ABSTRACT
The immune receptor Tim-3 is often highly expressed in human acute myeloid leukemia (AML) cells where it acts as a growth factor and inflammatory receptor. Recently, it has been demonstrated that Tim-3 forms an autocrine loop with its natural ligand galectin-9 in human AML cells. However, the pathophysiological functions of Tim-3 in human AML cells remain unclear. Here, we report for the first time that Tim-3 is required for galectin-9 secretion in human AML cells. However, this effect is cell-type specific and was found so far to be applicable only to myeloid (and not, for example, lymphoid) leukemia cells. We concluded that AML cells might use Tim-3 as a trafficker for the secretion of galectin-9 which can then be possibly used to impair the anticancer activities of cytotoxic T cells and natural killer (NK) cells.
Keywords:
Introduction
The T cell immunoglobulin and mucin domain 3 (Tim-3) is a plasma membrane-associated protein which is highly expressed in acute myeloid leukemia (AML) cells and is therefore considered as a potential target for anti-AML therapy.Citation1,2 Although the biochemical activities of Tim-3 have recently been elucidated,Citation3-7 the functional role of this protein/receptor in AML cells remains unclear and the reasons for striking increases in Tim-3 levels in these cells are also not yet understood. Galectin-9, which was found to be a natural Tim-3 ligand,Citation3,5 was recently suggested to form an autocrine loop, where newly released galectin-9 interacts with Tim-3 expressed on the cell surface.Citation3 Furthermore, in leukocytes Tim-3 is known to be shed by proteolytic enzymes including metalloproteinases.Citation8 This leads to the dissociation of Tim-3 from the plasma membrane as a soluble protein, which then can be detectable in human blood plasma.Citation8,9 The physiological role of this shedding remains unclear as well as the functions of soluble Tim-3. However, it is known that galectin-9 is capable of impairing the immunological activities of cytotoxic T cells as well as natural killer (NK) cellsCitation1,10,11 In cytotoxic T cells galectin-9 was found to induce apoptotic death,Citation11 while in NK cells it mainly affected cytokine production thus impairing their cytotoxicity function.Citation10 These effects might therefore help AML cells to escape immune attack by both cytotoxic T cells and NK cells.Citation1,10,11
Given the above, we hypothesized that Tim-3 might act as a trafficker for galectin-9 in AML cells. We found that galectin-9 is expressed in the AML cell line THP-1, in primary human AML cells, and in primary healthy human leukocytes (although in this case the expression was considerably lower). Galectin-9 is capable of forming a stable complex with Tim-3 in cell lysates. We also detected that in THP-1 and U-937 cell lines as well as in primary healthy leukocytes the surface presence of Tim-3 is much lower compared to primary human AML cells. The same effect was observed for galectin-9. Galectin-9 knockdown in U-937 cells reduced their total Tim-3 levels. Furthermore, Tim-3 knockdown in U-937 cells did not show reductions in intracellular galectin-9 levels but galectin-9 secretion was dramatically reduced. We therefore suggested that Tim-3 is required for galectin-9 secretion in AML cells. Primary human chronic lymphoid leukemia (CLL) cells were also capable of secreting galectin-9 but expressed very low amounts of Tim-3. These results suggest that Tim-3 might be required for galectin-9 secretion in AML cells. Using ELISA, we were able to detect galectin-9 and soluble Tim-3 in human blood plasma obtained from healthy donors. Furthermore, capturing soluble Tim-3 on ELISA plates also led to detection of galectin-9. These results suggest the presence of Tim-3-galectin-9 complexes in human blood plasma but do not indicate whether they are formed inside secreting cells, on their surface, or constitutively present in blood plasma.
Results
Galectin-9 is expressed in healthy primary human leukocytes and malignant human myeloid cells and forms a complex with Tim-3 in both cell types
We first assessed whether healthy primary human leukocytes (PHL) and malignant human myeloid cell lines express galectin-9 and if its expression could be affected by pro-inflammatory stimulation as well as growth factor-induced biological responses. For this purpose, we used PHL which were isolated from buffy coat blood obtained from healthy donors and THP-1 human myeloid leukemia cells which were reported to express galectin-9.Citation6
We found that THP-1 cells produced more galectin-9 compared to PHL, however, lipopolysaccharide (LPS, ligand of pro-inflammatory Toll-like receptor (TLR) 4Citation12) and stem cell growth factor (SCF—a major haematopoietic cytokine responsible for the promotion of leukemia progressionCitation13) significantly upregulated galectin-9 levels in PHL (LPS was however shown, to be a stronger activator). Interestingly, galectin-9 bands in PHL cells appeared slightly lower on the gel compared to THP-1 cells and the positive control (), suggesting potential differences in post-translational processing. Only SCF was able to significantly increase galectin-9 levels in THP-1 cells ().
Figure 1. Effects of LPS and SCF on galectin-9 protein expression in THP-1 cells and PHL (abbreviated as PL in the figure). Cells were treated for 4 h with the indicated concentrations of LPS and SCF, then harvested and galectin-9 protein expression levels were analyzed by Western blot as outlined in Materials and Methods. Images are from one experiment representatives of three which gave similar results. Human recombinant galectin-9 (R&D Systems) was used as a positive control. Data are mean values ± SEM of three independent experiments; *p < 0.05; **p < 0.01 vs. control.
