radicals.
Since many mutagens may act through the generation of reactive oxygen species (ROS), and ROS are also able to oxidate nitrogenous bases triggering mutations (Słoczyńska et al., Citation2014), the data obtained from the antioxidant tests gives important information regarding the possible action mechanism by which shrimp compounds might exert the antimutagenic activity.
Antimutagenic activity
Crude extracts soluble in hexane, acetone, methanol and water-soluble were evaluated for their antimutagenic activity at different concentrations (500, 50, 5 and 0.5 μg/mL), using positive mutagenicity controls. The crude extracts did not show significant differences (p ≤ 0.05) at the highest concentrations and in the treatments, it was possible to observe a dose-response effect (). The inhibition percentages were established as strong (>60%), moderate (40–60%), weak (20–40%) and negligible (<20%) (Ikken et al., Citation1999).
Table 2. Antimutagenic activity of crude extracts and fractions of white shrimp cephalotorax obtained after open column chromatography tested on Salmonella typhimurium TA100 strain at different concentrations (0.5–500 μg/mL). Mutagen sodium azide (0.1 mg/mL) was used as a positive control.
The HE only showed an inhibition percentage against sodium azide (AzS) of 56% at 500 μg/mL, which is considered a moderate inhibition; lower concentrations exerted no effect on AzS mutation. AE exerted a moderate inhibition of AzS with 54%, decreasing to 36% (50 μg/mL) and 27% (5 μg/mL), considering these last two as a weak effect. The ME inhibited up to 70% of AzS effect, which can be considered a strong effect. ME maintained higher inhibition values than AE and HE, at all the concentrations tested. Finally, the WE presented inhibition percentages similar to those described for ME, however, these were slightly lower. Based on the above, the effect of ME was the most promising as an antimutagenic compound against sodium azide, therefore, this extract was selected for further tests.
Open column chromatography
After the biological activity was studied, ME was subjected to an open column chromatography to separate the compounds according to their polarity, obtaining 45 eluates. The 45 eluates were subjected to thin-layer chromatography to combine those that showed similar patterns, thus obtaining 29 fractions in total (H1–H29); they were dried under a N2 stream and resuspended in methanol for further analysis.
Antioxidant activity of fractions
The DPPH test was performed in all 29 fractions. Fraction H8 was the one that presented the highest value (307.25 μM ET) (). On the other hand, when the ABTS method was performed, fraction H23 presented the highest activity (956.3 μM eT) (), with values similar to those of fractions H8, H22, H24, H25, H26 and H27. Based on the results, antioxidant activity was determined using lower concentrations and the half-maximal inhibitory concentration (IC50) for these fractions were determined (). Fractions with the lowest IC50 were H8, H25 and H27, therefore, these fractions were selected to evaluate their capability of protecting DNA against oxidative damage.
Table 3. Antioxidant activity of fractions of Litopenaeus vannamei cephalotorax by DPPH (500 μg/mL) and ABTS (100 μg/mL) assays.
Table 4. ABTS half-maximal inhibitory concentration (IC50) of Litopenaeus vannamei cephalotorax methanolic fractions.
Antimutagenic activity of fractions
Fractions showing the highest antioxidant activity were analyzed to determine their antimutagenic potential. Fractions H8, H25 and H27 were tested against sodium azide as mutagen ().
Fractions analyzed showed a greater antimutagenic activity than crude fractions; this may be since bioactive compounds in fractions are more isolated, therefore, compounds are able to inhibit the mutagenic effect of AzS. H27 fraction was the one that showed the highest antimutagenicity against AzS when tested on Salmonella typhimurium TA100 tester strain, which is prone to base pair substitutions (Maron & Ames, Citation1983), therefore, the compounds present in the fractions obtained from the methanolic extract might be capable of reversing this type of mutations.
Antigenotoxicity test of fractions
Cell viability by trypan blue staining
Carrying out this technique allowed us to know if blood cells used as the basis for the study with the Comet Assay were in good condition. Values above 95% viability were obtained on each test.
Determination of the fraction with the highest protective activity against oxidative damage to DNA
The methanolic extract and the fractions obtained from it (H8, H25 and H27) were evaluated (100, 50 and 25 μg/mL) by the Comet Assay. Blood samples were incubated for 1 h and, subsequently, oxidative damage was caused (100 mM H2O2) and residual genotoxic damage was evaluated. To calculate this, 100 comets per slide were counted and scored, and damage was expressed as Olive Tail Moment (OTM) ().
