ABSTRACT
Currently, there has been a growing interest in fermented foods and plant-based beverages (PBBs) by the consumers because of the benefits they provide to human health or due to restrictions in the diet associated to some pathologies or personal choices. Nuclear magnetic resonance (NMR) is a versatile technique that presents many advantages for the identification and quantification of metabolites in food with a variety of one- and two-dimensional experiments. This review delves into the current applications of NMR in the fields of fermented foods and PBBs. The interest from researchers in the analysis of fermented foods by NMR in the recent literature mainly focused on three main sub-areas: characterization of exopolysaccharides (EPS) and their functional, and rheological properties; metabolomics to find discriminant markers during and after the process of fermentation for the optimization of the productive process or development of products; and characterization of traditional and novel foods. However, the area of plant-based beverages studies by NMR presented a remarkable literature gap. The opportunities for future investigations concerning food authentication, traceability, and functional food development, among others, are presented.
Introduction
Recently, a sharp increase in the production of processed foods has been observed. This phenomenon is intimately connected to the prevalence of diseases like obesity or specific metabolic disorders.[Citation1,Citation2] In this sense, the consumption of minimally processed foods is encouraged to face this issue. Within this group, we can find fermented foods and plant-based beverages. These two types of food fit perfectly into the previously mentioned category because of their low to null degree of industrialization.[Citation3] In the recent years, consumers have been changing their dietary habits and a trend towards sustainable diets has been detected. The consumption of wholefoods and plant-based foods with less contribution from animal sources is widely promoted worldwide as it brings benefits for both consumers’ health and the environment.[Citation4,Citation5]
Fermented foods are foods or beverages that are produced by regulated microbial growth and enzymatic transformations of main and secondary dietary constituents. They can be prepared either from fermented milk (cheese, yogurt, kefir) or plants (kombucha, soy-based fermented foods, sauerkraut, and sourdough among others). The most important substances and microorganisms participating in the fermentation of food encompass alcohol and carbon dioxide (generated by yeast), acetic acid (produced by Acetobacter), lactic acid (produced by lactic acid bacteria (LAB)), as well as ammonia and fatty acids (originating from Bacillus and molds).[Citation6] LAB interact with the food matrix by transforming its components through three major pathways: glycolysis (fermentation of sugars), proteolysis (degradation of proteins), and to a minor extent but also playing a critical role to food flavor development, lipolysis (degradation of fat).[Citation7] The compounds formed during fermentation, including vitamins, antioxidant substances, free amino acids, and phenolic compounds, contribute to enhancing the antioxidant and antibacterial properties of food, as well as providing benefits to human health in terms of disease prevention.[Citation8–10]
Plant-based beverages are a rapidly expanding market worldwide.[Citation11] A wide variety of vegetal species have been chosen to produce plant-based beverages, for example: rice, soy, peanut, nuts, almonds, coconut, hazelnut, sesame, flax, sunflower, quinoa, among others.[Citation12] The benefits to human health of these products have been also studied, as they possess antioxidant activity and fatty acids which reduce the risk of cancer, diabetes, atherosclerosis, and cardiovascular diseases.[Citation13]
Nuclear magnetic resonance (NMR) is a versatile analytical technique that detects chemical compounds by exposing different nuclei with specific nuclear spin states to a static external magnetic field (B0) and a radiofrequency field (Rf) perpendicular to the direction of the magnetic field. It benefits from being a highly reproducible, and non-destructive approach that identifies various classes of organic compounds. It also facilitates the unambiguous elucidation of their structures and the quantitation of the generated signals. Moreover, NMR offers simplified sample extraction processes, and automation of procedures. However, its drawbacks encompass limited sensitivity, reduced spectrum resolution, signal overlapping, and challenges in reproducing and standardizing protocols.[Citation14,Citation15] Currently, two-dimensional NMR (2D NMR) has become a necessary tool for the complete structural elucidation of organic molecules and to solve the issue of overlapping of signals in complex mixtures. Over the years, NMR studies have employed techniques such as total correlation spectroscopy (TOCSY), correlation spectroscopy (COSY), heteronuclear 1H,13C single quantum coherence (1H-13C-HSQC), heteronuclear multiple bond correlation (HMBC), among others.[Citation16]
A comprehensive view of the composition of the food matrix is always obtained by the complimentary application of different analytical techniques. The study of fermented foods and plant-based beverages by other analytical techniques such as mass spectrometry has been the objective of previous articles, emphasizing the advantages for the detection and quantification of volatile compounds, lipids, proteins, and its high dynamic range.[Citation17–21] The objective of review is to describe the latest applications of NMR techniques in plant-based fermented foods, kefir, and plant-based beverages, highlighting the advantages of the techniques and identifying literature gaps. In addition to this, we discuss the challenges and opportunities for future studies in these areas to bridge these gaps.
NMR applications in fermented foods
In the recent years, the application of 1D and 2D NMR techniques in fermented foods has been mostly oriented towards three main areas. shows the overall distribution of main topics in studies carried out on fermented foods. Clearly, the analysis of fermented foods by 1D NMR metabolomics has received enormous attention from researchers (50%). Within this area, profiling and characterization studies were more common in fermented foods (48%), focusing on the chemical composition of the matrices or their ingredients. Less studies were focused on other useful approaches within metabolomics such as classification (8%) and studying the impact of processing or storage conditions and optimization of the fermentation process to enhance the properties of the final product (8%). Secondly, numerous studies focused on the structural characterization of the exopolysaccharides (EPSs) produced by LAB (40%). However, the use of these techniques was focused exclusively on the structural elucidation of the EPSs and not on the changes on its functional properties after its incorporation in food matrices. Finally, the area with a smaller number of studies in the recent years included the studies where NMR techniques were applied to study some ingredients or nutraceutical compounds in fermented foods from a nutritional view (10%). The studies in fermented foods by 1D and 2D NMR are presented in .
Table 1. 1D and 2D NMR studies in fermented foods.
NMR for the structural characterization of EPS
EPSs are high-molecular biopolymers from 10 to 1000 kDa produced by bacteria that are found in the biofilms produced by them.[Citation22] Based on their composition, they can be classified as homopolysaccharides (HoPs) which are constituted only by monosaccharides linked through glycosidic bonds or heteropolysaccharides (HePs), constituted by different monosaccharide units in different proportions; repeated units of di- up to heptasaccharides; and other functional groups like amines and acids.[Citation23] Within HePs, common examples are hyaluronic acid, gelatin, alginate and xantham gum.[Citation24] Not only their composition but also the producing strain affect their functional properties, among which we can mention antitumoral, antioxidant, antiviral, antidiabetic, and prebiotic.[Citation25] Since they are mostly or, in some cases, only composed of sugars, they are innocuous. This makes them excellent candidates for applications in the food sector, pharmacology, and cosmetic industry.[Citation26] The study of the EPSs produced by LAB obtained from fermented foods by 1D and 2D NMR focused on the structural elucidation of the polysaccharide. The most representative chemical assignments of the HoPs and HePs assigned by the authors cited in this review are presented in , respectively, to improve the interpretation of results and as a visual complement for the reader. The producer strains of each class of EPS were listed. It is noteworthy, that the complete structural elucidation of HePs is a highly complex task and requires more 2D experiments. Only 1 of the reviewed articles where a HePs was found focused on its complete structural elucidation. In any case, we listed all the producer strains of HePs and their compositions.
Figure 2. Representative chemical shifts of HoPs produced by LAB.
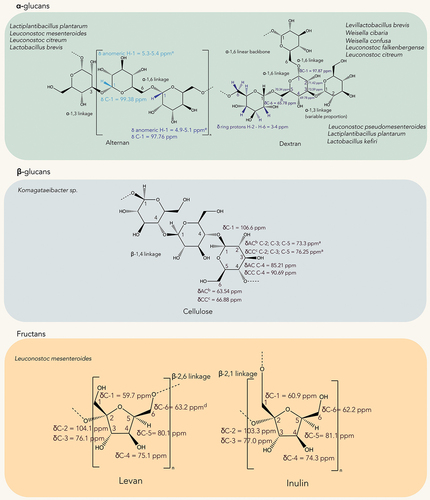
Figure 3. Representative chemical shifts of HePs produced by LAB.
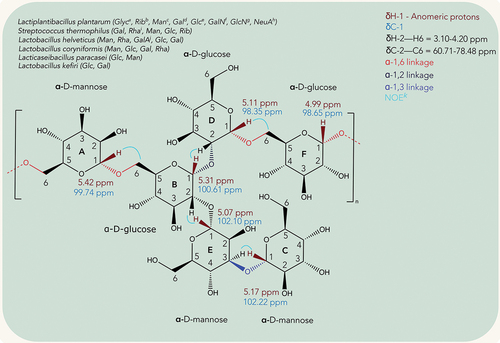
In this work, we explored the different EPSs produced by LAB from fermented foods focusing on the 1D and 2D NMR features that led to their partial or complete structural elucidation. Furthermore, we highlighted the functional properties of the EPSs to identify opportunities in fermented foods.
