ABSTRACT
Chemical analysis of archaeological artefacts is used with increasing regularity to understand how wine was produced, traded, and consumed in the past and to shed light on its antiquity. Based both on an extensive review of the published literature and on new analyses, here we critically evaluate the diverse range of methodological approaches that have been used for wine identification. Overall, we conclude that currently none of the proposed chemical ‘biomarkers’ for wine provide unequivocal evidence. Nevertheless, valid interpretations may be offered if systematically supported by additional contextual data, such as archaeobotanical evidence. We found the extraction and detection method to be particularly crucial for successful identification. We urge the use of controls and quantification to rule out false positives. DNA sequencing offers potential for identifying wine and provides much higher taxonomic resolution, but work is needed to determine the limits of DNA survival on artefacts.
1. Introduction
The identification of archaeological artefacts associated with the production and consumption of alcoholic beverages has had a long and chequered history, not least due to the lack of consensus regarding methods of analysis amongst the scientific community (Stern et al. Citation2008; McGovern and Hall Citation2016). This is particularly true for wine, an important commodity in the Mediterranean attested to by the earliest written records and pictorial evidence from this region (e.g. Hamilakis Citation1996). Whilst these sources are of course immensely useful, they say little about the antiquity of wine processing itself, its economic importance through time or the pervasiveness of wine consumption within and beyond the Mediterranean region. To answer these questions, it has long been recognised that chemical analysis of artefacts associated with wine, such as pottery vessels, transport amphorae, vats, and presses offers a potential answer. Unlike botanical remains of the grape, artefacts potentially offer rich contextual detail regarding the process of viticulture, the circumstances of wine consumption, the scale of its production and direction of trade.
Most of what we know of prehistoric wine consumption comes from organic residue analysis of ceramic containers. Recent chemical analysis of potsherds from Georgia (McGovern et al. Citation2017) claims to have pushed the antiquity of wine production back to the Early Neolithic period (around 6000–5800 BC), some 1000 years earlier than its previous earliest identification in a vessel from the Zagros Mountains (Iran, 5400–5000 BC; McGovern et al. Citation1996). Wine was also claimed to be identified in vessels from Northern Greece (around 4300 BC; Garnier and Valamoti Citation2016), Egypt (from 3000 BC; Guasch-Jané et al. Citation2004, Citation2006a, Citation2006b), Northern Italy (1500–1300 BC; Pecci et al. Citation2017), and in Southern France (around 500 BC; McGovern, Luley, et al., Citation2013), providing important insights into early establishment of the tradition throughout the Mediterranean. Analysis of Egyptian vessels has highlighted the role of wine in many aspects of political and religious life (Guasch-Jané et al. Citation2006a, Citation2006b, Citation2004; McGovern Citation1997). At Bronze Age palatial sites of the Eastern Mediterranean, residue analysis claims to directly demonstrate the importance of wine in social feasting to maintain political and social relations (Koh, Yasur-Landau, and Cline Citation2014; Koh and Betancourt Citation2010; McGovern et al. Citation2008). Wine seems also to have been adopted by populations living in areas where grapevines do not grow naturally, such as Scandinavia (c. 1500 BC to the first century AD; McGovern, Hall, and Mirzoian Citation2013).
Detecting wine in ancient containers is, in particular, a way to study the Mediterranean economy, by identifying pressing and storage structures for wine, and distinguishing them from those for olive oil production (Pecci, Cau Ontiveros, and Garnier Citation2013, Pecci, Giorgi, Salvini, et al. Citation2013). Historical sources from the Classical period described the following amphorae as specific wine containers and several studies tried to check this hypothesis: amphorae from Corfu and Mende (Greece, fifth to third century B.C; Foley et al. Citation2012), Roman Dressel amphorae (first century BC to first century AD; Arobba et al. Citation2014; Condamin and Formenti Citation1978; Formenti, Hesnard, and Tchernia Citation1978; Garnier et al. Citation2003; Pecci et al. Citation2017), Late Roman (LR, fourth century AD; Pecci, Salvini, and Cantini Citation2010), and Keay amphorae (fourth to fifth century BC; Woodworth et al. Citation2015). More recently, the wine was claimed to be identified in an Early Celtic princely site (seventh to fifth centuries BC), which demonstrates trade between the Mediterranean and the Celtic spheres (Rageot et al. Citation2019).
The identification of wine in ancient vessels has been attempted using a considerable number of analytical methods, both for extraction and detection. Furthermore, the interpretation of the molecular markers varies considerably from one author to another, resulting in a confusing situation for both chemists who attempt to carry out new analyses and archaeologists who need reliable information. To clarify this situation, in this paper, we strive to give a critical review of the chemical approaches that have been used to identify the wine in the archaeological record. We provide new empirical data resulting from testing the efficiency of these approaches and propose new guidelines for the interpretation of such datasets.
2. Tartaric acid: a reliable biomarker for wine in archaeological samples?
In addition to water and ethanol, wine is composed of hundreds of other molecules present in much lower amounts and responsible for the aroma, taste, and colour of the wine. Tartaric acid (TH2) ((a)) is the principal organic acid in grapes, together with malic and citric acids (Hale Citation1962; Ribéreau-Gayon et al. Citation2006, 4). In wine, tartaric acid mainly occurs in the form of dissociated tartrate salts (TH− and T2− ions): potassium bitartrate, potassium tartrate, calcium tartrate, potassium calcium tartrate, and calcium tartromalate, with quantities generally between 1 and 5 g/L (Ribéreau-Gayon et al. Citation2006, 21–22; Singleton Citation1996).
Figure 1. Structures of the main molecules reported in the literature to identify the wine in archaeological vessels: tartaric acid (a) and syringic acid (c) formed from the alkaline treatment of malvidin-3-glucoside polymers (b). Adapted from Guasch-Jané et al. (Citation2004).
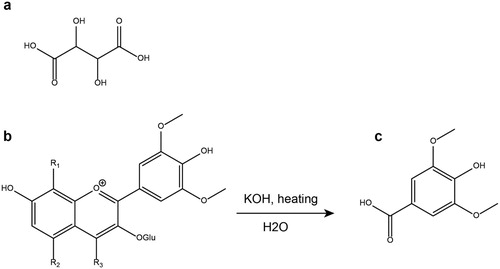
The polarity of tartaric acid and its salts makes it highly soluble in water and thus unlikely to be preserved in archaeological contexts (Barnard et al. Citation2011; Michel, McGovern, and Badler Citation1993; Singleton Citation1996), although tartrates are slightly less soluble in water than the acid. Fortunately, their polarity also offers a means of preservation by permitting binding to the ceramic matrix, through strong interactions between polar groups and Brønsted or Lewis sites of the ceramic paste, or hydrogen bonds with silicates (Garnier and Valamoti Citation2016; Michel, McGovern, and Badler Citation1993). Degradation experiments have shown that tartaric acid is better preserved in the ceramic matrix than other small acids (citric, malic, succinic, and fumaric acids) after seven years of burial in natural conditions (Pecci, Giorgi, Salvini, et al. Citation2013). Because of these properties, tartaric acid has been identified as a potential marker of wine since the inception of organic residue analysis (Condamin and Formenti Citation1978; Michel, McGovern, and Badler Citation1993).
