Abstract
Exploring the spatial relationship of various neuron pools in the spinal cord is crucial and difficult due to its complexity. The single-labelling tracing and sectioning were employed in previous studies exploring the distribution of spinal motor neuron pools, which could only delineate one single motor neuron pool in one specimen and could not achieve intact-tissue observation. Here, with combination of neuroanatomy tracing techniques and the optical clearing technique, we developed a multiple retrograde tracing method compatible with 3DISCO clearing. Fluoro-Gold, Fluoro-Ruby, Cholera Toxin Subunit B, Alexa Fluor 488 and 647 Conjugate were injected intramuscularly in hindlimbs of C57BL/6 adults. After labelling, the harvested spinal cords were optically cleared by 3DISCO method and imaged using confocal microscope. There were positive signals of all four tracers and four motor neurons pools targeting injected muscles were labelled. Three-dimension model of four motor neuron pools was successfully reconstructed based on tomography images showing the spatial relationship of different neuron pools. In conclusion, using this method, we first delineated the spatial relationship of four different motor neuron pools targeting four skeletal muscles in one spinal cord at the same time, which provide a holistic view of motor neuron pools in the spinal cord.
Introduction
The distribution of motor neurons in the spinal cord has significantly described through the previous works [Citation1–5]. Together, they have established that motor neurons in the ventral horn of the spinal cord are arranged into longitudinal columns. Recently, retrograde tracers, such as the horseradish peroxidase-family, the fluorescent inorganic compounds, and cholera toxin subunit B (CTb) family, have been instrumental in delineating the connectivity between the specific skeletal muscle and the innervating motor neuron pools in various mammalian species [Citation6–23]. These studies further characterized the organization of motor neuron columns throughout the spinal cord. However, the single-labelling tracing was employed in all these studies, which could only delineate one single motor neuron pool in one specimen at the same time. Labelled neurons could only be observed via sectioning, which always caused transformation and information missing due to the mechanical cutting or section holding. Exploring the three-dimension (3D) spatial relationship of different neuron pools in one intact specimen was difficult to achieve limited by temporal techniques of neuroanatomy tracing.
More recently, it provided us a completely new sight of tissue observation benefitted from the great development of tissue optical clearing methods, which allow us to investigate the complex cells with large projections deep in an intact tissue [Citation24–34]. Optical clearing by organic solvents, which reduces the light scattering inside tissues by matching the refractory indexes of different tissue layers to the solvent, makes it possible to perform shrinkage-mediated imaging under the laser confocal microscope and obtain high-resolution scanning pictures from deep in the intact spinal cord [Citation24].
Here, with combination of neuroanatomy tracing techniques and the optical clearing technique, a multiple retrograde tracing method compatible with 3DISCO clearing was developed and we provide a new tool to explore the spatial relationship of different motor neuron pools targeting specific muscles in mouse spinal cords from a holistic view.
Materials and methods
Animal care
All animal treatments and procedures of this study were performed in strict accordance with recommendations in the Institutional Animal Care Guidelines and approved ethically by the Administration Committee of Experimental Animals of Peking University People’s Hospital (Permit Number: 2015-16). To assure repeatability, three adult female C57BL/6 strain mice between 20 and 25 g were used. Animals were maintained on a 12/12 h light-dark cycle with ad libitum access to food and water. All efforts were made to minimize suffering.
Tracer preparation
Four retrograde tracers were chosen for labelling of the motor neurons in spinal cord. FG (Fluorochrome, Denver, CO, USA) was dissolved at 5% in distilled water and saved at 4 °C. Fluoro-Ruby (FR, Molecular Probes, Eugene, USA) and CTb (Recombinant), Alexa Fluor™ 488 and 647 Conjugate (CTb-488, CTb-647, Molecular Probes, Eugene, USA) were dissolved at 10% respectively in normal saline and 1× phosphate buffered saline (PBS) and saved at –20 °C. All these tracers were kept on the ice during preparation and injection.
Surgical procedure
Mice were anaesthetized by intramuscular injection of ketamine-xylazine (100 mg/kg ketamine; 10 mg/kg xylazine) and maintained under anaesthesia with isoflurane/oxygen. Prepare the surgical site according to the aseptic technique by trimming hair and disinfecting the surgical site with alternating scrubs of betadine and 75% alcohol (minimum of three cycles). Be sure to use sterile drapes to cover surgical areas. Make sure to adhere to sterile techniques throughout the procedures. Anterolateral incision was made on the left crus to reveal the muscles including gastrocnemius, tibialis anterior, lateral muscles and soleus (). Fascia was minimally disrupted over the muscles of interest and no blood vessels were disrupted.