Kefir and water kefir
Kefir grain is a traditional dairy product widely consumed around the world. Its consumption has demonstrated benefits for health, which are mostly due to the presence of microbes and primary and secondary metabolites produced during fermentation.[Citation27] Kefir consists mainly of a mixture of yeast, acetic acid bacteria, and LAB. These are all contained inside a matrix constituted by polysaccharides and proteins.[Citation28] The analysis of the EPS produced by Lactobacillus kefiri MSR 101 from Chinese kefir by high performance liquid chromatography (HPLC), Fourier transform infrared spectroscopy (FT-IR), and 1D NMR (1H and 13C) revealed the presence of a HePs composed of glucose and galactose in different proportions. From the 1H NMR analysis, the authors observed the typical signals in the ring proton and anomeric regions, but also in a third region from 2.3–1.2 ppm (the aliphatic or alkyl region). In this case, this information, and the signal in the region from 4.0–3.3 ppm confirmed the presence of N-acetyl groups in the structure of the EPS. 13C NMR provides valuable information about the carbon skeleton of a molecule. Its main disadvantage is limited sensitivity, that is mostly affected by two factors: the natural abundance of 13C is 1.108%, meaning that the probability of having 13C in the molecule is low (so a higher concentration of the analyte is needed to obtain a high-quality spectrum). 13C NMR analysis confirmed the previous assignments by showing the carbon atoms in the anomeric region from 110–94 ppm; the ring carbon region from 80–56 ppm; and the carbon of the alkyl proton at 16.5 ppm to confirm the N-alkyl group in the structure. Cytotoxicity assays of the EPS showed increasing antitumoral activity against HT-28 cells (colon cancer) in ranges from 50–400 ug/mL, making it a promising candidate for the development of antitumoral functional foods, after the corresponding in vivo and clinical trials.[Citation29]
The same matrix was fermented with a different species, Lacticaseibacillus paracasei ZY-1, and the EPS structure was completely elucidated by a combination of 1D and 2D NMR techniques. The following workflow was followed to elucidate the structure. Firstly, the 1H NMR showed the presence of six distinct anomeric signals in the region from 5.42–4.99 ppm. The next step was to analyze the 13C NMR to obtain information about the number and chemistry of each carbon. Six signals were found in the zone between 102.22–98.35 ppm. Here comes the application of the HSQC spectrum, the major advantage is the cross-peak between both axes indicating which proton is attached to each carbon, so each proton was linked to its corresponding C1 atom.[Citation30] Since protons H2 to H6 are in a zone where overlapping occurs, the COSY spectrum helped solving this issue. Not only by confirming the shifts observed in the 1H NMR but also indicating the coupling between protons and the extension of the spin systems inside each monomer, giving an initial idea of the position of each monomer inside the EPS. Finally, the full spatial distribution and branching point of the EPS were confirmed by the NOESY and HMBC experiments. In the NOESY experiment, the magnetization is transferred through the space, not through the bonding electrons. The NOE effect is observed with a cross-peak and indicates a distance between nuclei of less than 5Å ().[Citation30] Finally, the HMBC experiment provided long-range interactions between 1H and 13C nuclei (mostly2J and3J and rarely4J).[Citation30] With the help of FT-IR, the spatial distribution and composition of the repeating unit of the EPS were confirmed. The repeating unit of this HePs was composed by glucose and mannose (). The EPS from this strain showed excellent hydroxyl, 1,1-Diphenyl-2-picryl-hydrazyl (DPPH), and diammonium 2,2′-azino-bis(3-ethylbenzothiazoline-6-sulfonate) (ABTS) radical scavenging activities depending on the concentration, making it a very good candidate for development of antioxidant functional foods.[Citation31]
Water kefir is a yellowish beverage prepared by combining kefir grains, fruits, or their extracts in a sucrose solution.[Citation32] This beverage also contains alcohol, organic acids, and aroma compounds produced during the fermentation process. 2D NMR spectroscopy to obtain a comprehensive understanding of the untreated water kefir beverage’s composition and the kefir grains. As it was mentioned, EPSs can be classified in HoPs or HePs. Within HoPs, they are classified according to the bond and monomer composition. When glucose is the monomer unit, they can have α-1,6 and α-1,3 bonds (for example: reuteran, dextran, mutan, and alternan) or if the linkage is β-1,2 and β-1,3 (like cellulose and curdlan, respectively). When the monomer unit is fructose with β-2,6 and β-2,1 bond at O1 (levan and inulin, respectively) or in polygalactans when the repeating unit is a pentamer of galactose, rarely seen in LAB.[Citation24] The EPSs in water kefir were studied by 1D and 2D NMR with two different approaches. The first was direct precipitation with ethanol 80% v/v. The HSQC spectrum showed that glucose and fructose were the predominant signals, this indicated that sucrose was completely hydrolyzed during fermentation. From these two monomers, fructose was in higher proportion, pointing out the presence of fructans in this fraction. The α-glucan was confirmed by the 1H and 13C anomeric pair of signals C1H1 and the HSQC revealed the dextran α-1,6 signal and a ramification at the O3 position. The second strategy was a precipitation in successive steps (from 40% to 80% v/v of ethanol) to isolate and study each fraction. The most important findings of this approach were the presence of levans in kefir grains, suggesting the contribution of different strains in the beverage. However, the authors did not mention the involved strains in the study and could not establish if the levan had a β-2,6 or β −2,1 linkage.[Citation33] Notably, other studies could assign the signals to levan or inulin fructans with information from the 13C spectrum. By direct comparison of the findings in previous studies,[Citation34] the levan from this study could be an inulin-type levan based on the shift of C-1 at 60.5 ppm found by the authors that is distinctive of this type of levans.
Kombucha
Kombucha is a popular stimulating beverage made by fermenting sugared tea with acetic acid bacteria (AAB, mostly from the genera Acetobacter and Komagataeibacter) and a yeast symbiotic colony. Within AAB, it has been demonstrated that microorganisms of the Acetobacter genus can produce the so-called bacterial cellulose. Many researchers have focused their efforts to scale up the production of bacterial nanocellulose (BNC) since Acetobacter xylinum was discovered for its ability to generate cellulose.[Citation35] New trends are also emerging in the field of materials science, as world population is shifting towards sustainable and environmentally friendly options. In this field, nanocellulose is one of the options that can potentially replace fuel-based plastics because of its technological and rheological properties.[Citation36] To avoid concerns regarding deforestation for the obtention of this biopolymer, new synthetic strategies are being analyzed. So, a study investigated the technological characteristics of the BNC produced by Komagataeibacter rhaeticus DSM 16,663 T isolated from kombucha. Solid-state NMR is the fundamental technique for the study of the spatial distribution of molecules in a solid sample. Since solid samples are often inhomogeneous, they may experience differences in the magnetic environment of the nuclei (anisotropy). Modern approaches like magic angle spinning (MAS) have become necessary to average out this dispersion of the magnetic radiation in the protons of the solid network.[Citation37] In this case, the use of cross-polarization (CP-MAS) solid-state NMR facilitated the assessment of the crystallinity of BNC by analyzing the areas under C-4 in the spectrum corresponding to both crystalline and amorphous cellulose and the 13C spectrum allowed the assignment of carbon atoms from both crystalline and amorphous zones (). This study set a starting point for the obtention of sustainable and eco-friendly alternatives derived from bacteria in fermented foods. However, the authors highlighted the high production costs.[Citation38]
Fermented fruits and vegetables
The use of NMR spectroscopy for the analysis of both fermented fruit products and their EPSs is an area that has been largely underexplored. Fermented plant products firstly appeared in Japan and quickly gained popularity throughout Asia, particularly in China and Korea.[Citation39] Scientific evidence indicates that these products contain essential nutrients and a variety of bioactive components that give them functional activities, such as antioxidant,[Citation39,Citation40] and antihyperglycemic properties.[Citation41]
The EPS from Leuconostoc citreum B-2 isolated from a homemade pineapple fermented product was studied. Particularly, this EPS showed a highly branched structure with prevalence of α-1,6 glucopyranose units (75%), followed by α-1,3 branches (19%), typical of alternan-type glucans (). The HSQC spectrum allowed to discriminate between α-1,6 and α-1,3 signals and the 13C spectrum confirmed that this glucan had very few α-1,2 branches. Interestingly, the water retention capacity of this highly branched EPS was around 450%. This would allow its use to modulate the rheological behavior of novel dairy fermented products. Moreover, it showed high resistance to enzymatic digestion, making it a good candidate for incorporating probiotics into the structure to effectively reach the intestinal tissue.[Citation42]
Another study perfectly illustrates the concept that the degree of branching and consequently, the properties of the EPS, depend specifically on the producer strain. A highly linear dextran produced by Weissella cibaria YB-1 isolated from a Chinese cabbage was analyzed by NMR and FT-IR and its antioxidant properties were also studied. In this case, the 1D NMR analyses showed a 95% of α-1,6 bonds, followed by only a 5% of α-1,3 indicating a highly linear polymer. Particularly, the 13C spectrum allowed assignment of C-2 to C-5 and showed a clear signal at 65.78 ppm for C-6, exclusive of an α-1,6 linkage. The degree of linearity dramatically changed the water holding capacity of the EPS driving it to a 287% but not limiting its potential use as stabilizer or emulsifying agent in food or cosmetics, along with its good antioxidant properties to offer functional enhancements to novel products.[Citation43]
