ABSTRACT
The use of suitable DNA barcodes and the generation of databases with reference sequences have been considered a promissory approach for the identification of Chlorophyta and Cyanophyta microalgae. In this study, we carried out a molecular characterization and identification of strains isolated from freshwater systems in Ecuador using a dual barcode method. The target sequences for Chlorophyta were 18S rDNA and rbcL genes, and 16S rDNA and 16S–23S rDNA intergenic spacer (ITS) for Cyanophyta. We reported these DNA barcodes for 20 different Molecular Operational Taxonomic Units (MOTUs) for Chlorophyta and 10 for Cyanophyta. Our results show that the 18S V4 hypervariable region (300 bp) is sufficient for differentiating between isolates, but rbcL is a determinant for genus identification in Scenedesmaceae and Chlorellaceae strains. In Cyanophyta, both barcodes enabled the genus-level assignment of 9 out of 10 MOTUs. These results highlight the necessity of a second barcode additional to small ribosomal subunit sequences to improve molecular identification. Furthermore, the present study significantly contributes to the body of Ecuadorian barcode sequences of microalgae that are currently documented, making them available for future comparative diversity studies.
Introduction
Chlorophyta microalgae, also known as green microalgae, are eukaryotic microorganisms that comprise a diverse lineage, with approximately 8,000 described species [Citation1]. Cyanophyta microalgae or Cyanobacteria are prokaryotic microorganisms also known as blue-green algae. However, many Cyanobacteria are not blue-green, but purple, brownish, or just green [Citation2,Citation3]. Chlorophytes and Cyanobacteria are important primary producers in marine, freshwater, and terrestrial ecosystems [Citation4]. Both microorganisms have been used as biological indicators for monitoring and protecting aquatic environments [Citation5], and as platforms for the production of a wide variety of bioproducts ranging from biofuels – such as hydrogen, alcohols, and isoprenoids – to high-value bioactive and recombinant proteins [Citation6] and dietary supplementation [Citation7]. They are also regarded as attractive laboratory models for the genetic studies of fundamental processes [Citation8,Citation9]. There are even two genera of Chlorophyta, Chlorella and Prototheca, which are able to cause disease in humans and other mammals through active invasion and spread within host tissues [Citation10].
Traditionally, Chlorophyta microalgae have been classified based on morphological features observed under the microscope, including shape, chloroplast and pyrenoid position, and the presence of flagella [Citation11–13]. Regarding Cyanobacteria, the morphological traits considered for taxonomic classifications are cell size, trichome width, polarity, shape and arrangement, pigmentation, and the presence of characteristics such as gas vacuoles and a sheath [Citation14]. The subjective interpretation of these features and the fact that some strains may lose some important features – for example, gas vesicles or the form of the colony during long-term laboratory cultivation [Citation15,Citation16] – may lead to misidentification. Furthermore, this approach requires high-resolution equipment and specialist taxonomists [Citation17], particularly when dealing with taxa displaying phenotypic plasticity, species where there are few or difficult to observe structural characteristics, and cryptic species [Citation18]. To overcome these difficulties, both the use of DNA barcodes and the generation of databases with reference sequences have been considered a promissory approach for microalgae identification [Citation17,Citation19–22]
The use of DNA barcodes for biological identification, introduced by Hebert et al. [Citation23,Citation24], is based on short, easily amplified regions of DNA that vary greatly among species, but have a low intra-specific variability [Citation25,Citation26]. The most common quantitative approach in DNA taxonomy is based on a predetermined, fixed genetic divergence threshold of sequences to identify operational taxonomic units as equivalent to species, named Molecular Operational Taxonomic Units (MOTUs) [Citation27]. Currently, there is no consensus to define a universal threshold for microalgae taxa [Citation28,Citation29]. Moreover, the DNA barcoding of microalgae is limited by the lack of universally applied markers among research groups, and it seems more effective to employ multiple genes for barcoding. Many authors have highlighted that a single barcode marker does not meet the needs of the biodiversity community given the complexity and heterogeneity of microalgae [Citation30,Citation31]. Promising targets for potential Chlorophyta DNA barcodes have included chloroplast (rbcL, tufA, and Cp23S), mitochondrial (COI), and nuclear genes (18S rDNA, nuITS1, and nuITS2) [Citation18,Citation31–33].
The availability of sequence databases, for instance the International Nucleotide Sequence Database Collaboration and Barcode of Life Data System (BOLD), is crucial for allowing DNA barcoding to be useful for comparisons of species diversity and identification. A large number of the small subunit rDNA sequences (18S and 16S rDNA) have been deposited in the public DDBJ/EMBL/GenBank databases. Therefore, these regions are good candidates for identifying species and revealing species diversity in ecological systems [Citation34,Citation35,Citation36]. The V4 18S rDNA region is considered suitable for molecular analysis due to its greater size and variability of inversions, insertions, and deletions [Citation36,Citation37]. Another promising candidate is the rbcL marker; despite the lack of a universal primer pair for successful rbcL amplification, it is reportedly sufficiently variable to be able to distinguish among most chlorophyte species [Citation18,Citation38,Citation39]. In fact, there are currently c. 9,000 rbcL sequences from chlorophyte species deposited in the DDBJ/EMBL/GenBank database. To assess the genetic diversity of Cyanobacteria, the 16S rDNA sequence has traditionally been used [Citation40,Citation41]. The 16S-23S rDNA Internal Transcribed Spacer (ITS) is also broadly employed to genetically characterize Cyanobacterial strains [Citation42–46]. Furthermore, the secondary structure of the RNA transcribed from this sequence is taken into account for the elaboration of phylogenetic trees [Citation47].
Ecuadorian continental waters are believed to comprise a biodiversity reservoir of enormous global significance [Citation48]. Nevertheless, studies focused on microalgae from Ecuadorian freshwater systems are scarce and taxonomy studies only take into account its morphological features [Citation11,Citation49].
This study investigated the molecular variability and phylogeny of several Chlorophyta and Cyanophyta microalgae isolated from freshwaters in Ecuador. Dual DNA barcoding was used for molecular identification using 18S rDNA and plastid rbcL markers for chlorophytes, and 16S rDNA and ITS for Cyanobacteria. The present study is the most significant attempt at the DNA barcoding of freshwater microalgae in Ecuador.
Material and methods
Sampling
Access to freshwater ecosystems was conferred by the Ministerio de Ambiente del Ecuador (MAE) via the permission codes MAE-DNB.CM-2018-0093 and MAE-DNB.CM-2015-0011. Triplicate 1 L water samples were collected from different freshwater systems – including artificial ponds, water storage reservoirs, lagoons, lakes, and rivers – located in the Ecuadorian Andean and Amazon regions (Table S1). The sampling locations span the Andean region from north (Imbabura) to south (Azuay) as well as some water bodies from the Amazon region in order to isolate a good representation of microalgae species present in the country. The samples were kept in coolers on ice and taken to the laboratory for further analysis.