Tracing procedure
The gastrocnemius, tibialis anterior, lateral muscles and soleus were respectively injected with 4 μL of FR, 2 μL of FG, 2 μL of CTb-647 and 1 μL of CTb-488 depending on the size of muscles using a 32-gauge needle connected to a 5 μL-Hamilton syringe under microscopic guidance. Tracers were injected slowly until the muscles were completely Coloured ensuring that the entire muscle was filled with dyes (). In order to prevent the leakage of tracers, following cares should be taken during the labelling: (A) wipe the fascia with aseptic cotton buds and make it a little bit dry and become more cohesive; (B) inject slowly at a speed of about 1 μL/min and stay the needle in the muscle for 1 min before removal; (C) after removal of the needle, press the injection site with cotton bud slightly for 1 min; (D) swab each muscle after injection with cotton tip to decrease non-specific spread, and then close the skin.
Spinal cord harvest
After the intramuscular injections, the mice were kept for 7 days to allow for optimal retrograde transport of neuronal tracers. Then, mice were deeply anaesthetized and perfused with 37 °C saline followed by 4 °C 4% paraformaldehyde (PFA; Sigma-Aldrich, St. Louis, MO, USA dissolved by 1× PBS). After perfusion, intact spinal cord was removed between T12 and S2 region to ensure the entire motor neuron pool was covered. Spinal nerve roots were remained so as to recognize the specific segments of spinal cord (). Then, the spinal cord was fixed with the post-fixation solution (4% PFA) over night. The sample was immobilized with microsutures on a piece of flat folded aluminium foil during the fixation and optical clearing procedure in order to prevent the deformation.
Optical clearing
The optical clearing procedure was performed as the reported 3DISCO method [Citation25]. The following reagents were prepared prior to clearing: 50%, 70%, 80% tetrahydrofuran (THF) in distilled water, 100% THF, 100% dichloromethane (DCM) and 100% dibenzyl ether (DBE; Sigma-Aldrich). Transfer the spinal cord from the post-fixation solution directly into the 20 mL brown glass vial, and immediately add the first clearing solution (50% THF). Each vial was tightened and placed on a shaker at 30 rpm for 45 min. The spinal cord was exposed to increasing concentrations of THF (70%, 80% and 100%) for 45 min at each step. The 100% THF immersion was repeated twice with fresh 100% THF. Then, samples were immersed and rotated in 100% DCM for 20 min followed by 100% DBE for 30 min until the spinal cord became transparent. To assess any potential decrease in tissue size due to the clearing procedure, the length, width and thickness of the spinal cords were measured before and immediately after clearing on the coordinate paper.
Imaging
The spinal cord was mounted in 100% DBE with two pieces of cover glass. The confocal microscope (Zeiss LSM 780) was set at a bit depth of 8, averaging of 2, laser intensity of 4–15, pinhole of 1.0 airy units and gain of 650. Imaging was performed at 5× magnification for the holistic view of the spinal cord and nerve roots followed by 10× magnification for positive signal zone. Best-signal mode of Zeiss LSM 780 was chosen to scan, and the filters was automatically optimized. The details of excitation and filter setup were shown in . Adjustments were made to the gain in order to obtain a brighter image and maximum intensity projection (MIP) was performed to display the distribution of the signals. Three-dimension (3D) reconstruction was performed on the basis of scanning images of 10× magnification to build the stereoscopic distribution of the labelled neurons. Software Image J and Imaris 7.6.0 were used respectively for the MIP and 3D reconstruction.
Table 1. Properties of the retrograde tracers and setups of lasers and filters of Zeiss LSM 780 confocal microscope.
Results
All spinal cords were rendered translucent after the 3DISCO optical clearing procedure (). The clearing process reduced the volume of mouse spinal cords by approximately 50%. In order to obtain the optimal signal-to-noise ratio (SNR) of scanning images, multiple values of laser power were applied. Only 4% of 633 nm lasers was needed for scanning of signals traced by CTb-647 at 10× magnification, while 15% of 405 nm lasers was applied for FG signal imaging. Ten percent lasers were needed for both FR and CTb-488 signal imaging. Pseudo-colours were applied in scanning images (FG: blue, FR: red, CTb-488: green, CTb-647: magenta) to distinguish signals emitting from the four tracers.
Figure 3. Spinal cord before and after optical clearing procedure. (A) Before clearing. (B) After clearing.