In another study, the EPS from Lactobacillus plantarum HY isolated from a Sichuan pickle was studied. The analysis by 1H, 13C NMR and FT-IR revealed the presence of glucose, galactose, mannose and fucose as main constituents of the EPS. The absence of the signal at 5.4 ppm was the key to conclude that all the residues were pyranose-like. In this case, the EPS exhibited amylase-inhibiting properties, making it a promising candidate for exploring antidiabetic functional properties in novel products.[Citation44] Another study that worked with the same matrix (Sichuan pickle) but a different strain (Bacillus sp.) obtained two fractions from the EPS (S-1 and S-2). Even though the 1H and 13C NMR analysis showed that the units were the same as in the previous study (galactose, glucose, and mannose), the molecular weight of the S-2 fraction was higher. This consequently impacted on the hydroxyl groups quantity and distribution, giving this fraction a protective capacity against H2O2 depending on the concentration applied.[Citation45]
Northeastern sauerkraut is a fermented food prepared from cabbage. It is famous among Chinese customers because it possesses a unique flavor but also because of its high nutritional value, and its ease of preparation.[Citation46] The EPS produced by Levilactobacillus brevis HDE-9 isolated from sauerkraut revealed, with the help of the COSY experiment and the coupling constant values, that the EPS chain’s building block was glucose. This research established that this EPS was a glucan (such as dextran) produced from glucose consisting of α-1,6 units on the chain with no branching with further applications in the dairy industry.[Citation47] The analysis of the EPS produced by Lactobacillus coryniformis in the same matrix by NMR, FT-IR and HPLC allowed concluding that the EPS was totally confirmed to be a HePs composed of α -mannose, α -glucose, α -galactose, and α -rhamnose residues. This EPS not only showed very good radical scavenging activity but also bacterial biofilm inhibition properties and dispersion of pre-constituted biofilms of Salmonella typhimurium.[Citation48]
Recently, the EPS structural and biological characteristics from Lactiplantibacillus plantarum HDC-01 isolated from sauerkraut were investigated. In this case, the 1D and 2D NMR and FT-IR analyses revealed a mostly linear HoPs composed mostly by α-1,6 bonds with some α-1,3 branching. After matching each anomeric and ring proton to its carbon (1H,13C, and HSQC spectra), the COSY spectrum allowed the calculation of the JH2-H3, JH3-H4, and JH4-H5 coupling constants to confirm the presence of a pyranose in the structure. Then, the NOESY and HMBC spectra validated all the previous assignments by showing the inter-residue spatial connections. In terms of functional properties, the EPS exhibited milk coagulation properties and very good thermal stability, making it a very good candidate for its use in the dairy industry.[Citation49]
Sourdough
Sourdough is made by fermenting flour with native LAB and yeasts found in the flour and the environment.[Citation50] It is a matrix of variable composition, and this showcases the ability of bacteria to adapt to those changing conditions (availability of nutrients, carbohydrates, pH).[Citation51] The most important benefits of sourdough are not only those imparted by fermentation (prolonged shelf-life, bioactive compounds) but also improved rheological properties to different doughs and bread such as volume and texture.[Citation52]
The EPS produced by Lactobacillus brevis E25 from sourdough was compared with 2 commercially available EPSs, namely, alternan and dextran. According to the results, glucose was the only sugar found in the E25 EPS. 1D, 2D NMR, and methylation analysis of the E25 EPS revealed that it was a highly branched glucan with α-1,3 and α-1,6 glycosidic connections (), which was structurally comparable to a previous report of the EPS from Lactobacillus reuteri 180.[Citation53] In this case, where 3 HoPs with very subtle structural differences were compared, specific 2D NMR spectra could perfectly solve the overlapping problem and completely elucidate the three structures. TOCSY can be thought of as an extension of a 1H-1H COSY experiment. In a TOCSY experiment, a strong magnetic pulse is given so that the magnetization is transferred along the complete spin systems of the molecule, scaling their chemical shift differences to zero. So, the coupling between all the protons of each spin system can be readily observed.[Citation30] This advantage can be improved even further in case of many overlapping regions and similar spin systems like in sugars. This is done by projecting the result of the experiment into the 13C axis, so a HSQC-TOCSY spectrum is obtained. In this work, the HSQC-TOCSY, TOCSY, and HMBC spectra were the key in discerning glucose residues with identical substitution patterns but positioned at different locations within the structure, observed in the three EPSs.[Citation54] The EPS produced by strain Leuconostoc mesenteroides S81 was also isolated from sourdough. In this study, HPLC analysis was employed to determine the composition of monosaccharides of the EPS S81, and in this case fructose was found to be the only monomer in the structure. 1H NMR spectroscopy indicated that this EPS was a levan type as a β-2,6 fructan. Particularly, 13C NMR revealed specific features that confirmed the presence of a levan instead of an inulin type of HoPs. Firstly, the shift in the signal of C-6, now observed at 63.2 ppm confirmed the β-2,6 bond (). Secondly, the absence of the low intensity signal at 0.2 ppm from the levan C-3 suggested that this was also a linear levan. FT-IR analysis helped to confirm the presence of this furanoid units. This levan showed an important immunomodulatory effect and very good antioxidant activity in vitro, so it was proposed as a functional ingredient for the development of novel foods with both effects.[Citation55]
In another study, Weissella cibaria MED17 with a ropy phenotype was isolated from sourdough and the EPS produced by this strain was characterized. The 1H and 13C NMR spectra demonstrated that this EPS was a dextran-type glucan containing α-1,3 branches. Particularly, very few studies have informed the proportion of monomer units in the EPS based on NMR data. In this case, the proportion of α-1,6 to α-1,3 glucose units were 94.3:5.7%.[Citation56] The ratio of α-1,3 units was variable and depended on the present dextrans. Previous reports showed this proportion was between 2.4% and 3.4%,[Citation53,Citation57] whereas in this study levels of α-1,3 glucose units as branches around 5.7% were determined. This is significant because the degree of branching affects the technical properties of dextrans.[Citation53] Although the MED 17 EPS showed moderate antioxidant activity, its major features were its high degradation temperature (300℃) and microstructural features for its potential use as a thickening agent or in bakery products.[Citation56]
Some bacterial species can give the final product special characteristics in terms of nutritional and sensory attributes. Particularly, the conversion from glutamine to glutamate is carried out by L. sanfranciscensis via deamidation during sourdough fermentation. This process enhances bread flavor.[Citation58] L. sanfranciscensis has shown an essential role in prolonging the shelf-life and global texture characteristics of sourdough-based bread [Citation59], including its favorable improvement to scent, and enhancing the bioavailability of minerals and bioactive substances by creating more phytase.[Citation60] Within this context, 51 strains of L. sanfranciscensis were screened, and L. sanfranciscensis Ls-1001 resulted the highest EPS-producing strain. Therefore, it was analyzed for further EPS yield optimization and characterization by HPLC, FT-IR, and NMR. It was found that pyranose residues in the α-configuration were the main units of the polysaccharides, in agreement with the results obtained by FT-IR and the final yield was optimized to increase the production of this EPS with strong antioxidant activity.[Citation61]
In another study using a big number of strains isolated from sourdough, 72 microorganisms were identified from sourdoughs manufactured by Spanish bakers. Colonies of 22 isolates exhibited a ropy phenotype in the presence of sucrose, and the 1H NMR analysis of their EPS confirmed that 21 out of 22 were dextran producers. Notably, 3 Leuconostoc and 3 Weissella strains grew without a riboflavin supply and synthesized it themselves. The highest quantities of riboflavin and EPS were produced by W. cibaria strains (585–685 g/L and 6.5–7.4 g/L, respectively). As a result, these LAB strains represent excellent options to produce fermented foods that are bio-fortified with both dextrans and riboflavin.[Citation62]
Soybean fermented products
The analysis of the same chemical shift zones allowed the characterization of the EPSs produced by S. thermophilus SBC8781 from cow milk and soy milk, along with a combination of other analytical techniques like UV spectroscopy. The zones from 5.5–3.3 and 5.5–4.8 ppm revealed the presence of the protons attached to carbons 2 – 6 (ring protons), the hydroxyl protons and the anomeric protons. Both EPSs were HePs mainly composed by galactose, followed by other sugars in much lower proportions. Immunomodulatory essays of the EPSs showed a greater activity of the EPS from soy milk, which was proposed as a functional ingredient for the development of novel products with immunomodulatory activity, after the corresponding clinical trials.[Citation63]
Soybean paste is commonly consumed in Northern China, and it is prepared from fermented soybeans. This process involves several microorganisms, which might impact the flavor and color of the finished product.[Citation64] An EPS-producing strain, YF32, was isolated from soybean paste and identified as Leuconostoc pseudomesenteroides. The 1H and 13C NMR analyses revealed that this EPS was a glucan type made up of consecutive glucose units with an α-1,6 bond without branching. This EPS also presented high solubility, emulsifying properties, and thermal stability (Degradation temperature of 307 ℃) making it a good candidate for food applications.[Citation65]
NMR metabolomics in fermented foods