Enrichment and isolation
The collected freshwater samples were filtered through glass GF5 grade microfiber filters (pore size 0.7 μm, Chmlab). The harvested biomass underwent an enrichment step through suspension in liquid Bold’s Basal (BB) and Blue-Green 11 Media (BG11) [Citation50]. This was maintained for at least two weeks at an average temperature of 20 ± 2 °C, a relative humidity of 50%, and light intensity of 21.384 ± 1.584 µmol∙m-2∙s-1, with light/dark cycles of 16/8 h inside a germination chamber (Wisd, Korea). Enriched samples were streaked in solidified BB and BG-11 media, with 1% agar, and incubated under the same conditions described for enrichment for at least 4 weeks, until individualized green colonies appeared on agar plates. This process was repeated until unialgal cultures were obtained. The achievement of unialgal cultures was verified by light microscopy using an Olympus BX-40 with 100X phase contrast oil-immersion objective. Microscopic morphologic analysis was performed based on previous studies [Citation11,Citation51,Citation52]. Further identification was accomplished by comparison with the original species descriptions that are available at the AlgaeBase [Citation53].
Unialgal cultures were grown in an appropriate liquid medium for 4 weeks. One milliliter of the culture in a concentration ranging from 2 to 9 × 107 cells/mL was transferred to 2 mL of cryovials and mixed with a DMSO and glycerol solution to a final concentration of 5% (v/v) and 10% (v/v) respectively. After incubation at room temperature for 30 min, cryovials were placed in a freezer at −20 °C for two days and then transferred to a Dewar flask containing liquid nitrogen. Microalgae isolates were kept in a cryopreserved state in an ultra-freezer at −80 °C.
Molecular identification
Isolated microalgae were submitted to DNA extraction with PureLink® Genomic DNA Mini Kit (InvitrogenTM), following the instructions provided by the manufacturer with slight modifications in the lysis process. Briefly, microalgae colonies, from unialgal cultures grown for at least 4 weeks, were resuspended in 180 µL of Genomic Digestion Buffer and heated up to 90 °C for 12 to 17 minutes. After cooling, samples were processed as detailed by the manufacturer.
In addition, 16S rDNA and the 16S–23S rDNA intergenic spacer (ITS) were partially amplified for cyanobacterial identification, whereas fragments of 18S rDNA and rbcL were amplified for green algae identification. Primer combinations and their respective annealing temperatures are shown in . Amplification was performed in reactions of 25 µL containing 2X GoTaq® Green Master Mix (Promega), 0.25 µM of each primer, and 25 to 35 ng of extracted DNA. The reaction consisted of an initial denaturation step at 95 °C for 2 min, followed by 40 cycles as follows: 45 s at 95 °C, 30 s annealing at the proper temperature for each primer pair and 1 min at 72 °C, and a final extension step at 72 °C for 5 min.
Table 1. Primer combinations, the annealing temperature (Ta) and expected amplicon size (bp) for each set of primers used in this study
Direct sequencing of the PCR amplicon was performed in both directions at the Sequencing service of Universidad de Las Américas (Quito, Ecuador). For ITS amplification with multiple PCR products, the band around 300 base pairs (bp) was extracted from the gel using the Wizard® SV Gel and PCR Clean-Up System (Promega) prior to the sequencing. Sequences were edited with MEGA X software [Citation54] and compared against the GenBank database at the National Center for Biotechnology Information (NCBI) using the Basic Local Alignment Search Tool (BLAST). Reference sequences were downloaded from the GenBank database and aligned with barcoding sequences from these work strains using the Muscle Algorithm [Citation55]. Tree topologies and branch lengths were computed separately for the two markers with the maximum-likelihood method (ML). This was performed with 500 bootstrap replicates using the Kimura 2-parameter model with discrete gamma distribution, based on the corrected Akaike Information Criterion (AICc) in the ML model selection feature of MEGAX. Gloeobacter violaceus (NR074282.1) was used as an outgroup taxon for the Cyanobacteria tree due to its independent taxonomical position at the base of all Cyanobacteria [Citation56].
Results
Genetic diversity analysis among isolates
A total of 82 unialgal strains of Chlorophyta and 10 Cyanobacteria were isolated from freshwater bodies from the Andean and Amazon region of Ecuador (Table S1).
The 18S rDNA V4 hypervariable region was successfully amplified from DNA samples extracted from all the chlorophyte strains isolated using the universal primers described by Zimmerman et al. [Citation37]. This primer set has been extensively used for metabarcoding studies in epilithic diatoms communities [Citation57–59], so our results indicate that it could also be a useful marker for the Chlorophyta microalgae group. The fragments amplified were about 300 base pairs (bp) in length except for the CFU-22-04 strain with 700 bp. The sequences with a similarity of 100% were considered MOTUs. A total of 20 MOTUs were obtained, named from M-01 to M-20. M-01 (17%), M-02 (24%), and M-07 (27%) were the most abundant, followed by M-04 and M-08 (6%–7%). All of them were isolated from different freshwater systems of the Andean region but, in addition, 2 and 4 were also found in the Limoncocha lagoon, located in the Amazon region (). A second primer set was used to amplify the VI–V3 region of the 18S rDNA gene. All the strains tested were successfully amplified with the expected PCR product size of approximately 600 bp. One representative from each group was amplified with the oligos proposed by Hadi et al. [Citation38] for the rbcL gene. Sixty-two percent of the DNA samples extracted were successfully amplified with primer set Fw_rbcL_357/Rv_rbcL-1089 and 14% with the Fw_rbcL_192/Rv_rbcL_657 primer combination. The amplification failed for 5 strains.
Table 2. Molecular Operational Unit Taxonomic (MOTU) identified for Chlorophyta, indicating the number, location of isolates (Table S1), and GenBank accession numbers (AN)
The 16S rDNA and ITS amplification for Cyanobacteria strains was 100% successful. The sequence analysis of 16S rDNA amplicons revealed 10 molecular variants. shows the location and accession number for each Cyanobacteria MOTU. The ITS amplification retrieved fragments with different sizes among the samples, ranging from 100 bp to 300 bp. Three of the samples amplified multiple PCR products. In these cases, the band close to 300 bp was sequenced.