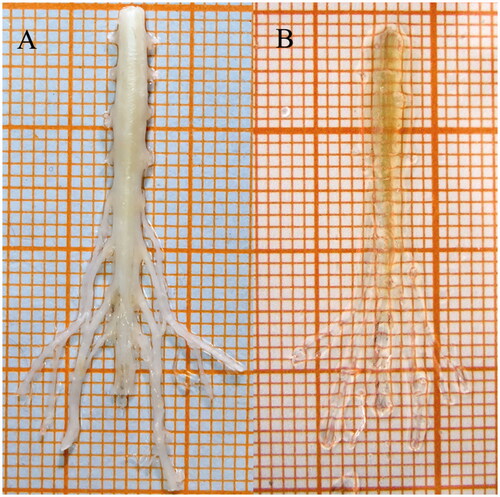
There were positive signals of all four tracers in three spinal cords. Motor neurons innervating the injected muscles were labelled as the classical stellate neural morphology. Labelled neurons in the ventral horn of the spinal cord that innervate the injected muscles locate in the spinal segment between L3 and L4 nerve root ( and ). The number of labelled neurons targeting the gastrocnemius, tibialis anterior, lateral muscles and soleus were respectively 67 ± 4.9, 64 ± 3.61, 113 ± 6.66 and 37 ± 3.6. There were several co-labelled neurons innervating the gastrocnemius, tibialis anterior, lateral muscles, but none of neurons targeting the tibialis anterior were co-labelled with others.
Figure 4. MIP images of single track. (A) Tibialis anterior motor neurons labelled by FG. (B) Soleus motor neurons labelled by CTb-488. (C) Gastrocnemius motor neurons labelled by CTb-488. (D) Lateral muscle motor neurons labelled by CTb-647.
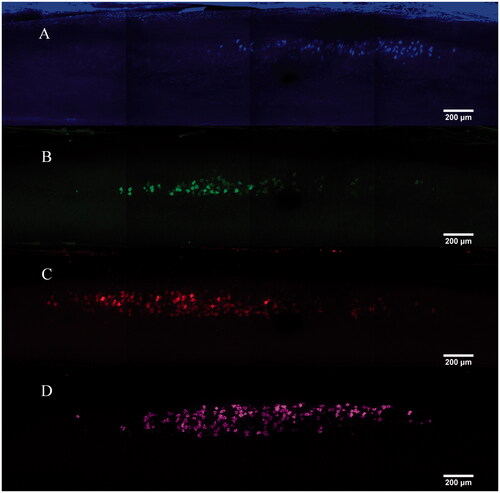
Figure 5. MIP images of multi-tracks in a holistic view [motor neurons innervating tibialis anterior (blue), gastrocnemius (red), soleus (green), lateral muscle (magenta)]. White arrows: dendrites or axons around the soma.
![Figure 5. MIP images of multi-tracks in a holistic view [motor neurons innervating tibialis anterior (blue), gastrocnemius (red), soleus (green), lateral muscle (magenta)]. White arrows: dendrites or axons around the soma.](/cms/asset/15a36e65-0753-431e-b498-17917771890f/ianb_a_1687493_f0005_c.jpg)
Tracers were diffused well in the neurons and brighten the cytoplasm leaving the cell nucleus as plaques. Neurons labelled by CTb-647 had the highest signal intensity, while FG-labelled neurons had the lowest signal intensity ( and ). There were relatively brighter background signals in scanning images except the imaging of CTb-647 tracing. Cell bodies of motor neurons were distinctly labelled by all four tracers, while dendrites or axons around the soma were only visible in neurons traced by FR, CTb-488 and CTb-647 ().
Table 2. Imaging results of motor neuron pools labelled by the four tracers.
The 3D reconstruction was successfully built on the basis of tomography images at 10× magnification ( and Supporting Information S1 video). The four motor neuron pools are arranged into relative individual columns in longitudinal spinal cord with partial overlap between different pools. The distribution vantage of four neuron pools in different planes was seen in .
Discussion
In our study, multiple tracing of motor neurons innervating different muscles was performed via intramuscular injection of four tracers (FG, FR, CTb-488 and CTb-647) and positive signals were successfully obtained under the laser confocal microscope. However, all the tracers had favourable labelling except FG, which displayed the lowest signal intensity and SNR. The most possible reason to explain this is the imaging filter setup. With two excitation peaks at approx. 330 and 390 nm, and two emission peaks at approx. 450 and 600 nm, the automatic filter setup of 410–499 nm might not cover the majority of the emission spectrum of FG. Therefore, higher laser power was required for scanning the FG signals, however, the intensity of signals still remained lower. Images of CTb-647 tracing have the best SNR, while images of FG, CTb-488 and FR tracing have more apparent background noise. This might be due to the emission spectrums of FG, CTb-488 and FR approximate to the auto fluorescence of the C57BL/6 spinal cord.