2.1. How reliable are methods for the extraction and detection of tartaric acid in archaeological samples?
A wide range of approaches has been used to extract and detect tartaric acid from archaeological artefacts (). For the extraction, the first step must involve breaking chemical bonds between tartaric acid and the ceramic matrix. To do this, alkaline solutions (e.g. NaOH or KOH) have been widely used (), but acid treatment has also been shown to be effective, probably as it promotes partial dissolution of the ceramic matrix (Correa-Ascencio and Evershed Citation2014; Garnier and Valamoti Citation2016). Methods involving only water or methanol extraction are ill suited, as they do not break these bonds. The second step is to solubilise the tartaric acid in the extracting solvent, with a wide range of organic solvents being used (). Here, we have compared two of the most common extraction methods using modern wine samples and pure authentic tartaric acid, the latter serving as a positive control ((a) and Table S2). We have shown that the method described as ‘alkaline fusion’ and subsequent extraction with ethyl acetate (Guasch-Jané et al. Citation2004; Pecci, Giorgi, Salvini, et al. Citation2013) was highly inefficient (less than 0.1% yield for pure tartaric acid standard), due to the low solubility of tartaric acid in ethyl acetate. In our trials, we applied the same protocol but used DCM as the extraction solvent; however, this approach did not improve extraction yields (data not shown). It is also likely that the alkaline conditions promote the formation of insoluble tartrate salts. In comparison, the protocol involving butylation in acidic conditions (Garnier and Valamoti Citation2016) yielded much higher amounts of tartaric acid (our trial resulted in 77% yield for pure tartaric acid standard). The reason for this is that butylation significantly increases the solubility of the analyte in organic solvents, such as the DCM used in this method. It is clear then that when considering previous studies, the method of extraction needs to be carefully scrutinised.
Figure 2. Comparative study of two extraction methods for (a) tartaric acid and (b) syringic acid (detection by GC–MS). Methods are taken from 1Guasch-Jané et al. (Citation2004) and Pecci, Giorgi, Salvini, et al. (Citation2013), 2Garnier and Valamoti (Citation2016). Details of the materials, methods, and results are available in Supplementary information (Tables S1, S2, and S3).
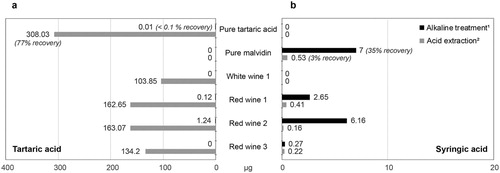
Table 1. Overview of the extraction and detection methods for tartaric acid in archaeological artefacts published in the literature.
Similarly, a wide range of approaches has been used for detecting tartaric acid in extracts from archaeological samples. In a pioneering study, Condamin and Formenti (Citation1978) detected tartaric acid using gas chromatography and thin layer-chromatography (GC and TLC) after two different preparation methods were applied (). Later studies mentioned the use of transmission and diffuse-reflectance infrared spectroscopy (DRIFTS) as a preliminary test to detect tartaric acid or its calcium salt, followed by ‘identification’ by liquid chromatography with ultraviolet detection (HPLC-UV) and/or Feigl spot tests (). More recently, capillary electrophoresis (CE) has been also proposed as a detection method based on experiments on modern wine (Teodor et al. Citation2014). All these methods, although they have laid the foundations for the study of ancient wine, are not appropriate for the identification of tartaric acid in archaeological samples since they either lack the specificity or sensitivity needed for rigorous identification (Boulton and Heron Citation2000; Stern et al. Citation2008). Feigl tests can detect tartaric acid only in concentrations greater than 5–10 μg/mL (Feigl Citation1960, 390; Stern et al. Citation2008), and are therefore unsuitable for detecting the levels likely to be present in degraded archaeological samples. In addition, these types of tests can produce false positives in the presence of several other compounds of similar structure (e.g. lactic, glucolic, glucuronic, dihydroxytartaric, citric, and malic acids; Feigl Citation1960, 389). This cautious interpretative approach is applied across other analytical applications, such as forensic analysis of narcotics where presumptive tests are used as only part of a set of minimum criteria for positive identification (e.g. United Nations Office on Drugs and Crime Citation2009). Infrared spectroscopy enables the analysis of bulk samples and identifies only the general type of chemical bonds that occur in the sample. It is therefore also not an appropriate method for the analysis of archaeological artefacts that usually involve complex molecular mixtures, often degraded and potentially contaminated (Boulton and Heron Citation2000). In particular, absorption bands from other organic products (for example resin or pitch used to seal the surfaces) may hide the tartaric acid bands (Stern et al. Citation2008). Without mass spectrometry (MS), the use of GC, HPLC or CE relies only on the retention/migration time to identify a compound and therefore lacks specificity. UV detection cannot provide precise enough information about the structure to allow identification of the compound.
To improve the sensitivity and the selectivity in the detection of tartaric acid, Guasch-Jané and co-authors (Citation2004) proposed using liquid chromatography/mass spectrometry in tandem mass spectrometry mode (LC/MS/MS; ). This method is highly reliable because it is based on the retention time, molecular mass, and on the MS fragmentation pattern. Furthermore, triple quadrupole instruments enable the use of multiple reaction monitoring (MRM) mode to improve detection, with a limit of detection (LOD) at 0.01 μg/mL (Guasch-Jané et al. Citation2004; Stern et al. Citation2008). To date, this method remains the best option, but GC–MS is also suitable for detecting tartaric acid, albeit with higher LODs ().
2.2. Interpretive issues: is tartaric acid a reliable biomarker of wine?
Given suitable methods for the extraction and detection of tartaric acid, as discussed above, a number of interpretative challenges still need to be considered before the wine can confidently be identified. First, as with many of the small organic acids, tartaric acid occurs in a large range of plants (e.g. Stafford Citation1959). Fortunately, in many edible fruits, tartaric acid is present at much lower concentrations (generally less than 100 mg/L; Singleton Citation1996) than in grapes. Consequently, such fruits are unlikely to release enough tartaric acid to be detected in pottery, especially after degradation. Some plants do have high levels of tartaric acid; these include tamarind (Rao and Mathew Citation2012; El-Siddig et al. Citation2006), the fruit of the Chinese hawthorn tree (McGovern et al. Citation2004), and some cultivars of pomegranate (Hasnaoui et al. Citation2011; Melgarejo, Salazar, and Artés Citation2000; Poyrazoğlu, Gökmen, and Artιk Citation2002). Note, however, the concentration of tartaric acid in many modern edible plant species, especially wild plants, has not been measured. Secondly, unlike lipids which are generally targeted in organic residue analysis due to their relative insolubility in the burial matrix (Heron, Evershed, and Goad Citation1991), tartaric acid is highly susceptible to migration from the surrounding sediment to the artefact studied (Barnard et al. Citation2011). Therefore appropriate controls, such as the exterior surface of the sherd or the surrounding soil, should always be used when looking for such compounds (Koh and Betancourt Citation2010; McGovern et al. Citation2017, McGovern, Luley, et al., Citation2013). Phthalates derived from plastics used to conserve and store artefacts offer another potential source of tartaric acid contamination given the structure of the precursor molecules (). Since phthalates are frequently identified in potsherds stored in plastic bags, it was important to verify that acid treatment of these compounds did not lead to the release of tartaric acid. Using the acid extraction method (, details of materials and methods in Supplementary information), testing of a pure phthalate standard and plastic bags did not produce tartaric acid, although other sources should also be considered (glue, consolidants, etc.) in the future.
Figure 3. Absence of formation of ‘wine markers’ following application to phthalates of the acidic extraction method.