Figure 1. Protective effect (olive time moment) to different concentrations (25 to 100 μg/mL) of methanolic extract and fractions H8, H25, H27 on blood cells exposed to H2O2. The value is expressed as means ± S.D. from two independent experiments. Different letters represent statistical difference (p ≤ 0.05).
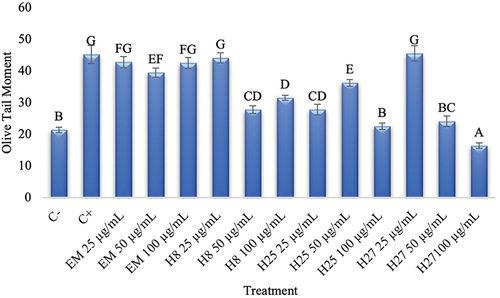
shows that, in the negative control (C-), a value of 21.41 OTM was obtained. The positive control (C+) presented a high genotoxic damage, with values of 45.3 OTM; this is due to the use of hydrogen peroxide, which can cause genotoxic damage to blood cells. It has been reported that H2O2 can cause the DNA helix to break due to its interaction with the most oxidizable sites; in fact, the reaction occurs widely at guanine (8-oxo-7,8-dihydroguanine) and, possibly, with thymine (glycol gymine) (Tayeb, Citation2020).
Significant differences were observed after treatments; the ME presented similar values to the positive control, suggesting that this extract did not provide protection to the DNA. On the other hand, when fractions were analyzed (100 and 50 μg/mL), they were able to reduce the OTM. Fraction H27, at a concentration of 100 μg/mL, showed lower values than those of the negative control; therefore, this extract, in addition to providing protection against H2O2 damage, may also have a repair effect on DNA, thus reversing the basal damage found in cells ().
Figure 2. Comets observed in the DNA oxidative damage protection assay. (a) control + (b) control – H2O2, (c) H2O2 + methanolic extract, (d) H2O2 + H8 fraction, (e) H2O2 + H25 fraction, and (f) H2O2 + fraction H 27.
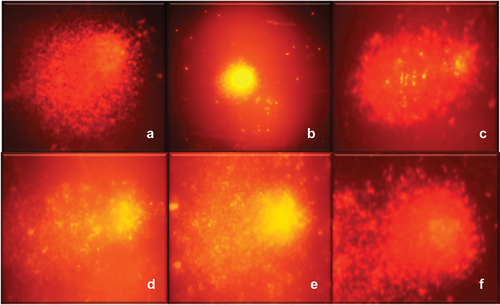
The lowest values obtained for each fraction were H27 with an OTM of 16.41, followed by H25 and H8 with OTM of 22.58, and 27.8, respectively. As for ME the OTM was 39.52. When presenting values lower than those of the negative control, the compounds in fraction H27 should continue to be studied. To our knowledge, this is the first report of antigenotoxic activity by compounds extracted from shrimp cephalothorax.
Chemical-structural characterization
Based on the results obtained from biological activity, fraction H27 was the one that could exert the highest antigenotoxic activity, therefore, spectrophotometric studies were carried out to determine its complexity and determine the presence of functional groups.
Spectrometry UV-Vis
Regarding the physical characteristics, fraction H27 was obtained as a purple liquid. A scan was performed to determine the absorbance signals in the wavelength range from 190 to 1100 nm (). Signals between 200 and 220 nm have been reported as characteristic of aromatic and phenolic compounds (Boulet et al., Citation2017). On the other hand, the signals between 450 and 500 nm, characteristic of carotenoids (Lichtenthaler & Buschmann, Citation2001) present in white shrimp such as astaxanthin, β-carotene, echinone and canthaxanthin (Arredondo Figueroa et al., Citation2003), were not observed, this could be due to the affinity of these pigments for non-polar or low polarity solvents such as hexane and acetone (Rivera & Canela, Citation2012).
1H-NMR analysis
On the other hand, a study was carried out by means of proton magnetic nuclear resonance and, according to the signals that could be observed in the spectrum (), it can be affirmed that the fraction is still complex and that it is made up of several chemical structures, therefore, there is a great variety of signals resulting from the mixture of compounds present.