Table 3. MOTU identified for Cyanobacteria, indicating the number, location of isolates (Table S1), and GenBank accession numbers (AN)
Similarity search based on 18S and rbcL markers for Chlorophyta
In order to perform the molecular identification, the 18S rDNA sequences obtained were submitted to searches against the GenBank database at the NCBI. The closest matches for the 18S rDNA V4 region had a similarity of up to 99–100%, as compared with the sequences available in the Genbank database, excepting M-05 (96%) and M-015 (98%) (). Most of the MOTUs obtained belong to the Scenedesmaceae and Chlorellaceae families. Six out of the twenty MOTUs matched with only one species, four matched with one genus, and the rest matched with more than one species with 100% similarity belonging to the same family or genus. Sequencing of the V1-V3 region was performed in order to improve molecular identification, and it also retrieved more than one identical sequence from different species for several MOTUs (Table S2). The M-02 MOTU for this region was the only strain that matched 100% with a sequence from an Ecuadorian microalga (MF677854.1 Coelastrella sp.). The 18S V4 sequence of M-05 was about 400 bp higher than the others; however, the V1-V3 region had the same length as the other strains. The BLAST results showed three sequence entries with the same length as M-005, that is for Scenedesmaceae sp. Tow 9/21 P-14 w (AY197639.1) was the closest match with a 100% query cover and 96% similarity. Nonetheless, it has a 100% identity with the sequence entries of Desmodesmus sp., Acutodesmus deserticola, and Scenedesmus armatus, with a 40% query cover, which is consistent with the results obtained for the 18S rDNA V1-V3 region. The same similarity searches were carried out for the rbcL sequences that were successfully amplified. The similarity with the Genbank sequences was lower than those obtained for the 18S rDNA barcodes. The closest matched identities ranged from 92% to 100% with a smaller number of hits per species ().
Table 4. Molecular identification of the Chlorophyta strains used in this study, including the percentage of identity, accession number, and the name of the identified species in the GenBank database, based on the 18S V4 and rbcL marker sequence. * indicates query cover below 100%
The results presented in , based on the 18S and rbcL sequences, suggest that species-level identification was achieved for eleven out of the twenty-one MOTUs (M-01, 03, 04, 07, 08, 10, 11, 13, 14, 16, 17, and 19), and four (M-05, 12, and 20) were determined at the genus level. In the case of M-09, the identification was not possible given that both barcodes matched two different species with a 100% identity. However, microscope visualization showed spherical green cells, which were more similar to Parachlorella kessleri than the ellipsoidal cells of Chloroidium saccharophilum. The same scenario occurred for M-18, and so a change of name was proposed from O. amblystomatis (with the accession number KY091671) to Chlorococcum amblystomatis [Citation60]. These authors recently reported about a novel Chlorococcum strain isolated from a pond in Portugal that was 100% identical to M-18 and KY091671. The 18S sequence-based identification assigned M-06 to Micractinium inermun, and matched 100% to the M. inermun strain NIES-2171 (AB731604) described by Hoshina & Fujiwara [Citation61]. Under a light microscope, we observed solitary spherical cells without mucilaginous coverings, consistent with the description of M. inermun, but we were not able to distinguish the chloroplast features. Nevertheless, the closest match for the rbcL sequence was Chlorella sp. The nucleotide search for M. inermun rbcL sequences retrieved zero entries. The rbcL amplification for M-02 and M-15 was not successful, and the 18S barcode did not allow for species-level identification. The morphological analysis using light microscopy showed that the cells of M-02 are grouped and broadly ellipsoidal to spherical, the chloroplasts are hollow, spherical and entirely cover the cell sphere, and they contain a pyrenoid similar to the Coelastrella species. No characteristic features were observed in M-15 that permitted differentiation between the genera.
A Phylogenetic analysis was carried out to resolve the genus-level identification for the Scenedesmaceae strains. Genetic distances (p-distance) among Scenedesmaceae family sequences for 18SV4 ranged from 0.011 to 0.102. Nevertheless, the variability found among Scenedesmaceae sequences for 18SV1-V3 fragments was lower. For the V1-V3 region, the p-distance between M-01 and 02 was 0.006, while for the V4 region was 0.018. The phylogenetic tree using reference 18S rDNA sequences from the GenBank database () clearly demonstrates that sequences from these strains group together with their closest matches from the GenBank database () in well-supported clades. M-01 and M-02 are clustered with Tetradesmus and Coelastrella sequences, respectively, and separated from the other Scenedesmaceae strains (M-03, M-04, M-05 and M-20) that are grouped in a separated clade. The genetic distances for rbcL sequences ranged from 0.064 to 0.107 among M-01, 03, 04, and 20, with the lowest distance when comparing M-03 and M-04 with M-20. The rbcL region obtained for M-05 matched only in terms of 63 bp with the sequence amplified from the other strains; therefore, it was not included in the rbcL comparison. Hadi et al. [Citation38] reported that Desmodesmus rbcL interspecific distances ranged from 0.015 to 0.086. Thus, according to the BLAST results and genetic distances, M-04, M-05, and M-20 belong to the Desmodesmus genus and they are probably different species. This idea is supported by the phylogenetic tree constructed using rbcL and 18S joined sequences, which separated M-04 and M-20 into two different clusters (). also shows M-18 and Oophila amblystomatis (KY091671) clustered with the Chlorococcum genus in a monophyletic clade, as reported by Correia et al. [Citation60], thus confirming the identification of M-18 as Chlorococcum amblystomatis.
Figure 1. Phylogram constructed using 18SV4 rDNA sequences from Ecuadorian strains and sequences in the GenBank database belonging to the Chlorophyceae class. The phylogram was constructed using the maximum-likelihood method with a Kimura 2-parameter model using a discrete Gamma distribution (+G). Numerical values at the nodes of the branches indicate bootstrap values above 50%
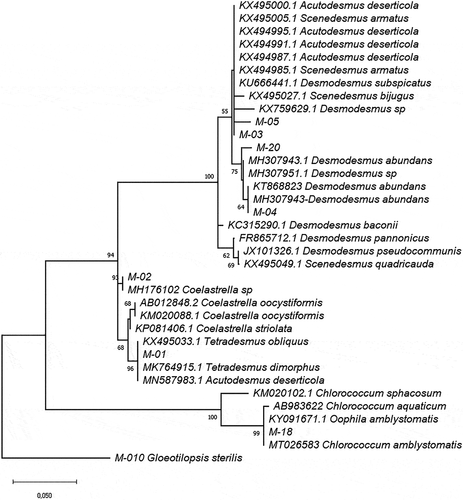
Figure 2. Phylogram constructed using 18SV4 rDNA joined to rbcL sequences of the Ecuadorian MOTUs. The phylogram was constructed using the maximum-likelihood method with a Kimura 2-parameter model. Numerical values at the nodes of the branches indicate bootstrap values above 50%
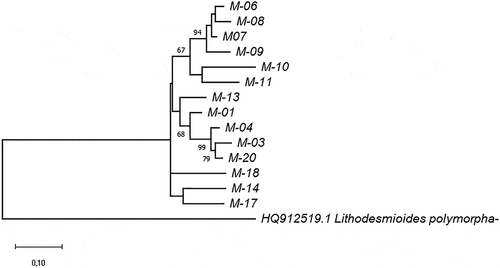
The phylogenetic tree of Trebouxiophyceae class strains using reference 18S rDNA sequences showed two clades corresponding to the Chlorellaceae and Trebouxiaceae families. The genetic distances between Chlorellaceae strains ranged from 0.007 to 0.014 for 18SV4 fragments, and from 0.002 to 0.019 for the 18SV1-V3 region, with M-09 being the most different. Chlorellaceae Ecuadorian sequences were separated into three clusters: M-06 with Micractinium, M-07 and M-08 with Chlorella spp., and M-09 with Parachlorella and Chloroidium spp. (). The analysis carried out with both barcodes joined together () also indicated that M-08 is closer to M-07 than M-09. The phylogenetic tree did not resolve the genus identification for the M-15 sequence.
