Abstract
Gold nanoparticles (AuNPs) is the most excellent anticancer theranostic nanoparticles synthesized through efficient, simple and green synthesis method using extracts of Trichosanthes kirilowii, extensively characterized by UV-spectroscopy, FT-IR and TEM techniques. The AuNPs, synthesized by means of T. kirilowii extracts identified that nanoparticles were ∼50 nm in size, which is an admirable nano dimension attained by green synthesis. In agreement with the outcome of microscopic cellular morphological observations, MTT assay showed effective, selective, anticarcinogenic effect of AuNPs on HCT-116 cells in a dose-dependent manner. The AuNPs significantly enhance ROS generation, cause mitochondrial membrane damage and induce morphological changes using AO/EtBr staining assay. Furthermore, AuNPs treatment induces G0/G1 phase cell-cycle arrest in HCT-116 cells. Also, AuNPs treatment activates caspase expression and downregulates the anti-apoptotic expression in HCT-116 cells. Our results point out that the phytoconsituents isolated from T. kirilowii can act as appropriate reducing and stabilizing agents in the properties of AuNPs; hereby, it leads to the green synthesis of an anti-carcinogenic agent with highly efficient potential for cancer treatment.
Introduction
Colon cancer is a lethal malignant tumour with an elevated incident rate in the age of 40–50, which is a severe cause of death. It accounts for 1.36 million new cases and 774,000 deaths every year all over the world [Citation1]. Chronic constipation, obesity, chronic inflammation in the gastrointestinal tract, alcohol consumption and high-fat diets are considered as a major cause of colorectal cancer [Citation2,Citation3]. A vast amount of research has been concentrated upon the precise mechanism of colon cancer, and the efficient preventive/therapeutic agent for colon cancer has become a focal point of remedial health investigations [Citation4]. Recently, therapeutic approach for colon cancer generally includes chemotherapy, surgery and immunotherapy [Citation5]. Among these, the intention of chemotherapy was to induce the apoptosis in colon cancer cells, thereby restrain the tumour growth, and clinically it is the most commonly applied approach [Citation6]. But, long-term exposure of chemotherapy results in severe systemic adverse effects and constricted therapeutic index [Citation7]. This provokes attention in the improvement of alernative strategies, including nanotechnology base therapeutics [Citation8].
Presently, drug delivery method such as nanoscale systems (nanoparticles, liposomes and micelles) have been growing steadily considering the response to cancer chemotherapy. It has enormous applications, such as controlled drug expulsion, increased drug uptake by cancer cells and the capacity to enhance drug stability and solubility [Citation9,Citation10]. Prominently, they could accumulate in the regions of tumor cells and deliver the respective drugs beneath the enhanced permeation and retention (EPR) effect. Also, these nanocarriers can accumulate and penetrate passively in tumor cells/tissues, and their therapeutic effects are far away from being acceptable [11,12]. Metal nanoparticles have been synthesized by means of biomolecules which is highly present in the biological sources, possess distinct physio-chemical properties, such as broad optical properties, low-cost nanosynthesis, surface functionalization and high surface-to-volume ratio which offered novel opportunity in the cancer treatment. A range of physical and chemical methods were generally used for the metal nanoparticle synthesis. These methods are cost-effective as well as non-ecofriendly, also this method of synthesis uses toxic chemicals [Citation13]. For that reason, green synthesis of nanoparticles, especially gold, is an area of interest. The major significant benefits of this green synthesis is lack of toxic solvents/reagents, waste generation and prevention of by-products during metal nanoparticle green synthesis; it also promotes the use of renewable natural resources [Citation14].
Among the metal nanoparticles, research scientists are focusing on AuNPs because of its exclusive properties like drug delivery into a particular region of cancer cells [Citation15]. In addition, it can act as a special microscopic probe on account of selective accumulation in cancer cells viewing bright-light scattering [Citation16]. It has wide-ranging applications including physical, medical, catalytic and various biological applications[Citation17]. In recent times, it has been materialized as an outstanding drug of target delivery for abundant therapeutic agents because of its constancy, lesser toxicity, biocompatibility and static nature. AuNPs were recently investigated as broadly used nanoparticles with respect to biocompatibility and cytotoxicity in connection with cellular interactions, it has been demonstrated that the AuNPs was low endocytic and had inhibition on cancer cell proliferation [Citation18,Citation19].