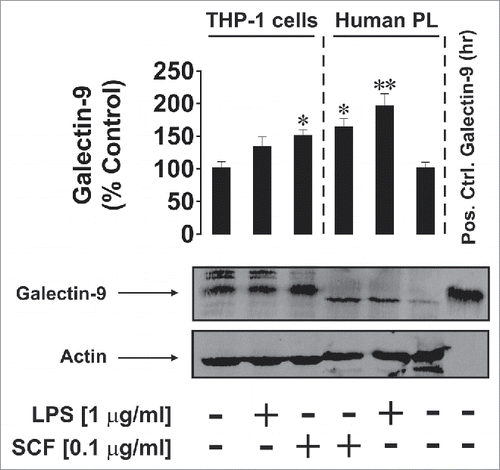
We then asked whether galectin-9 is capable of forming a complex with Tim-3 in cell lysates. For this purpose, we coated MaxiSorp ELISA plates with rabbit capture antibody directed against galectin-9 followed by the addition of cell lysates (see Materials and Methods). After incubation, galectin-9 was extracted and detected by Western blot analysis using anti-galectin-9 rabbit antibody. Tim-3 was also detected using highly specific mouse antibody. We found that in both THP-1 and PHL cells Tim-3 and galectin-9 gave positive staining at above 70 KDa which corresponds to the sum of Tim-3 and galectin-9 molecular weights detected in these cells by Western blot analysis (). Traces of free galectin-9 were still detectable, while Tim-3 was almost undetectable. The fact that SDS-PAGE electrophoresis did not separate these proteins indicates the formation of a stable complex. Since this did not occur with recombinant proteins expressed in E. Coli (which lack glycosylation), it is reasonable to surmise that the lectin (galectin-9) binds to sugar moieties on Tim-3 protein. The fact that both un-complexed proteins were detected by Western blot in fresh cell lysates suggests the requirement of both time and the presence of a surface (a solid phase—ELISA plate bottom in our case) to form such a steady complex.
Figure 2. Galectin-9 and Tim-3 form a stable complex. Lysates obtained from 300,000 THP-1 cells or 1,500,000 PHL (abbreviated as PL in the figure; expression levels of both proteins were ca. 5 times lower in PHL compared to THP-1) were loaded onto an ELISA plate pre-coated with rabbit anti-galectin-9 antibody as described in Materials and Methods). Following incubation, captured proteins were extracted and subjected to Western blot analysis (see Materials and Methods for details). Both Tim-3 (using mouse anti-Tim-3 antibody) and galectin-9 (using rabbit anti-galectin-9 antibody) were stained. Images are from one experiment representative of four which gave similar results.
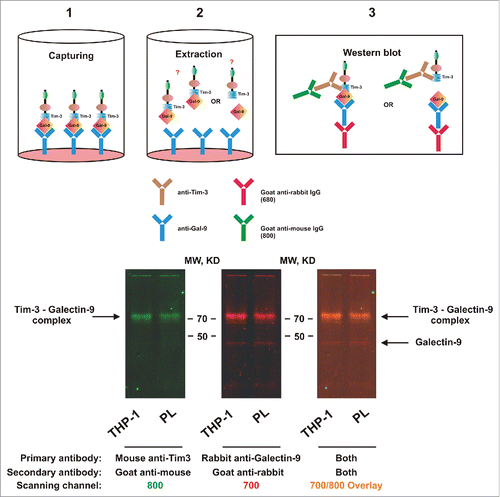
Next, we analyzed the cellular distribution of Tim-3 and galectin-9 comparing intracellular levels with cell-surface expressions. We used THP-1 and U-937 human myeloid cell lines, primary human AML cells (AML-PB001F) as well as PHL. We measured the total amounts of both proteins using in-cell Western assay in comparison to the amounts present on the cell surface using in-cell assay (also called on-cell assay; as described in the Materials and Methods). We observed that resting THP-1, U-937, and PHL cells expressed substantially more Tim-3 and galectin-9 intracellularly than on the surface. In contrast, primary AML cells had comparatively greater surface presence of both proteins (). Interestingly the ratios of Tim-3surface/Tim-3total and galectin-9surface/galectin-9total were quite similar to each other in all studied cell types ().
Figure 3. Comparative analysis of total and surface-based Tim-3 and galectin-9 proteins in human myeloid leukemia cell lines, PHL, and primary human AML cells. The indicated numbers of resting U-937 and THP-1 cells, PHL and primary human AML cells (AML-PB001F) were subjected to in-cell assay (ICA, detecting surface proteins) or in-cell Western (ICW, total protein detection) analysis as described in Materials and Methods. Images are from one experiment representative of five which gave similar results. Data are mean values ± SEM of five independent experiments; *p < 0.05; **p < 0.01 vs. control.
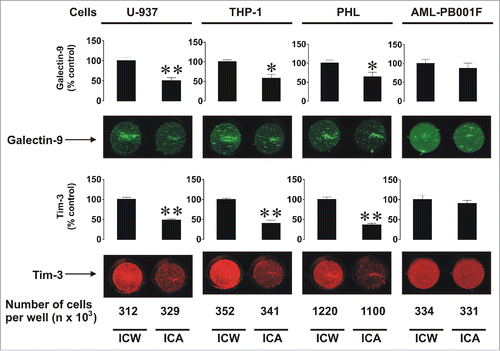
Tim-3 is required for galectin-9 release in human myeloid leukemia cells
We next asked whether Tim-3 is required for galectin-9 release and used U-937 cells (higher siRNA transfection efficiency could be reached with this cell line using our DOTAP transfection method) to investigate our hypothesis. We also studied if galectin-9 is required for Tim-3 production. First, we knocked down galectin-9 expression in U-937 cells as outlined in Materials and Methods. Non-treated normal and galectin-9 knockdown U-937 cells as well as those treated for 4 h with 2 µg/mL anti-Tim-3 stimulatory antibodyCitation5 were analyzed. Successful knockdown of galectin-9 was confirmed by Western blot analysis () as well as quantitative real-time reverse transcription PCR (qRT-PCR; Fig. S1). We found that galectin-9 knockdown reduced cellular Tim-3 levels as well as affecting galectin-9 levels (both intracellular and released ()). Previously, we reported that Tim-3 activates the mTOR pathway resulting in upregulation of glycolysis and release of pro-angiogenic vascular endothelial growth factor (VEGF). We found that galectin-9 knockdown upregulated stimulatory phosphorylation of mTOR (position S2448, ). This result was in line with the upregulation of glycolysis and VEGF release (). Interestingly, we could not observe a significant increase in intracellular levels of methylglyoxal (MGO, ) in either case, indicating that the efficiency of glycolysis correlates with the activity of glycolytic enzymes, since MGO is spontaneously formed out of dihydroxyacetone phosphate when glycolytic enzymes are oversaturated. MGO catalyzes glycation followed by generation of advanced glycation end products (Fig. S2). Higher amounts of these products could be produced by cancer cells given their dependence on glycolysis.