Figure 3. Phylogram constructed using 18SV4 rDNA sequences from Ecuadorian strains and similar sequences in the GenBank database belonging to the Trebouxiophyceae class. The phylogram was constructed using the maximum-likelihood method with the Kimura 2-parameter model using a discrete Gamma distribution (+G). Numerical values at the nodes of the branches indicate bootstrap values above 50%
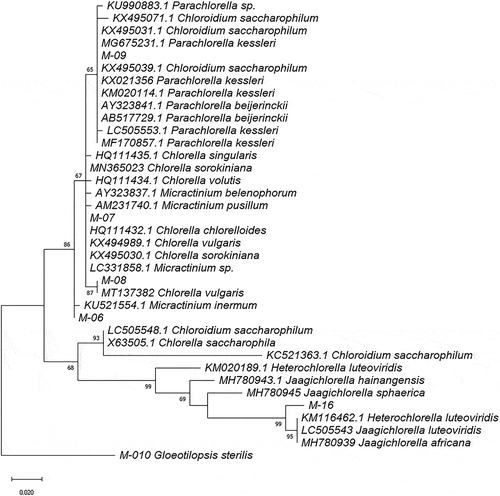
Similarity search based on 16S and ITS markers for Cyanobacteria
In order to perform the molecular identification, the 16S sequences obtained were submitted to searches against the GenBank database. The closest matches for the 16S rDNA region ranged from 98% to 100% similarity with sequences available in the GenBank database ().
Table 5. Molecular identification of the Cyanobacteria strains used in this study, including the percentage of identity, accession number, and the name of the identified species in the GenBank database, based on the 16S and ITS marker sequence. * indicates query cover below 100%
Two MOTUs (C-02 and 06) matched with only one species, four (C-04, 07, 08, and 09) matched with one genus, and two (C-01 and 10) matched with more than one species with a 100% similarity. The GenBank sequences similar to C-03 and C-05 correspond to undescribed Cyanobacteria species isolated from the Rhine basin (KP143908.1) and Antarctica mats (HQ 827,392.1), respectively. The same similarity searches were carried out for ITS sequences. The similarity with GenBank sequences was lower than those obtained for the 16S barcode. The closest match identities ranged from 92% to 100% with a smaller number of hits per species (). There were no closest matches corresponding to more than one species. Moreover, the query cover was lower than 100% for C-04 (76%), C-05 (50%), and C-08 (50%).
The species-level identification based on both barcoding sequences () was achieved for three MOTUs (C-02, 06, and 10), and six could be determined at the genus level (C-01, 03, 04, 07, 08, and 09). In the case of C-05, the sequence similarity for ITS was too low to even assign the genera of the strain. Light microscopic observation did not allow a species- or genus-level assignment for C-05. However, morphological features observed for C-03 matched those of the Pseudanabaena genus.
The phylogenetic analysis for Cyanobacteria strains was accomplished based only on 16S rDNA sequences due to the lack of homogeneity in ITS fragment length. The genetic distances between sequences ranged from 0.03 to 0.25. The most similar sequences were C-07, C-09, and C-03, and the greatest distance was noted between C-05 and C-09. The phylogenetic tree constructed using reference 16S rDNA sequences from the GenBank database () reflects the BLAST results for Cyanobacteria strain identification (). The unidentified strain C-11 grouped into the Pseudanabaena cluster. However, the C-05 strain did not cluster into any of the genera assigned to the other strains or with the closest genera matched with the ITS sequence.
Figure 4. Phylogram constructed using 16S rDNA sequences from Ecuadorian cyanobacteria and similar sequences at the GenBank database. The phylogram was constructed using the maximum-likelihood method with Kimura 2 distance. Numerical values at the nodes of the branches indicate bootstrap values above 50%
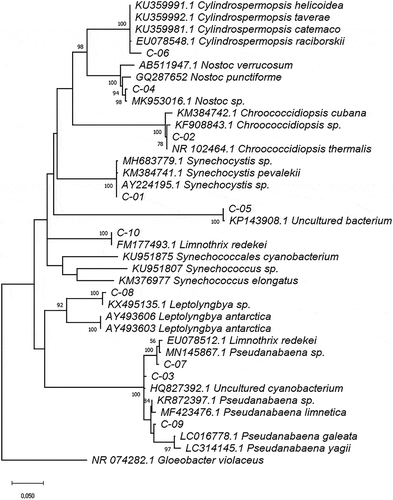
Discussion
This study focused on the dual barcoding characterization of microalgae isolated from Ecuadorian freshwater environments. However, with the data presented, we did not intend to perform an ecological biodiversity assessment of Chlorophyta and Cyanobacteria taxa present in Ecuador. The molecular analysis was conducted in order to obtain the DNA barcodes from strains present in the country and compare them to sequences present in the Genbank database. Moreover, it can help to differentiate between isolates that are morphologically indistinguishable. This will facilitate the identification and selection of strains for further studies.
Several DNA barcode systems have been proposed as a potential solution for tackling the great diversity of Chlorophyta and Cyanobacteria, yet there is no consensus about which marker should be used [Citation18,Citation38,Citation62]. In this work, we followed the nested strategy proposed by the Consortium for the Barcode of Life (CBOL) protist working group using the variable V4 region of 18S rDNA as the universal eukaryotic pre-barcode and second group-specific barcode [Citation31]. Our results show that the 18S V4 region is equally effective as the V1 and V3 regions, which are commonly used for identifying Chlorophyta microalgae [Citation19,Citation63,Citation64]. In fact, our results show that this marker has a higher nucleotide diversity in a smaller region than the V1-V3 marker. Furthermore, the primer universality achieved for this barcode is higher than the rbcL marker, as described in previous studies [Citation18,Citation38]. The V4 region allowed us to differentiate between isolates of Scenedesmaceae and Chloraceae families that were alike under light microscopic visualization. It is noteworthy that the BLAST results, when compared with the GenBank database, allowed for the identification of 38% of 18SV4 sequences from the strains isolated at the species level. This rate increased to 52% when the rbcL BLAST results were taken into account. Several studies highlight the necessity of using several barcodes to properly identify strains at the species level, especially in the Scenedesmaceae [Citation65–67] and Chloraellaceae families [Citation68]. The use of rbcL is not very common in Chlorophyta microalga phylogenetics, but it was useful for characterizing our collection, despite the rate of unsuccessful amplifications reported by others [Citation31,Citation38].