Trichosanthes kirilowii is an Cucurbitaceae family herb used in East Asian countries, it was investigated foremost in the old Chinese pharmaceutical monograph [Citation20]. Scientific researchs on phytoconstiuents specified that flavonoids, terpenoids, nitrogenous compounds and phytosterols might be the significant active compounds. In Chinese medicine (TCM), T. Kirilowii has been used broadly as anti-inflammatory, cytotoxic and antioxidant agent, and also for treating thoracic obstruction [Citation21]. In this study, we aimed to analyse the therapeutic anti-carcinogenic effect of AuNPs synthesized from T. Kirilowii in HCT-116 cells.
Materials and methods
HAuCl4, cell culture reagents, Dulbecco’s Modified Eagle Medium (DMEM, high glucose), fetal bovine serum (FBS), trypsin/EDTA, penicillin-streptomycine, Dulbecco’s phosphate buffer saline (PBS), DCFH-DA, acridine orange and ethidium bromide(AO/EB) Rhodamine-123, were obtained from Sigma Aldrich (St. Louis, MO, USA). Caspase 3&9, bcl-2, bax, bid monoclonal antibodies were purchased from Cell Signaling (Danvers, MA). All other chemicals utilized in this study were of analytical grade, and utilized without further purification.
Sample preparation
Fresh T. kirilowii plant were collected from Shandong, Hebei, Shanxi, China, and authenticated. The collected plant was thoroughly washed by running water and shade-dried for 10 days. The dried samples were powdered using an electronic grinder. 100 g of finely powdered samples were mixed with 1000 ml of distilled water and boiled for 30 min at 60 °C. Then, the sample was filtered and stored at 4 °C for further analysis.
Biosynthesis of AuNPs
A stock solution of 12.69 mM of gold chloroauric acid was prepared by dissolving gold precursor (500 mg) in 100 ml of deionized water. It was again diluted by deionized water to prepare gold chloroauric acid at 1.53 mM concentration. The AuNPs were synthesized by mixing 10 ml of gold chloroauric acid (aqueous) at room temperature and 10 ml of T. kirilowii extract, then mixed with deionized water (50 ml). The progress in gold ions reduction via T. Kirilowii extract to synthesize AuNPs was observed via UV–Vis spectroscopy. Morphological appearance, size and stability of the synthesized AuNPs were examined by taking aliquot of samples from the reaction mixture, subsequent to definite time intervals for the period of reduction reaction. The size and shape is determined by using TEM and the compounds are detected by FTIR.
Characterization of AuNPs
The T. kirilowii AuNPs were exemplified in order to evaluate their characteristics and most excellent scientific applications. The reaction progress of T. Kirilowii AuNPs was assessed through a mixture of scanning reaction in the range 400–700 nm by Lambda Perkin Elmer UV-T. kirilowii AuNPs vis spectrophotometer. The distribution of nanoparticle’s size/stability of synthesized T. kirilowii AuNPs was determined by Malvern, Zeta-sizer Nano ZSP. Morphological appearance and particle size were measured by Transmission Electron Microscopy (TEM). X ray diffraction studies were carried out using XRD–6000/6100 model XRD instrument. FTIR spectrophotometer used to identify the probable functional groups might be responsible for reduction reaction and AuNPs stabilization.
Cytotoxicity determination
The colorimetric MTT assay was performed to assess the cellular toxic effect of AuNPs in vitro. HCT-116 colon cancer cells and normal fibroblast 3T3 cells were loaded in a 96 numbered well plates, incubated in 5%CO2 at 37 °C upto 24 h. HCT-116 cells were treated with various concentrations of AuNPs, incubated at 37 °C upto 72 h. Later, medium was discarded and then 20 μL of MTT solution (4 mg/ml) was added to all the wells. Further, the well plates were incubated upto 4 h at 37 °C. Finally, the MTT medium was detached from each well without disturbing the cells and DMSO (100 μL) was added into each well in order to dissolve the formazan crystals. The absorbance was determined by using a microplate reader at 570 nm. This assay was done independently four times, and each independent experiment was performed in a triplicate manner.