The omics sciences encompass the study of various substances crucial for living organisms, including lipids (lipidomics), proteins (proteomics), genes (genomics), and metabolites (metabolomics).[Citation66,Citation67] The latter, represents a potent, effective, and sensitive approach in analytical terms that is extensively used in food science. Its wide recognition is evident through its applications in two primary research areas: investigations of the impact of food on human health and of food processing and transformation.[Citation67,Citation68] Metabolomics techniques are mainly classified as targeted or untargeted. The hypothesis-based, targeted technique focuses on a subset of substances and is more focused on their quantification. This technique is more limited in that it only reaches a small number of metabolites; nonetheless, the changes produced by external alterations can be associated with fully identified molecules.[Citation69–71] The untargeted strategy, instead, creates a hypothesis; it is a more popular option because it covers a broader variety of molecules and can be effective in identifying discriminating metabolites between groups of samples. Both approaches integrate the analytical data with multivariate statistical techniques to extract possible classification patterns between the groups or generate mathematical models that classify samples based on discriminant metabolites.[Citation71,Citation72] In this section, we present the use of NMR-based metabolomics approaches in fermented foods where NMR was the core technique of the studies. For deeper insight regarding the multivariate statistical techniques commonly applied, as well as data pretreatment and normalization steps, the reader is referred to excellent reviews in area.[Citation73–76]
Fermented plant-based beverages
Nowadays, the popularity of non-dairy products is widely rising due to the increase of the population that is allergic to dairy or because they choose it as a lifestyle, specifically in Asian and African countries. In this sense, novel non-dairy probiotic drinks can be a good alternative. A study aimed to increase the overall quality of coconut milk kefir (CMK) (compared to coconut milk, CM) by studying its antioxidant and antimicrobial activities and identifying the peptides and metabolites in it. The latter were screened at the beginning and the end of the fermentation process by 1H NMR metabolomics to detect changes in them. CMK displayed a bigger variety of bioactive metabolites compared to CM, and the NMR technique enabled the quantification of these metabolites in both matrices. Both products contained sugars like xylose and sucrose, with higher quantities observed in CM. However, specific compounds such as lactic acid, biotin, butyrate, caprylic acid, and riboflavin were exclusively detected in CMK. Additionally, γ-aminobutyric acid (GABA) was found in higher concentrations in CMK compared to CM. Overall, CMK demonstrated superiority over CM, exhibiting elevated antioxidant power, antimicrobial activity, and an enhanced nutritional profile.[Citation77] In another study, the metabolite profile of coconut milk was studied by 1H NMR metabolomics after fermentation with Lactiplantibacillus plantarum ngue16, pasteurization, and different cold storage times (1, 7, 14, and 21 days). The extracts were classified into two groups, samples from day 1 (group 1) were separated from those at 14 and 21 days (group 2). Group 2 samples showed similarities, with a slight variation in their metabolites profile. Notably, fermented coconut milk stored for 7 days revealed a greater diversity of metabolites. The metabolites contributing to the differences between the 14th and 21st days of cold storage included alanine, ethanol, o-Phosphorylethanolamine, valine, GABA, succinic acid, acetic acid, tyrosine, and uridine. On the other hand, 1,3-dihydroxyacetone, 3-hydroxyphenylacetate, o-Phosphorylethanolamine, oleanolic acid, amino acids (phenylalanine, threonine, and isoleucine), choline, lactic acid, glutamate, sugars (glucose and fructose) were more abundant in fermented milk on the first day of storage. These findings suggested that both the fermentation process and subsequent cold storage could influence the variation in metabolites during the cold storage of fermented coconut milk.[Citation78]
Soymilk changes during fermentation have also been studied recently. In one study, the changes in the flavor, nutritional quality, and functional properties of soy milk during fermentation with Bacillus subtilis at four different time points (0, 24, 48, 84 h) were analyzed using 1H NMR metabolomics. The NMR data highlighted distinct metabolomic profiles at the four time points, indicating a substantial difference in the matrix before and after fermentation. Throughout the fermentation process, a total of 44 discriminant metabolites were identified. These contributed to changes in nutritional content, flavor, and functional properties of fermented soymilk. In comparison to the unfermented sample, the sample exhibited upward trends in free amino acids (e.g.: lysine, isoleucine, valine, leucine, tyrosine, and phenylalanine), while decreasing trends were observed for glucose-1-phosphate, 6-phosphogluconic acid, lactate, and glycine after 24 hours. A more complex pattern was observed by the authors between the 24-hour and 48-hour samples. They observed the same levels of free amino acids, genistein, acetate, and GABA, but a decrease in soybean lecithin and 6-phosphogluconic acid levels. Then, the pairwise comparison analysis of the 48-hour and 84-hour samples revealed an increase in pyruvate, GABA, isovalerate, and 2-methylbutyrate levels, and a decrease in 2,3-butanedione, histamine, and isobutyrate levels.[Citation79]
Fermented vegetable foods
Kimchi is a very famous and traditional fermented food consumed in Korea, and it has become a widely known product throughout the world due to its health advantages and nutritional profile.[Citation80] The components of kimchi are categorized into three main groups: vegetables (Chinese cabbage and radishes); seasonings like red pepper, garlic, and onion among others; and optional additives, such as jeotgal, a fermented fish sauce commonly consumed in Korea. Jeotgal is typically produced through the fermentation of highly salted sea animals, including whole fish, fish roe, or internal organs of fish.[Citation81] The influence of incorporating this ingredient into kimchi fermentation was investigated using 1H NMR metabolomics, with the quantification of metabolites conducted within the same analytical approach. Four groups of samples were analyzed, namely, kimchi without jeotgal (CK), kimchi with salt instead of jeotgal (NK), and two types of kimchi samples with jeotgal: kimchi with salted anchovies (SK), and kimchi with salted shrimps (MK). Kimchi with jeotgal showed higher levels of alanine, arginine, glutamate, isoleucine, leucine, lysine, valine, and GABA than kimchi without jeotgal. SK had greater alanine, arginine, glutamate, and lysine concentrations than CK and NK after 40 days of fermentation, and MK had much higher values. Isoleucine, leucine, and valine concentrations were less than 2 mM in CK and NK, but doubled in MK and SK. Additionally, the concentration of GABA exhibited fluctuations throughout the fermentation process in all four kimchi variations, with slightly elevated levels observed in MK and SK until the 16th day of fermentation. Jeotgal was identified as a crucial ingredient in kimchi fermentation, contributing to the enhancement of its nutraceutical properties.[Citation82] In another study, 17 probiotic type strains of LAB were studied to find better probiotic or co-culture starters for kimchi fermentation. The metabolite profile of the candidates was compared to that of L. mesenteroides, the commercial standard starter. Five candidates showed moderate to high growing rates in simulated kimchi juice at 37°C so their metabolite profiles were studied by gas chromatography coupled to mass spectrometry (GC-MS) and 1H NMR during kimchi fermentation. Both L. mesenteroides- and L. fermentum-kimchi had low glucose content, indicating high consumption rates for cell development. Mannitol is formed by the reduction of fructose that gives kimchi a fresh sweet flavor; its level was high in L. mesenteroides-, L. fermentum-, and L. reuteri-kimchi when compared to other samples. Notably, the lactate concentration in L. rhamnosus- and L. paracasei-kimchi was much lower, likely altering the quality of the sour flavor. Among the 15 amino acids discovered, alanine, glutamate, glutamine, glycine, and serine were the most common residues (>80%), and the glutamate concentration for umami taste was the highest in kimchi containing L. reuteri. When combined with sensory evaluations, these findings led to the conclusion that L. fermentum and L. reuteri hold promise as potential candidates for probiotic starters or co-cultures, suggesting their suitability for the development of health-promoting kimchi products.[Citation83]
More than 100 types of kimchi have been reported, mainly being prepared from fermented cabbage. Therefore, one study examined the effects of microorganisms found in the primary ingredients of four kimchi types during fermentation. High-throughput sequencing and 1H NMR spectroscopy was used to investigate the fermentation characteristics, the structure of the microbial communities, and the production of metabolites in food samples that were prepared changing only the main ingredients and keeping the seasonings. The concentrations of sugars and other compounds like ethanol, glycerol, and glycine in cabbage kimchi (C) and young radish kimchi (Y) changed in similar ways as the fermentation progressed; however, there was a marked increase in the concentration of mannitol in sample C compared to sample Y. Fructose, sucrose, and glycine concentrations decreased in green onion kimchi (G), but mannitol, butyrate, and ethanol concentrations increased, with butyrate and ethanol concentrations higher in sample G than in other samples. During fermentation in leaf mustard kimchi, there was a decrease in the concentrations of fructose and sucrose and an increase in the levels of mannitol, lactate, butyrate, and ethanol. The variation in bacterial composition resulted in variations in the metabolites generated in food. As a result, the primary ingredients played a significant role in food quality.[Citation84]