Figure 4. The effects of galectin-9 knockdown on Tim-3 expression and the mTOR pathway. Non-treated normal (wild type) and galectin-9 knockdown U-937 cells, as well as those which underwent 4 h treatment with 2 µg/mL anti-Tim-3 stimulatory antibodies, were analyzed for Tim-3 and galectin-9 levels, galectin-9 release (A), pS2448 mTOR intracellular levels, glycolysis, MGO, and VEGF secretion (B). Images are from one experiment representative of three which gave similar results. Data are mean values ± SEM of 3–5 independent experiments; *p < 0.05; **p < 0.01 vs. control; a p < 0.05; aap < 0.01 vs. anti-Tim-3.
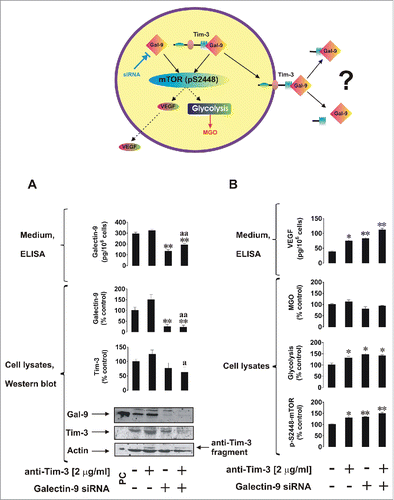
In Tim-3 knockdown U-937 cells (successful knockdown was confirmed by Western blot, , and qRT-PCR, Fig. S1), we saw a significant reduction in Tim-3 levels. No reduction in Tim-3/galectin-9 levels and no other effects were observed when using negative control random siRNA (, Fig. S1) which confirmed the specificity of the events observed. Tim-3 knockdown did not lead to changes in intracellular galectin-9 levels but dramatically reduced galectin-9 secretion, suggesting that Tim-3 is required for the release of galectin-9 (). Reduction in Tim-3-mediated mTOR phosphorylation at S2448 and respectively downregulation of glycolysis/VEGF release was also observed in Tim-3 knockdown U-937 cells. No significant changes in MGO levels were observed suggesting that glycolytic efficiency—a key energy pathway in leukemia cells—was not affected ().
Figure 5. Tim-3 is required for galectin-9 secretion in human myeloid leukemia cells. Non-treated normal (wild type), Tim-3 knockdown and transfected with random siRNA U-937 cells, as well as the same cells which underwent 4 h treatment with 2 µg/mL anti-Tim-3, were subjected to analysis for Tim-3 and galectin-9 levels, galectin-9 release (A), pS2448 mTOR intracellular levels, glycolysis, MGO, and VEGF secretion (B). Images are from one experiment representative of three which gave similar results. Data are mean values ± SEM of 3–5 independent experiments; *p < 0.05; **p < 0.01 vs. control; ap < 0.05; aap < 0.01 vs. anti-Tim-3; bp < 0.05; bbp < 0.01 vs. random siRNA.
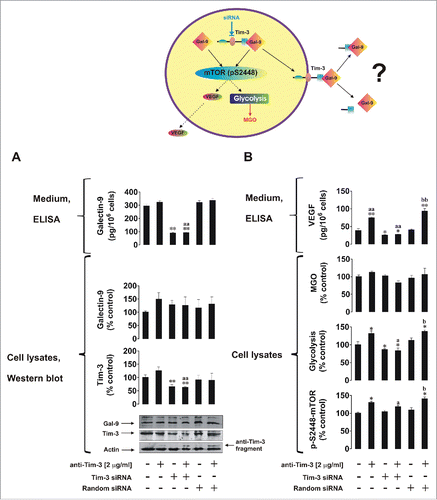
Tim-3 is a specific trafficker for galectin-9 in certain cell types
It is generally known that many cell types, including lymphoid cells, are capable of producing galectin-9 but not all of them express Tim-3. We therefore compared the capability of primary human AML cells and primary human CLL cells to produce galectin-9. Both cell types secreted detectable amounts of galectin-9. In AML cells, but not in CLL cells, the amount of secreted galectin-9 was significantly increased by 4 h of exposure to 2 µg/mL anti-Tim-3 (). Both PHL and AML cells expressed Tim-3 but this expression was much higher in AML cells compared to PHLs (Tim-3/actin ratios were analyzed, ). CLL cells expressed almost undetectable amounts of Tim-3 but still they were capable of releasing galectin-9, indicating that they probably use a different trafficker for this protein.