Determination of mitochondrial membrane potential (MMP)
MMP was determined by Rhodamine-123 (lipophilic cationic dye). The HCT-116 cells were loaded in 6-numbered well plate and treated with AuNPs, then incubated upto 24 h. Later, HCT-116 cells were incubated for 30 min with Rh-123 dye. The mitochondrial membrane potential was qualitatively measured by using the Floid cell imaging station. Accordingly, HCT-116 cells were trypsinaized, the intensity (fluorescence) was calculated at 485/530 nm by using spectrofluorometer.
Measurement of ROS generation
ROS production (Intracellular) was determined by DCFH-DH (2,7-diacetyl dichlorofluorescein), a non-fluorescent probe which can enter into the intracellular matrix, then it is oxidized by ROS generation to form fluorescent dichlorofluorescein (DCF). HC-116 cells were loaded in 6-numbered well plate treated with AuNPs at various concentrations upto 24 h and then kept in a CO2 incubator upto 24 h. Later, HCT-116 cells were incubated with DCFH-DA (100 μl) for 10 min at 37 °C. The fluorescence excitation and emission at 485 ± 10 and 530 ± 12.5 nm, respectively, was quantified by using a multimode reader. Finally, all the images were recorded using fluorescent microscope (Nikon, Eclipse TS100, Tokyo, Japan).
Fluorescence microscopic analysis of cell death
AO/EtBr dual staining were used to identify the process of apoptosis by means of morphological examination. AO stain was uptaken by viable and non-viable cells which discharge green colour fluorescence. EtBr was taken up by nonviable cells alone, which emits red colour fluorescence with DNA intercalation. HCT-116 cells were loaded in 6 well plates and treated with AuNPs for 24 h. Later, dye mixture (20 µl) was added into the AuNPs treated cells and instantly it was observed by using fluorescent microscope. Untreated HCT-116 cells were treated as control. Then, the results were expressed as mean ± SEM for three independent examinations.
Cell-cycle analysis
The HCT-116 cells were plated in 6-numbered well plate and then treated with AuNPs. The detached and adherent cells were collected by the trypsinization method, and fixed in ethanol (ice-cold; 70%) for nearly 1 h. After that, the remaining pellets were washed twofold times by phosphate-buffered saline (PBS) and incubated for 30 min at 37 °C in 1 ml of PBS (propidium iodide 50 μg; Triton X-100 90.1%; EDTA 1 mM and RNaseA 0.5 mg). Subsequent to staining, the samples were examined with the help of FACS Aria III.
Assays for caspase activity by ELISA
The HCT-116 cells were loaded in a 96 well plate and these cells were treated with 15 and 20 μg/ml of AuNPs for 24 h. Later, HCT-116 cells were isolated and homogenated according to the caspase 3, 8 and 9 colorimetric assay kit made use of the apoptotic process. A fluorescence excitation wavelength and emission wavelength were measured by spectrofluorometer at 485 ± 20 nm and 528 ± 20 nm, respectively.
RT-PCR analysis
The whole RNA was isolated from control and AuNPs-treated HCT-116 cells using Trizol reagent. The diluted RNA sample was quantified spectrophotometrically via determining the absorbance at 260 nm. The decontaminate RNA sample was reverse transcribed by way of reverse transcriptase enzyme into a single-strand cDNA. The cDNA was amplified with particular primers of caspase-3, Bax, Bid, Bcl-2 and caspase-9. The GAPDH was used as an internal control.
Results and discussion
The common method AuNPs synthesis with related sizes to report in this study was attained by Turkevich and Frensby via gold hydrochlorate reduction by sodium triscitrate solution at 100 °C [Citation22]. Here, we mention the uses of easier and greener method of AuNPs synthesis. By mixing the T. kirilowii extract with chloroauric acid (aqueous), the solution colour was transmuted from yellow to ruby-red, which indicates AuNPs formation. The AuNPs formation was apparent from the change of pale yellow colour solution to deep red in addition to the presence of typical plasmon peak in the 525–540 nm range with a peak maximum approximately 527–535 nm range in the UV-spectrum.
