ABSTRACT
Tigilanol tiglate is an oncolytic small molecule that is undergoing clinical trials. A recent study revealed the capacity of this pyroptosis inducer to elicit hallmarks of immunogenic cell death. In addition, intratumoral injection of tigilanol tiglate can sensitize subcutaneous cancers to subsequent immune checkpoint inhibitors targeting CTLA-4 alone or in combination with PD-1.
Main text
In the ever-evolving landscape of cancer therapy, the pursuit of interventions that amplify cancer immunosurveillance stands as a cornerstone in the quest for enhanced efficacy. Among these strategies, the induction of immunogenic cell death (ICD) emerges as a promising avenue. ICD, characterized by the release of danger signals from dying cancer cells, serves as a potent stimulant for the immune system, triggering a cascade of events that culminate in the recognition and elimination of tumor cells by effector/cytotoxic lymphocytes.Citation1
The concept of ICD encompasses a spectrum of cellular demise pathways endowed with immunostimulatory properties. Apoptosis, the most well-characterized form of programmed cell death, has long been recognized for its immunologically silent nature. However, accumulated evidence unveiled the immunogenic potential of apoptotic cell death when accompanied by the exposure or release of danger-associated molecular patterns (DAMPs) such as calreticulin, annexin A1, ATP, and high mobility group box 1 (HMGB1). Similarly, necroptosis, ferroptosis, or pyroptosis, have emerged as alternative immunogenic cell demises, offering novel targets for therapeutic intervention.Citation2,Citation3
Intratumoral injection of immunostimulatory factors has gained considerable attention in recent years to reach improved tumor debulking together with limited systemic adverse events. Such local therapies are attempted with a wide range of agents, including adopted immune cells, antibodies, cytokines, pattern recognition receptor (PRR) agonists, vaccines, as well as ICD-inducing oncolytic viruses, peptides, and small molecules.Citation4–7
Along this line, tigilanol tiglate (TT) is a natural activator of the protein kinase C (PKC) family members that is approved for intratumoral treatment of cutaneous cancers in dogs and currently under investigation in humans. Mechanistically, preclinical studies have shown that intralesional administration of TT triggers tumor ablation by disrupting local vasculature and promoting hemorrhagic necrosis. Yet, its local administration has been associated with antitumor effects of distant untreated (“abscopal”) lesions in a Phase I clinical trial, thus indicating that TT has immunosurveillance-enhancing potential.Citation8
In a recent study published in the Journal for the Immunotherapy of Cancer, Cullen and colleagues investigated the immunogenic properties of tigilanol tiglate (TT).Citation9 In squamous cell carcinoma and melanoma cell lines, TT successively promoted dissipation of the mitochondrial membrane potential, mitochondrial swelling, outer mitochondrial membrane permeabilization, mitochondrial degradation, cytoplasmic vacuolization, and cellular swelling prior to cell death. At the molecular level, the in vitro TT treatment triggered proteolytic activation of caspases, Bid, poly [ADP-ribose] polymerase (PARP) as well as of gasdermin E (GSDME). Thus, TT apparently induces caspase-dependent GSDME-mediated pyroptosis. Of note, this effect seemed largely PKC-independent. Cellular assimilation of TT was accompanied by the phosphorylation of Ire1α and eiF2α, indicating the activation of the endoplasmic reticulum (ER)/mitochondrial unfolded and integrated stress responses. Importantly, treatment with TT stimulated ROS production, release of ATP and HMGB1 into the extracellular milieu, and externalization of the ER chaperone calreticulin. Moreover, whereas type I interferon (IFN-I) secretion was not detected, production of pro-inflammatory cytokines like interleukin (IL)6, IL8, C-X-C motif chemokine ligand (CXCL)9, and CXCL10 was detected. In vivo, mouse vaccination with cancer cells treated with a high (but not low) dose of TT protected against rechallenge with live malignant cells. Collectively, these data supported the immunogenicity of TT-induced pyroptotic cell death. Accordingly, in the therapeutic B16-F10 melanoma model, intratumoral delivery of TT stimulated infiltration by T lymphocytes of this otherwise immune cold tumor. Furthermore, such intervention reverted resistance to dual immunotherapy blocking the immune checkpoint cytotoxic T lymphocyte-associated protein 4 (CTLA-4), alone or in combination with programmed cell death protein 1 (PDCD1, best known as PD-1) ().Citation9
Figure 1. Tigilanol tiglate promotes pyroptotic cell death and sensitizes to immune checkpoint inhibitors. IT administration of TT initiates mitochondrial and ER stress responses, leading to generation of ROS, induction of the ISR as illustrated by the phosphorylation of eIf2alpha, caspase activation, and subsequent GSDME-mediated pyroptotic cell death. This cell demise shows immunogenicity due to the release/surface exposure of DAMPs (e.g., calreticulin, ATP, HMGB1) together with pro-inflammatory cytokines (e.g., IL6, IL8, CXCL9, CXCL10). This cascade of events triggers a tumor-specific T cell response which can be enhanced by systemic immunotherapy with inhibitors of the CTLA-4 and PD-1 immune checkpoints. CTLA-4, cytotoxic T lymphocyte associated protein 4; CXCL, chemokine (C-X-C motif) ligand; DAMP, damage-associated molecular pattern; ER, endoplasmic reticulum; GSDME, gasdermin E; HMGB1, high mobility group box 1; IL, interleukin; ISR, integrated stress response; IT, intratumoral; PD-1, programmed cell death protein 1; ROS, reactive oxygen species; TT, tigilanol tiglate. Created with BioRender.com.