Sunki stands as a distinctive, traditional fermented pickle crafted solely in the Kiso district of Nagano Prefecture, Japan. The preparation involves the fermentation of red turnip (Brassica rapa L.) leaves with LAB (Tamang, Watanabe, and Holzapfel 2016). To study its aqueous fraction, this traditional fermented food was analyzed by 1D and 2D (HSQC) NMR metabolomics. The Sunki pickle composition comprised 3 sugars, 3 alcohols, 4 alditols, 9 amines, 10 organic acids, 19 amino acids, and 6 other chemicals. Notably, the levels of glucose, fructose, sucrose, and malic acid (primary metabolites found in turnip leaves) were generally below the detection limit in most samples. Dominating signals revealed major metabolites resulting from LAB carbohydrate fermentation, including lactic acid, ethanol, and acetic acid. Moreover, lower intensity signals were found from amino acids and their breakdown derivatives (amines and 2-hydroxy acids).[Citation85] The same research group also performed an NMR metabolomics approach to characterize another fermented food, Nozawana (Brassica rapa L. var. hakabura), to evaluate the effect of 4 different starter cultures in the water-soluble compounds compared to a sample without starter culture. Two starter cultures, Lactiplantibacillus plantarum K4G4 and K5G3, were differentiated based on their lactic acid production, followed by Latilactobacillus curvatus ♯4G2. Levilactobacillus brevis K4G1, on the other hand, showed higher presence of ethanol, acetic acid, and mannitol. The sample without a starter culture was characterized by predominant glucose and fructose signals. Subsequently, variables with major contributions were excluded, and multivariate statistical methods were repeated to assess the impact of low-intensity variables on class separation and introduce additional discriminating molecules. This led to the classification of Lactiplantibacillus plantarum K4G4 and K5G3 into group 1, and Latilactobacillus curvatus ♯4G2, Levilactobacillus brevis K4G1, and the sample without a starter culture into group 2. In the differentiation, succinic acid, acetoin, and docosahexaenoic acid (DHA) played significant roles in the first group, while choline, ornithine, GABA, and citric acid were more important in the second group.[Citation86]
For sauerkraut, two organic samples from two different producers (SK1 and SK2) were analyzed by 1H NMR analysis. The results showed the discrimination between the two samples based on both then strain composition and the metabolite profile. The metabolite profile showed unique changes during the fermentation process in both samples. Lactic acid and acetic acid reached maximum concentration in the samples at different days during the fermentation process. However, other acids like malic and butyric reached a peak in concentration only in SK2 at the end of the fermentation process. This effect was illustrated by the changes in sensory evaluation and technological properties of the final products.[Citation87] This article portrays the use of NMR for simultaneous quantification of metabolites during fermentation. This use could be extended to assess the quality of fermented products and studies regarding the evolution of the profile after specific storage conditions.
Kombucha
Spray drying is an industrial method that is used to produce powdered food because it is cost-effective and simple to regulate and operate.[Citation88] Kombucha tea is generally a sticky matrix, the responsible molecules for its viscosity are sugars and organic acids. These molecules would play a critical role at the time of spray drying the matrix. Adding a drying agent of high molecular weight could be a good strategy to face this issue.[Citation89] In this context, a study evaluated the effects of spray drying on the physicochemical, antioxidant, and antibacterial characteristics of kombucha using Arabic gum as a drying agent. The subsequent impact on the metabolite profile after the spray drying process was studied by 1H NMR metabolomics. Using sugared tea (ST) as a reference, the results revealed important differences between the ST and fresh kombucha tea (FKT) samples, particularly for sucrose and glucose. The authors observed a statistically significant (p < 0.05) decrease in the concentration of sucrose after fermentation of ST. On the other hand, the glucose level was higher in the FKT sample compared to the ST samples. A significant reduction in the concentrations of simple sugars, ethanol, acetic acid, and alanine in encapsulated kombucha tea samples compared to FKT samples was observed due to a dilution with the wall components and the effects of the high heat treatment. The authors demonstrated the influence of an industrial process on metabolites in fermented foods. Besides, this strategy could also be a good solution for allergic consumers searching for safe beverages and for those with Halal diets.[Citation90,Citation91]
The effect of different microbial organisms has also been studied in kombucha. A study revealed the different interactions among important species in kombucha microbiomes by constructing synthetic microbial pairs using a combination of metagenomics and NMR. Manipulation of these bacteria in kombucha could alter both the fermentation properties of kombucha as well as the creation of biofilms, which is useful for kombucha beverage manufacturers, biofilm engineers, and synthetic ecologists. Bacteria and yeast (B. bruxellensis and different strains of Komagataeibacter spp.) were used to prepare fermented kombucha with biofilms. Metagenomic sequencing revealed that B. bruxellensis emerged as the prevailing yeast. These findings aligned with a taxonomic survey of kombucha fermentations throughout the United States. Remarkably, functional kombucha fermentations were prepared with B. bruxellensis and K. rhaeticus. These co-cultures demonstrated the production of biofilms and achieved low pH levels, two key indicators of kombucha fermentation. Profiling the wasted media of both yeast species using NMR spectroscopy revealed B. bruxellensis greater capacity to ferment and generate critical metabolites in kombucha tea and may explain why it encourages biofilm development.[Citation92]
A very interesting, but rarely seen, application of NMR in the field of fermented foods is the detection of frauds or false claims in the labelling. One significant reason of the popularity of kombucha is the notion that it contains bacteria that help with gut health, which is reinforced by package claims like “live” and “probiotic.” A total of thirty-nine commercially available kombucha products, categorized as either non-alcoholic (soft) or alcoholic (hard), were examined, with 74.4% of them making at least one specific claim. The ‘hard’ kombucha, a relatively recent product, involves an anaerobic phase to enable ethanol accumulation beyond 0.5%. Through NMR analysis of their chemical composition, approximately one-third of the samples initially classified as soft kombucha products were re-labeled as “soft-aberrant” due to their alcohol by volume surpassing 0.5%. Samples with higher ethanol content tended to have lower residual sugar and a higher concentration of glycerol, a byproduct of fermentation. Analyzing correlations between these compounds revealed a positive association between ethanol and glycerol (r = 0.83, p < 0.005) and a negative association between ethanol and sucrose (r = 0.39, p < 0.01).[Citation93]
Functional ingredients can also be added during kombucha manufacturing to further enhance its functional properties and add different flavors at the same time. For example, soursop (Annona muricata L.) is a white-fleshed, aromatic, and juicy fruit with a creamy texture, and sour taste[Citation94] and previous reports mention it as a source of bioactive metabolites.[Citation95] In a particular study, the optimization of soursop kombucha production was carried out using a response surface methodology, and the impact of various storage conditions on quality, metabolites, and biological activity was investigated. A significant reduction (p < 0.05) in sucrose concentration was detected from day 7 to day 21 of storage, while a significant increase was observed for fructose and glucose during this period. This change was attributed to the hydrolysis caused by the invertase enzyme secreted by yeasts and bacteria.[Citation96] Notably, the fructose concentration consistently remained lower than glucose under all storage conditions. Furthermore, the levels of acetic acid in soursop kombucha samples showed an increase from day 7 to day 21, particularly when stored at room temperature. This increase was associated with the presence of Acetobacter bacteria, which contributed to the generation of acetic acid in the soursop kombucha samples. Over the period from 0 hours to day 21, a gradual decrease in the concentration of ethanol concentration in soursop kombucha samples was observed. Moreover, the decrease in ethanol concentration suggested that storage conditions could improve the overall quality of the fermented product for Halal consumers.[Citation97]
Fermented foods can also play a role as replacement of typical ingredients in other fermented foods. For example, kombucha was used as a replacement of baker’s yeast to produce sourdough bread. For this task, kombucha was introduced in the formulation after spray drying and encapsulation with Arabic gum. Kombucha not only imparted leavening properties to the dough, but also prolonged the final product’s shelf life and improved its functional properties. Using 1H NMR analysis combined with multivariate analysis, the bioactive compounds of sourdough starter with and without the addition of kombucha were identified. Two distinct sourdough starters were created: a liquid traditional sourdough starter (LTSS) and a liquid kombucha sourdough starter (LKSS). In particular, the LKSS was found to contain 15 bioactive compounds with diverse functions that have the potential to enhance biological function when compared to the LTSS.[Citation98]
Soybean fermented products