The established systematic mechanism for NPs synthesis via phyto-chemical driven reaction shows that T. kirilowii extract have multifarious reducing molecules including antioxidants, phenolic moieties, and enzymes, by which gold cations are reduced into AuNPs [Citation23]. The theoretical HAuCl4 reduction is evaluated by the phytoconstituents to zerovalent gold synthesis, consequently gold atoms agglomerised into nanosized particles and finally it provides spherical AuNPs by stabilization with the help of phytochemicals [Citation24]. The maximum peak is a typical characteristic feature of spherical-sized AuNPs. The reaction after 24 h to 30th day was evident from the plasmonic peak stability through the determined reaction kinetics by UV-Vis spectroscopy and confirmed the reaction completion ().
Figure 1. UV–Visible spectrum absorption pattern and SAED pattern of gold nanoparticles synthesised from T. kirilowii. (A) UV pattern of Au-T. kirilowii; (B) SAED pattern of Au-T. kirilowii.
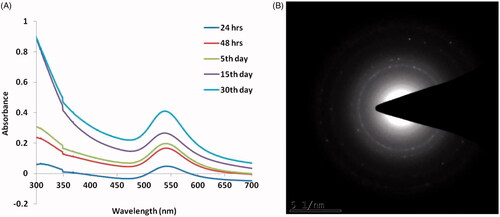
Spectrophotometrically AuNPs concentration was assessed by the Beer–Lambert law with an extinction coefficient (1.8 × 1010 M−1 cm−1) [Citation25] for a particle diameter of ∼50 nm. The longitudinal plasmon absorption band is a powerful function of the nanoparticle aspect ratio, the diversity was observed in the optical-based properties of synthesized AuNPs [Citation26]. Through adsorption and desorption, biomolecules specifically interacts with the nanoparticle face and its growth rates had affected kinetically, which in turn controls the nanoparticle’s shape [Citation27]. It has been reported that reducing agents mediated controlled reduction of metal salts generally in favour of producing spherical nanoparticles [Citation28].
The obtained images corroborate the homogeneous AuNPs distribution as the majority of the particle size ranges from 40 to 50 nm in diameter and was consistent by the TEM measurements. The nanoparticles crystal structure was evidenced by SAED pattern (). The gold nanoparticles were completely stable even after more than 36 days. The crystallinity nature of the biosyntheised AK-GNPs was assessed using XRD and the patterns were depicted in . AK-GNPs exhibited three distinct peaks at 111, 200 and 220, which are the characteristic peaks exhibited by the gold nanocrystals.
Figure 2. HR-Transmission electron microscopy analysis and X-ray diffraction pattern of gold nanoparticles synthesised from T. kirilowii. (A) HR-TEM analysis of Au-T. kirilowii; (B) XRD pattern of Au-T. kirilowii.
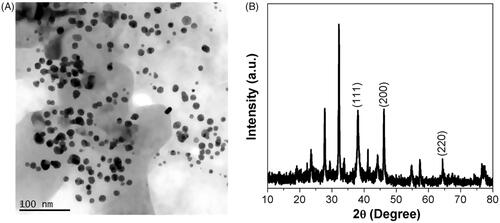
FTIR assessment illustrated numerous peaks in the spectrum of nanoparticles indicating that T. kirilowii extracts mediated nanoparticle synthesis (). Evaluation of the spectrum from AuNPs and T. kirilowii extracts revealed a high similarity, indicated the proficient adsorption of organic compounds on AuNPs. The FTIR spectra exhibited a number of sharp absorption peaks situated at 3329 cm−1, 2138 cm−1, 1636 cm−1, 955 cm−1 and 718 cm−1, respectively, responsible for N–H stretching of amines, CΞC stretching of alkyne groups, C–N stretching, bending vibration of C=C alkanes, plane vibrations (amino acids), O–H stretching vibration, amide II vibration, C–H stretching of aromatic compounds and carbonyl stretch vibration in ketones.
Figure 3. Fourier-transform infrared spectroscopy analysis of gold nanoparticles synthesised from T. kirilowii.