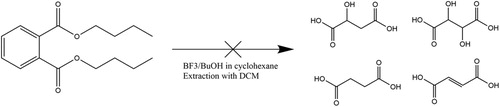
Due to its lack of specificity, the identification of grape juice/wine through the presence of tartaric acid needs to be carefully considered and supported by information on the archaeological context. Firstly, knowledge of the burial environment is important. The presence of tartaric acid in arid archaeological contexts such as Egypt (Guasch-Jané et al. Citation2006a, Citation2006b, Citation2004; McGovern, Mirzoian, and Hall Citation2009) would seem to be justified, but in other more humid contexts, tartaric acid is more likely to have been leached from the artefact (Singleton Citation1996).
Second, most studies typically report analysis of fewer than 15 vessels, usually where there is a prior belief that wine was a potential commodity, either from the context (e.g. Mediterranean amphorae) or associated botanical remains. Samples from regions or periods where wine is less likely to have been available are rarely analysed for the presence of tartaric acid. Here, there is a danger of circular reasoning leading to false-positive conclusions. To examine this last point, we tested a range of Russian prehistoric potsherds from the site of Zamostje 2 (n = 6, Table S4) located ca. 110 km north of Moscow (Bondetti et al., Citationin press), using the acidic extraction and GC–MS approach (Garnier and Valamoti Citation2016 method). Although these vessels are similar in date to those from the Caucasus (sixth millennium BC) where early viniculture has been identified (McGovern et al. Citation2017), they are from a hunter-gatherer site in a region not suitable for wine cultivation and with no archaeological evidence for contact with regions implicated in early wine cultivation. Instead, previous lipid residue analysis of these samples has shown the presence of fish and plant biomarkers (Bondetti et al., Citationin press) as well as carbonised remains of plants, such as Viburnum spp., present in charred surface deposits (foodcrusts). Surprisingly, tartaric acid was identified in all of these samples, but in variable quantities (). Foodcrusts containing plant macro remains were particularly enriched in the compound (>5 μg/g of foodcrust). The results clearly point to a false positive for wine identification, most likely explained by the presence of tartaric acid in Viburnum fruits (Ersoy, Ercisli, and Gundogdu Citation2017; Özrenk et al. Citation2011). These results show that in the absence of other botanical or historical data, the presence of tartaric acid in significant quantities in archaeological samples cannot be taken as definitive evidence of the presence of wine, but only of fruits. Extensive testing of potential sources of tartaric acid must be carried out taking into account the historical context and environmental setting before a robust interpretation can be made. The presence of other acids (e.g. cinnamic, malic, citric, ascorbic, aconitic, and gluconic acids) cannot be used as corroborative evidence for ancient wine because these compounds occur in a very wide range of plants (e.g. ).
Figure 4. Box plot of the quantities of tartaric acid extracted from (a) sherds and (b) charred surface deposits from different archaeological contexts. × indicates outliers (below or above 1.5 times the interquartile range). The maximum concentration (μg/g) is indicated in italics. Details of the materials and methods are available in Supplementary information (Table S4).
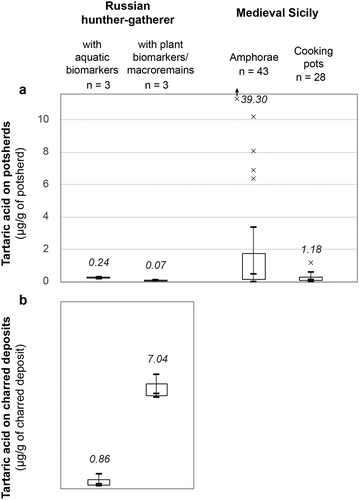
Table 2. Comparative study of modern fruit beverages extracted using the protocol of Garnier and Valamoti (Citation2016).
Comparative analyses of amphorae and cooking pots from early Medieval Sicily (Table S4) show that tartaric acid was identified in both sets of samples, although in different concentrations (). The presence of tartaric acid was unexpected in cooking pots as these predominantly contained lipids from animal products. This result shows that when detected at low concentration, tartaric acid could be the result of contamination leading to the false-positive identification of wine/fruit. We suggest that a minimum limit of detection should be considered for more robust interpretations of the presence of fruit. From our results, we propose a limit of >1 μg per g of ceramic, but ideally, this should be assessed with reference to suitable controls from the same contexts, such as sediments and forms that are unlikely to have come into contact with wine or grape juice (e.g. handles, ceramic tiles, exterior bases).
We also suggest that the extraction of tartaric acid should be undertaken routinely. Increasing the number of samples analysed would help verify the robustness of the method. For example, at the Iron Age site of Vix Mont-Lassois in Eastern France, tartaric acid was found to be only associated with specific vessel forms (Greek fine wares) despite the fact that a much larger assemblage was analysed, thereby increasing the confidence of the interpretation (Rageot et al. Citation2019). The acid extraction method is also very effective in extracting polar compounds strongly bound to the ceramic matrix so, if applied more widely, this approach could greatly enhance the detection of plant products in general (especially fruits) in the future.
3. Red or white: can wine pigments be extracted from archaeological vessels?
Wines are characterised by a large diversity of phenolic compounds: phenolic acids and derivatives and polyphenols (flavonoids, anthocyanins, and tannins) mainly derived from grapes, but also possibly from storage containers (e.g. oak barrels; Ribéreau-Gayon et al. Citation2006, 142–152). Ageing plays an important role in phenolic composition as polyphenols undergo a wide range of reactions, mostly polymerisations, which are still not fully characterised (Ribéreau-Gayon et al. Citation2006, 141). Malvidin (usually occurring as its glycoside, malvidin-3-glucoside, (b)), is the main anthocyanin in red wine and is largely responsible for its red colour (Ribéreau-Gayon et al. Citation2006, 146). Directly after fermentation, 100s of mg/L of free malvidin are detected in wine, but this concentration decreases rapidly during ageing of wine, due to polymerisation processes (Ribéreau-Gayon et al. Citation2006, 147).
The polymerisation process improves the stability of the compounds and favours their preservation over time in archaeological samples (Guasch-Jané et al. Citation2004; Singleton Citation1996). However, it also makes them difficult to extract from archaeological samples (Singleton Citation1996). To overcome this issue, the presence of tannins has been “detected” through spot/presumptive tests (; Petit-Domínguez, García-Giménez, and Rucandio Citation2003; Romanus et al. Citation2009) to measure the phenol content. However, such methods are not appropriate (Boulton and Heron Citation2000; Stern et al. Citation2008) as they are susceptible to false positives in the presence of monomeric phenolic compounds, such as ascorbic acid, gallic acid, vanillin (Singleton and Rossi Citation1965) that occur widely in the plant kingdom.
Table 3. Overview of the extraction and detection methods for polyphenols, malvidin and syringic acid in archaeological artefacts published in the literature.
An alternative analysis for polyphenol relies on chemical cleavage and analysis of the resulting products. Garnier and co-authors (Citation2003; ) used a thermally assisted hydrolysis and methylation-gas chromatography–mass spectrometry method (THM–GC–MS), after in-situ treatment with tetramethylammonium hydroxide (TMAH). Pyrolytic markers released by this method, however, are difficult to trace to specific polyphenols and the pyrolysis approach can cause unwanted side reactions leading to analytical artefacts. Alkaline degradation () was used as early as the 1970s (Condamin and Formenti Citation1978; Formenti, Hesnard, and Tchernia Citation1978) to break the polyphenol polymers and release various unspecific small acids. More recently, this method has been used to characterise syringic acid (), a small acid released from malvidin and malvidin polymers by a two-step cleavage of the C ring ((c); Guasch-Jané et al. Citation2004; Singleton Citation1996). Similarly, an acid-catalysed reaction has been proposed for releasing syringic acid from malvidin polymers, using boron trifluoride solution in butanol and cyclohexane (; Garnier and Valamoti Citation2016). As with tartaric acid, the best methods for detecting syringic acid are GC–MS or LC/MS/MS in MRM mode ().