There existed co-labelling between neurons innervating the gastrocnemius, lateral muscles and soleus. Due to less fascia separating the adjacent muscle especially the gastrocnemius and soleus, slight dye permeation was occurred through the neighbouring muscles. However, few neurons were co-labelled relative to the number of single labelling neurons. In spite of this, great cares should be taken to prevent leakage while injecting and fascia should be minimally disrupted over the muscles of interest.
Distribution of motor neurons in mice innervating muscles of limbs has been delineated in several previous studies [Citation20–22]. They all used monoretrograde labelling via intramuscular injection with FG and observed by cryosections. However, details of dye injection differed between these studies. In order to perform injections more accurately, Morri and coworkers successfully delineated the superficial lines of motor end plates (MEP) on muscles of limbs via acetylcholinesterase histochemistry on mouse carcases. Then, tracer injections were performed targeting the full length of the MEP regions in the mouse limbs. However, better consistency of labelled motor neuron pools in spinal cords was found in mice that smaller muscles were injected, while relatively apparent differences were found in those injecting the larger muscles [Citation20]. According to our recent research, MEPs in limb muscles are distributed as laminar microstructure in a 3D model especially multiple lamination in the multi-head muscles, but not all the rims of MEP laminas reach the surface of muscles forming the MEP line [Citation35]. Injection targeting the full MEP regions may not cover the majority of the MEPs. Therefore, we believe that injections in multiple sites of the targeting muscle may improve the efficiency of labelling.
A number of methods for the whole tissue imaging have recently emerged. Various Methods for optical tissue clearing were developed including organic solvent-based clearing method (UDISCO, Murray’s clear, 3DISCO), water-based clearing methods (Scale, SeeDB, FRUIT, CUBIC) and hydrogel-based clearing methods (CLARITY, PACT) [Citation34,Citation36]. UDISCO, 3DISCO and Murray’s clear provide maximal transparency but fluorescence of fluorescent proteins (FPs) cannot be preserved after Murray’s clear. 3DISCO has great compatibility with fluorescent dyes and FPs and achieves fast and efficient clearing within several hours for a spinal cord. However, it results in obvious tissue shrinkage due to dehydration. The recent developed clearing technique UDISCO has better FP preservation over months [Citation34] but there exists worse SNR and longer clearing time compared with 3DISCO according to our pilot experiments. The water-based clearing methods and hydrogel-based clearing methods, like provided Scale, SeeDB, CUBIC and PACT, provide better preservation of sample size, but lower transparency which could not meet the need of the whole spinal cord imaging. Besides that, it always takes a couple of days to perform the entire clearing procedure [Citation36].
Optical clearing methods allow us to investigate the complex cells with large projections deep in tissues without missing of structural information [Citation25]. Combination of neural tracing and optical clearing methods brings a new way for visualization of neural circuits and distribution of various neurons. Zygelyte et al. [Citation37] described a retro-DISCO method optically clearing the mouse spinal cord without loss of fluorescent signals to image retrograde labelled motor neurons. This method combined 3DISCO and retrograde tracing with immersion of nerve trunk stumps, which allowed more rapid and precise quantification of labelled motor neurons compared to sectioning. In addition, Launay et al. [Citation38] successfully delineated a 3D map of corneal sensory neurons within an intact adult mouse trigeminal ganglion with the combination of the 3DISCO clearing method with light-sheet microscopy, retrograde tracer, and automatic counting. Although there are many advantages of tissue optical clearing method, cares must be taken when explained the results. Varying degrees of tissue shrink existed in organic solvent-based clearing methods due to the dehydration procedure, which caused the size decrease when homogeneous shrink happened, but the size decrease and transformation if asymmetrical shrink existed [Citation34]. Furthermore, it is not compatible with organic solvent-based clearing method for all the tracers, therefore it must be evaluated the compatibility while combining the optical clearing method and neural tracing. Additionally, due to the short fluorescence preservation period of 3DISCO method, we suggested imaging the samples immediately after clearing.