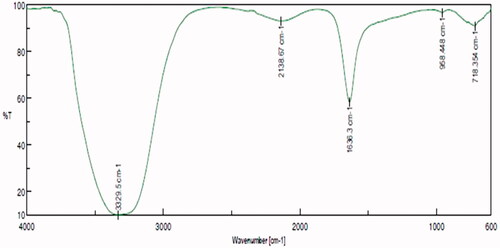
The anti-carcinogenic effect of phytoconstiuent-mediated AuNPs creates a center of attention on growing interest in AuNPs synthesis, which are being well thought-out as novel agents. In addition, AuNPs act as nanocarriers in the targeted system of drug delivery [Citation29]. In this report, we elucidated the therapeutic potential anti-carcinogenic effect of AuNPs on HCT-116 cancer cells and 3T3 cells. In order to find out the safe working concentration, HCT-116 cells were treated with 5, 10, 15, 20, and 25 μg/ml concentrations of AuNPs. These findings showed AuNPs considerably reduced the cell viability by 86.47%, 71.25%, 61.14%, 53.24%, 41.29% and 29.87% at 5, 10, 15, 20, and 25 μg/ml concentration, respectively; approximately 50% cell viability inhibition was documented with AuNPs at 15.5 μg/ml (). Particularly, HCT-116 cells were very much sensitive to T. kirilowii extract mediated AuNPs based on a concentration-dependent manner. However, no significant toxicity was observed () in normal 3T3 cells which clearly shows the safety nature to the normal cells.
Figure 4. Cytotoxic evaluation of AuNPs at various concentrations against HCT-116 cell lines and 3T3 fibroblast normal cells. All the values are expressed as mean ± SD, #p < .05 when compared with control group.
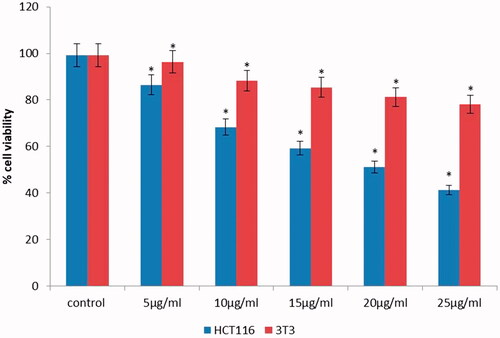
Previous reports stated that different types of nanoparticles induce ROS generations [Citation30,Citation31], a serious incident which causes mainly apoptosis and aging. Examples of ROS are superoxide ions, hydroxyl radicals, H2O2 and singlet oxygen. ROS overproduction eventually simulates oxidative stress, leads to lipid peroxidation (LPO), apoptosis and duplex DNA breaks [Citation32]. Intracellular ROS production was evaluated by DCFH-DA staining (). In our study, AuNPs nanoparticles exhibit drastically enhanced levels of ROS generation in HCT-116 cells in a concentration-dependent manner. Enhanced intracellular RO generations can alter the mitochondrial membrane permeability, resultant in collapse of mitochondrial membrane potential [Citation33]. Further, we have analyzed AuNP-induced loss of ΔΨm in HCT-116 cells with Rhodamine-123 (). Increasing concentration of AuNPs induced loss of of ΔΨm in concentration-dependent manner. After the treatment, fluorochrome uptaken by the control cells were observed, whereas in AuNPs-treated cells, the Rhodamine-123 uptaken was notably lesser than the untreated HCT-116 cells. Our findings signified that damage occurs in the mitochondrial membrane potential after the AuNP treatment. Also, this study investigated that AuNPs induce apoptosis by damaging the potential mitochondrial membrane.
