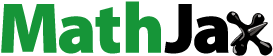
Abstract
Tuberculosis has been a major health problem worldwide for years; therefore, it is important to develop and produce an effective vaccine against this disease. In this study, the immunogenicity of Mycobacterium tuberculosis fusion protein (FP) encapsulated in liposomes containing DDA/TDB was evaluated. The FP was expressed in E. coli BL21 and encapsulated in liposomal formulations. Three weeks after the last subcutaneous immunization, IFN-γ, IL-4, IL-17, and IL-12 in spleen cell culture supernatants, and IgG2a, IgG1, and IgG2b titres in sera were measured. The greatest IFN-γ and IL-12 interleukin concentrations were observed in the DDA/TDB/CHOL liposomes containing the FP. Initial injection with BCG improved the efficacy of the DDA/TDB/CHOL/FP vaccine. The IgG2a/IgG1 ratio was also high in the DDA/TDB/CHOL/FP group; furthermore, the IgG2a/IgG1 ratio was increased in the BCG-primed, DDA/TDB/CHOL/FP-boosted group, indicating induction of a cellular immune response. Our study showed that the FP-containing DDA/TDB/CHOL liposomes induced a Th1 response. However, the groups that first received BCG and then DDA/TDB/CHOL/FP had the greatest Th1 response in terms of IFN-γ and IL-12 production of all the groups. This suggests that these formulations enhance the BCG vaccine’s effectiveness.
Introduction
Despite the development of therapeutic medicines over the past few decades, tuberculosis (TB) remains the second-leading cause of single-agent infectious mortality and the leading cause of multi-agent infectious mortality worldwide [Citation1,Citation2]. Bacillus Calmette-Guerin (BCG) has been used since 1921 and is still prescribed for the vaccination of infants in most countries [Citation3]. The efficacy of this vaccine has not been completely clarified and consensus exists only regarding the vaccination of newborns. The vaccine’s efficacy in infants varies from 0–80% [Citation4]. Two strategies have been investigated to design and manufacture TB vaccines; one is to replace BCG with other vaccines to stimulate cellular immunity, and the other is to apply various other vaccines to boost BCG’s effect. In this regard, various vaccines including subunit, vector, and DNA vaccines have been designed and studied [Citation5].
Subunit vaccines containing purified antigens have been extensively studied with a major advantage of having fewer side effects than other vaccine types [Citation6,Citation7]. The general strategy regarding immunization with subunit vaccines is to use dominant and major antigens to stimulate the immune system [Citation8]. HspX, PPE44, and EsxV have recently been tested as TB vaccine candidates [Citation9–11]. PPE44 belongs to the Pro-Pro-Glu (PPE) protein family and is less abundant in BCG than in H37Rv Mycobacterium (M.) tuberculosis. Additionally, PPE44 subunit vaccines have provided stronger protection in mice against M. tuberculosis than BCG [Citation10]. HspX is another dominant M. tuberculosis antigen. In M. tuberculosis-infected individuals, HspX induces interferon gamma (IFN-γ) production in T cells. HspX also induces interleukin 12 (IL-12) release which drives cells towards a TH1 response [Citation12]. It has also been reported that Esx proteins are suitable vaccine candidates because they are specific T cell targets [Citation13]. Generally, when administered alone, purified recombinant proteins only slightly stimulate the immune system; therefore, an appropriate adjuvant is necessary to produce an effective vaccine [Citation14,Citation15]. Liposomes are microscopic vesicles composed of phospholipid bilayers that can act as immunoadjuvants for protein antigens. Several studies have shown that cationic liposomes stimulate cellular immunity significantly more than neutral or anionic liposomes [Citation16–20]. Dimethyldioctadecylammonium (DDA) is a positively charged lipid and effective adjuvant that belongs to the lipophilic quaternary amines group [Citation21]. It has been reported that the combination of DDA and trehalose-6,6'-dibehenate (TDB) induces a strong immune response against M. tuberculosis infection [Citation15]. In addition, TDB stimulates antigen-presenting cells (APCs) and induces strong Th1 and Th17 responses [Citation22–24]. The aim of this study was to prepare and characterize an appropriate DDA/TDB liposome formulation containing M. tuberculosis HspX, PPE44, and EsxV proteins and evaluate its immunogenicity in mice in the presence and absence of BCG.
Materials and methods:
Cloning and expression of fusion proteins
Briefly, for fusion protein (FP) synthesis, HspX, PPE44, and EsxV gene sequences were derived from the NCBI site and their protein sequences from the Uniprot site. Two AEAAAKEAAAKA linkers were inserted between the three protein sequences and the nucleotide sequence was optimized by for expression in Escherichia (E.) coli using JCat software. The sequence encoding the FP was then inserted into plasmid pET21b. E. coli Top10 BL21 cells were transformed with the plasmid, expression was induced, and the recombinant protein was purified by Ni-NTA chromatography (QIAGEN). The protein concentration was determined with a Bicinchoninic Acid (BCA) Protein Assay Kit (Thermo Scientific, USA) and the purified protein was analyzed by sodium dodecyl sulphate-polyacrylamide gel electrophoresis (SDS-PAGE) and Western blotting [Citation25].