Simple morphology, phenotypic plasticity, and the presence of cryptic species in the Scenedesmaceae and Chlorellaceae families have contributed to taxonomic complications [Citation69] resulting in constant reassignments [Citation70]. Regarding the Scenedesmaceae family, the Desmodesmus and Acutodesmus genera were considered to be Scenedesmus subgenera by Hegewald [Citation71] until they were separated as genera of their own by An et al. [Citation72]. This could explain why strains with identical 18S rDNA gene sequences are assigned with different genera, as was the case for the closest matches M-01 and M-03. On the other hand, the same scenario occurs for the Chlorellaceae family. Most of the Chlorella-like species possess few morphological characteristics useful for species characterization, and many studies often report “little green balls” for Chlorella spp [Citation33]. For instance, the Parachlorella genus was reassigned from Chlorella kessleri (Fott and Nováková) [Citation68] and Chloroidium was reassigned as a new genus from Chlorella saccharophila and C. ellipsoidea by Huss et al. [Citation73]. Some authors reported a lack of variability in the 18S rDNA gene when discriminating between the genera of these families [Citation33], thus they suggest the use of a second marker for proper identification, such as rbcL and the ribosomal RNA ITS regions. The plastid-encoded rbcL gene has been widely used for phylogenetic inference in green algae [Citation18,Citation31,Citation38], but it has described as taxon-specific, which is consistent with the lack of amplification in some of our isolates. In spite of this, the rbcL barcode was a determinant in resolving the identification of the M-01, M-03, M-07, M-09, M-013, and M-019 sequences. The rbcL gene is considered to be evolving more rapidly than the 18S rDNA gene [Citation33]; this may be the reason for the lower percentage of identifications with the rbcL sequences deposited in the GenBank database and the strains in this work, as compared to the 18S V4 region. Heterochlorella and Jaagichlorella are very close taxa with a general Chlorella-like morphology, and they belong to the Trebouxiaceae family. Previous studies reported the necessity of an integrated approach using Small Subunit (SSU) and ITS rDNA sequences and including ecological distribution to resolve the taxonomical status in this group [Citation74]. This could explain the lack of resolution of 16S rDNA barcoding to identify the strain corresponding to MOTU M-15. Darienko et al. [Citation74] proposed the transfer of Heterochlorella luteoviridis to Jaagichlorella. Additionally, it seems that Heterochlorella (Jaagichlorella) luteoviridis and J. africana have different distribution patterns. Strains of J. luteoviridis were commonly isolated from aquatic systems while the others were collected from terrestrial habitats. According to this differentiation, our strains could be named Jaagichlorella luteoviridis; nevertheless, we would have to sequence the ITS region to confirm this assignment. Our results support the necessity of a second marker for DNA barcoding in microalgae, and highlight the lack of reference sequences linked to a precise morphological identification.
The strains we isolated are distributed worldwide. They are all found in freshwater systems from different geographic regions, and their sequences match previously published sequences. Scenedesmaceae and Chlorella strains have been found to be the most common aquatic algae isolated from the aquatic systems sampled in our study, as they are the most commonly reported genera of freshwater coccoid green algae with a ubiquitous distribution [Citation33,Citation39]. Some of these species, like Tetradesmus dimorphus, Coelastrella sp., Chlorella vulgaris and C. sorokiniana, have been found throughout the Andean region and present no variability in the barcode sequences. Hadi et al. [Citation38] performed a similar study focussed on the assessment of DNA barcodes for neotropical freshwater Chlorophytes. They analyzed a subset of green microalga strains isolated from Brazilian inland water bodies and most of them also belonged to Scenedesmaceae and Chloraceae species.
It has been demonstrated that the differences in genetic distances for the 16S rDNA barcode are useful in defining taxonomic units in Cyanobacteria [Citation75,Citation76]. Consistent with that, nine of our isolate sequences clustered with Leptolyngbya, Pseudanabaena, Nostoc, Cylindrospermopsis, Synechoscystis, Limnothrix, and Chroococidiopsis genera, based on the 16S rDNA barcode. However, the 16S sequence of two of these strains (C-01 and C-10) matched with more than one species with a 100% identity. This could be due to the lack of genetic interspecific differentiation reported between taxonomic units in prokaryotes [Citation76,Citation77]. Another explanation could be the misidentification of the strains based on morphological characteristics, as it has been reported that many Cyanobacteria strains in culture collections are wrongly classified [Citation78,Citation79]. The C-05 MOTU did not group with any other genera and the sequence only matched (99%) with an unidentified cyanobacterium isolated from aquatic systems (KP141908.1 in Germany; KJ766163.1 in Taiwan) and humid soil (KF358628.1 in China). The C-03 strain, that grouped with Pseudanabaena genera, matched 100% with an unidentified cyanobacterium isolated from mats present in maritime Antarctica. It is worth highlighting that the origin of the C-03 sample is the Chimborazo Volcano (6.300 masl), which has a permanent glacier and is the source of the stream from which the strain was isolated.
Nevertheless, an accurate taxonomy can hardly be achieved using only one barcode, since it is based on nucleotide substitutions of a single gene [Citation76]. Thus, we also sequenced the ITS region of 16S-23S rDNA, as it has been broadly used as a gene marker to examine cyanobacterial community structures [Citation80–82]. Nevertheless, we did not construct a phylogenetic tree based on this barcode because of the heterogeneity of the size of the amplicons, which makes it difficult to align the sequences. Despite this, the ITS barcode allowed us to disambiguate the C-01, C-03, and C-10 strains, and corroborate the 16S matches of C-02 at a species level and C-04, C-07, C-08, and C-09 at a genus level. The ITS region is an effective molecular marker for characterizing intra-species diversity [Citation80,Citation82]. The sequences of our isolates for this marker are more variable than the 16S rDNA BLAST results, and in some cases, the low percentage of query cover hampered the molecular identification of the strain.
Many of the strains isolated have been described to be of biotechnological interest to the food industry, biofuel production, and pharmaceutical and environmental activities. The Chlorella, Chlorococcum, Nostoc, and Synechococcus species are of great interest to the first two sectors due to high lipid accumulation and the production of fatty acids and polyunsaturated fatty acids (PUFAs) [Citation60,Citation83,Citation84]. It has also been shown that these species play a role in the biodegradation of pesticide pollutants and metal removal [Citation85]. Cyanobacteria species belonging to the Nostoc and Synechococcus genera have been used for the development of antiviral, antibacterial, and anti-inflammatory agents, and are of interest to pharmaceutical researchers [Citation83].