Organic foods are perceived to be superior to non-organic foods in terms of stated health advantages and environmental sustainability. Indeed, organic produce tends to be slightly more expensive, and NMR can serve as a tool to discern any metabolic differences associated with this certification. In a study, the chemical profiles of non-organic (G, T, U, UB) and organic (C, COF, A, R, B, Z) soybeans (G. max [L.] Merr.) were examined, along with the changes in metabolites after fermentation with Rhizopus oligosporus. Notably, a difference was observed only between samples G and Z. Subsequently, 1D and 2D NMR analyses were conducted on these two groups to identify discriminating metabolites. Phenolic compounds and isoflavones were not observed in the non-organic (G) and organic (Z) unfermented soybean spectra, this was confirmed by the absence of signals in the aromatic region from 5.5–9.0 ppm. The authors attributed this observation to the presence of high-intensity carbohydrate signals. Regarding the discriminating metabolites, caprylate, sucrose, and lysine were found in higher concentrations in non-organic G, whereas organic Z exhibited higher levels of citrate, isoleucine, choline, valine, and stachyose. Based on these results, these two groups were then fermented using R. oligosporus (GF and ZF, respectively). Thirty-two metabolites in the samples were identified. As it usually happens in the analysis of complex mixtures, there is an overlapping of signals mostly in the region from 3 to 5 ppm. In these cases, the use of 2D techniques is essential to solve this issue. In this case, the authors confirmed the structure of the metabolites by J-resolved 2D-NMR and HSQC spectra. Fermented soybeans showed a clearly distinct profile compared to the unfermented ones. In the former, metabolite signals were found in aliphatic and aromatic regions beside those of carbohydrates region (3.5–5.5). The comparison of the peak intensity showed higher intensities in GF samples (including aromatic and aliphatic regions), whereas ZF presented stronger signals in the region of carbohydrates.[Citation99]
In another study regarding the certification of organic practices in soy fermented products, conventional soy sauce (CS) and organic soy sauce (OS) were characterized and differentiated using 1H NMR-based metabolomics. Soy sauce is a traditional fermented product that originated in East Asian countries but is now used in cooking all around the world. Its flavoring compounds improve the taste of dishes, while its coloring agents improve the appearance of the dipped food. The metabolomics approach gave a clear separation of CS and OS and information about key metabolites that contribute to this separation. OS had higher quantities of leucine, threonine, isoleucine, valine, acetate, choline, lactate, phenylalanine, and tyrosine, whereas CS had higher levels of glucose, glutamate, and sucrose. The abundance ratio of 13C to 12C was also measured using 1H NMR spectroscopy, which revealed an enhanced ratio of 13C isotope in OS samples, showing that the OS was prepared using organically produced wheat and soybean. The findings may be useful in increasing consumers’ awareness about food fraud. This may also be an opportunity in the area for future traceability and authenticity studies of soy raw materials.[Citation100]
Miso is another traditional fermented food from Japan. The base ingredient is soy (like in natto and tempeh) but the fermentation is caused by Aspergillus oryzae in this case. Other microorganisms such as Saccharomyces cerevisiae or LAB species may also be involved in the fermentation process.[Citation101] 1H NMR metabolomic analysis was used to study the differences and properties between three types of miso: soybean miso, rice miso, and barley miso. The authors found that ethanol, glucose, and amino acids contributed to the discrimination of miso samples. Rice and barley miso were richer in ethanol and glucose, respectively. Soybean miso was differentiated by its high amino acid content, which included branched-chain amino acids. The authors highlighted the advantages of NMR not requiring any pretreatment in this case, its relative simplicity to measure and proposed it for food quality and control studies.[Citation102]
It has been reported that antioxidant peptides are released after the hydrolysis of rice protein.[Citation103] When these peptides were added to fermented foods, an inhibition of angiotensin-converting enzyme (ACE) with inhibitory action against hypertension was observed.[Citation104] Within this context, a rice protein hydrolysate was incorporated to fermented products (yogurts with soy and a Chinese beverage with barley) and 1H NMR metabolomics was used to identify discriminating metabolites. The products without the rice hydrolysate were richer in sugars including sucrose, glucose, and maltose. During fermentation, decreases in these molecules were observed in the beverages and this corresponded with an increase in lactate concentration. After the rice hydrolysate was added, metabolites from other chemical families like organic acids (acetate, pyruvate) and free amino acids (phenylalanine, alanine, tyrosine, leucine, and proline) were observed. This aligned with previous findings indicating elevated concentrations of pyruvate in the lacto-fermentation of dairy yogurt.[Citation105] Additionally, acetate emerges as a byproduct in the metabolism of pyruvate. Notably, two significant aroma compounds, diacetyl, and acetoin, arisen from the metabolism of pyruvate, contributed to the overall enhancement of the quality of the fermented products.[Citation106]
Kefir
Within the advantages of NMR measurements, they do not require any previous separation technique or sample derivatization steps, and they provide fast and direct information about the composition of a mixture. As a result, NMR spectroscopy enables in-situ monitoring and quantitative analysis in real time. During fermentation, chemical changes in kefir and two types or yogurt were monitored using an in-situ quantitative NMR approach. Remarkably, ethanol showed a sharp increase from 15 h of fermentation only in the kefir sample. This ethanol is produced from glucose at lower pH by the yeast and is what clearly distinguished kefir from the other samples. This was confirmed by the increase of the ethanol signals in the spectrum. Moreover, a sharp decrease was observed in the signals of citric acid from 10 h of fermentation in the kefir sample mainly due to the citric acid metabolism to produce acetaldehyde and diacetyl. Finally, the decrease in the galactose signal was explained by the production galactose-rich EPS, kefiran.[Citation107,Citation108]
Fruit juice
Commercialization of fermented fruit juice is difficult due to multiple variables such as the product’s high acidity, safety, unknown health benefits, and low popularity among consumers. On the other hand, it has a long shelf life and is resistant to food-borne spoilage microbes. This is a challenging area in which little research has been conducted. Dragon fruit (Hylocereus undatus) juice underwent fermentation by LAB, and the differences in metabolites, in comparison to non-fermented dragon fruit juice, were assessed through 1H NMR analysis. The differentiating metabolites between the two groups included lysine, alanine, acetic acid, succinic acid, lactic acid, glucose, iso-butyrate, and betaine. Dragon fruit fermented juice proved to be a novel functional food with enhanced nutritional benefits, better consumer perception and shelf-life.[Citation109] The same research group studied the impact of fermentation in cantaloupe (Cucumis melo) juice with L. plantarum FBS05 by 1H NMR metabolomics. Fermented cantaloupe juice not only presented more bioactive metabolites than the non-fermented juice, but also increased antimicrobial and antifungal activities mainly attributed to the presence of lactic acid, gamma-Aminobutyric acid (GABA), alpha-aminobutyric acid, acetoin, acetoacetate, phenylpropanoic acid, beta-alanine, gluconic acid and choline. Apart from this, the fermented juice also contributed to prolonging the shelf life of the non-fermented juice and increased consumer’s acceptance when mixed with non-fermented juice in different concentrations. This way, the fermented product (often with low popularity among consumers) was introduced and accepted by them.[Citation110] Similar effects were also observed in fermented jackfruit juice (Artocarpus heterophyllus Lam.) with Lactobacillus casei ATCC334 where the 1H NMR analysis revealed increased levels of GABA and lactic acid in the fermented product and a significant increase in the antimicrobial activity against Salmonella enterica serovar Typhimurium and Staphylococcus aureus. These changes were accompanied by an increase in antioxidant activity, good consumer acceptance, and a high rate of survival of the Bacillus sp. in the product even after 3 weeks of storage, making it a good candidate for the development of probiotic novel foods.[Citation111]
In another study, NMR emerged as a suitable technique for a comprehensive analysis of lactic acid-fermented fruit material, facilitating the optimization of malolactic fermentation with L. plantarum in Sea buckthorn juice (SBJ). For situations where malolactic fermentation is primarily employed for deacidification, the authors concluded to opt for shorter fermentation times, lower starter pH, and utilize acid-producing strains such as DSM 16,365 or DSM 20,174. On the other hand, if the modification of secondary metabolites, such as quinic acid or phenolic compounds, is the desired outcome, or if there is an intention to enhance the microbial or oxidative stability of the material, considerations should lean towards higher starter pH and extended fermentation times.[Citation112]
Sourdough
Spelt flour was utilized to prepare sourdough and the changes in the metabolome and the antioxidant power were studied by 1H NMR, in vitro, and ex vivo assays. The 1H NMR approach was optimized for the physico-chemical characterization of spelt flour extracts at different time points throughout the entire four-day fermentation process. This study illustrated the use of NMR for analyzing food composition along different timepoints. It could be extended to monitoring other bioactive components along the production chain to assess the effect of the addition of other functional ingredients to fermented foods. In the unfermented sample (T0), the spectrum region from 3.0 to 4.5 ppm was predominantly characterized by carbohydrates, particularly spelt starch. During the initial 24 hours, sourdough bacteria and yeasts hydrolyzed the spelt starch, leading to observable changes in this spectral region. Additionally, there was an increase in signals associated with aromatic compounds following the second fermentation step. Nevertheless, aromatic compounds like polyphenols were not specifically determined due to the high complexity of the matrix, which resulted in peaks overlapping and/or line broadening.[Citation113] The observed matrix effect, previously noted in other bakery matrices, could potentially be addressed by pre-treatment with C18 solid-phase extraction (SPE) cartridges before NMR analysis, enabling the identification of polyphenols.[Citation114]
Characterization of food products or their components
As we previously showed, NMR has been widely used for the characterization of EPS produced by LAB. In this section, the technique has also been utilized to characterize fermented food matrices and their components in combination with other analytical techniques and biological approaches.