Liposome preparation
DDA/TDB liposome formulations with or without cholesterol were prepared by the thin film method. Briefly, cholesterol (CHOL) (3 µmol/ml), DDA (4 µmol/ml), and TDB (0.5 µmol/ml) for each formulation were dissolved in chloroform-methanol (2:1) and transferred into borosilicate glass tubes. The tubes were connected to a rotary evaporator to remove the organic solvent, which formed a thin lipid layer at the bottom of the tube. To remove the organic solvent, the lipid film was freeze-dried [Citation26]. Finally, the lipid film was hydrated in NaCl-histidine buffer (140 mM NaCl containing 10 mM histidine, pH 6.5) with or without the FP (1 mg/ml) at 47 °C. After multiple vortexing, liposomes were sonicated for 5 min at 47 °C and extruded through 400, 200, and 100 nm polycarbonate membrane filters at 47 °C.
Liposome characterization
A dynamic light scattering instrument (Nano-ZS; Malvern, UK) was used to measure the liposomes’ mean sizes and zeta potentials. Particle sizes and polydispersity indexes (PI) were reported as means ± standard deviations (n = 3). Zeta potentials were reported as means ± zeta deviations (n = 3).
Indirect evaluation of protein encapsulation efficiency
To determine the amount of antigen encapsulation in formulations, Amicon® Ultra-4 Centrifugal Filter Units (100 kDa) were used. The encapsulated formulations were centrifuged in Amicon filters at 2000×g for 30 min and the protein percent encapsulation was calculated using the following formula:
Vaccination protocol
Sixty 6–8 week-old female Balb/C mice were purchased from the Pasteur Institute of Iran (Tehran, Iran), divided into 10 groups of six mice per group, and immunized with the formulations shown in . Based on previous studies, each mouse received 50 μg of antigen on days 0, 14, and 28 [Citation27]. Two groups of mice were primed with BCG on day 0 and then boosted with either DDA/TDB/CHOL/FP or FP on days 14 and 28.
Table 1. Mouse injection groups and quantities.
Cell culture
Three weeks after the last injection the mice were killed by cervical dislocation. The spleens were transferred to microtubes containing 1 ml of cold RPMI medium without antibiotics. The spleens were crushed using a cell strainer and the cells were inserted into falcon tubes with 4–5 ml of cold RPMI medium. The spleen cells were centrifuged at 300×g for 5 min at 4 °C, the supernatants were removed, and 10 ml of cold ammonium chloride buffer was added and slowly mixed with the cell pellet to lyse the red blood cells. The cells were washed with 5 ml of cold RPMI and counted. To each 24-well plate, 3 × 106 cells were added in 5 ml of complete RPMI media containing 10% FBS and 1% penicillin-streptomycin. Either 5 μg/ml of the fusion antigen or 3% PHA as a positive control was added. The plates were incubated in a CO2 incubator for 72 h, culture supernatants were collected, transferred to a sterile microtube, and centrifuged at 3000×g for 2 min at 4 °C. The culture supernatants were stored at −80 °C until use.
Cytokine assay
Enzyme-linked immunosorbent assays (ELISAs) were used to measure IFNγ, IL-12, IL-4 and IL-17A cytokine concentrations. In brief, 100 μl of the diluted capture antibody with a coating buffer was added to the wells of 96-well plates and incubated overnight at 4 °C. After washing with 300 μl of PBS buffer containing 0.05% Tween 20, the blocking solution was added to the wells and the plates were incubated overnight at 4 °C. After washing, various dilutions of the standard and FP-stimulated cell supernatants were added to the wells. Then, 100 μl of the diluted detection antibody was added to each well and the plates were incubated at room temperature with shaking for 2 h. After that, 100 μl of diluted streptavidin-HRP was added to each well and the plate was incubated for 30 min at room temperature. After washing, 100 μl of TMB substrate solution was added per well and the plate was incubated in the dark at room temperature for 15 min. Finally, 50 μl of stop solution was added to the wells and their optical densities (ODs) were measured on an ELISA reader at 450 nm with background subtraction at 630 nm.
Antibody isotype assay
Blood was collected before killing the mice, and their serum was isolated and stored at −20 °C. IgG2a, IgG2b, and IgG1 concentrations were measured by ELISA according to the manufacturer's instructions. A 96-well plate was coated with 100 μl of PBS buffer containing 5 μg of the FP per well and incubated overnight at 4 °C. After washing, the wells were blocked with 200 μl of blocking buffer containing 2% BSA per well and incubated overnight at 4 °C. Serum samples diluted 1/200, 1/2,000, and 1/20,000 were added to the wells. After washing, 100 μl of the detection antibodies were added to the wells and the plate was incubated at room temperature with shaking for 2 h. After washing, 100 μl of TMB substrate solution was added to each well, the plate was incubated for 15 min at room temperature, and 50 μl of stop solution was added to each well. Optical densities were measured on an ELISA reader at 450 nm with background subtraction at 630 nm.