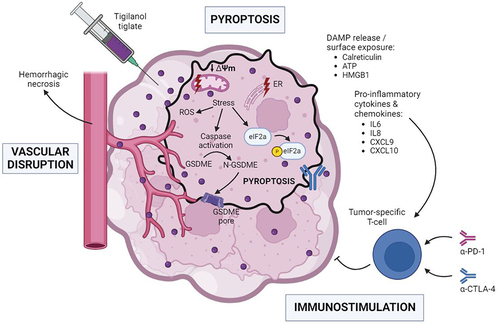
In conclusion, the induction of ICD through intratumoral delivery of TT or other oncolytic agents presents a promising avenue for cancer treatments. This approach holds the potential to invigorate cancer immunosurveillance, particularly in immune cold tumors, which typically exhibit resistance to conventional immunotherapies. By triggering ICD, TT may effectively convert immune cold into hot tumors, thereby restoring their responsiveness to systemic immune checkpoint blockers that are widely used in clinical practice.Citation5,Citation10 Thus, TT enters a competitive space in which a number of oncolytic agents are being compared for their capacity to elicit clinically relevant anticancer immune responses that can be amplified by blockade of immune checkpoints.
Acknowledgments
J.G.P is supported by the SIRIC Cancer Research and Personalized Medicine (CARPEM); Multi-Organism Institute (ITMO) Aviesan Cancer (National Alliance for Life Sciences and Health), Institut National du Cancer (INCa), and Fondation pour la Recherche Médicale (FRM). GK is supported by the Ligue contre le Cancer (équipe labellisée); Agence National de la Recherche (ANR-22-CE14-0066 VIVORUSH, ANR-23-CE44-0030 COPPERMAC, ANR-23-R4HC-0006 Ener-LIGHT); Association pour la recherche sur le cancer (ARC); Cancéropôle Ile-de-France; Fondation pour la Recherche Médicale (FRM); a donation by Elior; European Joint Programme on Rare Diseases (EJPRD) Wilsonmed; European Research Council Advanced Investigator Award (ERC-2021-ADG, Grant No.101052444; project acronym: ICD-Cancer, project title: Immunogenic cell death (ICD) in the cancer-immune dialogue); The ERA4 Health Cardinoff Grant Ener-LIGHT; European Union Horizon 2020 research and innovation programmes Oncobiome (grant agreement number: 825410, Project Acronym: ONCOBIOME, Project title: Gut OncoMicrobiome Signatures [GOMS] associated with cancer incidence, prognosis, and prediction of treatment response, Prevalung (grant agreement number 101095604, Project Acronym: PREVALUNG EU, project title: Biomarkers affecting the transition from cardiovascular disease to lung cancer: towards stratified interception), Neutrocure (grant agreement number 861878: Project Acronym: Neutrocure; project title: Development of “smart” amplifiers of reactive oxygen species specific to aberrant polymorphonuclear neutrophils for treatment of inflammatory and autoimmune diseases, cancer, and myeloablation); National support managed by the Agence Nationale de la Recherche under the France 2030 programme (reference number 21-ESRE-0028, ESR/Equipex+ Onco-Pheno-Screen); Hevolution Network on Senescence in Aging; Institut National du Cancer (INCa); Institut Universitaire de France; LabEx Immuno-Oncology ANR-18-IDEX-0001; a Cancer Research ASPIRE Award from the Mark Foundation; PAIR-Obésité INCa_1873, the RHUs Immunolife and LUCA-pi (ANR-21-RHUS-0017 and ANR-23-RHUS-0010, both dedicated to France Relance 2030); Seerave Foundation; SIRIC Cancer Research and Personalized Medicine (CARPEM). This study contributes to the IdEx Université de Paris Cité ANR-18-IDEX-0001. Views and opinions expressed are those of the author(s) only and do not necessarily reflect those of the European Union, the European Research Council or any other granting authority. Neither the European Union nor any other granting authority can be held responsible for them.