Syringic acid itself is not a biomarker for wine as it is found widely in natural products (e.g. in swiss chard, pumpkin, honey; Trifunovic et al. Citation2015; Rezig et al. Citation2012; Pyrzynska and Biesaga Citation2009), has been found in soils (Mukherjee et al. Citation2006) and can be released on the degradation of lignin, for example during wood combustion or tar production (Simoneit et al. Citation1993). Natural degradation of cork stoppers has also been suggested to be an alternative source of syringic acid in archaeological artefacts (Woodworth et al. Citation2015). Therefore, syringic acid can only be considered as a biomarker of (red) wine, if it can be shown to directly derive from malvidin or malvidin polymer precursors. The extraction method is therefore crucial as it is imperative that free syringic acid is removed from the sample before the treatment that cleaves malvidin. Methods to extract free syringic acid including solid phase extraction (SPE) and washing with DCM/MeOH have been applied to date (; Barnard et al. Citation2011; Garnier and Valamoti Citation2016). However, there has been no assessment of the risk of false positives related to free syringic acid tightly bound to the artefact surface or released by molecules other than malvidin (e.g. lignins) when using these methods.
The effective release of syringic acid from malvidin using different approaches has also not been systematically assessed. Here we have examined the efficiency of acid and base treatment for releasing syringic acid from purified authentic malvidin, and red and white wines ( and Table S3). Interestingly, the alkaline conditions favoured by some researchers (Guasch-Jané et al. Citation2004; Pecci, Giorgi, Salvini, et al. Citation2013) were shown to be much more effective than the acid protocol (Garnier and Valamoti Citation2016), the latter barely releasing any syringic acid from the standard or the wine samples analysed ((b)).
Overall, the utility of syringic acid as a biomarker for red wine would seem to be limited. Concerns regarding the extraction methods and the specificity of the molecule in its free form need to be carefully considered. Even if appropriate measures and controls are deployed to rule out the presence of free syringic acid, it may still be released from a wide range of other natural substances potentially occurring in archaeological artefacts, including Pistacia fruits and leaves (Stern et al. Citation2008), cereals (Sun et al. Citation2002), and beer (Nardini and Ghiselli Citation2004), using acid or alkaline treatment. A final point is that the absence of syringic acid and presence of tartaric acid can never be used as evidence of white wine (Guasch-Jané et al. Citation2006a), given that the two molecules have different preservation pathways.
4. From grape juice and syrup to wine: is it possible to chemically identify ancient fermentation?
As tartaric acid occurs naturally in fresh grapes and other fruits, it cannot be considered as a marker for fermented fruit beverages. The detection of tartaric acid can be related to the presence of fruit juice or fruit syrup that were also consumed in the past (e.g. defrutum in Antiquity; Barnard et al. Citation2011). It has been suggested that malvidin could be a fermentation marker because it is released from the seeds and skin of grapes during the fermentation process (Garnier and Valamoti Citation2016). However, malvidin release begins at the maceration stages (Ribéreau-Gayon et al. Citation2006, 191–192), and is present in black grape juice, which excludes its use as a fermentation marker. Succinic, fumaric, pyruvic, citramalic (α-methylmalic), and lactic acids are produced during the fermentation of wine (Ribéreau-Gayon et al. Citation2006, 6–7) but cannot be used as markers of transformation per se because they are synthesised by several plants and fungi that occur naturally in soils (e.g. Bennet-Clark Citation1933 and ). When detected together with tartaric acid, they can, however, be considered as additional compounds possibly originating from a fruit-based fermented beverage. In the absence of tartaric acid, they provide no chemotaxonomic information.
5. Can DNA be a more reliable solution?
Genetic testing of archaeological remains represents one of the biggest developments in archaeological science in the past 35 years (Der Sarkissian et al. Citation2015). While ancient DNA (aDNA) researchers have frequently focused on human skeletal remains (Slatkin and Racimo Citation2016), the same methodologies have been used to investigate numerous species and substrates, including a few projects that targeted wine-related DNA on archaeological ceramics. As these studies precede the era of “palaeogenomics”— the current approach wherein full genomes can be characterised using high-throughput sequencing – it is important to re-evaluate these findings based on our growing knowledge of DNA survival and degradation (Kistler et al. Citation2017).
In 2003, Cavalieri et al. (Citation2003) reported the identification of yeast (Saccharomyces cerevisiae) DNA from a putative wine jar from the ancient Egyptian tomb U-j of King Scorpion I. As the primary driver of fermentation, S. cerevisiae holds a key position in viniculture and exploring its genetic history may lead to new insights into the origins of winemaking. With this in mind, Cavalieri et al. extracted DNA from a yellowish residue in the 5100-year-old jar, which they interpret as lees (dead yeast and fermentation debris). The researchers used the polymerase chain reaction (PCR) to amplify a segment of DNA that encodes ribosomal RNA in fungi, and the residue yielded several DNA sequences between 540 and 840 basepairs (bp) in length. The closest match in a reference database for the longest sequence was S. cerevisiae strain 288C, with only four nucleotide differences. The researchers interpret the results to indicate that the ancient Egyptians fermented wine with a S. cerevisiae strain which was very closely related to modern yeast.
Based on current knowledge of DNA survival, it is rare for archaeological specimens to yield DNA >300 bp, especially from hot environments like Egypt. Using the jar’s age and burial location, degradation modelling software (Smith et al. Citation2003) calculates that all DNA should have been destroyed by depurination (λ = 0.3011). Thus, it is likely that the amplified yeast DNA was a recent contaminant. Even though the research team followed strict protocols to avoid contamination, trace amounts of modern yeasts could have entered the jar during excavation, storage, or laboratory processing. Recent experiments have also shown that laboratory reagents frequently contain trace amounts of microbial DNA (Salter et al. Citation2014), and S. cerevisiae contamination has been reported in several instances (e.g. Loeffler et al. Citation1999). Given that S. cerevisiae strain 288C is a common laboratory organism (Mortimer and Johnston Citation1986) the central conclusion of ancient yeast DNA does not stand up to scrutiny.
In addition to the search for wine-related microbes, two projects have used PCR to target short genetic barcodes (<100 bp) in the chloroplast genome of species likely to have been stored in amphorae. In 2008, Hansson and Foley (Citation2008) tested two 2400-year-old Greek amphorae, finding genetic signatures of olives and oregano in the first vessel and mastic (Pistacia) resin in the second. The authors interpret the first amphora as holding olive oil, and the second as a wine container, as the resin is traditionally used as a preservative for wine. In an expanded study of nine amphorae dredged from the sea floor, Foley et al. (Citation2012) again found genetic evidence for olive and Pistacia, but also grapevine, thus supporting written accounts that some amphorae were used for transporting wine in antiquity. The researchers also found evidence for many other plant taxa including juniper, pine, legumes (Fabaceae), Zingiberaceae (ginger family), and Juglandaceae (walnut family). Based on these findings, the researchers argue that ancient amphorae were used to transport a much wider assortment of products than is often assumed.
While these researchers followed aDNA methodologies standards for the time, based on our improved knowledge of DNA degradation, it is difficult to surmise how much of the amphora genetic signal originates from ancient contents. Anaerobic underwater environments have been identified as encouraging DNA survival (Kistler et al. Citation2015), but the amphorae were recovered between one to 20 years before DNA testing and were stored in a museum in Greece (i.e. in the same region where many of the observed species grow today), leading to potential exposure to contaminants. A core concern in these studies is the reliance on PCR, a method that amplifies low levels of DNA and so suffers from being extremely sensitive to trace levels of modern contamination. PCR has been likened to finding a needle in a haystack and it played an important role in the development of aDNA research, but it is also to blame for a series of erroneous studies of fossilised materials (Cooper and Poinar Citation2000). Hansson, Foley, and their colleagues did an admirable job of testing for modern contamination by trying to amplify relatively long DNA fragments, but it is still plausible that modern DNA was inadvertently amplified and there is no clear way to authenticate the results with other data.