Statistical analysis
One-way analysis of variance (ANOVA) was used to compare the control and experimental groups, and the Tukey–Kramer test was used to compare every two groups using SPSS (version 16). p < .05 was considered significant.
Results
Fusion protein characterization
The expressed FP contained 642 amino acids with an isoelectric pH of 4.74 and a molecular weight of about 70 kDa. After Ni-NTA purification and dialysis with 10 mM histidine buffer, the FP concentration was determined by BCA protein assay to be 1 mg/ml .
Liposome characterization
The liposome particle sizes, zeta potentials, and polydispersity indexes are shown in . The TDB/DDA/CHOL empty control liposome size was 205 ± 44.6 nm; however, encapsulation of the FP increased the size significantly. This could be due to the negatively charged FP at pH 6.5 (pI 4.74) electrostatically interacting with both the inner and outer leaflets of the positively charged DDA liposomes. The interaction between the FP and the outer leaflet could mediate liposome aggregation. The TDB/DDA/CHOL empty control liposome zeta potential was 56 mV due to the positively charged DDA in the formulation. Encapsulation of the FP in the liposomes reduced the zeta potential. This reduction correlated with the percent encapsulation, which indicates that the FP interacts electrostatically with the liposome surface ().
Table 2. Liposome sizes, zeta potentials, polydispersity indexes (PDI), and fusion protein encapsulation efficiencies in the various liposomal formulations (means ± SDs, n = 3).
Liposomal encapsulation efficiency
The encapsulation efficiencies of the FP in DDA/TDB/CHOL/FP, DDA/CHOL/FP, DDA/TDB/FP, and DDA/FP liposomes were 75, 81, 87, and 94%, respectively (). This high encapsulation efficacy is due to the negatively charged FP at pH 6.5 (pI = 4.74) electrostatically interacting with positively charged DDA on both the inner and outer leaflets of the liposomes.
Cytokine assay
The IFN-γ concentrations in all the vaccinated groups (), except the DDA/CHOL/FP and FP groups, were significantly greater than that of the histidine buffer-vaccinated control group (p < .05). Moreover, the highest IFN-γ concentrations were observed in the BCG, BCG/DDA/TDB/CHOL/FP, and BCG/FP groups (). Despite the IFN-γ increase in the FP-encapsulated liposomes, the difference between the groups that received free vs. encapsulated protein was not significant (p > .05). The IL-17 concentrations were significantly greater in all groups than those of groups that received the histidine buffer and FP alone (). The highest IL-17 concentrations were observed in the DDA/TDB/CHOL/FP and BCG/DDA/TDB/CHOL/FP groups (). Similarly, the IL-12 concentrations were significantly greater in all groups except the DDA/Chol/FP, DDA/TDB/FP, and DDA/FP groups than those of the histidine buffer and FP alone groups (p < .05, ). The highest IL-12 concentration was observed in the BCG/DDA/TDB/CHOL/FP group ().The IL-4 concentrations were significantly greater in all groups than those of the histidine buffer and FP groups (p < .05). The highest IL-4 concentration was observed in the BCG/DDA/TDB/CHOL/FP group ().
Figure 1. Cytokine concentrations in immunized mice three weeks after the last injection. Mice were immunized three times at two-week intervals. Their spleens were removed and the splenocytes were cultured and stimulated in vitro with the HspX, PPE44, EsxV fusion protein (FP) (5 μg/ml). IFN-γ (A), IL-17 (B), IL-12 (C) and IL-4 (D) concentrations in splenocyte supernatants were measured by sandwich ELISAs after 72 h of in vitro incubation. DDA: Dimethyldioctadecylammonium; TDB: Trehalose-6,6'-dibehenate; CHO: Cholesterol; FP: Fusion protein; BCG: Bacillus Calmette-Guerin.