Figure 6. Mitochondrial membrane potential of HCT-116 cells exposure of AuNPs determined by fluorescent dye Rhodamine-123. (A) Morphological images of control and Au-T. kirilowii-treated HCT-116 cells. (B) Mitochondrial membrane potential of HCT-116 cells
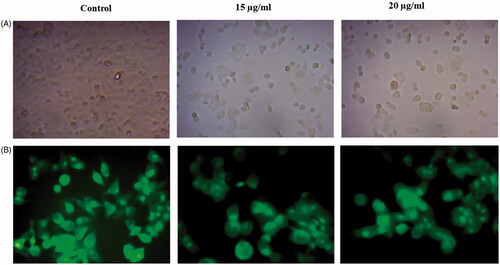
Similarly, Cui et al. [Citation34] reported that aggregation of AuNPs in the mitochondria of cancer cells which induces cytotoxicity. Recently, Mkandawire et al. demonstrated that AuNPs caused limited outer mitochondrial membrane rupture and lastly, it induces apoptosis in breast cancer cells [Citation35]. These reports demonstrated that mitochondrial membrane damage stimulated by AuNPs play a central role in cell death/apoptosis. Our results were concordant with the above findings, AuNPs with definite potential capability to cause apoptosis via the loss of mitochondrial membrane potential.
Moreover, we carried out AO/EB staining method to understand the therapeutic effect of AuNPs on morphological alerations in HCT-116 cells after the treatment. Using a fluorescence microscope in acridine orange ethidium bromide assay, AO/EB staining method illustrated that untreated cells appeared homogeneously green whereas early hours apoptotic cell was yellowish green or yellow colour. Acridine orange was used in conjunction with ethidium bromide to distinguish between viable, necrotic and apoptotic cells [Citation36]. shows control HCT-116 cells as well as necrotic and apoptotic cells after the staining of Acridine orange/ethidium bromide. The HCT-116 control cells did not receive AuNPs treatment represented in green color (live cells) and AuNPs treated cells showed late apoptotic stage in orange colour (dead cells) with chromatin clumping and condensation. The cell-cycle analysis is being beneficial, paricularlly for the sensitization of malignant cells to specific cancer treatment method [Citation37,Citation38]. AuNPs efficiently arrest the cell-cycle progress specifically at the phase of G0/G1and it consequently leads to apoptosis [Citation39]. In the current study also, AuNPs (15 and 20 μg/ml) treated cells showed apoptoic cells which was achieved by the arrest in the phase of G1 (). Our results also suggested that AuNPs competently arrest the cell-cycle progress by the induction of apoptosis in HCT-116 cells.
In this study, we determined the apoptotic gene expression levels (Bax, Bcl-2, caspase-3 and caspase-9) in AuNPs exposed HCT-116 cells at 15 and 20 µg/mL concentration for 24 h. The obtained data exhibited that the apoptotic gene expression levels were considerably altered in HCT-116 cells due to the exposure of AuNPs. The gene level of bid gene was found to be 0.7 and 0.9-fold higher in AuNPs exposed cells as compared to untreated cells. Furthermore, we elucidated the therapeutic effect of AuNPs on the apoptotic expression levels of caspase-3,8 and caspase-9 (). The caspase-3 expression was found to be 1.36 fold and caspase-9 was 1.21 fold increased in AuNPs treated cells in comparison with control cells. We also found higher pro-apoptotic gene expression level (bax, 0.73 fold) and decreased antiapoptotic gene expression level bcl-2, 0.41 fold) in AuNPs exposed cells than those of untreated cells (). Hence, our results suggest that AuNPs significantly provoke apoptosis in HCT-116 cells. The morphological feature of fragmentation and condensation (nuclear material) specify the stimulation of apoptosis, ulimately leading to cell death. The inhibitions of cell growth happen owing to cell permeability disruption, reactive oxygen species production, activation of caspase cascade-mediated apoptosis and cell-cycle arrest [Citation40,Citation41].
Conclusion
We have demonstrated an environment friendly and high cost-efficient protocols for synthesizing T. kirilowii-mediated AuNPs. Our finding data revealed that AuNPs induce noteworthy cytotoxicity in HCT-116 cells based on a dose-dependent manner. Furthermore, gene expression analysis exhibited that proteins expression levels involved in the apoptosis were altered by AuNPs exposure. Generally, our data suggest that AuNPs might induce apoptosis in HCT-116 cells through bid, bax/bcl-2 and casapase pathways. Our in vitro data illustrated the apoptosis induction obviously by AuNPs which demands further research investigation to find out if in vivo exposure cost could endure for AuNPs application. The application of AuNPs may lead to an invention of possible antiapoptotic agent in cancer therapy.