Exploring the anatomical distribution of various categories of neurons in the central nervous system are the basis of researches in neural circuit and neurologic disease. Our method can be used in studying the positional relation of different motor neuron pools corresponding to the specific muscle and exploring the microcircuit in spinal cord. Imaging the intact spinal cord with optical clearing method is easier to perform and provides more precise quantification of neurons. Furthermore, multiple retrograde tracing from muscles to spinal cord allows the distribution analysis of different motor neuron pools.
Although there were positive signals in all four tracing tracks, FG signals could not be completely scanned applying with the other three tracers simultaneously due to the optimized filtration setup of the laser confocal microscope. Therefore, we recommended use the FR, CTb-488 and CTb-647 for retrograde multiple neural tracing of motor neurons via the intramuscular injection. Additionally, the XY plane of the 3D model remained the high resolution, while lower image quality was obtained on the XZ and YZ plane due to the scanning interval of 5 μm in the Z stack. However, the spatial relationship of the different motoneuron pools was still distinguished in the 3D model.
Conclusion
With combination of the neuroanatomy tracing technique and optical clearing technique, a multiple retrograde tracing method compatible with 3DISCO clearing was developed and there provides a new tool to explore the spatial relationship of different motor neuron pools targeting specific muscles in mouse spinal cords from a holistic view.
S1_video.mp4
Download ()Acknowledgements
Thanks are due to the assistance of the research team of Pro. Dan Zhu (Tingting Yu, Jianyi Xu, Yusha Li, Yisong Qi) in Huazhong University of Science and Technology.
Disclosure statement
No potential conflict of interest was reported by the authors.
Additional information
Funding
References
- Sherrington CS. Notes on the arrangement of some motor fibres in the lumbo-sacral plexus. J Physiol. 1892;13(6):621–772.17.
- Romanes GJ. The development and significance of the cell columns in the ventral horn of the cervical and upper thoracic spinal cord of the rabbit. J Anat. 1941;76(Pt 1):112–130.5.
- Romanes GJ. Motor localization and the effects of nerve injury on the ventral horn cells of the spinal cord. J Anat. 1946;80:117–131.
- Romanes GJ. The motor cell columns of the lumbo-sacral spinal cord of the cat. J Comp Neurol. 1951;94(2):313–363.
- Rexed B. A cytoarchitectonic atlas of the spinal cord in the cat. J Comp Neurol. 1954;100(2):297–379.
- Kristensson K, Olsson Y. Retrograde axonal transport of protein. Brain Res. 1971;29(2):363–365.
- Kristensson K, Olsson Y. Uptake and retrograde axonal transport of peroxidase in hypoglossal neurons. Electron microscopical localization in the neuronal perikaryon. Acta Neuropathol. 1971;19(1):1–9.
- McHanwell S, Biscoe TJ. The localization of motoneurons supplying the hindlimb muscles of the mouse. Philos Trans R Soc Lond B Biol Sci. 1981;293(1069):477–508.
- Jenny AB, Inukai J. Principles of motor organization of the monkey cervical spinal cord. J Neurosci. 1983;3(3):567–575.
- Nicolopoulos-Stournaras S, Iles JF. Motor neuron columns in the lumbar spinal cord of the rat. J Comp Neurol. 1983;217(1):75–85.
- Brichta AM, Callister RJ, Peterson EH. Quantitative analysis of cervical musculature in rats: histochemical composition and motor pool organization. I. Muscles of the spinal accessory complex. J Comp Neurol. 1987;255(3):351–368.
- Callister RJ, Brichta AM, Peterson EH. Quantitative analysis of cervical musculature in rats: histochemical composition and motor pool organization. II. Deep dorsal muscles. J Comp Neurol. 1987;255(3):369–385.
- Horner M, Kummel H. Topographical representation of shoulder motor nuclei in the cat spinal cord as revealed by retrograde fluorochrome tracers. J Comp Neurol. 1993;335(3):309–319.
- Novikova L, Novikov L, Kellerth JO. Persistent neuronal labeling by retrograde fluorescent tracers: a comparison between Fast Blue, Fluoro-Gold and various dextran conjugates. J Neurosci Methods. 1997;74(1):9–15.
- Vanderhorst VG, Holstege G. Organization of lumbosacral motoneuronal cell groups innervating hindlimb, pelvic floor, and axial muscles in the cat. J Comp Neurol. 1997;382(1):46–76.
- McKenna JE, Prusky GT, Whishaw IQ. Cervical motoneuron topography reflects the proximodistal organization of muscles and movements of the rat forelimb: a retrograde carbocyanine dye analysis. J Comp Neurol. 2000;419(3):286–296.