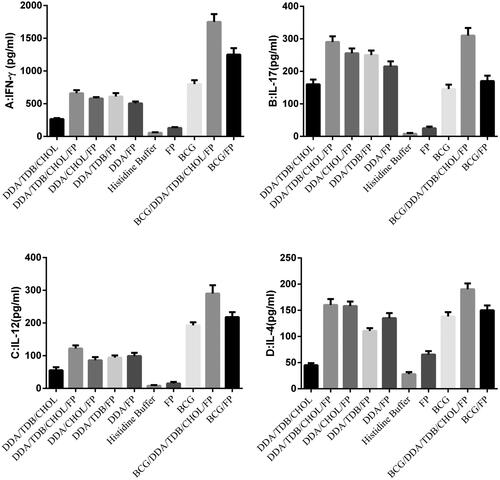
Antibody isotype assay
To assess humoral cellular immune responses, IgG1, and IgG2a and IgG2b, titers, respectively, were measured by ELISA in mouse sera diluted 1/200, 1/2,000, and 1/20,000 (. The IgG1 concentrations were significantly greater in all the groups than in the histidine buffer and DDA/TDB/CHOL control groups (p < .005). The highest IgG1 titres were observed in the DDA/TDB/FP and DDA/TDB/FP groups (). The IgG2a titres were significantly greater in the DDA/TDB/CHOL/FP, DDA/TDB/FP, DDA/FP, and BCG/DDA/TDB/CHOL/FP groups than in the histidine buffer control group (p < .005, ). The IgG2b titre was significantly greater in the BCG/DDA/TDB/CHOL/FP group than in the histidine buffer control group. Differences between other groups and the histidine buffer control group were not significant (). The IgG2a/IgG1 ratios in the DDA/TDB/CHOL, DDA/TDB/FP, BCG, BCG/DDA/TDB/CHOL/FP, and BCG/FP groups were greater than those of other groups, indicating a cellular immune response. The greatest IgG2a/IgG1 ratios occurred in the BCG alone and BCG-primed groups ().
Figure 2. IgG1 (A), IgG2a (B), and IgG2b (C) titers in sera of immunized BALB/c mice. Mice were immunized subcutaneously three times at two-week intervals. Blood samples were collected from the mice three weeks after the last Injection. The IgG1, IgG2a, and IgG2b titers were determined by ELISAs. The assays were performed in triplicate at 200, 2000 and 20,000 -fold dilutions for each serum sample. DDA: Dimethyldioctadecylammonium; TDB: Trehalose-6,6'-dibehenate; CHOL: Cholesterol; FP: Fusion protein; BCG: Bacillus Calmette-Guerin.
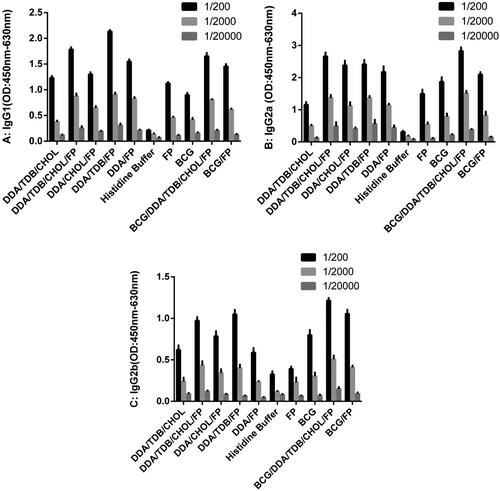
Table 3. IgG2a/IgG1 Ratioes of diluted serum from immunized mice (OD: 450 nm–630 nm).
Discussion
Because of the BCG vaccine’s infectiveness, especially in adult pulmonary TB, and because vaccination is the best-known way to control infectious diseases, developing an effective vaccination to prevent TB is a major priority in controlling this disease [Citation28]. Stimulation of a Th1-cell-mediated immune response is an important criterion to evaluate TB vaccines. Three antigens recently introduced as TB vaccine candidates are HspX, PPE44, and EsxV. Yuan et al. (2015) observed that the recombinant BCG strain expressing HspX and Ag85 antigens stimulated stronger CD4 and CD8 responses than BCG alone [Citation29]. Niu et al. (2015) showed that the application of HspX protein along with other proteins in a subunit vaccine (LT69) with DDA adjuvant provided a stronger and longer-lasting immune response than BCG vaccine alone [Citation30]. Previous studies have shown that EsxV and EsxW proteins are recognized by T cells and cause strong responses in TB patients. Additionally, Knudsen et al. (2013) demonstrated that these proteins are safe and important vaccine candidates [Citation31].