So far, three publications have reported wine-related DNA in amphorae, but each study has shortcomings that lead us to question some findings. In each case, the researchers only used PCR amplification, which while being an indispensable method in molecular biology experiments, has the unfortunate ability to readily recover trace amounts of modern DNA contamination. Even with many controls, these studies have produced a limited amount of genetic data that could in part (or in whole) originate from recent sources, thus preventing us from confidently inferring the ancient contents of the vessels.
6. Conclusions and future work
Through a review of the literature and some validation experiments, we provide the following conclusions related to prospects for identifying wine associated with archaeological artefacts.
Ideally, the analysis should be undertaken on a substantial number of vessels, including vessels not typically associated with wine, to give confidence in the approach.
Tartaric acid can be reliably extracted from archaeological artefacts but only using the method of Garnier and Valamoti (Citation2016).
Interpretation of the presence of tartaric acid has to also consider the wider historical and archaeological context and be supported by archaeobotanical evidence (e.g. Garnier and Valamoti Citation2016) as it may be derived from other types of fruits.
Small amounts of tartaric acid are present in soils and have been detected in artefacts that are not typically associated with wine. Therefore, it is recommended that suitable negative controls (soil or other types of archaeological artefacts that should not hold wine) are always analysed in tandem with the archaeological artefact and that quantification is always carried out.
Syringic acid can be reliably extracted from archaeological artefacts but only using alkaline treatment (Guasch-Jané et al. Citation2004; Pecci, Giorgi, Salvini, et al. Citation2013), and after removing free syringic acid.
It remains doubtful that syringic acid can be reliably traced to malvidin derived from wine.
With the current state of knowledge, it is not possible to identify wine production using only biomolecular approaches. More research into finding new and better molecular proxies for the identification of wine residues is necessary to allow more conclusive interpretations, perhaps with identifications relying on a suite of biomarkers. This can potentially be achieved through a multi-instrumental approach which would increase the range of potentially informative molecules that can be identified.
A metabolomic approach has been recently tested on wine from the Modern period, with interesting potential (Roullier-Gall et al. Citation2017), but this should now be tested on older ceramics of unknown content.
Polyphenols have real potential as biomarkers because of their resistance to degradation, but their ubiquitous nature and rapid polymerisation are challenging. Proteomics and amino acid preservation in ancient wine could be another possibility that is yet to be explored (Roullier-Gall et al. Citation2017).
DNA could offer the potential to identify wine associated with artefacts but requires new approaches deploying high-throughput sequencing and experimental work to determine the limits of survival in different conditions. High-throughput DNA sequencing approaches can provide an unbiased picture of the range of taxa present and reveal tell-tale signs of DNA damage (Llamas et al. Citation2017).
Supplemental Material
Download MS Word (38.7 KB)Acknowledgements
We are grateful to Paola Orecchioni and Antonino Meo from the Università degli studi di Roma Tor Vergata, Viva Sacco from the Ecole Française de Rome, and Olga Lozovskaya from the Institute for the History of Material Culture, Russian Academy of Sciences for providing the archaeological pottery. We also thank Ed Bergström for his valuable support in the comparative study of extraction methods. The two anonymous reviewers are also thanked for their comments and questions, which helped to improve the discussion and the quality of the paper.
Disclosure statement
No potential conflict of interest was reported by the author(s).
Notes on contributors
Léa Drieu graduated with a Master's degree in Analytical Science from the University of Strasbourg and a PhD in Prehistory from the University Côte d’Azur (Nice, France). Her research is centred on the content of ancient pottery, in particular in the framework of her post-doctoral position at the University of York, focusing on Mediterranean Early Medieval trade and the content of transport amphorae.
Maxime Rageot is a Post-Doctoral Researcher in the Biomolecular archaeology Group at the University of Tübingen (Germany) and in the Department of Pre- and Protohistory at the University of Munich (LMU). His research interests lie in the systems of transformation of ancient organic substances in recent Prehistory, using archaeology, analytical chemistry and experimental-archaeology.
Nathan Wales holds a Marie Skłodowska-Curie Individual Fellowship in the Department of Archaeology at the University of York, United Kingdom. His research is focused on characterizing ancient DNA from archaeological samples to understand the cultivation and spread of domesticated plants, especially grapevine.
Ben Stern (PhD) is a Lecturer in Archaeological Science. Ben’s research falls at the interface between analytical chemistry and archaeology, and mainly focuses on organic residue analysis to find direct molecular, trace element and isotopic evidence for the identification and use of archaeological materials.
Jasmine Lundy is a PhD candidate at BioArch, The University of York. The focus of her PhD research is to understand cuisine in Sicily during the Middle Ages, using organic residue analysis (ORA) to identify the use of cooking pots and other domestic containers. Previously, Jasmine studied an MSc in Archaeological Science at the University of Oxford. There her research focused on the use of supercritical fluids in an alternative method for extracting lipids from archaeological ceramics.
Maximilian Zerrer graduated with a BA in Archaeology from the University of Tübingen and is currently reading for an MSc in Archaeological Science at Tübingen.
Isabella Gaffney is a PhD student at the University of York. She graduated with a Bsc in chemistry from the University of Nottingham and an Msc in bioarchaeology from the University of York. Her current research investigates the chemical indicators of drought stress found in ancient maize.
Manon Bondetti is a Postdoctoral Research Assistant at BioArCh, University of York. She is a science-based archaeologist with a major interest in prehistoric pottery function and ancient culinary practices in Eurasia, using organic residue analysis.
Cynthianne Spiteri is the Junior Professor for Archaeometry at the Eberhard Karls Universität, Tübingen. She specialises in Organic Residue Analysis, which aims at characterising lipid biomolecules extracted from ceramic vessels and dental calculus using Gas Chromatography (GC), GC-Mass Spectrometry (GC-MS), GC-combustion-Isotope Ratio-Mass Spectrometry (GC-c-IRMS) and Sequential Thermal Desorption and Pyrolysis GC-MS. Her main research interests lie in the study of ancient diets and cuisine, in particular during periods of transition.
Jane Thomas-Oates is Chair of Analytical Science and Director of the Centre of Excellence in Mass Spectrometry at the University of York, York, UK. She is a biological mass spectrometrist, who applies state of the art mass spectrometry in interdisciplinary research programmes, with particular interests in (modern and ancient) plant science, agriculture and the environment.
Oliver E. Craig is a Professor of Archaeological Science at the University of York, where he directs the BioArCh research group. His main research is occupied with the analysis of ancient biomolecules from ancient skeletal remains and archaeological artefacts to provide insights into past human activities. His period interests are varied but lie in temporal transitions in human diets, cuisine and subsistence practices and the impact that dietary changes had on social evolution, health and the environment. He has published over 100 peer reviewed articles on this topic.