Disclosure statement
No potential conflict of interest was reported by the authors.
Additional information
Funding
References
- American Cancer Society. Cancer facts & figures 2019. Atlanta (GA): American Cancer Society; 2019.
- Camilleri M, Malhi H, Acosta A. Gastrointestinal complications of obesity. Gastroenterology. 2017;152:1656–1670.
- Alsheridah N, Diet AS. Obesity and colorectal carcinoma risk: results from a national cancer registry-based middle-eastern study. BMC Cancer. 2018;18:1227.
- Alam MN, Almoyad M, Huq F. Polyphenols in colorectal cancer: current state of knowledge including clinical trials and molecular mechanism of action. Biomed Res Int. 2018;2018:Article ID 4154185.
- Augestad KM, Merok MA, Ignatovic D. Tailored treatment of colorectal cancer: surgical, molecular, and genetic considerations. Clin Med Insights Oncol. 2017;11:117955491769076.
- Mishra J, Drummond J, Quazi SH, et al. Prospective of colon cancer treatments and scope for combinatorial approach to enhanced cancer cell apoptosis. Crit Rev Oncol Hematol. 2013;86:232–250.
- Wen H, Jung H, Li X. Drug delivery approaches in addressing clinical pharmacology-related issues: opportunities and challenges. AAPS J. 2015;17:1327–1340.
- Xin Y, Yin M, Zhao L, et al. Recent progress on nanoparticle-based drug delivery systems for cancer therapy. Cancer Biol Med. 2017;14:228–241.
- Din FU, Aman W, Ullah I, et al. Effective use of nanocarriers as drug delivery systems for the treatment of selected tumors. Int J Nanomedicine. 2017;12:7291–7309.
- Senapati S, Mahanta AK, Kumar S, et al. Controlled drug delivery vehicles for cancer treatment and their performance. Signal Transduct Target Ther 2018;3:7.
- Golombek SK, May JN, Theek B, et al. Tumor targeting via EPR: strategies to enhance patient responses. Adv Drug Deliv Rev. 2018;130:17–38.
- Chitgupi U, Qin Y, Lovell JF. Targeted nanomaterials for phototherapy. Nanotheranostics. 2017;1:38–58.
- Makarov VV, Love AJ, Sinitsyna OV, et al. "Green" nanotechnologies: synthesis of metal nanoparticles using plants. Acta Naturae. 2014;6:35–44.
- Dahoumane SA, Jeffryes C, Mechouet M, et al. Biosynthesis of inorganic nanoparticles: a fresh look at the control of shape, size and composition. Bioengineering (Basel). 2017;4:14.
- Singh P, Pandit S, Mokkapati V, et al. Gold nanoparticles in diagnostics and therapeutics for human cancer. IJMS. 2018;19:1979.
- Dreaden EC, Austin LA, Mackey MA, et al. Size matters: gold nanoparticles in targeted cancer drug delivery. Ther Deliv. 2012;3:457–478.
- Yeh YC, Creran B, Rotello VM. Gold nanoparticles: preparation, properties, and applications in bionanotechnology. Nanoscale 2012;4:1871–1880.
- Selim ME, Hendi AA. Gold nanoparticles induce apoptosis in MCF-7 human breast cancer cells. Asian Pac J Cancer Prev. 2012;13:1617–1620.
- Grijalva M, Vallejo-López MJ, Salazar L, et al. Cytotoxic and antiproliferative effects of nanomaterials on cancer cell lines: a review. In Unraveling the safety profile of nanoscale particles and materials-from biomedical to environmental applications. IntechOpen; 2017. UK.
- Bahadur B, Reddy KJ, Rao MLN. Medicinal plants: an overview. In: Reddy KJ, Bir Bahadur, Bhadraiah B, Rao MLN, editors. Advances in Medicinal Plants. Chennai: University Press; 2007. p. 1–50
- Bensky D, Clavey S, Stöger E, et al. Chinese herbal medicine materia medica. Seattle: Eastland Press. 2004.