Moreover, Romano et al. emphasized the ability of PPE44 to stimulate both cellular and humoral immunities. It was also observed that this antigen-induced immunity to TB comparable to that of BCG [Citation32]. Most novel TB subunit vaccines are designed to prevent new infections, but cannot prevent reactivation of latent TB infections. It has been proposed that a novel vaccine should include antigens from different infectious stages [Citation33]. Furthermore, simultaneous vaccination with multiple M. tuberculosis antigens may improve the protective effects against all infectious stages [Citation5]. Therefore, our aim was to evaluate the immunogenicity of a novel multistage TB vaccine that combined EsxV and PPE44, which are acute-phase antigens, and HspX, a latent phase immunodominant antigen, to target both infectious stages. When administered alone, purified recombinant proteins stimulate the immune system poorly and do not provide long-term protection [Citation14]. Consequently, using adjuvants to stimulate the immune system and an antigen delivery system, such as liposomes, to effectively deliver antigens to antigen-presenting cells (APCs), could aid in the development of an effective vaccine. Here, besides providing an optimal method to express and purify a recombinant M. tuberculosis fusion protein [Citation34], we investigated the immunogenicity of this protein with liposomes and DDA and TDB adjuvants. Due to the liposomes’ sizes and specific physicochemical properties, they are considered as foreign bodies by the host and phagocytosed by the reticuloendothelial system. In fact, liposomes cause passive targeting of their contents to APCs and ultimately enhance immune responses [Citation35]. Liposome migration into the lymph nodes, and their ability to effectively provide their contents in a hydrophobic microenvironment into APCs induces the immune system [Citation36]. Studies have shown that liposomes can enhance cellular immune responses based on their phospholipid formulations [Citation37,Citation38]. In this study, we used liposomal nanoparticles with DDA and TDB as adjuvants. Recent studies have reported DDA as an effective adjuvant for stimulating cellular and humoral immunity. DDA is a synthetic amphiphilic lipid comprising a hydrophilic positively charged dimethylammonium head group attached to two alkali hydrocarbon chains. DDA tends to form bilayer vesicles similar to liposomes derived from natural phospholipids [Citation21]. A unique way to increase the stability and effectiveness of DDA as an adjuvant is to combine it with TDB; moreover, adding TDB to a cationic or neutral liposome would not change the liposome’s physicochemical properties. In fact, TDB is a synthetic trehalose-6,6-dimycolate (TDM) often related to cord factor extracted from the cell walls of Mycobacterium, and also a strong immune-stimulator of Th1 and Th17 pathways [Citation39]. TDB is less toxic and has shorter fatty acid chains than TDM [Citation40]. The liposomes containing DDA are physically unstable, even at 4 °C. Other vesicles change due to degradation or fusion, while liposomes containing small amounts of TDB are stable [Citation40]. Various studies have shown that positively charged liposomes stimulate cellular immunity more effectively than neutral or negatively charged ones [Citation7,Citation41,Citation42]. Because the antigen used in our study is negatively charged, the amount of antigen adsorbed is increased by the positively charged liposomes. In our study, the liposome sizes varied from 205 nm in DDA/TDB/CHOL to 1139 nm in DDA/FP; however, no significant differences in immune responses were observed between the groups, consistent with a study by Henriksen et al. [Citation43]. The encapsulation efficiency in all groups was high considering the positively charged liposome and the negatively charged protein; however, encapsulation efficiency was greater in the larger than in the smaller liposomes. The zeta potentials were positive in all the groups, indicating the composition of the formulations were appropriately chosen according to previous studies [Citation27] (). Cholesterol has been used to increase liposome stability; however, Perrie et al. (2013) showed that increasing cholesterol in liposomal formulations reduced antigen loading [Citation44]. Human and animal studies have shown that IFN-γ is the most important cellular immune cytokine in stimulating a protective response against M. tuberculosis. In addition, IL-12, produced by macrophages and dendritic cells, induces differentiation of naïve T cells into Th1 cells [Citation45]. In our study, the highest IFN-γ and IL-12 concentrations occurred in the groups initially vaccinated with BCG. Also, the highest concentrations of these two cytokines occurred in the BCG/DDA/TDB/CHOL/FP group.
Recent studies have also shown that IL-17, via stimulation of chemokine production, plays a major role in granuloma maturity and neutrophil migration to sites of infection [Citation46]. In our study, IL-17 was high in all groups except the control buffer and FP groups. Although Th17, in response to the studied vaccine in animal models, has been reported to have a contradictory role, one study showed that low IL-17 levels have no relation with the increased sensitivity to M. tuberculosis, while in another study, low IL-17 levels impaired granuloma formation and chemokine expression and ultimately increased the sensitivity to M. tuberculosis [Citation47,Citation48]. However, Becattini and co-workers showed that a combination of stimulatory antigens generates Th1, Th2, and Th17 memory cells with different frequencies. In fact, T cell clones can