Additional information
Funding
References
- Arobba, D., F. Bulgarelli, F. Camin, R. Caramiello, R. Larcher, and L. Martinelli. 2014. “Palaeobotanical, Chemical and Physical Investigation of the Content of an Ancient Wine Amphora from the Northern Tyrrhenian sea in Italy.” Journal of Archaeological Science 45: 226–233. doi: 10.1016/j.jas.2014.02.024
- Barnard, H., A. N. Dooley, G. Areshian, B. Gasparyan, and K. F. Faull. 2011. “Chemical Evidence for Wine Production Around 4000 BCE in the Late Chalcolithic Near Eastern Highlands.” Journal of Archaeological Science 38: 977–984. doi: 10.1016/j.jas.2010.11.012
- Bennet-Clark, T. A. 1933. “The Role of the Organic Acids in Plant Metabolism.” New Phytologist 32: 37–71. doi: 10.1111/j.1469-8137.1933.tb06998.x
- Bondetti, M., S. Scott, A. Lucquin, J. Meadows, O. Lozovskaya, E. Dolbunova, P. Jordan, and O. E. Craig. in press. “Fruits, Fish and the Introduction of Pottery in the Eastern European Plain: Lipid Residue Analysis of Ceramic Vessels From Zamostje 2.” Quaternary International. doi:10.1016/j.quaint.2019.05.008.
- Boulton, N., and C. Heron. 2000. “The Chemical Detection of Ancient Wine.” In Ancient Egyptian Materials and Technologies, edited by P. T. Nicholson, and I. Shaw, 599–608. Cambridge: Cambridge University Press.
- Cavalieri, D., P. E. McGovern, D. L. Hartl, R. Mortimer, and M. Polsinelli. 2003. “Evidence for S. Cerevisiae Fermentation in Ancient Wine.” Journal of Molecular Evolution 57: S226–S232. doi: 10.1007/s00239-003-0031-2
- Condamin, J., and F. Formenti. 1978. “Détection du Contenu D’amphores Antiques (Huiles, vin).” Etude Méthodologique. ArchéoSciences, Revue D’Archéométrie 2: 43–58.
- Cooper, A., and H. N. Poinar. 2000. “Ancient DNA: do it Right or not at all.” Science 289: 1139–1139. doi: 10.1126/science.289.5482.1139b
- Correa-Ascencio, M., and R. P. Evershed. 2014. “High Throughput Screening of Organic Residues in Archaeological Potsherds Using Direct Acidified Methanol Extraction.” Analytical Methods 6: 1330–1340. doi: 10.1039/c3ay41678j
- Der Sarkissian, C., M. E. Allentoft, M. C. Ávila-Arcos, R. Barnett, P. F. Campos, E. Cappellini, L. Ermini, et al. 2015. “Ancient Genomics.” Philosophical Transactions of the Royal Society B: Biological Sciences 370: 20130387. doi: 10.1098/rstb.2013.0387
- El-Siddig, K., H. P. M. Gunasena, B. A. Prasad, D. Pushpakumara, K. V. R. Ramana, P. Vijayanand, and J. Williams. 2006. Tamarind: Tamarindus Indica L (Revised Version). Southampton: University of Southampton/International Centre for Underutilised Crops.
- Ersoy, N., S. Ercisli, and M. Gundogdu. 2017. “Evaluation of European Cranberrybush (Viburnum Opulus L.) Genotypes for Agro-Morphological, Biochemical and Bioactive Characteristics in Turkey.” Folia Horticulturae 29: 181–188. doi: 10.1515/fhort-2017-0017
- Feigl, F. 1960. Spot Tests in Organic Analysis. Amsterdam: Elsevier Publishing Company.
- Foley, B. P., M. C. Hansson, D. P. Kourkoumelis, and T. A. Theodoulou. 2012. “Aspects of Ancient Greek Trade Re-Evaluated with Amphora DNA Evidence.” Journal of Archaeological Science 39: 389–398. doi: 10.1016/j.jas.2011.09.025
- Formenti, F., A. Hesnard, and A. Tchernia. 1978. “Note sur le Contenu D’une Amphore Lamboglia 2 de L'épave de la Madrague de Giens.” Archaeonautica 2: 95–100. doi: 10.3406/nauti.1978.868
- Garnier, N., P. Richardin, V. Cheynier, and M. Regert. 2003. “Characterization of Thermally Assisted Hydrolysis and Methylation Products of Polyphenols from Modern and Archaeological Vine Derivatives Using Gas Chromatography–Mass Spectrometry.” Analytica Chimica Acta 493: 137–157. doi: 10.1016/S0003-2670(03)00869-9
- Garnier, N., and S. M. Valamoti. 2016. “Prehistoric Wine-Making at Dikili Tash (Northern Greece): Integrating Residue Analysis and Archaeobotany.” Journal of Archaeological Science 74: 195–206. doi: 10.1016/j.jas.2016.03.003
- Guasch-Jané, M. R., C. Andrés-Lacueva, O. Jáuregui, and R. M. Lamuela-Raventós. 2006a. “First Evidence of White Wine in Ancient Egypt From Tutankhamun’s Tomb.” Journal of Archaeological Science 33: 1075–1080. doi: 10.1016/j.jas.2005.11.012
- Guasch-Jané, M. R., C. Andrés-Lacueva, O. Jáuregui, and R. M. Lamuela-Raventós. 2006b. “The Origin of the Ancient Egyptian Drink Shedeh Revealed Using LC/MS/MS.” Journal of Archaeological Science 33: 98–101. doi: 10.1016/j.jas.2005.06.013
- Guasch-Jané, M. R., M. Ibern-Gómez, C. Andrés-Lacueva, O. Jáuregui, and R. M. Lamuela-Raventós. 2004. “Liquid Chromatography with Mass Spectrometry in Tandem Mode Applied for the Identification of Wine Markers in Residues From Ancient Egyptian Vessels.” Analytical Chemistry 76: 1672–1677. doi: 10.1021/ac035082z
- Hale, C. R. 1962. “Synthesis of Organic Acids in the Fruit of the Grape.” Nature 195: 917. doi: 10.1038/195917a0
- Hamilakis, Y. 1996. “Wine, oil and the Dialectics of Power in Bronze Age Crete: A Review of the Evidence.” Oxford Journal of Archaeology 15: 1–32. doi: 10.1111/j.1468-0092.1996.tb00071.x
- Hansson, M. C., and B. P. Foley. 2008. “Ancient DNA Fragments Inside Classical Greek Amphoras Reveal Cargo of 2400-Year-old Shipwreck.” Journal of Archaeological Science 35: 1169–1176. doi: 10.1016/j.jas.2007.08.009
- Hasnaoui, N., R. Jbir, M. Mars, M. Trifi, A. Kamal-Eldin, P. Melgarejo, and F. Hernandez. 2011. “Organic Acids, Sugars, and Anthocyanins Contents in Juices of Tunisian Pomegranate Fruits.” International Journal of Food Properties 14: 741–757. doi: 10.1080/10942910903383438
- Heron, C., R. P. Evershed, and L. J. Goad. 1991. “Effects of Migration of Soil Lipids on Organic Residues Associated with Buried Potsherds.” Journal of Archaeological Science 18: 641–659. doi: 10.1016/0305-4403(91)90027-M
- Kistler, L., O. Smith, R. Ware, G. Momber, R. Bates, P. Garwood, S. Fitch, M. Pallen, V. Gaffney, and R. G. Allaby. 2015. “Thermal age, Cytosine Deamination and the Veracity of 8,000 Year Old Wheat DNA from Sediments.” bioRxiv. doi:10.1101/032060.
- Kistler, L., R. Ware, O. Smith, M. Collins, and R. G. Allaby. 2017. “A new Model for Ancient DNA Decay Based on Paleogenomic Meta-Analysis.” Nucleic Acids Research 45: 6310–6320. doi: 10.1093/nar/gkx361
- Koh, A. J., and P. P. Betancourt. 2010. “Wine and Olive oil From an Early Minoan I Hilltop Fort.” Mediterranean Archaeology and Archaeometry 10: 15–23.