The few reports that have focused on the diversity of Chlorophyta and Cyanobacteria in Ecuador were conducted based on morphological identification [Citation11,Citation49]. In spite of the increasing application of molecular phylogeny in the floristic study of microalgae, there are scarcely any barcode sequences available for Ecuadorian strains. Scientists have only reported the ITS2 sequences for Stichococcus sp. and Diplosphaera sp. strains [Citation86], and 18S rDNA sequences for Chlorella sp., Coelastrella sp., Scenedesmus sp., and diatom strains [Citation87,Citation88]. Furthermore, there are sequences for ribosomal genes (16S and 23S) available in the GenBank database (popset MH090926–32 and MH101455–61) for seven cyanobacteria from geothermal springs in Ecuador (unpublished) and for the rbcL gene of Nostoc sp. from the lichen genus Peltigera [Citation89]. However, our study considerably increases the molecular characterization of the Chlorophyta and Cyanobacteria strains present in Ecuador, thereby contributing to knowledge regarding the biodiversity of these taxa. All barcode sequences in this work are being reported for the first time for Ecuadorian Clorophyta and Cyanobacteria strains, except for 18SV1V3 of M-02 MOTU (Coelastrella sp.), which were reported previously.
Disclosure of potential conflicts of interest
No potential conflict of interest was reported by the author(s).
Supplemental Material
Download Zip (29.3 KB)Acknowledgments
We are grateful to Byron Freire and Gabriel Iturralde for the technical support and resources from the Research Laboratories at UDLA.
Supplementary material
Supplemental data for this article can be accessed here.
Additional information
Funding
References
- Guiry MD. How many species of algae are there? J Phycol. 2012;48(5):1057–1063.
- Hollingsworth PM. Refining the DNA barcode for land plants. Proc Natl Acad Sci U S A. 2011;108(49):19451–19452.
- Hubert N, Hanner R, Holm E, et al. Identifying Canadian freshwater fishes through DNA barcodes. PLoS One. 2008;3(6):e2490.
- Torres MA, Barros MP, Campos SCG, et al. Biochemical biomarkers in algae and marine pollution: a review. Ecotoxicol Environ Saf. 2008;71(1):1–15.
- Omar WMW. Perspectives on the use of algae as biological indicators for monitoring and protecting aquatic environments, with special reference to Malaysian freshwater ecosystems. Trop Life Sci Res. 2010;21(2):51–67.
- Al-Haj L, Lui Y, Abed R, et al. Cyanobacteria as chassis for industrial biotechnology: progress and prospects. Life. 2016;6(4):42.
- Mišurcová L, Škrovánková S, Samek D, et al. Health benefits of Algal Polysaccharides in human nutrition. Adv Food Nutr Res. 2012; 66:75–145. Elsevier Inc.
- Khanna N, Lindblad P. Cyanobacterial hydrogenases and hydrogen metabolism revisited: recent progress and future prospects. Int J Mol Sci. 2015;16(12):10537–10561.
- Zehr JP. Nitrogen fixation by marine cyanobacteria. Trends Microbiol. 2011;19(4):162–173.
- Jagielski T, Bakuła Z, Gawor J, et al. The genus Prototheca (Trebouxiophyceae, Chlorophyta) revisited: implications from molecular taxonomic studies. Algal Res. 2019;43:101639.
- Guamán C, Nory B, Romero PG. Catálogo de microalgas y cianobacterias de agua dulce del Ecuador. Quito: Laboratorio de Biotecnología Energética; 2016.
- Nakayama T, Watanabe S, Mitsui K, et al. The phylogenetic relationship between the Chlamydomonadales and Chlorococcales inferred from 18SrDNA sequence data. Phycol Res. 1996;44(1):47–55.
- Škaloud P, Neustupa J, Radochová B, et al. Confocal microscopy of chloroplast morphology and ontogeny in three strains of Dictyochloropsis (Trebouxiophyceae, Chlorophyta). Phycologia. 2005;44(3):261–269.
- Komárek J, Kaštovský J, Mareš J, et al. Taxonomic classification of cyanoprokaryotes (cyanobacterial genera) 2014, using a polyphasic approach. Preslia. 2014;86:295–335.
- Gugger M, Lyra C, Henriksen P, et al. Phylogenetic comparison of the cyanobacterial genera Anabaena and Aphanizomenon. Int J Syst Evol Microbiol. 2002;52(Pt 5):1867–1880.
- Lehtimäki J, Lyra C, Suomalainen S, et al. Characterization of Nodularia strains, cyanobacteria from brackish waters, by genotypic and phenotypic methods. Int J Syst Evol Microbiol. 2000;50(3):1043–1053.
- Manoylov KM, Graham L. Taxonomic identification of algae (morphological and molecular): species concepts, methodologies, and their implications for ecological bioassessment. J Phycol. 2014;50(3):409–424. Graham L, editor.
- Hall JD, Fučíková K, Lo C, et al. An assessment of proposed DNA barcodes in freshwater green algae. Cryptogam Algol. 2010;31:529–555.
- Alemzadeh E, Haddad R, Ahmadi A. Phytoplanktons and DNA barcoding : characterization and molecular analysis of phytoplanktons on the Persian Gulf. Iran J Microbiol. 2014;6(4):296–302.
- Bock C, Krienitz L, Pröschold T. Taxonomic reassessment of the genus Chlorella (Trebouxiophyceae) using molecular signatures (barcodes), including description of seven new species. Fottea. 2011;11(2):293–312.
- Esling P, Lejzerowicz F, Pawlowski J. Accurate multiplexing and filtering for high-throughput amplicon-sequencing. Nucleic Acids Res. 2015;43(5):2513–2524.
- Pawlowski J, Holzmann M. A plea for DNA barcoding of foraminifera. J Foraminiferal Res. 2014;44(1):62–67.
- Hebert P, Cywinska A, Ball S, et al. Biological identifications through DNA barcodes. Proc Biol Sci. 2003;270(1512):313–321.
- Hebert PDN, Ratnasingham S, Dewaard JR. Barcoding animal life: cytochrome c oxidase subunit 1 divergences among closely related species. Proc R Soc Lond B. 2003;270(suppl_1):S96–S99.
- Schloss PD, Handelsman J, Relman D. Toward a census of bacteria in soil. PLoS Comput Biol. 2006;2(7):0786–0793.
- Stackebrandt E, Goebel BM. Taxonomic note: a place for DNA-DNA reassociation and 16S rDNA sequence analysis in the present species definition in bacteriology. Int J Syst Bacteriol Microbiol Soc. 1994;44(4):846–849.
- Blaxter ML, Godfray HCJ, Knapp S. The promise of a DNA taxonomy. Philos Trans R Soc B Biol Sci. 2004;359(1444):669–679.