In the proton spectrum it can be seen, at high field, signals with chemical shifts between δ = 0.8–2.1 ppm due to the presence of protons adjacent to aliphatic carbons linked in simple bonds (H-C); these signals are difficult to attribute to specific compounds when there is a mixture of compounds, since there is an overlapping of signals; however, they are characteristics of single links. As for the signals found with chemical shifts between δ = 2.1and 3.0 ppm, the signals can be attributed to aliphatic protons attached to carbon atoms adjacent to carbonyl groups (HC-C=) (Zghari et al., Citation2017). Moving to a low field, there were signals with chemical shifts between δ = 3.0–4.0 ppm, which are characteristic of protons attached to carbons that are single bonded to oxygen (H-C-O) (Zghari et al., Citation2017). The signals with chemical shifts between δ = 5.0–6.0 are attributed to the presence of protons that are attached to carbons linked by double bonds, also known as vinyl protons (Rivero et al., Citation2017). Regarding the signals found in the low field, with chemical shifts between δ = 6.6–8.0, these can be attributed to protons that are attached to carbons of aromatic rings adjacent to hydroxyl groups (Charisiadis et al., Citation2014).
13C-NMR analysis
A carbon magnetic nuclear resonance study was also performed to determine chemical shifts; however, due to the yields obtained, the concentration of the sample does not allow to appreciate all the signals (), however, those that were detected can be attributed as described in the following lines. At low field, with chemical shifts between δ = 0–50 ppm, the signals attributed to carbons linked in single bonds can be observed; moving to higher field, signals between δ = 50–70 ppm are attributed to carbons adjacent to a hydroxyl group; the signal seen at a shift δ = 100 ppm is attributed to aromatic-type compounds (Zghari et al., Citation2017).
The different compounds that compose fraction H27 seems to be responsible for the biological activities in the cephalothorax fraction of L. vannamei, for example, hydroxyl groups, aromatic rings and/or carbons that have double bonds they have characteristics that could help stabilize ROS, this being also a mechanism of antimutagenic activity (Słoczyńska et al., Citation2014). The mechanisms of action of the antioxidant activity can be by electron transfer (SET) or proton transfer (HAT), mechanisms which depend on the chemical structure of the compounds present in our extracts and fractions.
Conclusion
The methanolic and water-soluble extracts were able to exert antioxidant and inhibit the mutations of a known mutagen, therefore, they could be a possible source of chemopreventive compounds. Fraction H27, isolated from the methanolic extract, was able to protect DNA of cells against oxidative damage; spectroscopic studies suggest the presence of double bonds, aromatic rings and hydroxyl groups in the compounds of the fraction, nevertheless further studies are needed to identify the chemical structure for the compound responsible of the biological activity.
Acknowledgments
The authors acknowledge the National Council for Science and Technology (CONACyT) of Mexico for financing grant proposals A1-S-9762 and the graduated scholarship granted to Héctor-Enrique Trujillo-Ruiz. CVU 1078709.
Disclosure statement
No potential conflict of interest was reported by the author(s).
Additional information
Funding
References
- Arredondo Figueroa, J. L., Pedroza Islas, R., Ponce Palafox, J. T., & Vernon Carter, E. J. (2003). Pigmentation of pacific white shrimp (litopenaeus vannamei, Boone 1931) with esterified and saponified carotenoids from red chili capsicum annuum) in comparison to astaxanthin. Revista Mexicana de Ingeniería Química, 2(2), 101–8.
- Boulet, J. C., Ducasse, M. A., & Cheynier, V. (2017). Ultraviolet spectroscopy study of phenolic substances and other major compounds in red wines: Relationship between astringency and the concentration of phenolic substances. Australian Society of Viticulture and Oenology Inc.
- Brand Williams, W., & Cuvelier, M. E. (1995). Use of a free radical method to evaluate antioxidant activity. LWT - Food Science & Technology, 28(1), 25–30. https://doi.org/10.1016/S0023-6438(95)80008-5
- Charisiadis, P., Kontogianni, V. G., Tsiafoulis, C. G., Tzakos, A. G., Siskos, M., & Gerothanassis, I. P. (2014). 1H-NMR as a structural and analytical tool of intra- and intermolecular hydrogen bonds of phenol-containing natural products and model compounds. Molecules, 19(9), 13643–13682. https://doi.org/10.3390/molecules190913643
- Della Valle, A., Dimmito, M. P., Zengin, G., Pieretti, S., Mollica, A., Locatelli, M., Cichelli, A., Novellino, E., Ak, G., Yerlikaya, S., Baloglu, M. C., Celik Altunoglu, Y., & Stefanucci, A. (2020, May 8). Exploring the nutraceutical potential of dried pepper capsicum annuum L. on market from Altino in Abruzzo Region. Antioxidants (Basel), 9(5), 400. https://doi.org/10.3390/antiox9050400. PMID: 32397242; PMCID: PMC7278808.