- Koh, A. J., A. Yasur-Landau, and E. H. Cline. 2014. “Characterizing a Middle Bronze Palatial Wine Cellar from Tel Kabri, Israel.” PLoS One 9: e106406. doi: 10.1371/journal.pone.0106406
- Llamas, B., G. Valverde, L. Fehren-Schmitz, L. S. Weyrich, A. Cooper, and W. Haak. 2017. “From the Field to the Laboratory: Controlling DNA Contamination in Human Ancient DNA Research in the High-Throughput Sequencing era.” STAR: Science & Technology of Archaeological Research 3: 1–14. doi: 10.1080/20548923.2016.1258824
- Loeffler, J., H. Hebart, R. Bialek, L. Hagmeyer, D. Schmidt, F. P. Serey, M. Hartmann, J. Eucker, and H. Einsele. 1999. “Contaminations Occurring in Fungal PCR Assays.” Journal of Clinical Microbiology 37: 1200–1202. doi: 10.1128/JCM.37.4.1200-1202.1999
- McGovern, P. E. 1997. “Wine of Egypt’s Golden Age: An Archaeochemical Perspective.” Journal of Egyptian Archaeology 83: 69–108. doi: 10.1177/030751339708300105
- McGovern, P. E., D. L. Glusker, L. J. Exner, and G. R. Hall. 2008. “The Chemical Identification of Resinated Wine and a Mixed Fermented Beverage in Bronze Age Pottery Vessels of Greece.” In Archaeology Meets Science: Biomolecular Investigations in Bronze Age Greece: The Primary Scientific Evidence, 1997–2003, edited by Y. Tzedakis, H. Martlew, and M. Jones, 169–218. Oxford: Oxbow.
- McGovern, P. E., D. L. Glusker, L. J. Exner, and M. M. Voigt. 1996. “Neolithic Resinated Wine.” Nature 381: 480–481. doi: 10.1038/381480a0
- McGovern, P. E., D. L. Glusker, R. A. Moreau, A. Nuñez, C. W. Beck, E. Simpson, E. D. Butrym, L. J. Exner, and E. C. Stout. 1999. “A Funerary Feast Fit for King Midas.” Nature 402: 863–864. doi: 10.1038/47217
- McGovern, P. E., and G. R. Hall. 2016. “Charting a Future Course for Organic Residue Analysis in Archaeology.” Journal of Archaeological Method and Theory 23: 592–622. doi: 10.1007/s10816-015-9253-z
- McGovern, P. E., G. R. Hall, and A. Mirzoian. 2013. “A Biomolecular Archaeological Approach to “Nordic Grog.”.” Danish Journal of Archaeology 2: 112–131. doi: 10.1080/21662282.2013.867101
- McGovern, P., M. Jalabadze, S. Batiuk, M. P. Callahan, K. E. Smith, G. R. Hall, E. Kvavadze, et al. 2017. “Early Neolithic Wine of Georgia in the South Caucasus.” Proceedings of the National Academy of Sciences of the USA 114: E10309–E10318. doi: 10.1073/pnas.1714728114
- McGovern, P. E., B. P. Luley, N. Rovira, A. Mirzoian, M. P. Callahan, K. E. Smith, G. R. Hall, T. Davidson, and J. M. Henkin. 2013. “Beginning of Viniculture in France.” Proceedings of the National Academy of Sciences of the USA 110: 10147–10152. doi: 10.1073/pnas.1216126110
- McGovern, P. E., A. Mirzoian, and G. R. Hall. 2009. “Ancient Egyptian Herbal Wines.” Proceedings of the National Academy of Sciences of the USA 106: 7361–7366. doi: 10.1073/pnas.0811578106
- McGovern, P. E., J. Zhang, J. Tang, Z. Zhang, G. R. Hall, R. A. Moreau, A. Nuñez, et al. 2004. “Fermented Beverages of pre- and Proto-Historic China.” Proceedings of the National Academy of Sciences of the USA 101: 17593–17598. doi: 10.1073/pnas.0407921102
- Melgarejo, P., D. M. Salazar, and F. Artés. 2000. “Organic Acids and Sugars Composition of Harvested Pomegranate Fruits.” European Food Research and Technology 211: 185–190. doi: 10.1007/s002170050021
- Michel, R. H., P. E. McGovern, and V. R. Badler. 1993. “The First Wine & Beer. Chemical Detection of Ancient Fermented Beverages.” Analytical Chemistry 65: 408A–413A.
- Mortimer, R. K., and J. R. Johnston. 1986. “Genealogy of Principal Strains of the Yeast Genetic Stock Center.” Genetics 113: 35–43.
- Mukherjee, G., A. Sachan, S. Ghosh, and A. Mitra. 2006. “Conversion of Sinapic Acid to Syringic Acid by a Filamentous Fungus Paecilomyces Variotii.” Journal of General and Applied Microbiology 52: 131–135. doi: 10.2323/jgam.52.131
- Nardini, M., and A. Ghiselli. 2004. “Determination of Free and Bound Phenolic Acids in Beer.” Food Chemistry 84: 137–143. doi: 10.1016/S0308-8146(03)00257-7
- Özrenk, K., M. Gündoğdu, N. Keskin, and T. Kaya. 2011. Some physical and chemical characteristics of Gilaburu (Viburnum opulus L.) fruits in Erzincan region.
- Pecci, A., MÁ Cau Ontiveros, and N. Garnier. 2013. “Identifying Wine and oil Production: Analysis of Residues From Roman and Late Antique Plastered Vats.” Journal of Archaeological Science 40: 4491–4498. doi: 10.1016/j.jas.2013.06.019
- Pecci, A., J. Clarke, M. Thomas, J. Muslin, I. van der Graaff, L. Toniolo, D. Miriello, G. M. Crisci, M. Buonincontri, and G. Di Pasquale. 2017. “Use and Reuse of Amphorae. Wine Residues in Dressel 2–4 Amphorae from Oplontis Villa B (Torre Annunziata, Italy).” Journal of Archaeological Science: Reports 12: 515–521. doi: 10.1016/j.jasrep.2017.02.025
- Pecci, A., S. Domínguez-Bella, M. P. Buonincontri, D. Miriello, R. De Luca, G. Di Pasquale, D. Cottica, and D. Bernal-Casasola. 2018. “Combining Residue Analysis of Floors and Ceramics for the Study of Activity Areas at the Garum Shop at Pompeii.” Archaeological and Anthropological Sciences 10: 485–502. doi: 10.1007/s12520-017-0573-7
- Pecci, A., G. Giorgi, L. Salvini, and MÁ Cau Ontiveros. 2013. “Identifying Wine Markers in Ceramics and Plasters Using gas Chromatography–Mass Spectrometry. Experimental and Archaeological Materials.” Journal of Archaeological Science 40: 109–115. doi: 10.1016/j.jas.2012.05.001
- Pecci, A., E. Innocenti, G. Giorgi, M. Á. C. Ontiveros, F. Cantini, E. S. Potrony, C. Alós, and D. Miriello. 2016. “Organic Residue Analysis of Experimental, Medieval, and Post-Medieval Glazed Ceramics.” Archaeological and Anthropological Sciences 8: 879–890. doi: 10.1007/s12520-015-0262-3
- Pecci, A., L. Salvini, and F. Cantini. 2010. “Residue Analysis of Some Late Roman Amphora Coming From the Excavations of the Historical Center of Florence.” In LRCW3 Late Roman Coarse Wares, Cooking Wares and Amphorae in the Mediterranean. Archaeology and Archaeometry. Comparison Between Western and Eastern Mediterranean, 2185, edited by S. Menchelli, S. Santoro, M. Pasquinucci, and G. Guiducci, 363–367. Oxford: Archaeopress (BAR Series).