- Pawlowski J, Lejzerowicz F, Apotheloz-Perret-Gentil L, et al. Protist metabarcoding and environmental biomonitoring: time for change. Eur J Protistol. 2016;55:12–25.
- Pernice MC, Logares R, Guillou L, et al. General patterns of diversity in major marine Microeukaryote Lineages. PLoS One. 2013;8(2):e57170.
- DeSalle R, Goldstein P. Review and interpretation of trends in DNA barcoding. Front Ecol Evol. 2019;7:1–11.
- Pawlowski J, Audic S, Adl S, et al. CBOL Protist Working Group: barcoding Eukaryotic richness beyond the animal, plant, and fungal kingdoms. PLoS Biol. 2012;10(11):e1001419.
- Buchheim MA, Keller A, Koetschan C, et al. Internal transcribed spacer 2 (nu ITS2 rDNA) sequence-structure phylogenetics: towards an automated reconstruction of the green algal tree of life. PLoS One. 2011;6(2):e16931.
- Fawley MW, Fawley KP, Buchheim MA. Molecular diversity among communities of freshwater microchlorophytes. Microb Ecol. 2004;48(4):489–499.
- Medlin LK, Metfies K, Mehl H, et al. Picoeukaryotic plankton diversity at the Helgoland time series site as assessed by three molecular methods. Microb Ecol. 2006;52(1):53–71.
- Not F, Del Campo J, Balagué V, et al. New insights into the diversity of Marine Picoeukaryotes. PLoS One. 2009;4(9):e7143.
- Hadziavdic K, Lekang K, Lanzen A, et al. Characterization of the 18S rDNA gene for designing universal Eukaryote specific primers. PLoS One. 2014;9(2):e87624.
- Zimmermann J, Jahn R, Gemeinholzer B. Barcoding diatoms: evaluation of the V4 subregion on the 18S rDNA gene, including new primers and protocols. Org Divers Evol. 2011;11(3):173–192.
- Hadi SIIA, Santana H, Brunale PPM, et al. DNA barcoding green microalgae isolated from neotropical Inland waters. PLoS One. 2016;11(2):e0149284.
- Lortou U, Gkelis S. Polyphasic taxonomy of green algae strains isolated from Mediterranean freshwaters. Biol Res-Thessaloniki. 2019;26(1):11.
- Dadheech PK, Glöckner G, Casper P, et al. Cyanobacterial diversity in the hot spring, pelagic and benthic habitats of a tropical soda lake. FEMS Microbiol Ecol. 2013;85(2):389–401.
- Rosselló-Mora R. Updating prokaryotic taxonomy. J Bacteriol. 2005;187(18):6255–6257.
- Boyer SL, Flechtner VR, Johansen JR. Is the 16S-23S rDNA internal transcribed spacer region a good tool for use in molecular systematics and population genetics? A case study in cyanobacteria. Mol Biol Evol. 2001;18(6):1057–1069.
- Otsuka S, Suda S, Li R, et al. Phylogenetic relationships between toxic and non-toxic strains of the genus Microcystis based on 16S to 23S internal transcribed spacer sequence. FEMS Microbiol Lett. 1999;172(1):15–21.
- Rocap G, Distel DL, Waterbury JB, et al. Resolution of Prochlorococcus and Synechococcus ecotypes by using 16S-23S ribosomal DNA internal transcribed spacer sequences. Appl Environ Microbiol. 2002;68(3):1180–1191.
- Wilmotte A. Molecular evolution and taxonomy of the Cyanobacteria. In: D.A. B, editor. Mol Biol Cyanobacteria. Dordrecht: Springer Netherlands; 1994. p. 1–25.
- Wilmotte A, Neefs JM, De Wachter R. Evolutionary affiliation of the marine nitrogen-fixing cyanobacterium Trichodesmium sp. strain NIBB 1067, derived by 16S ribosomal RNA sequence analysis. Microbiology. 1994;140(8):2159–2164.
- Mikhailyuk T, Glaser K, Tsarenko P, et al. Composition of biological soil crusts from sand dunes of the Baltic Sea coast in the context of an integrative approach to the taxonomy of microalgae and cyanobacteria. Eur J Phycol. 2019;54(3):263–290.
- Ríos-Touma B, Holzenthal RW, Huisman J, et al. Diversity and distribution of the Caddisflies (Insecta: trichoptera) of Ecuador. Peer J. 2017;5:e2851.
- Gunkel G, Beulker C. Limnology of the crater Lake Cuicocha, Ecuador, a cold water tropical lake. Int Rev Hydrobiol. 2009;94(1):103–125.
- Andersen RA. Algal culturing techniques. London: Elsevier Academic Press; 2005.
- Bellinger EG, Sigee DC. Freshwater algae: identification, enumeration and use as bioindicators. Wileyblackwell; United States of America, 2015.
- Serediak N, Huynh M. Algae identification lab guide : accompanying manual to the algae identification field guide. Canada. CA and A-F, editor. Ottawa: Agriculture and Agri-Food Canada; 2011.
- Guiry MD, Guiry GM. AlgaeBase. Galway: World-wide electronic publication, National University of Ireland. https://www.algaebase.org; searched on 01 November 2020.
- Kumar S, Stecher G, Li M, et al. MEGA X: molecular evolutionary genetics analysis across computing platforms. Mol Biol Evol. 2018;35(6):1547–1549.
- Edgar RC. MUSCLE: multiple sequence alignment with high accuracy and high throughput. Nucleic Acids Res. 2004;32(5):1792–1797.
- Mareš J, Hrouzek P, Kaňa R, et al. The primitive Thylakoid-Less Cyanobacterium Gloeobacter is a Common Rock-Dwelling Organism. PLoS One. 2013;8(6):e66323.
- Mora D, Abarca N, Proft S, et al. Morphology and metabarcoding: a test with stream diatoms from Mexico highlights the complementarity of identification methods. Freshw Sci. 2019;38(3):448–464.
- Zimmermann J, Abarca N, Enk N, et al. Taxonomic reference libraries for environmental barcoding: a best practice example from diatom research. PLoS One. 2014;9(9):e114758.
- Zimmermann J, Glöckner G, Jahn R, et al. Metabarcoding vs. morphological identification to assess diatom diversity in environmental studies. Mol Ecol Resour. 2015;15(3):526–542.
- Correia N, Pereira H, Silva JT, et al. Isolation, identification and biotechnological applications of a novel, robust, free-living Chlorococcum (Oophila) amblystomatis strain isolated from a local pond. Appl Sci. 2020;10(9):3040.
- Hoshina R, Fujiwara Y. Molecular characterization of Chlorella cultures of the National Institute for Environmental Studies culture collection with description of Micractinium inermum sp. nov., Didymogenes sphaerica sp. nov., and Didymogenes soliella sp. nov. (Chlorellaceae, Tr.). Phycol Res. 2013;61:124–132.