- Aljabali AAA, Akkam Y, Al Zoubi MS, et al. Synthesis of gold nanoparticles using leaf extract of ziziphus zizyphus and their antimicrobial activity. Nanomaterials (Basel). 2018;8:174.
- Marslin G, Siram K, Maqbool Q, et al. Secondary metabolites in the green synthesis of metallic nanoparticles. Materials (Basel). 2018;11:940.
- Nath S, Ghosh SK, Panigrahi S, PT, et al. Assisted wet chemical route to synthesize gold nanoparticles. Indian J Chem. 2004; 43:1147–1151.
- Zuber A, Purdey M, Schartner E, et al. Detection of gold nanoparticles with different sizes using absorption and fluorescence based method. Sens Actuators B. 2016; 227:117–127.
- Kajani AA, Bordbar AK, Esfahani SH, et al. Gold nanoparticles as potent anticancer agent: green synthesis, characterization, and in vitro study. RSC Adv. 2016;6:63973–63983.
- Jo MR, Yu J, Kim HJ, et al. Titanium dioxide nanoparticle-biomolecule interactions influence oral absorption. Nanomaterials (Basel). 2016;6:225.
- Mohamad NA, Arham NA, Jai J, et al. Plant extract as reducing agent in synthesis of metallic nanoparticles: a review. Adv Mater Res. 2013;832:350–355.
- Patil MP, Jin X, Simeon NC, et al. Anticancer activity of Sasa borealis leaf extract-mediated gold nanoparticles. Artif Cells Nanomed Biotechnol. 2018;46:82–88.
- Martínez-Torres AC, Zarate-Triviño DG, Lorenzo-Anota HY, et al. Chitosan gold nanoparticles induce cell death in HeLa and MCF-7 cells through reactive oxygen species production. Int J Nanomedicine. 2018;13:3235–3250.
- Sun H, Liu Y, Bai X, et al. Induction of oxidative stress and sensitization of cancer cells to paclitaxel by gold nanoparticles with different charge densities and hydrophobicities. J Mater Chem B. 2018;6:1633–1639.
- Phaniendra A, Jestadi DB, Periyasamy L. Free radicals: properties, sources, targets, and their implication in various diseases. Ind J Clin Biochem. 2015;30:11–26.
- Marchi S, Giorgi C, Suski JM, et al. Mitochondria-ROS crosstalk in the control of cell death and aging. J Signal Transduct. 2012;2012:329635.
- Cui W, Li J, Zhang Y, et al. Effects of aggregation and the surface properties of gold nanoparticles on cytotoxicity and cell growth. Nanomedicine. 2012;8:46–53.
- Mkandawire MM, Lakatos M, Springer A, et al. Induction of apoptosis in human cancer cells by targeting mitochondria with gold nanoparticles. Nanoscale. 2015;7:10634–10640.
- Baharara J, Ramezani T, Divsalar A, et al. Induction of apoptosis by green synthesized gold nanoparticles through activation of caspase-3 and 9 in human cervical cancer cells. Avicenna J Med Biotechnol. 2016;8:75–83.
- Ramalingam V, Revathidevi S, Shanmuganayagam TS, et al. Gold nanoparticle induces mitochondria-mediated apoptosis and cell cycle arrest in nonsmall cell lung cancer cells. Gold Bull. 2017;50:177–189.
- Xu W, Luo T, Li P, et al. RGD-conjugated gold nanorods induce radiosensitization in melanoma cancer cells by downregulating α(v)β3 expression. Int J Nanomedicine. 2012;7:915–924.
- Choudhury D, Xavier PL, Chaudhari K, et al. Unprecedented inhibition of tubulin polymerization directed by gold nanoparticles inducing cell cycle arrest and apoptosis. Nanoscale. 2013;5:4476–4489.
- Jayaraj M, Renganathan A, Sathishkumar G, et al. Biogenic metal nanoformulations induce Bax/Bcl2 and caspase mediated mitochondrial dysfunction in human breast cancer cells (MCF 7). RSC Adv. 2015;5:2159–2166.
- Parida UK, Biswal SK, Bindhani BK. Green synthesis and characterization of gold nanoparticles: study of its biological mechanism in human SUDHL-4 cell line. Adv Biol Chem. 2014;04:360–375.