- Petit-Domínguez, M. D., R. García-Giménez, and M. I. Rucandio. 2003. “Chemical Characterization of Iberian Amphorae and Tannin Determination as Indicative of Amphora Contents.” Microchimica Acta 141: 63–68. doi: 10.1007/s00604-002-0930-8
- Poyrazoğlu, E., V. Gökmen, and N. Artιk. 2002. “Organic Acids and Phenolic Compounds in Pomegranates (Punica Granatum L.) Grown in Turkey.” Journal of Food Composition and Analysis 15: 567–575. doi: 10.1016/S0889-1575(02)91071-9
- Pyrzynska, K., and M. Biesaga. 2009. “Analysis of Phenolic Acids and Flavonoids in Honey.” Trends in Analytical Chemistry 28: 893–902. doi: 10.1016/j.trac.2009.03.015
- Rageot, M., A. Mötsch, B. Schorer, D. B. Winkler, F. Sacchetti, B. Chaume, P. D. Casa, et al. 2019. “New Insights Into Early Celtic Consumption Practices: Organic Residue Analyses of Local and Imported Pottery From Vix-Mont Lassois.” PLoS ONE 14: e0218001. doi: 10.1371/journal.pone.0218001
- Rao, Y. S., and K. M. Mathew. 2012. “Tamarind.” In Handbook of Herbs and Spices (2nd ed., Vol. 2), Woodhead Publishing Series in Food Science, edited by K. W. Peter, 512–533. Oxford: Woodhead.
- Rezig, L., M. Chouaibi, K. Msaada, and S. Hamdi. 2012. “Chemical Composition and Profile Characterisation of Pumpkin (Cucurbita Maxima) Seed oil.” Industrial Crops and Products 37: 82–87. doi: 10.1016/j.indcrop.2011.12.004
- Ribéreau-Gayon, P., Y. Glories, A. Maujean, and D. Dubourdieu. 2006. Handbook of Enology. Vol. 2, The Chemistry of Wine Stabilization and Treatment. Chichester: John Wiley.
- Romanus, K., J. Baeten, J. Poblome, S. Accardo, P. Degryse, P. Jacobs, D. De Vos, and M. Waelkens. 2009. “Wine and Olive oil Permeation in Pitched and Non-Pitched Ceramics: Relation with Results from Archaeological Amphorae From Sagalassos, Turkey.” Journal of Archaeological Science 36: 900–909. doi: 10.1016/j.jas.2008.11.024
- Roullier-Gall, C., S. S. Heinzmann, J.-P. Garcia, P. Schmitt-Kopplin, and R. D. Gougeon. 2017. “Chemical Messages from an Ancient Buried Bottle: Metabolomics for Wine Archeochemistry.” npj Science of Food 1: 1. doi: 10.1038/s41538-017-0001-5
- Salter, S. J., M. J. Cox, E. M. Turek, S. T. Calus, W. O. Cookson, M. F. Moffatt, P. Turner, J. Parkhill, N. J. Loman, and A. W. Walker. 2014. “Reagent and Laboratory Contamination can Critically Impact Sequence-Based Microbiome Analyses.” BMC Biology 12: 87. doi: 10.1186/s12915-014-0087-z
- Salvini, L., A. Pecci, and G. Giorgi. 2008. “Cooking Activities During the Middle Ages: Organic Residues in Ceramic Vessels from the Sant'Antimo Church (Piombino-Central Italy).” Journal of Mass Spectrometry 43: 108–115. doi: 10.1002/jms.1283
- Simoneit, B. R. T., W. F. Rogge, M. A. Mazurek, L. J. Standley, L. M. Hildemann, and G. R. Cass. 1993. “Lignin Pyrolysis Products, Lignans, and Resin Acids as Specific Tracers of Plant Classes in Emissions from Biomass Combustion.” Environmental Science & Technology 27: 2533–2541. doi: 10.1021/es00048a034
- Singleton, V. L. 1996. “An Enologist’s Commentary on Ancient Wines.” In The Origins and Ancient History of Wine, edited by Patrick E. McGovern, Stuart J. Fleming, and Solomon H. Katz, 67–77. New York: Gordon and Breach.
- Singleton, V. L., and J. A. Rossi. 1965. “Colorimetry of Total Phenolics with Phosphomolybdic-Phosphotungstic Acid Reagents.” American Journal of Enology and Viticulture 16: 144–158.
- Slatkin, M., and F. Racimo. 2016. “Ancient DNA and Human History.” Proceedings of the National Academy of Sciences 113: 6380–6387. doi: 10.1073/pnas.1524306113
- Smith, C. I., A. T. Chamberlain, M. S. Riley, C. Stringer, and M. J. Collins. 2003. “The Thermal History of Human Fossils and the Likelihood of Successful DNA Amplification.” Journal of Human Evolution 45: 203–217. doi: 10.1016/S0047-2484(03)00106-4
- Stafford, H. A. 1959. “Distribution of Tartaric Acid in the Leaves of Certain Angiosperms.” American Journal of Botany 46: 347–352. doi: 10.1002/j.1537-2197.1959.tb07022.x
- Stern, B., C. Heron, T. Tellefsen, and M. Serpico. 2008. “New Investigations Into the Uluburun Resin Cargo.” Journal of Archaeological Science 35: 2188–2203. doi: 10.1016/j.jas.2008.02.004
- Sun, R., X. F. Sun, S. Q. Wang, W. Zhu, and X. Y. Wang. 2002. “Ester and Ether Linkages Between Hydroxycinnamic Acids and Lignins from Wheat, Rice, rye, and Barley Straws, Maize Stems, and Fast-Growing Poplar Wood.” Industrial Crops and Products 15: 179–188. doi: 10.1016/S0926-6690(01)00112-1
- Teodor, E. D., G. I. Badea, A. Alecu, L. Calu, and G. L. Radu. 2014. “Interdisciplinary Study on Pottery Experimentally Impregnated with Wine.” Chemical Paper 68: 1022–1029. doi: 10.2478/s11696-014-0559-1
- Trifunovic, S., A. Topalovic, M. Knezevic, and V. Vajs. 2015. “Free Radicals and Antioxidants: Antioxidative and Other Properties of Swiss Chard (Beta Vulgaris L. Subsp. Cicla).” Poljoprivreda i Sumarstvo 61: 73–92.
- United Nations Office on Drugs and Crime. 2009. Recommended Methods for the Identification and Analysis of Cannabis and Cannabis Products: Manual for Use by National Drug Testing Laboratories (Revised and Updated). New York: United Nations Publications.
- Woodworth, M., D. Bernal, M. Bonifay, D. De Vos, N. Garnier, S. Keay, A. Pecci, et al. 2015. “The Content of African Keay 25/Africana 3 Amphorae: Initial Results of the CORONAM Project.” In ArchaeoAnalytics: Chromatography and DNA Analysis in Archaeology, edited by C. Oliveira, R. Morais, and Á Morillo Cerdán, 41–57. Município de Esposende, Esposende.
- Zhang, T., S. Xu, Y. Li, R. Wen, and G. Yang. 2018. “Orthogonal Optimization of Extraction and Analysis for Red Wine Residues in Simulated and Archaeological Materials Using LC/MS and HPLC Methods.” Microchemical Journal 142: 175–180. doi: 10.1016/j.microc.2018.06.029