- Leliaert F, Verbruggen H, Vanormelingen P, et al. DNA-based species delimitation in algae. Eur J Phycol. 2014;49(2):179–196.
- Lee SR, Oak JH, Chung IK, et al. Effective molecular examination of eukaryotic plankton species diversity in environmental seawater using environmental PCR, PCR-RFLP, and sequencing. J Appl Phycol. 2010;22(6):699–707.
- Wang Q, Song H, Liu X, et al. Morphology and molecular phylogeny of coccoid green algae Coelastrella sensu lato (Scenedesmaceae, Sphaeropeales), including the description of three new species and two new varieties. J Phycol. 2019;55(6):1290–1305.
- Ancona-Canché K, López-Adrián S, Espinosa-Aguilar M, et al. Molecular phylogeny and morphologic data of strains of the genus Coelastrella (Chlorophyta, Scenedesmaceae) from a tropical region in North America (Yucatan Peninsula). Bot Sci. 2017;95(3):527–537.
- Hegewald E, Wolf M. Phylogenetic relationships of Scenedesmus and Acutodesmus (Chlorophyta, Chlorophyceae) as inferred from 18S rDNA and ITS-2 sequence comparisons. Plant Syst Evol. 2003;241(3–4):185–191.
- Zou S, Fei C, Wang C, et al. How DNA barcoding can be more effective in microalgae identification: a case of cryptic diversity revelation in Scenedesmus (Chlorophyceae). Sci Rep. 2016;6(1):1–13.
- Krienitz L, Hegewald EH, Hepperle D, et al. Phylogenetic relationship of Chlorella and Parachlorella gen. nov. (Chlorophyta, Trebouxiophyceae). Phycologia. 2004;43(5):529–542.
- Sciuto K, Lewis LA, Verleyen E, et al. Chodatodesmus australis sp. nov. (Scenedesmaceae, Chlorophyta) from Antarctica, with the emended description of the genus Chodatodesmus, and circumscription of Flechtneria rotunda gen. et sp. nov. J Phycol. 2015;51(6):1172–1188.
- Eliáš M, Němcová Y, Škaloud P, et al. Hylodesmus singaporensis gen. et sp. nov., a new autosporic subaerial green alga (Scenedesmaceae, Chlorophyta) from Singapore. Int J Syst Evol Microbiol. 2010;60(5):1224–1235.
- Hegewald E. A new subdivision of the genus Scenedesmus Meyen. Nov Hedwigia. 1978;30(1–4):343–376.
- An SS, Friedl T, Hegewald E. Phylogenetic relationships of Scenedesmus and Scenedesmus-like Coccoid Green Algae as Inferred from ITS-2 rDNA Sequence Comparisons. Plant Biol. 1999;1(4):418–428.
- Huss VAR, Frank C, Hartmann EC, et al. Biochemical taxonomy and molecular phylogeny of the genus Chlorella sensu lato (Chlorophyta). J Phycol. 1999;35(3):587–598.
- Darienko T, Pröschold T. The genus Jaagichlorella Reisigl (Trebouxiophyceae, Chlorophyta) and its close relatives: an evolutionary puzzle. Phytotaxa. 2019;388(1):047–068.
- Cadillo-Quiroz H, Didelot X, Held NL, et al. Patterns of gene flow define species of Thermophilic Archaea. PLOS Biol. 2012;10(2):e1001265.
- Eckert EM, Fontaneto D, Coci M, et al. Does a barcoding gap exist in prokaryotes? Evidences from species delimitation in cyanobacteria. Life. 2015;5(1):50–64.
- Schleifer KH. Classification of bacteria and archaea: past, present and future. Syst Appl Microbiol. 2009;32(8):533–542. Dec 1.
- Valério E, Chambel L, Paulino S, et al. Molecular identification, typing and traceability of cyanobacteria from freshwater reservoirs. Microbiology. 2009;155(2):642–656.
- Komárek J, Anagnostidis K. Modern approach to the classification system of Cyanophytes 4 - Nostocales. Algol Stud für Hydrobiol. 1989; Suppl Vol. 56:247–345.
- Moreira C, Vasconcelos V, Antunes A. Genetic characterization of Microcystis aeruginosa isolates from Portuguese freshwater systems. World J Microbiol Biotechnol. 2016;32(7):32.
- Kurobe T, Lehman PW, Hammock BG, et al. Biodiversity of cyanobacteria and other aquatic microorganisms across a freshwater to brackish water gradient determined by shotgun metagenomic sequencing analysis in the San Francisco Estuary, USA. PLoS One. 2018;13(9):1–20.
- Yoshida M, Yoshida T, Satomi M, et al. Intra-specific phenotypic and genotypic variation in toxic cyanobacterial Microcystis strains. J Appl Microbiol. 2008;105(2):407–415.
- Singh R, Parihar P, Singh M, et al. Uncovering potential applications of cyanobacteria and algal metabolites in biology, agriculture and medicine: current status and future prospects. Front Microbiol. 2017;8:1–37.
- Włodarczyk A, Selão TT, Norling B, et al. Newly discovered Synechococcus sp. PCC 11901 is a robust cyanobacterial strain for high biomass production. Commun Biol. 2020;3(1):215.
- Vela-garcía N, Guamán-Burneo MC, González-Romero NP. Efficient bioremediation from metallurgical effluents through the use of microalgae isolated from the Amazonic and highlands of Ecuador. Rev Int Contam Ambie. 2019;35(4):917–929.
- Hodač L, Hodač H, Hallmann C, et al. Widespread green algae Chlorella and Stichococcus exhibit polar-temperate and tropical-temperate biogeography. FEMS Microbiol Ecol. 2016;92(8):122.
- Aray-Andrade MM, Uyaguari-Diaz MI, Rafael Bermú Dez J, et al. Short-term deleterious effects of standard isolation and cultivation methods on new tropical freshwater microalgae strains dist. PeerJ. 2018;6:e5143.
- Ballesteros I, Castillejo P, Haro AP, et al. Genetic barcoding of Ecuadorian epilithic diatom species suitable as water quality bioindicators. C R Biol. 2020;343(1):41–52.
- Chagnon PL, Magain N, Miadlikowska J, et al. Strong specificity and network modularity at a very fine phylogenetic scale in the lichen genus Peltigera. Oecologia. 2018;187(3):767–782.
- Nübel U, Muyzer G, Garcia-pichel F, et al. PCR primers to amplify 16S rDNA genes from cyanobacteria PCR primers to amplify 16S rDNA genes from Cyanobacteria. Microbiology. 1997;63:3327–3332.
- Visco JA, Apothéloz-Perret-Gentil L, Cordonier A, et al. Environmental monitoring: inferring the diatom index from next-generation sequencing data. Environ Sci Technol. 2015;49(13):7597–7605.