- García Romo, J. S., Noguera Artiaga, L., Gálvez Iriqui, A. C., Hernández Zazueta, M. S., Valenzuela Cota, D. F., González Vega, R. I., Plascencia Jatomea, M., Burboa Zazueta, M. G., Sandoval Petris, E., Robles Sánchez, R. M., Juárez, J., Hernández Martínez, J., Santacruz Ortega, H. C., & Burgos Hernández, A. (2020). Antioxidant, antihemolysis, and retinoprotective potentials of bioactive lipidic compounds from wild shrimp (Litopenaeus stylirostris) muscle. CyTA - Journal of Food, 18(1), 153–163. https://doi.org/10.1080/19476337.2020.1719210
- Guerra, J. I. (2001). Estrés oxidativo, enfermedades y tratamientos antioxidantes. Anales de Medicina Interna, 18(6), 50–59. Recuperado en 13 de febrero de 2024. http://scielo.isciii.es/scielo.php?script=sci_arttext&pid=S021271992001000600010&lng=es&tlng=es
- Ikken, Y., Morales, P., Martínez, A., Marín, M. L., Isabel Haza, A., & Isabel Cambero, M. (1999). Journal of Agricultural and Food Chemistry, 47(8), 3257–3264. https://doi.org/10.1021/jf990166n
- Lichtenthaler Hartmut, K., & Claus, B. (2001). Food Analytical Chemistry, F4.2.1–F4.2.6.
- Lopez-Saiz, C. M., Hernández, J., Cinco-Moroyoqui, F. J., Velázquez, C., Ocaño-Higuera, V. M., Plascencia-Jatomea M., Robles-Sanchez, M., Machi-Lara, L., & Burgos Hernández, A. (2016). Antimutagenic compounds of white shrimp (Litopenaeus vannamei): Isolation and structural elucidation. Evidence-Based Complementary and Alternative Medicine, 2016, 1–7. https://doi.org/10.1155/2016/8148215
- Marinaccio, L., Zengin, G., Pieretti, S., Minosi, P., Szucs, E., Benyhe, S., Novellino, E., Masci, D., Stefanucci, A., & Mollica, A. (2023). Food-inspired peptides from spinach Rubisco endowed with antioxidant, antinociceptive and anti-inflammatory properties. Food Chemistry: X, 18, Article 100640. https://doi.org/10.1016/j.fochx.2023.100640.
- Maron, D. M., & Ames, B. N. (1983). Revised methods for the Salmonella mutagenicity test. Mutation Research/Environmental Mutagenesis and Related Subjects, 113(3–4), 173–215. https://doi.org/10.1016/0165-1161(83)90010-9
- Mitterer, D., Bordim, J., Lise, C., Breda, L., Casagrande, M., & Lima, V. (2021). Consumer awareness of food antioxidants. Synthetic vs. Natural. Food Sciences and Technology, 41(suppl 1), 208–212. https://doi.org/10.1590/fst.15120
- Olive, P. L., Banáth, J. P., & Durand, R. E. (1990). Heterogeneity in radiation-induced DNA damage and repair in tumor and normal cells measured using the “comet” assay. Radiation Research, 122(1), 86–94.