Figure 6. Galectin-9 and Tim-3 levels in primary human AML, CLL, and PHL cells as well as in blood plasma. (A) Non treated primary human AML and CLL cells, as well as those exposed for 4 h to anti-Tim-3, were precipitated and medium was subjected to measurement of galectin-9 by ELISA. Data represent mean values ± SEM of three independent experiments; *p < 0.05 vs. control. (B) PHL, primary human AML, and CLL cells were subjected to Western blot analysis for Tim-3. Images are from one experiment representative of four which gave similar results. Data are mean values ± SEM of four independent experiments. (C) Blood plasma of six healthy donors was subjected to ELISA assays for galectin-9, soluble Tim-3, and Tim-3-galectin-9 complex (for details, please see Materials and Methods and diagrams underneath the table). Data are mean values ± SEM of 3–5 independent experiments.
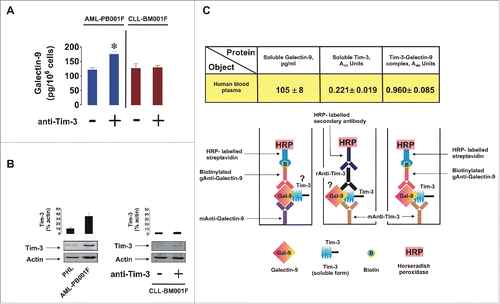
Finally, we observed that blood plasma of healthy donors contained both galectin-9 and soluble Tim-3 as measured by ELISA. Intriguingly, when capturing Tim-3 on the ELISA plate and applying detection antibody directed against galectin-9 we were able to detect a clear signal () which was not applicable when intact cell culture medium or galectin-9 standards were used. This means that a complex formed by non-cell-associated soluble Tim-3 and galectin-9 is present in the blood plasma of healthy donors. Our previous experiments demonstrated that anti-Tim-3 antibody does not compete with galectin-9.Citation5
Discussion
Galectin-9 is a typical galectin family member which has a tandem structure.Citation10,14 It has been reported to specifically interact with the immune receptor Tim-3 inducing mTOR pathway activation as well as forming an autocrine loop with Tim-3 in human AML cells.Citation3,5 Due to very high expression levels of Tim-3 in AML cells this protein is currently considered as a target for anti-AML therapy. Furthermore, galectin-9 was reported to specifically target cytotoxic T cells and NK cells and impair their ability to attack and kill leukemia cells.Citation10,11,15
Our present study clearly shows that human myeloid leukemia cells express and secret galectin-9 ( and Fig. S1). Importantly, galectin-9 production is upregulated by pro-inflammatory stimulation (LPS) and growth factor (SCF) action in both healthy human leukocytes and AML cells (THP-1 cell line). Release of galectin-9 was clearly dependent on the Tim-3. Tim-3 knockdown in U-937 cells led to significant reductions in galectin-9 secretion compared to normal (wild type) U-937 cells (). Tim-3 protein levels were also reduced (but not significantly) in galectin-9 knockdown U-937 cells compared to wild type cells (). However, Tim-3 mRNA levels were significantly downregulated in galectin-9 knockdown U-937 cells ( Fig. S1) suggesting that transcription of the Tim-3 gene depends on intracellular levels of galectin-9. Interestingly, Tim-3 knockdown did not affect intracellular galectin-9 levels in U-937 cells, as measured by Western blot analysis, but dramatically reduced the capability of these cells to secrete galectin-9. These results suggest that galectin-9 levels influence Tim-3 expression at a genomic level, whereas Tim-3 protein controls the capability of myeloid leukemia cells to secrete galectin-9.
Recent evidence demonstrated that A disintegrin and metalloproteases (ADAM) 10 and 17 control shedding of Tim-3 from the cell surface in HEK-293 cells and human primary CD14+ monocytes.Citation8 This suggests that Tim-3 might undergo shedding when it is in complex with galectin-9, which allows dissociation of the Tim-3 fragment together with the full-length galectin-9 protein. This hypothesis might explain why LPS reduces cellular Tim-3 surface expressionCitation6,8 taking into account our results demonstrating LPS-induced upregulation of galectin-9 levels. Our previous study demonstrated that Tim-3 protein production is not reduced by LPSCitation5 while surface presence is affected.Citation6,8 This might be the result of increased intracellular galectin-9 levels leading to enhanced galectin-9 secretion which, as we have described above, requires Tim-3. ADAM10/17 or other proteolytic enzymes can then possibly shed the Tim-3/galectin-9 complex. Further evidence also supports the rapid shedding process such as via ligand-induced Tim-3-mediated activation of the mTOR pathway.
Although Tim-3 is a weaker signal transducer compared to Kit (SCF receptor) or TLR4, a moderate level of mTOR activation could be the result of swift removal of the extracellular domain which terminates the signal prematurely compared to those observed for non-cleaved receptors (as TLR4 or Kit). Furthermore, in support of a Tim-3/galectin-9 complex shedding scenario, other factors, such as HIV-1 infection, lead to increases in both soluble Tim-3 and galectin-9 levels in blood plasma.Citation9,16 Also, phorbol 12-myriastate 13-acetate (PMA) was found to upregulate galectin release in Jurkat T cells,Citation17 where it also upregulates Tim-3 expression/externalisation.Citation18 PMA is also known to promote Tim-3 proteolytic shedding.Citation8 Matrix metalloproteinase inhibitor BB-94 (ADAM10 was also found to be sensitive to itCitation19) was able to abolish PMA-induced galectin-9 release in Jurkat T cells (immortalised T lymphocytic cell line derived from T cell leukemiaCitation17), suggesting that proteolysis is probably involved in shedding Tim-3 complexed with galectin-9.