- Choi D, Li D, Raisman G. Fluorescent retrograde neuronal tracers that label the rat facial nucleus: a comparison of Fast Blue, Fluoro-ruby, Fluoro-emerald, Fluoro-Gold and DiI. J Neurosci Methods. 2002;117(2):167–172.
- Tosolini AP, Morris R. Spatial characterization of the motor neuron columns supplying the rat forelimb. Neuroscience. 2012;200:19–30.
- Bacskai T, Fu Y, Sengul G, et al. Musculotopic organization of the motor neurons supplying forelimb and shoulder girdle muscles in the mouse. Brain Struct Funct. 2013;218(1):221–238.
- Tosolini AP, Mohan R, Morris R. Targeting the full length of the motor end plate regions in the mouse forelimb increases the uptake of fluoro-gold into corresponding spinal cord motor neurons. Front Neurol. 2013;4:58.
- Bacskai T, Rusznak Z, Paxinos G, et al. Musculotopic organization of the motor neurons supplying the mouse hindlimb muscles: a quantitative study using Fluoro-Gold retrograde tracing. Brain Struct Funct. 2014;219(1):303–321.
- Mohan R, Tosolini AP, Morris R. Targeting the motor end plates in the mouse hindlimb gives access to a greater number of spinal cord motor neurons: an approach to maximize retrograde transport. Neuroscience. 2014;274:318–330.
- Mohan R, Tosolini AP, Morris R. Segmental distribution of the motor neuron columns that supply the rat hindlimb: a muscle/motor neuron tract-tracing analysis targeting the motor end plates. Neuroscience. 2015;307:98–108.
- Erturk A, Mauch CP, Hellal F, et al. Three-dimensional imaging of the unsectioned adult spinal cord to assess axon regeneration and glial responses after injury. Nat Med. 2011;18(1):166–171.
- Erturk A, Becker K, Jahrling N, et al. Three-dimensional imaging of solvent-cleared organs using 3DISCO. Nat Protoc. 2012;7(11):1983–1995.
- Chung K, Wallace J, Kim SY, et al. Structural and molecular interrogation of intact biological systems. Nature. 2013;497(7449):332–337.
- Ke MT, Fujimoto S, Imai T. SeeDB: a simple and morphology-preserving optical clearing agent for neuronal circuit reconstruction. Nat Neurosci. 2013;16(8):1154–1161.
- Kuwajima T, Sitko AA, Bhansali P, et al. ClearT: a detergent- and solvent-free clearing method for neuronal and non-neuronal tissue. Development (Cambridge, England). 2013;140(6):1364–1368.
- Susaki EA, Tainaka K, Perrin D, et al. Whole-brain imaging with single-cell resolution using chemical cocktails and computational analysis. Cell. 2014;157(3):726–739.
- Tainaka K, Kubota SI, Suyama TQ, et al. Whole-body imaging with single-cell resolution by tissue decolorization. Cell. 2014;159(4):911–924.
- Yang B, Treweek JB, Kulkarni RP, et al. Single-cell phenotyping within transparent intact tissue through whole-body clearing. Cell. 2014;158(4):945–958.
- Hama H, Hioki H, Namiki K, et al. ScaleS: an optical clearing palette for biological imaging. Nat Neurosci. 2015;18(10):1518–1529.
- Susaki EA, Tainaka K, Perrin D, et al. Advanced CUBIC protocols for whole-brain and whole-body clearing and imaging. Nat Protoc. 2015;10(11):1709–1727.
- Pan C, Cai R, Quacquarelli FP, et al. Shrinkage-mediated imaging of entire organs and organisms using uDISCO. Nat Methods. 2016;13(10):859–867.
- Yin X, Yu T, Chen B, et al. Spatial distribution of motor endplates and its adaptive change in skeletal muscle. Theranostics. 2019;9(3):734–746.
- Orlich M, Kiefer F. A qualitative comparison of ten tissue clearing techniques. Histol Histopathol. 2017;33(2):181–199.
- Zygelyte E, Bernard ME, Tomlinson JE, et al. RetroDISCO: clearing technique to improve quantification of retrograde labeled motor neurons of intact mouse spinal cords. J Neurosci Methods. 2016;271:34–42.
- Launay PS, Godefroy D, Khabou H, et al. Combined 3DISCO clearing method, retrograde tracer and ultramicroscopy to map corneal neurons in a whole adult mouse trigeminal ganglion. Exp Eye Res. 2015;139:136–143.