- Osuna-Ruiz, I., López-Saiz, C. M., Burgos-Hernández, A., Velázquez, C., Nieves-Soto, M., & Hurtado-Oliva, M. A. (2016). Antioxidant, antimutagenic and antiproliferative activities in selected seaweed species from Sinaloa, Mexico. Pharmaceutical Biology, 54(10), 2196–2210. https://doi.org/10.3109/13880209.2016.1150305
- Pizzino, G., Irrera, N., Cucinotta, M., Pallio, G., Mannino, F., Arcoraci, V., Squadrito, F., Altavilla, D., & Bitto, A. (2017). Oxidative stress: Harms and benefits for human health. Oxidative Medicine and Cellular Longevity, 2017, 1–13. https://doi.org/10.1155/2017/8416763
- Rea, R., Pellegrini, N., Proteggente, A., Pannala, A., Yang, M., & Rice Evans, C. (1999). Antioxidant activity applying an improved ABTS radical cation decolorization assay. Free Radical Biology & Medicine, 26(9–10), 1231–1237. https://doi.org/10.1016/S0891-5849(98)00315-3
- Renner, H. W., & Delincee, H. (1988). Different antimutagenic actions of linoleic and linolenic acid derivatives on busulfan-induced genotoxicity in Chinese hamsters. Nutrition Research, 8(6), 635–642. https://doi.org/10.1016/S0271-5317(05)80077-6
- Rivera, S., & Canela, R. (2012). Influence of sample processing on the analysis of carotenoids in maize. Molecules, 17(9), 11255–11268. https://doi.org/10.3390/molecules170911255
- Rivero-Cruz, J. F., de San Miguel E, R., Robles-Obregón, S., Hernández-Espino, C. C., Rivero-Cruz, B. E., Pedraza-Chaverri, J., & Esturau-Escofet, N. (2017). Prediction of antimicrobial and antioxidant activities of Mexican propolis by 1H-NMR spectroscopy and Chemometrics Data Analysis. Molecules, 22(7), 1184. https://doi.org/10.3390/molecules22071184
- Sinan, K. I., Yagi, S., Llorent-Martínez, E. J., Ruiz-Medina, A., Gordo-Moreno, A. I., Stefanucci, A., Mollica, A., Bene, K., & Zengin, G. (2023). Understanding the chemical composition and biological activities of different extracts of secamone afzelii leaves: A potential source of bioactive compounds for the food industry. Molecules, 28(9), 3678. https://doi.org/10.3390/molecules28093678
- Singh, N. P., McCoy, M. T., Tice, R. R., & Schneider, E. L. (1998). A simple technique for quantitation of low levels of DNA damage in individual cells. Experimental Cell Research, 175(1), 184–191. https://doi.org/10.1016/0014-4827(88)90265-0
- Słoczyńska, K., Powroźnik, B., Pękala, E., & Waszkielewicz, A. M. (2014). Antimutagenic compounds and their possible mechanisms of action. Journal of Applied Genetics, 55(2), 273–285. https://doi.org/10.1007/s13353-014-0198-9
- Steward, W. P., & Brown, K. (2013). Cancer chemoprevention: A rapidly evolving field. British Journal of Cancer, 109(1), 1–7. https://doi.org/10.1038/bjc.2013.280
- Tayeb, B. B. (2020). Evaluation of human genomic DNA damage caused by H2O2 in vitro using single-celll gel electrophoresis. Current Topics in Toxicology, 16, 119–125.
- Uribe-Yunda, D. F., & Navas, M.-C. (2012). Mecanismos moleculares involucrados en la mutagenicidad inducida por aflatoxina B1. Revista Ciencias de La Salud, 10(3), 403–419. http://www.scielo.org.co/scielo.php?script=sci_arttext&pid=S169272732012000300008&lng=en&tlng=
- Wilson-Sanchez, G., Moreno-Félix, C., Velazquez, C., Plascencia Jatomea, M., Acosta, A., Lorena Machi Lara, L., Aldana Madrid, M. L., Ezquerra Brauer, J. M., Robles Zepeda, R., & Burgos Hernandez, A. (2010). Antimutagenicity and antiproliferative studies of lipidic extracts from white shrimp (Litopenaeus vannamei). Marine Drugs, 8(11), 2795–2809. https://doi.org/10.3390/md8112795
- World Health Organization – WHO. (2020). Cancer. https://www.who.int/health-topics/cancer#tab=overview
- Yañez, L., García Nieto, E., Rojas, E., Carrizales, L., Mejia, J., Calderon, J., Razo, I., & Diaz-Barriga, F. (2003). DNA damage in blood cells from children exposed to arsenic and lead in a mining area. Environmental Research, 93(3), 231–240. https://doi.org/10.1016/j.envres.2003.07.005
- Zghari, B., Doumenq, P., Romane, A., & Boukir, A. (2017). GC-MS, FTIR and 1H,13C NMR structural analysis and identification of phenolic compounds in olive mill wastewater extracted from oued oussefrou effluent (Beni Mellal-Morocco). Journal of Materials and Environmental Sciences, 8(12), 4496–4509. https://doi.org/10.26872/jmes.2017.8.12.475