Kefir and kefir yogurt
The microbiological composition of the grains has been extensively studied in recent years, with the composition depending on the source, which directly determines the type of metabolites present in kefir. Based on previous findings that highlighted the ability of various isolates from kefir to produce bacteriocins – genetically coded antimicrobial molecules – a study explored the diverse metabolite landscape of kefir grains. The objective was to evaluate whether certain kefir samples contained molecules distinct from bacteriocins, acids, alcohols, or hydrogen peroxide, with the potential to be extracted for the development of commercial antimicrobial products. A fraction named FK-1000 was isolated from kefir serum (the supernatant obtained after kefir centrifugation) by dialysis against distilled water with a membrane pore of 1 kDa. This fraction was subjected to characterization through RP-HPLC, mass spectrometry, and 1H NMR. The 1H NMR analysis revealed that the FK-1000 fraction primarily consisted of sugars (β-glucose, α-glucose, and β-galactose) and aliphatic amino acids, with a notable presence of polymers of alanine, tyrosine, and phenylalanine. The HPLC analysis indicated that the FK-1000 fraction comprised two sub-fractions. Further NMR analysis of sub-fraction 1 exhibited a similar profile to the FK-1000 fraction, although with a predominance of sugar and alanine polymers. Conversely, the 1H NMR results of subfraction 2 revealed the presence of a complex mixture that was difficult to characterize. Notably, both the FK-1000 fraction and sub-fraction 1 exhibited bactericidal and bacteriostatic effects against P. aeruginosa and methicillin-resistant S. aureus across different pH conditions, representing a novel class of antimicrobial molecules isolated from kefir.[Citation115]
Low-field NMR (LF-NMR) is another technique that is being applied in food science. The main reasons for this include its high sensitivity in identifying differences in water mobility and accompanying water transitions inside substances.[Citation116] The distribution of free and bound water in a food matrix can be studied with this technique. The T2 relaxation time (spin-spin relaxation of the H nuclei) is a useful parameter for determining this in fermented milk protein gel networks. The T23 parameter represents free water confined inside the gel’s network structure and is used to study milk gel systems.[Citation117] Regarding other fermented matrices, the technique has not been extensively applied and its advantages to study the rheological and structural properties of fermented foods have not been explored. In a recent study, kefir yogurt was fermented by mixed bacteria and by L. plantarum L1. The fermented products were then analyzed by a combination of scanning electron microscopy, confocal laser scanning microscopy, and LF-NMR. The aim was to study the relationship between the chemical profile, texture, rheological behavior, microstructure, and water distribution in these matrices. The LF-NMR analysis showed that the kefir group had the smallest area of water distribution and the lowest content of free water (given by the T23 free water region). The investigation revealed that both L. plantarum L1 and kefir-fermented milk exhibited pseudoplastic characteristics. However, the introduction of yeast and acetic acid bacteria had diverse impacts on their rheological properties and microstructure. The latter decomposes macromolecules such as proteins and fat into small peptides and small lipid particles. These particles fill the pores of the yogurt gel structure, making them smaller and form a dense network structure that closely combines water molecules.[Citation118]
Soy sauce
Biogenic amines constitute organic compounds present in fermented foods, originating from decarboxylation of amino acids or the amination and transamination of aldehydes and ketones facilitated by microbes.[Citation119] The excessive consumption of this metabolites can induce physiological and toxicological effects, potentially leading to poisoning. Consequently, it is crucial to minimize the biogenic amine content in fermented foods.[Citation119] Given these considerations, investigating the microbial composition and factors influencing the microbial profile of traditional soy sauce becomes of paramount importance. In a study, the impact of incorporating coriander during soy sauce fermentation was assessed, considering both the microflora and the levels of biogenic amines. The latter were determined by a quantitative 1H NMR analysis. The levels of biogenic amines were significantly lower (p < 0.05) in the coriander-infused soy sauce compared to the control sauce. These findings suggested that incorporating coriander during Korean soy sauce fermentation was beneficial, as it contributed to the reduction of both biogenic amine levels and the bacteria responsible for their formation.[Citation120]
Fermented vegetables
Food decomposition and deterioration will certainly accelerate because of the oxidation reaction. Under oxidation conditions, several hazardous by-products, such as reactive oxygen species, will be overproduced.[Citation121] As we have previously seen, fermented foods constitute a valuable source of antioxidants. The effects of oxidative stress can be improved by inhibition of key enzymes. Molecular docking serves as a valuable tool for screening potential enzyme inhibitors, offering a significant advantage in the initial stages of drug development. Investigating the interaction between small molecules and target enzymes is of high importance in the development of novel drugs. So, bioactive components in 5-year pickled radish were isolated and characterized, where NMR was used for identification, along with other separation and purification techniques. The identified structures included, α-linolenic acid, chaenomic acid A, β-sitosterol, 5-hydroxymethylfurfural, β-sitosterol-3-O-glucose glycosides, and 1-monopalmitin. These bioactive elements were commonly detected in numerous plants, but it was the first observation of α-linolenic acid in pickled radish. Molecular docking analysis indicated that β-sitosterol and β-sitosterol-3O-glucose glycosides displayed notable affinity for multiple crucial enzymes engaged in oxidation reactions.[Citation122]
Sourdough
A mixture of sourdough prepared using wheat germ and wholemeal semolina derived from the endosperm of wheat kernels was employed to enhance the sensory and nutritional characteristics of newly crafted pasta. The aim was to inhibit the oxidation of lipids and extend the product’s shelf life. In this case, NMR played an important role as a quality control tool during pasta storage to study the evolution of lipids over time. Three distinct formulations were created: an initial formulation utilized semolina, a second one incorporated raw wheat germ, wholemeal semolina, and semolina, while the final formulation consisted of semolina combined with sourdough. The investigation into lipid oxidation phenomena through 1H NMR revealed a greater occurrence of primary lipid oxidation in pasta with semolina (CP) compared to pasta containing semolina, wholemeal semolina, and raw germ (RGP) and pasta made with semolina and wholemeal semolina but with the addition of fermented wheat germ (SP). As a result, a hypothesized protective influence of wheat germ was suggested.[Citation123]
NMR applications in plant-based beverages
Surprisingly, the field of plant-based beverages has not been extensively studied by NMR techniques in recent years. Despite being an emerging alternative to dairy products and with a rise of an allergic population to dairy products or turning to emerging dieting trends like veganism, there is a consistent lack of studies studying either their functional properties when incorporated to different matrices, their metabolism products, the discriminating metabolites between them, or their origin and quality. NMR metabolomics and food authenticity were the focus of a few studies in this area.
A combination of a GC-MS with an 1H NMR metabolomics approach was used to detect food biomarkers in human urine from healthy volunteers after consumption of a soy-based drink, cow milk and cheese. The drink consisted of 90% soy drink and 10% added soy-based vegetable cream. Three food intake biomarkers of the soy-based drink were successfully identified by NMR metabolomics, namely, trigonelline, pyridoxine and trans-aconitate, while some other signals in the spectra required further efforts to be assigned. Nevertheless, the authors emphasized the need for evaluating the identified compounds in observational studies under uncontrolled conditions and within a broader dietary context. This evaluation is crucial to determine their applicability as biomarkers, considering their presence in various food matrices.[Citation124]
A fast method by a combination of 1H NMR and DOSY to detect food thickeners in one almond milk and one bio-oat drink with chocolate flavor (among other food matrices) was developed. Food thickeners are defined as simple polysaccharides (such as Arabic gum, guar gum, carboxy-methylcellulose, carrageenan, starch, and pectin) or proteins (such as gelatin) that when hydrated, generate a viscous liquid.[Citation125] The authors divided the results into 2 groups: food with detected and undetected thickeners with a comparison with the label declaration on their content. They highlighted that although thickeners are frequently used in food at low concentrations, the NMR approach allowed for their fast identification at approximately concentrations higher than 0.5% − 1% (w/w) in several premixtures and food. Within the group where thickeners were detected, the almond milk was present, where the signals at 5.08 (H-1 of galacturonic acid) and 4.96 ppm (H-1 from galactose) marked the presence of pectin. The bio-oat drink was included in the second group of products where thickeners could not be detected by this novel method. The main factors addressed by the author regarding these results were concentration of the ingredients in the matrix, concentration of the thickener, solubility in D2O, and temperature since the analysis of complex carbohydrates at low temperatures produced a broadening of signals.[Citation126]
Conclusions. Challenges and opportunities in the area
A significant knowledge gap exists around plant-based beverages analysis by NMR. Our careful review of the literature over the recent years reveals that the potential applications of NMR in this area have been relatively unexplored compared to other well-established areas of NMR research. This knowledge gap presents a compelling opportunity for innovation and the development of novel alternatives. In this article, we emphasize the need for further exploration and innovation at the intersection of plant-based beverages industry and NMR spectroscopy, highlighting the potential for new findings and insights in this underdeveloped field.