Disclosure statement
J.G.P. is the inventor of patents covering the diagnosis, prognosis, and treatment of cancers, including patents licensed to Turstone Biologics and Therafast Bio. GK has been holding research contracts with Daiichi Sankyo, Eleor, Kaleido, Lytix Pharma, PharmaMar, Osasuna Therapeutics, Samsara Therapeutics, Sanofi, Sutro, Tollys, and Vascage. GK is on the Board of Directors of the Bristol Myers Squibb Foundation France. GK is a scientific co-founder of everImmune, Osasuna Therapeutics, Samsara Therapeutics and Therafast Bio. GK is in the scientific advisory boards of Hevolution, Institut Servier, Longevity Vision Funds and Rejuveron Life Sciences. GK is the inventor of patents covering therapeutic targeting of aging, cancer, cystic fibrosis and metabolic disorders. GK’s wife, Laurence Zitvogel, has held research contracts with Glaxo Smyth Kline, Incyte, Lytix, Kaleido, Innovate Pharma, Daiichi Sankyo, Pilege, Merus, Transgene, 9 m, Tusk and Roche, was on the on the Board of Directors of Transgene, is a cofounder of everImmune, and holds patents covering the treatment of cancer and the therapeutic manipulation of the microbiota. GK’s brother, Romano Kroemer, was an employee of Sanofi and now consults for Boehringer-Ingelheim. The funders had no role in the design of the study; in the writing of the manuscript or in the decision to publish the results.
Additional information
Funding
References
- Kroemer G, Chan TA, Eggermont AMM, Galluzzi L. Immunosurveillance in clinical cancer management. CA Cancer J Clin. 2024;74(2):187–3. doi:10.3322/caac.21818.
- Catanzaro E, Demuynck R, Naessens F, Galluzzi L, Krysko DV. Immunogenicity of ferroptosis in cancer: a matter of context? Trends Cancer. 2024;10(5):407–416. doi:10.1016/j.trecan.2024.01.013.
- Meier P, Legrand AJ, Adam D, Silke J. Immunogenic cell death in cancer: targeting necroptosis to induce antitumour immunity. Nat Rev Cancer. 2024;24(5):299–315. doi:10.1038/s41568-024-00674-x.
- Kepp O, Marabelle A, Zitvogel L, Kroemer G. Oncolysis without viruses – inducing systemic anticancer immune responses with local therapies. Nat Rev Clin Oncol. 2020;17(1):49–64. doi:10.1038/s41571-019-0272-7.
- Yamazaki T, Pitt JM, Vetizou M, Marabelle A, Flores C, Rekdal Ø, Kroemer G, Zitvogel L. The oncolytic peptide LTX-315 overcomes resistance of cancers to immunotherapy with CTLA4 checkpoint blockade. Cell Death Differ. 2016;23(6):1004–1015. doi:10.1038/cdd.2016.35.
- Xie W, Mondragon L, Mauseth B, Wang Y, Pol J, Levesque S, Zhou H, Yamazaki T, Eksteen JJ, Zitvogel L. et al. Tumor lysis with LTX-401 creates anticancer immunity. Oncoimmunol. 2019;8(7):1594555. doi:10.1080/2162402X.2019.1594555.
- Shalhout SZ, Miller DM, Emerick KS, Kaufman HL. Therapy with oncolytic viruses: progress and challenges. Nat Rev Clin Oncol. 2023;20(3):160–177. doi:10.1038/s41571-022-00719-w.
- Panizza BJ, de Souza P, Cooper A, Roohullah A, Karapetis CS, Lickliter JD. Phase I dose-escalation study to determine the safety, tolerability, preliminary efficacy and pharmacokinetics of an intratumoral injection of tigilanol tiglate (EBC-46). EBioMedicine. 2019;50:433–441. doi:10.1016/j.ebiom.2019.11.037.
- Cullen JK, Yap PY, Ferguson B, Bruce ZC, Koyama M, Handoko H, Hendrawan K, Simmons JL, Brooks KM, Johns J. et al. Tigilanol tiglate is an oncolytic small molecule that induces immunogenic cell death and enhances the response of both target and non-injected tumors to immune checkpoint blockade. J Immunother Cancer. 2024;12(4):12. doi:10.1136/jitc-2022-006602.
- Sharma P, Goswami S, Raychaudhuri D, Siddiqui BA, Singh P, Nagarajan A, Liu J, Subudhi SK, Poon C, Gant KL. et al. Immune checkpoint therapy—current perspectives and future directions. Cell. 2023;186(8):1652–1669. doi:10.1016/j.cell.2023.03.006.