Our results also demonstrated that CLL bone marrow mononuclear cells (containing elevated levels of CD4+ T cells with a cytotoxic phenotypeCitation20) are capable of secreting galectin-9 but they only produce very low amounts of Tim-3 (). This suggests that Tim-3 is a cell type-specific trafficker of galectin-9 in AML cells, while other cell types (such as CLL bone marrow mononuclear cells) might use other galectin-9-specific proteins, which can be externalized. In support of this, there is evidence that galectin-9 may cause Tim-3-independent effects (including apoptosis) in lymphoid cells.Citation10,21
In human blood plasma obtained from healthy donors, we found that both galectin-9 and soluble Tim-3 are present (). However, anti-Tim-3 detection antibodies also gave rise to a positive signal to visualize the product captured by anti-galectin-9 antibody (). This clearly indicates that a Tim-3/galectin-9 complex is present in human blood plasma. However, our results do not provide any indication at which point the complex was formed.
Our results suggest that Tim-3 is required for galectin-9 secretion in AML cells and this mechanism may be then used to impair the anticancer activity of cytotoxic T cells and NK cells, which might allow malignant blood cells to escape immune attack. Our hypothesis is summarized in the .
Figure 7. Tim-3-dependent secretion of galectin-9 in human AML cells could potentially impair the anti-leukemic activity of cytotoxic T cells and NK cells.
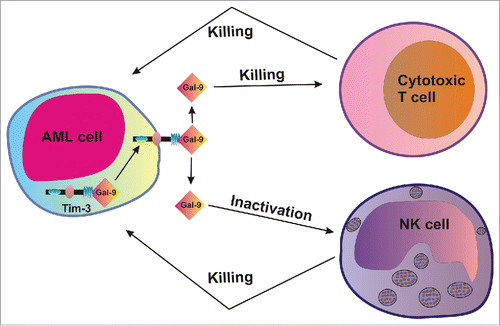
Our findings also demonstrated that decrease in intracellular galectin-9 level increases mTOR activity and its downstream effects (efficient glycolysis and VEGF production, ). In myeloid leukemia cell lines as well as in PHL, the activity of the mTOR pathway is always lower than in primary AML cells.Citation4,5 One of the reasons for this might be externalisation of the Tim-3/galectin-9 complex in primary AML cells (confirmed in our studies—see ). In this case, while there are lower amounts of galectin-9 inside the cells Tim-3/galectin-9 complexes are externalized on the cell surface, which can initiate signaling events before further modification or proteolytic shedding. These effects are presented in , which summarizes Tim-3 signal transducing activities in PHL/AML cell lines compared with primary human AML cells.
Figure 8. Distribution of complexes and corresponding effects of Tim-3 and galectin-9 in healthy and malignant human white blood cells. (A) Scheme demonstrating intracellular signaling pathways induced by Tim-3-galectin-9 complexes. (B) Tim-3/galectin-9 distribution and corresponding effects in PHL and human myeloid leukemia cell lines (THP-1 and U-937). (C) Tim-3/galectin-9 distribution and corresponding effects in primary human AML cells.
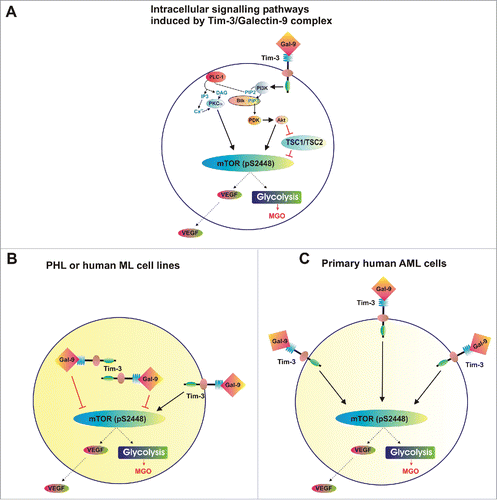
Our results also highlight that both Tim-3 and galectin-9 are promising therapeutic targets for possibly curing AML. Absence of the Tim-3/galectin-9 autocrine loop or the timely capture and subsequent inactivation of biologically active galectin-9 may reduce the ability of AML cells to impair the anticancer activities of NK cells and cytotoxic T cells. This may therefore result in the natural eradication of cancer cells by functional NK cells and cytotoxic T cells.
Materials and methods
Materials
RPMI-1640 medium, foetal bovine serum and supplements, LPS (from P. aeruginosa), DOTAP transfection reagent, primers and galectin-9 silencing RNA (siRNA) were purchased from Sigma (Suffolk, UK). Maxisorp™ microtitre plates were provided either by Nunc (Roskilde, Denmark) or were a kind gift of Oxley Hughes Ltd (London, UK). Mouse monoclonal antibodies directed against mTOR and β-actin as well as rabbit polyclonal antibodies against phospho-S2448 mTOR, galectin-9, Tim-3, and HRP-labeled rabbit anti-mouse secondary antibody were purchased from Abcam (Cambridge, UK). Goat anti-mouse and goat anti-rabbit fluorescence dye-labeled antibodies were obtained from Li-Cor (Lincoln, Nebraska USA). ELISA-based assay kits for the detection of VEGF were purchased from Bio-Techne (R&D Systems, Abingdon, UK). All other chemicals purchased were of the highest grade of purity.
Primary human AML and CLL cells
Primary human AML mononuclear blasts (AML-PB001F, newly diagnosed/untreated) were purchased from AllCells (Alameda, CA, USA) and handled in accordance with manufacturer’s instructions. Primary human bone marrow derived CLL mononuclear cells (CLL-BM001F, newly diagnosed/untreated leukemia) were also obtained from AllCells (Alameda, CA, USA) and handled in accordance with manufacturer’s protocol. Cells from six different patients were analyzed following ethical approval (REC reference: 16-SS-033).