give rise to progeny with multiple fates, demonstrating that the main patterns of clonotype sharing between different Th cell subsets are characteristic for different antigens, and that Th1 cells may be produced indirectly from Th17 cells [Citation49]. In our study, all the mouse groups immunized with the liposomes and/or FP had significantly greater IL-17 concentrations than the controls. In addition, Khader and coworkers reported that IL-17 can act as an effector molecule against M. tuberculosis infection [Citation50]. Unlike Th1 and Th17 lymphocytes, Th2 lymphocytes suppress Th1 responses via IL-4 production and therefore can contribute to the progression of TB [Citation51]. However, low Th2 response levels seem to be able to prevent the immunopathological effects of protective cellular immunity and balance the host's immune system after infection. In this study, the high IL-4 levels in all except the histidine buffer and FP groups was consistent with other studies that showed that a Th1 protective response against M. tuberculosis is associated with elevated IL-4 expression, which inhibits Th2 responses [Citation52,Citation53]. T helper cells and cytokines such as IFN-γ and IL-4, which are respectively secreted by Th1 and Th2 cells, are related to IgG responses [Citation54]. IL-4 is associated with IgG1 response, whereas IFN-γ is associated with the production of IgG2a and IgG2b. Therefore, by evaluating the IgG2a/IgG1 ratio, it can be determined whether the immune response is directed toward the cellular or humoral pathway. In our study, the responses were Th1-directed. Furthermore, evidence suggests that specific antibodies may limit the dissemination of M. tuberculosis and may contribute, at least in part, to immunity. In addition, antibodies provide protection against intracellular pathogens by modulating immunity via Fc receptor-mediated phagocytosis [Citation55]. An important strategy to enhance the immunological memory of BCG is a prime/boost regimen. In this method, the mice were first primed with BCG and then a subunit vaccine alone or with a liposome was injected to boost the effect of BCG. Similar to other studies, in our study, the immunological memory following the BCG vaccine was enhanced [Citation39,Citation56,Citation57]. In this regard, our study showed that the mice that first received BCG, and then DDA/TDB/CHOL/FP or FP formulations, had the greatest Th1 responses in terms of IFN-γ and IL-12, indicating that these formulations can enhance the BCG effect. Our study showed that liposomes containing DDA or TDB, combined with fusion proteins, induce Th1 responses in mice. In future studies, these formulations should be investigated in an M. tuberculosis-infected mouse challenge model to assess this vaccine candidate.
Acknowledgments
This study was part of the Ph.D. thesis of Davood Mansury that was done in Nanotechnology Research Center and Antimicrobial Resistance Research Center, MUMS, Iran.
Disclosure statement
The authors report no conflicts of interest. The authors alone are responsible for the content and writing of this article.
Additional information
Funding
References
- Kastner E, Hussain MJ, Bramwell VW, et al. Correlating liposomal adjuvant characteristics to in-vivo cell-mediated immunity using a novel Mycobacterium tuberculosis fusion protein: a multivariate analysis study. J Pharm Pharmacol. 2015;67:450–463.
- Zumla A, George A, Sharma V, et al. The WHO 2014 global tuberculosis report—further to go. The Lancet Global Health. 2015;3:e10–ee2.
- Anderson ST, Kaforou M, Brent AJ, et al. Diagnosis of childhood tuberculosis and host RNA expression in Africa. N Engl J Med. 2014;370:1712–1723.
- Trunz BB, Fine P, Dye C. Effect of BCG vaccination on childhood tuberculous meningitis and miliary tuberculosis worldwide: a meta-analysis and assessment of cost-effectiveness. Lancet. 2006;367:1173–1180.
- Xin Q, Niu H, Li Z, et al. Subunit vaccine consisting of multi-stage antigens has high protective efficacy against Mycobacterium tuberculosis infection in mice. PLoS One. 2013;8:e72745. .
- Black M, Trent A, Tirrell M, et al. Advances in the design and delivery of peptide subunit vaccines with a focus on toll-like receptor agonists. Expert Rev Vaccines. 2010;9:157–173.
- Mohammed AR, Bramwell VW, Kirby DJ, et al. Increased potential of a cationic liposome-based delivery system: enhancing stability and sustained immunological activity in pre-clinical development. Eur J Pharma Biopharmaceut. 2010;76:404–412.
- Andersen P. TB vaccines: progress and problems. Trends Immunol. 2001;22:160–168.
- Teutschbein J, Schumann G, Möllmann U, et al. A protein linkage map of the ESAT-6 secretion system 1 (ESX-1) of Mycobacterium tuberculosis. Microbiol Res. 2009;164:253–259.
- Cuccu B, Freer G, Genovesi A, et al. Identification of a human immunodominant T-cell epitope of mycobacterium tuberculosis antigen PPE44. BMC Microbiol. 2011;11:167. .
- Caccamo N, Meraviglia S, La Mendola C, et al. Characterization of HLA‐DR‐and TCR‐binding residues of an immunodominant and genetically permissive peptide of the 16‐kDa protein of Mycobacterium tuberculosis. Eur J Immunol. 2004;34:2220–2229.
- Marongiu L, Donini M, Toffali L, et al. ESAT-6 and HspX improve the effectiveness of BCG to induce human dendritic cells-dependent Th1 and NK cells activation. PLoS One. 2013;8:e75684. .
- Bertholet S, Ireton GC, Kahn M, et al. Identification of human T cell antigens for the development of vaccines against Mycobacterium tuberculosis. J Immunol. 2008;181:7948–7957.
- O'Hagan DT, De Gregorio E. The path to a successful vaccine adjuvant-'the long and winding road'. Drug Discov Today. 2009;14:541–551.