THP-1 and U937 human myeloid cell lines
THP-1 human myeloid leukemia monocytes and U937 human myeloid monocytes were obtained from the European Collection of Cell Cultures (Salisbury, UK). Cells were cultured in RPMI 1640 media supplemented with 10% foetal bovine serum, penicillin (50 IU/mL) and streptomycin sulfate (50 µg/mL).
Primary human leukocytes obtained from healthy donors (buffy coats)
PHL were obtained from buffy coat blood (originated from healthy donors undergoing routine blood donation) which was purchased from the National Health Blood and Transfusion Service (NHSBT, UK) following ethical approval (REC reference: 16-SS-033). Mononuclear-rich leukocytes were obtained by Ficoll-density centrifugation according to the manufacturer’s protocol. Cell numbers were counted using a haemocytometer and diluted accordingly with HEPES-buffered Tyrode’s solution before the indicated treatments.Citation4
Stem cell factor
Human SCF protein was produced in E. Coli and purified in accordance with previously published protocols.Citation20
Transfer of Galectin-9/Tim-3 siRNA into U-937 cells and qRT-PCR
We employed a galectin-9-specific siRNA target sequence (uga ggu gga agg cga ugu ggu ucc c) which was previously described.Citation14 For Tim-3 knockdown, we used commercially available siRNA purchased from Santa Cruz Biotechnology, CA, USA, as described before.Citation7 As a control, we used corresponding random siRNA (uac acc guu agc aga cac c dtdtCitation22). Transfection into U-937 cells was performed using DOTAP reagent according to the manufacturer's protocol.
To monitor galectin-9 and Tim-3 mRNA levels, we used qRT-PCR.Citation21 Total RNA was isolated using a GenElute™ mammalian total RNA preparation kit, followed by a target protein mRNA reverse transcriptase–polymerase chain reaction (RT-PCR) performed in accordance with the manufacturer's protocol (Sigma). This was followed by quantitative real-time PCR. Primer selection was as follows: Galectin-9, 5′-CTTTCATCACCACCATTCTG-3′, 5′-ATGTGGAACCTCTGAGCACTG-3′ Tim-3, 5′-CATGTTTTCACATCTTCCC-3′ actin, 5′-TGACGGGGTCACCCACACTGTGCCCATCTA-3′, 5′-CTAGAAGCATTTGCGGTCG-ACGATGGAGGG-3′. Reactions were performed using a LightCycler® 480 real-time PCR system and respective SYBR Green I Master kit (obtained from Roche, Burgess Hill, UK). Analysis was performed according to the manufacturer's protocol. Values representing galectin-9 and Tim-3 mRNA levels were normalized against β-actin.
Western blot analysis
Expressions of Tim-3 and galectin-9 were determined by Western blot analysis and compared to β-actin in order to determine equal protein loading, as previously described.Citation4 Li-Cor goat secondary antibodies, conjugated with fluorescent dyes, were used in accordance with manufacturer's protocol to visualize target proteins (using a Li-Cor Odyssey imaging system). Western blot data were quantitatively analyzed using Odyssey software and values were subsequently normalized against those of β-actin.
Detection of phospho-S2448 mTOR in cell lysates by ELISA
Phosphorylation of mTOR was analyzed by ELISA as described before.Citation23 Briefly, 96-well ELISA plates were coated with mouse anti-mTOR antibodies followed by blocking with 2% BSA. Cell lysates were then added to the wells and incubated at room temperature for 2 h (with constant agitation). Plates were then washed with Tris-Buffered Saline containing Tween 20 (TBST). Following washing with TBST buffer plates were incubated with HRP-labeled goat anti-rabbit IgG for 1 h at room temperature. Plates were then washed using TBST and bound secondary antibodies were visualized by the peroxidase reaction (ortho-phenylenediamine/H2O2).
In-cell Western and in-cell (on-cell) assay
We employed a standard Li-Cor in-cell Western assay to analyze total Tim-3 and galectin-9 expressions in the studied cells.Citation4 The in-cell (also called on-cell) assay was employed to characterized Tim-3 and galectin-9 surface presence in the studied cells.
Characterization of Tim-3 and galectin-9 interactions in cell lysates
An ELISA-based analysis was used where plates were first coated with anti-galectin-9 antibody followed by blocking with 2% BSA. Cell lysates were then applied and incubated for at least 4 h at room temperature after which plates were extensively washed with TBST. A glycine-HCl pH lowering buffer (pH 2.0) was then applied to extract the bound proteins. Extracts were mixed with equal volumes of lysis buffer (pH 7.5) and with 4× sample buffer for SDS-PAGE at a ratio of 1:3. Samples were then subjected to Western blot analysis, using rabbit anti-galectin-9 and mouse anti-Tim-3 primary antibodies, as described above. The plate was subjected to analysis with galectin-9 detection antibody followed by standard ELISA steps to ensure that no galectin-9 remained in the wells. Signals obtained were similar to those where PBS was used instead of the sample confirming that all the protein was extracted.
Detection of galectin-9 and VEGF secretion, soluble forms of Tim-3 and Tim-3-galectin-9 complexes
Galectin-9 and VEGF levels released into the medium were analyzed by ELISA (R&D Systems assay kits) according to the manufacturer's instructions. Soluble Tim-3 was detected using similar approach where mouse anti-Tim-3 (mAnti-Tim-3) was employed as a capture antibody and rabbit anti-Tim-3 (rAnti-Tim-3)—for detection (, scheme). HRP-labeled anti-Rabbit secondary antibody (Abcam) was used to visualize the reaction. Tim-3-galectin-9 complexes in blood plasma were detected by ELISA. Mouse anti-Tim-3 (mAnti-Tim-3) was employed as a capture antibody and biotinylated goat anti-galectin-9 (gAnti-Galectin-9, R&D Systems) as detection antibody. The reaction was visualized using HRP-labeled streptavidin (R&D Systems; , scheme). In all cases plates were washed using TBST and bound secondary antibodies visualized by the peroxidase reaction (ortho-phenylenediamine/H2O2).