- Holten-Andersen L, Doherty T, Korsholm K, et al. Combination of the cationic surfactant dimethyl dioctadecyl ammonium bromide and synthetic mycobacterial cord factor as an efficient adjuvant for tuberculosis subunit vaccines. Infect Immun. 2004;72:1608–1617.
- Verma I, Pandey R, Khuller G. Liposomes as adjuvant for anti-mycobacterial vaccine development. 2004;42(10):949-954.
- Barratt G, Tenu J-P, Yapo A, et al. Preparation and characterisation of liposomes containing mannosylated phospholipids capable of targetting drugs to macrophages. Biochim Biophys Acta Biomembr. 1986;862:153–164.
- Flynn JL. Immunology of tuberculosis and implications in vaccine development. Tuberculosis (Edinb). 2004;84:93–101.
- Cooper AM, Adams LB, Dalton DK, et al. IFN-gamma and NO in mycobacterial disease: new jobs for old hands. Trends Microbiol. 2002;10:221–226.
- Ottenhoff TH, Verreck FA, Hoeve MA, et al. Control of human host immunity to mycobacteria. Tuberculosis (Edinb). 2005;85:53–64.
- Hilgers LT, Snippe H. DDA as an immunological adjuvant. Res Immunol. 1992;143:494–503.
- Schoenen H, Bodendorfer B, Hitchens K, et al. Cutting edge: Mincle is essential for recognition and adjuvanticity of the mycobacterial cord factor and its synthetic analog trehalose-dibehenate. J Immunol. 2010;184:2756–2760.
- Agger EM, Rosenkrands I, Hansen J, et al. Cationic liposomes formulated with synthetic mycobacterial cordfactor (CAF01): a versatile adjuvant for vaccines with different immunological requirements. PLoS One. 2008;3:e3116.
- Werninghaus K, Babiak A, Gross O, et al. Adjuvanticity of a synthetic cord factor analogue for subunit Mycobacterium tuberculosis vaccination requires FcRgamma-Syk-Card9-dependent innate immune activation. J Exp Med. 2009;206:89–97.
- Amini Y, Tafaghodi M, Amel Jamehdar S, et al. Heterologous expression, purification, and characterization of the HspX, Ppe44, and EsxV proteins of Mycobacterium tuberculosis. Rep Biochem Molecular Biol. 2018;6:125–130.
- Feitosa E, Barreleiro P, Olofsson G. Phase transition in dioctadecyldimethylammonium bromide and chloride vesicles prepared by different methods. Chem Phys Lipids. 2000;105:201–213.
- Hojatizade M, Badiee A, Khamesipour A, et al. DDA/TDB liposomes containing soluble Leishmania major antigens induced a mixed Th1/Th2 immune response in BALB/c mice. Nanomed J. 2017;4:71–82.
- Andersen P, Doherty TM. Opinion: The success and failure of BCG–implications for a novel tuberculosis vaccine. Nat Rev Micro. 2005;3:656.
- Yuan X, Teng X, Jing Y, et al. A live attenuated BCG vaccine overexpressing multistage antigens Ag85B and HspX provides superior protection against Mycobacterium tuberculosis infection. Appl Microbiol Biotechnol. 2015;99:10587–10595.
- Niu H, Peng J, Bai C, et al. Multi-stage tuberculosis subunit vaccine candidate LT69 provides high protection against Mycobacterium tuberculosis infection in mice. PLoS One. 2015;10:e0130641.
- Knudsen NPH, Nørskov-Lauritsen S, Dolganov GM, et al. Tuberculosis vaccine with high predicted population coverage and compatibility with modern diagnostics. Proc Natl Acad Sci. 2014;111:1096–1101.
- Romano M, Rindi L, Korf H, et al. Immunogenicity and protective efficacy of tuberculosis subunit vaccines expressing PPE44 (Rv2770c). Vaccine 2008;26:6053–6063.
- Pepponi I, Diogo GR, Stylianou E, et al. Plant‐derived recombinant immune complexes as self‐adjuvanting TB immunogens for mucosal boosting of BCG. Plant Biotechnol J. 2014;12:840–850.
- Yousef Amini MT, Saeid AJ, Zahra M, et al. Heterologous expression, purification, and characterization of the HspX, Ppe44, and EsxV proteins of Mycobacterium tuberculosis. Rep Biochem Molecular Biol. 2017;6(2):125-130.
- Gregoriadis G. Immunological adjuvants: a role for liposomes. Immunol Today. 1990;11:89–97.
- Frezard F. Liposomes: from biophysics to the design of peptide vaccines. Brazilian J Med Biol Res. 1999;32(2):181-189.
- Jaafari MR, Badiee A, Khamesipour A, et al. The role of CpG ODN in enhancement of immune response and protection in BALB/c mice immunized with recombinant major surface glycoprotein of Leishmania (rgp63) encapsulated in cationic liposome. Vaccine. 2007;25:6107–6117.