Characterization of glycolysis and MGO levels
Glycolytic degradation of glucose was analyzed using a colorimetric assay as described before.Citation20 Briefly, the approach was based on the ability of cell lysates, used as a multi-enzyme preparation, to convert glucose into lactate in the absence of oxygen (this was achieved by employing an anaerobic chamber). Cell lysates were incubated for 1 h at 37°C with 1% glucose solution within the anaerobic chamber. 2% trichloroacetic acid solution was then used to precipitate proteins. This was followed by carbohydrate precipitation using saturated CuSO4 solution in combination with Ca(OH)2 (at a final concentration of 60 mg/mL). Lactate was then converted into acetic aldehyde using concentrated H2SO4 at 90°C for 1 min and cooled the samples down on ice. We then detected acetaldehyde using the veratrole (1,2-dimethoxybenzene) test. MGO was also detected colorimetrically following biochemical modifications.Citation24,25 Briefly, MGO was condensed with reduced glutathione (GSH, 1 mM) for 10 min at 37°C. The complex was then converted into lactate by Glyoxalases I and II at pH 8.0 (Glyoxalase I converted the complex in D-lactoyl-glutathione, which was then transformed into lactate by Glyoxalase IICitation26). The whole process is highlighted in Fig. S2). Lactate was then measured colorimetrically as described above.
Statistical analysis
Each experiment was performed at least three times and statistical analysis was conducted using a 2-tailed Student's t test. Statistical probabilities (p) were expressed as *p < 0.05, **p < 0.01, and ***p < 0.001.
Disclosure of potential conflicts of interest
No potential conflicts of interest were disclosed.
KONI_A_1195535_s02.docx
Download MS Word (414.1 KB)Funding
L.V. and M.B. were supported by a grant from Oncosuisse (KFS 3728082015).
References
- Kikushige Y, Miyamoto T. TIM-3 as a novel therapeutic target for eradicating acute myelogenous leukemia stem cells. Int J Hematol 2013; 98:627-633; PMID:24046178; http://dx.doi.org/10.1007/s12185-013-1433-6
- Kikushige Y, Miyamoto T. Identification of TIM-3 as a Leukemic Stem Cell Surface Molecule in Primary Acute Myeloid Leukemia. Oncology 2015; 89 Suppl 1:28-32; PMID:26551150; http://dx.doi.org/10.1159/000431062
- Kikushige Y, Miyamoto T, Yuda J, Jabbarzadeh-Tabrizi S, Shima T, Takayanagi S, Niiro H, Yurino A, Miyawaki K, Takenaka K et al. A TIM-3/Gal-9 Autocrine Stimulatory Loop Drives Self-Renewal of Human Myeloid Leukemia Stem Cells and Leukemic Progression. Cell Stem Cell 2015; 17:341-352; PMID:26279267; http://dx.doi.org/10.1016/j.stem.2015.07.011
- Gonçalves Silva I, Gibbs BF, Bardelli M, Varani L, Sumbayev VV. Differential expression and biochemical activity of the immune receptor Tim-3 in healthy and malignant human myeloid cells. Oncotarget 2015; 6:33823-33833; PMID:26413815; http://dx.doi.org/10.18632/oncotarget.5257
- Prokhorov A, Gibbs BF, Bardelli M, Ruegg L, Fasler-Kan E, Varani L, Sumbayev VV. The immune receptor Tim-3 mediates activation of PI3 kinase/mTOR and HIF-1 pathways in human myeloid leukaemia cells. Int J Biochem Cell Biol 2015; 59:11-20; PMID:25483439; http://dx.doi.org/10.1016/j.biocel.2014.11.017
- Ma CJ, Li GY, Cheng YQ, Wang JM, Ying RS, Shi L, Wu XY, Niki T, Hirashima M, Li CF et al. Cis association of galectin-9 with Tim-3 differentially regulates IL-12/IL-23 expressions in monocytes via TLR signaling. PLoS One 2013; 8:e72488; PMID:23967307; http://dx.doi.org/10.1371/journal.pone.0072488
- Zhang Y, Ma CJ, Wang JM, Ji XJ, Wu XY, Moorman JP, Yao ZQ. Tim-3 regulates pro- and anti-inflammatory cytokine expression in human CD14+ monocytes. J Leukoc Biol 2012; 91:189-196; PMID:21844165; http://dx.doi.org/10.1189/jlb.1010591
- Moller-Hackbarth K, Dewitz C, Schweigert O, Trad A, Garbers C, Rose-John S, Scheller J. A disintegrin and metalloprotease (ADAM) 10 and ADAM17 are major sheddases of T cell immunoglobulin and mucin domain 3 (Tim-3). J Biol Chem 2013; 288:34529-34544; PMID:24121505; http://dx.doi.org/10.1074/jbc.M113.488478
- Clayton KL, Douglas-Vail MB, Nur-ur Rahman AK, Medcalf KE, Xie IY, Chew GM, Tandon R, Lanteri MC, Norris PJ, Deeks SG et al. Soluble T cell immunoglobulin mucin domain 3 is shed from CD8+ T cells by the sheddase ADAM10, is increased in plasma during untreated HIV infection, and correlates with HIV disease progression. J Virol 2015; 89:3723-3736; PMID:25609823; http://dx.doi.org/10.1128/JVI.00006-15
- Golden-Mason L, McMahan RH, Strong M, Reisdorph R, Mahaffey S, Palmer BE, Cheng L, Kulesza C, Hirashima M, Niki T et al. Galectin-9 functionally impairs natural killer cells in humans and mice. J Virol 2013; 87:4835-4845; PMID:23408620; http://dx.doi.org/10.1128/JVI.01085-12
- Wang F, He W, Zhou H, Yuan J, Wu K, Xu L, Chen ZK. The Tim-3 ligand galectin-9 negatively regulates CD8+ alloreactive T cell and prolongs survival of skin graft. Cell Immunol 2007; 250:68-74; PMID:18353298; http://dx.doi.org/10.1016/j.cellimm.2008.01.006
- Akira S, Takeda K. Toll-like receptor signalling. Nat Rev Immunol 2004; 4:499-511; PMID:15229469; http://dx.doi.org/10.1038/nri1391
- Broudy VC. Stem cell factor and hematopoiesis. Blood 1997; 90:1345-1364; PMID:9269751
- Hsu YL, Wang MY, Ho LJ, Huang CY, Lai JH. Up-regulation of galectin-9 induces cell migration in human dendritic cells infected with dengue virus. J Cell Mol Med 2015; 19:1065-1076; PMID:25754930; http://dx.doi.org/10.1111/jcmm.12500
- Khaznadar Z, Henry G, Setterblad N, Agaugue S, Raffoux E, Boissel N, Dombret H, Toubert A, Dulphy N. Acute myeloid leukemia impairs natural killer cells through the formation of a deficient cytotoxic immunological synapse. Eur J Immunol 2014; 44:3068-3080; PMID:25041786; http://dx.doi.org/10.1002/eji.201444500
- Tandon R, Chew GM, Byron MM, Borrow P, Niki T, Hirashima M, Barbour JD, Norris PJ, Lanteri MC, Martin JN et al. Galectin-9 is rapidly released during acute HIV-1 infection and remains sustained at high levels despite viral suppression even in elite controllers. AIDS Res Hum Retroviruses 2014; 30:654-664; PMID:24786365; http://dx.doi.org/10.1089/aid.2014.0004
- Chabot S, Kashio Y, Seki M, Shirato Y, Nakamura K, Nishi N, Nakamura T, Matsumoto R, Hirashima M. Regulation of galectin-9 expression and release in Jurkat T cell line cells. Glycobiology 2002; 12:111-118; PMID:11886844; http://dx.doi.org/10.1093/glycob/12.2.111
- Yoon SJ, Lee MJ, Shin DC, Kim JS, Chwae YJ, Kwon MH, Kim K, Park S. Activation of mitogen activated protein kinase-Erk kinase (MEK) increases T cell immunoglobulin mucin domain-3 (TIM-3) transcription in human T lymphocytes and a human mast cell line. Mol Immunol 2011; 48:1778-1783; PMID:21621846; http://dx.doi.org/10.1016/j.molimm.2011.05.004
- Sahin U, Weskamp G, Kelly K, Zhou HM, Higashiyama S, Peschon J, Hartmann D, Saftig P, Blobel CP. Distinct roles of ADAM10 and ADAM17 in ectodomain shedding of six EGFR ligands. J Cell Biol 2004; 164:769-779; PMID:14993236; http://dx.doi.org/10.1083/jcb.200307137
- Porakishvili N, Roschupkina T, Kalber T, Jewell AP, Patterson K, Yong K, Lydyard PM. Expansion of CD4+ T cells with a cytotoxic phenotype in patients with B-chronic lymphocytic leukaemia (B-CLL). Clin Exp Immunol 2001; 126:29-36; PMID:11678896; http://dx.doi.org/10.1046/j.1365-2249.2001.01639.x
- Moritoki M, Kadowaki T, Niki T, Nakano D, Soma G, Mori H, Kobara H, Masaki T, Kohno M, Hirashima M. Galectin-9 ameliorates clinical severity of MRL/lpr lupus-prone mice by inducing plasma cell apoptosis independently of Tim-3. PLoS One 2013; 8:e60807; PMID:23585851; http://dx.doi.org/10.1371/journal.pone.0060807
- Wang C, Liu J, Wang L, Geng X. Solubilization and refolding with simultaneous purification of recombinant human stem cell factor. Appl Biochem Biotechnol 2008; 144:181-189; PMID:18456949; http://dx.doi.org/10.1007/s12010-007-8112-0
- Yasinska IM, Gibbs BF, Lall GS, Sumbayev VV. The HIF-1 transcription complex is essential for translational control of myeloid hematopoietic cell function by maintaining mTOR phosphorylation. Cell Mol Life Sci 2014; 71:699-710; PMID:23872956; http://dx.doi.org/10.1007/s00018-013-1421-2
- Chaplen FW, Fahl WE, Cameron DC. Detection of methylglyoxal as a degradation product of DNA and nucleic acid components treated with strong acid. Anal Biochem 1996; 236:262-269; PMID:8660503; http://dx.doi.org/10.1006/abio.1996.0165
- Chaplen FW, Fahl WE, Cameron DC. Method for determination of free intracellular and extracellular methylglyoxal in animal cells grown in culture. Anal Biochem 1996; 238:171-178; PMID:8660607; http://dx.doi.org/10.1006/abio.1996.0271
- Thornalley PJ. The glyoxalase system in health and disease. Mol Aspects Med 1993; 14:287-371; PMID:8277832; http://dx.doi.org/10.1016/0098-2997(93)90002-U