- Badiee A, Jaafari MR, Samiei A, et al. Coencapsulation of CpG oligodeoxynucleotides with recombinant Leishmania major stress-inducible protein 1 in liposome enhances immune response and protection against leishmaniasis in immunized BALB/c mice. Clin Vaccine Immunol. 2008;15:668–674.
- Larrouy-Maumus G, Layre E, Clark S, et al. Protective efficacy of a lipid antigen vaccine in a guinea pig model of tuberculosis. Vaccine. 2017;35:1395–1402.
- Davidsen J, Rosenkrands I, Christensen D, et al. Characterization of cationic liposomes based on dimethyldioctadecylammonium and synthetic cord factor from M. tuberculosis (trehalose 6, 6′-dibehenate)—a novel adjuvant inducing both strong CMI and antibody responses. Biochimica et Biophysica Acta (BBA)-Biomembranes. 2005;1718:22–31.
- Shuttleworth SJ, Allin PM, Sharma K. Functionalised polymers: recent developments and new applications in synthetic organic chemistry. Synthesis. 1997;1997:1217–1239.
- Firouzmand H, Badiee A, Khamesipour A, et al. Induction of protection against leishmaniasis in susceptible BALB/c mice using simple DOTAP cationic nanoliposomes containing soluble Leishmania antigen (SLA). Acta Tropica. 2013;128:528–535.
- Henriksen-Lacey M, Devitt A, Perrie Y. The vesicle size of DDA: TDB liposomal adjuvants plays a role in the cell-mediated immune response but has no significant effect on antibody production. J Control Release. 2011;154:131–137.
- Kaur R, Henriksen-Lacey M, Wilkhu J, et al. Effect of incorporating cholesterol into DDA: TDB liposomal adjuvants on bilayer properties, biodistribution, and immune responses. Mol Pharmaceutics. 2014;11:197–207.
- Bhatt K, Salgame P. Host innate immune response to Mycobacterium tuberculosis. J Clin Immunol. 2007;27:347–362.
- Seiler P, Aichele P, Bandermann S, et al. Early granuloma formation after aerosol Mycobacterium tuberculosis infection is regulated by neutrophils via CXCR3‐signaling chemokines. Eur J Immunol. 2003;33:2676–2686.
- Khader SA, Pearl JE, Sakamoto K, et al. IL-23 compensates for the absence of IL-12p70 and is essential for the IL-17 response during tuberculosis but is dispensable for protection and antigen-specific IFN-gamma responses if IL-12p70 is available. J Immunol. 2005;175:788–795.
- Yoshida YO, Umemura M, Yahagi A, et al. Essential role of IL-17A in the formation of a mycobacterial infection-induced granuloma in the lung. J Immunol. 2010;184:4414–4422.
- Becattini S, Latorre D, Mele F, et al. T cell immunity. Functional heterogeneity of human memory CD4 T cell clones primed by pathogens or vaccines. Science. 2015;347:400–406.
- Khader SA, Bell GK, Pearl JE, et al. IL-23 and IL-17 in the establishment of protective pulmonary CD4+ T cell responses after vaccination and during Mycobacterium tuberculosis challenge. Nat Immunol. 2007;8:369.
- Rook GA, Hernandez-Pando R, Dheda K, et al. IL-4 in tuberculosis: implications for vaccine design. Trends in Immunol. 2004;25:83–88.
- Demissie A, Abebe M, Aseffa A, et al. Healthy individuals that control a latent infection with Mycobacterium tuberculosis express high levels of Th1 cytokines and the IL-4 antagonist IL. J Immunol. 2004;172:6938–6943. 4δ2.
- Ordway DJ, Costa L, Martins M, et al. Increased Interleukin-4 Production by CD8 and gammadelta T cells in health-care workers is associated with the subsequent development of active tuberculosis. J Infect Dis. 2004;190:756–766.
- Kara EE, Comerford I, Fenix KA, et al. Tailored immune responses: novel effector helper T cell subsets in protective immunity. PLoS Pathog. 2014;10:e1003905.
- Jacobs AJ, Mongkolsapaya J, Screaton GR, et al. Antibodies and tuberculosis. Tuberculosis (Edinb). 2016;101:102–113.
- Brandt L, Skeiky YA, Alderson MR, et al. The protective effect of the Mycobacterium bovis BCG vaccine is increased by coadministration with the Mycobacterium tuberculosis 72-kilodalton fusion polyprotein Mtb72F in M. tuberculosis-infected guinea pigs. Infect Immun. 2004;72:6622–6632.
- Goonetilleke NP, McShane H, Hannan CM, et al. Enhanced immunogenicity and protective efficacy against Mycobacterium tuberculosis of bacille Calmette-Guérin vaccine using mucosal administration and boosting with a recombinant modified vaccinia virus Ankara. J Immunol. 2003;171:1602–1609.