Several concepts might be investigated, particularly in the context of quantitative research, nutritional content analyses, authenticity testing, mixture analysis, traceability studies, quality assessment, and determining the level of processing, to fill this gap in our understanding of plant-based product science and NMR spectroscopy given the inherent advantages of the technique that were previously mentioned. This includes, but is not limited to, the following: highlighting the use of NMR spectroscopy for quantitative studies of nutritional components in plant-based foods, including carbohydrates, lipids, proteins, and bioactive substances. This can help us gain a deeper insight into the nutritional benefits of these foods; developing NMR-based methods to authenticate the geographic origin and quality of plant-based beverages. This is especially relevant in combating food fraud, where substitution or mixing of ingredients with others of poor quality is increasingly occurring to ensure that consumers receive the genuine plant-based products they expect for the special price they pay; identifying the components of mixtures or a “100% pure” presumed product to avoid fraud; utilizing NMR to trace the journey of plant-based ingredients from their source to the final product and their metabolism in the human body. This can enable new rigorous and faster quality control strategies and verify the integrity of plant-based supply chains, essential for consumers and regulatory agencies; employing NMR to assess the degree of processing in plant-based products, aligning with the trend towards minimally processed foods. This would help meet consumer demands for healthier, less-processed alternatives by quantifying the impact of processing on the metabolite profile of these products as it has been carried out for other food matrices; developing new functional foods with the addition of different nutraceutical ingredients such as seeds (whole or their defatted flour), fruits (juice, fresh pulp or powdered peel) and studying changes in NMR signals of specific compounds such as vitamins, carbohydrates or antioxidants; developing new products tailored for consumers with chronic diseases such as diabetes (sugar-free beverages) and controlling the level of specific metabolites (sugar concentration) in new or existing products in the market; investigating the impact of gastrointestinal in vitro digestion by NMR metabolomics (with optional contribution of other analytical techniques) to give valuable information about their role in the gut microbiota and their potential contribution as prebiotics for bacteria; developing tools for the fast certification of the BIO claim observed in most products of this area including the markers that differentiate BIO plant-based products from conventional plant-based products and whether they confer significant benefits in nutritional terms.
The area of fermented foods has been extensively studied using NMR and covers a wide range of applications, as evidenced by the large number of articles in recent years. However, there are still some underexplored sub-areas. Although we have observed that EPSs have been the primary focus of a considerable number of publications, there is a lack of studies regarding their applications in other matrices. Their water-solubility could be harnessed to develop innovative functional products such as fruit juice, flavored water drinks, plant-based beverages, yogurt, ice-cream, and creamy desserts where they could potentially have a probiotic effect. They could also be used to enhance the viscosity of sweet desserts without the need for additional fat, enabling the formulation of fat-free or calorie-reduced products. Additionally, they could be incorporated into gluten-free products like muffins, bread, puff pastry dough, and pasta to improve their sensory quality and rheological properties. However, we believe that the primary challenge in these applications lies in the matrix effect. This is since nearly all food matrices contain sugars or carbohydrates, which can complicate their identification in a complex matrix using NMR techniques, even after matrix pre-treatment. Another challenge in this area is the use of EPSs in novel products. Their properties have been extensively studied and all have proposed as candidates for food development, but no studies have incorporated them in another matrix to study their functional effects in a new matrix. The main difficulty lies in the matrix effect and the overlapping of signals, but we have seen that NMR offers plenty of advantages to overcome this issue. Regarding the sensory impact of fermented products, more studies could be dedicated to finding ways of introducing these products to the consumers either by incorporating them inside another matrix (by fortification or partial replacement of the ingredients), mixing them with other fermented or non-fermented foods or diluting them with water in the case of fermented juices without or minimally altering their functional properties. This could also be extended to finding healthier ways of conservation of different foods, given the antibacterial properties observed in these matrices.
Abbreviations
2D NMR | = | two-dimensional NMR |
1H-13C-HSQC | = | heteronuclear 1H,13C single quantum coherence |
COSY | = | correlation spectroscopy |
DOSY | = | diffusion ordered spectroscopy |
EPS | = | Exopolysaccharide |
HoPs | = | Homopolysaccharide |
HePs | = | Heteropolysaccharide |
FT-IR | = | Fourier transform infrared spectroscopy |
GC-MS | = | gas chromatography coupled to mass spectrometry |
HMBC | = | heteronuclear multiple bond correlation |
LAB | = | Lactic acid bacteria |
LF-NMR: | = | Low-field NMR |
NOESY | = | nuclear Overhauser Effect spectroscopy |
TOCSY | = | total correlation spectroscopy |
Disclaimer
This study was carried out within the ON Foods – Research and innovation network on food and nutrition Sustainability, Safety and Security – Working ON Foods” project and received funding from the European Union Next-GenerationEU (PIANO NAZIONALE DI RIPRESA E RESILIENZA (PNRR) – Mission 4 Component 2 Investment 1.3 – D.D. 1550 11/10/2022, PE0000003, CUP B43C22000770006). This manuscript reflects only the authors’ views and opinions, neither the European Union nor the European Commission can be considered responsible for them.
Acknowledgments
The authors acknowledge the support of “ON Foods - Research and innovation network on food and nutrition Sustainability, Safety and Security - Working ON Foods” to Fondazione Edmund Mach, funded by the Italian Ministry of University and Research, PNRR, Mission 4 Component 2 Investment 1.3 - Project code PE00000003, CUP B43C22000770006. This study has also received funding from the Autonomous Province of Trento, with EU co-financing (FRUITOMICS, FESR 2014–2020 Program of the Autonomous Province of Trento, Italy).
Disclosure statement
No potential conflict of interest was reported by the author(s).
Additional information
Funding
References
- Enes, C. C.; de Camargo, C. M.; Justino, M. I. C. Ultra-Processed Food Consumption and Obesity in Adolescents. Revista de Nutrição. 2019, 32, e180170. DOI: 10.1590/1678-9865201932e180170.
- Juul, F.; Martinez-Steele, E.; Parekh, N.; Monteiro, C. A.; Chang, V. W. Ultra-Processed Food Consumption and Excess Weight Among US Adults. Br. J. Nutr. 2018, 120(1), 90–100. DOI: 10.1017/S0007114518001046.
- Huybrechts, I.; Rauber, F.; Geneviève, N.; Casagrande, C.; Kliemann, N.; Wedekind, R.; Biessy, C.; Scalbert, A.; Touvier, M.; Aleksandrova, K., et al. Characterization of the Degree of Food Processing in the European Prospective Investigation into Cancer and Nutrition: Application of the Nova Classification and Validation Using Selected Biomarkers of Food Processing. Front. Nutr. 2022, 9, 1035580. DOI: 10.3389/fnut.2022.1035580.
- Willett, W.; Rockström, J.; Loken, B.; Springmann, M.; Lang, T.; Vermeulen, S.; Garnett, T.; Tilman, D.; DeClerck, F.; Wood, A., et al. Food in the Anthropocene: The EAT–Lancet Commission on Healthy Diets from Sustainable Food Systems. Lancet. 2019, 393 (10170), 447–492. DOI: 10.1016/S0140-6736(18)31788-4.
- Jarmul, S.; Dangour, A. D.; Green, R.; Liew, Z.; Haines, A.; Scheelbeek, P. F. Climate Change Mitigation Through Dietary Change: A Systematic Review of Empirical and Modelling Studies on the Environmental Footprints and Health Effects of ‘Sustainable diets’. Environ. Res. Lett. 2020, 15(12), 123014. DOI: 10.1088/1748-9326/abc2f7.
- Marco, M. L.; Heeney, D.; Binda, S.; Cifelli, C. J.; Cotter, P. D.; Foligné, B.; Gänzle, M.; Kort, R.; Pasin, G.; Pihlanto, A., et al. Health Benefits of Fermented Foods: Microbiota and Beyond. Curr. Opin. Biotechnol. 2017, 44, 94–102. DOI: 10.1016/j.copbio.2016.11.010.
- Bintsis, T. Lactic Acid Bacteria as Starter Cultures: An Update in Their Metabolism and Genetics. AIMS Microbiol. 2018, 4(4), 665–684. DOI: 10.3934/microbiol.2018.4.665.
- Bibra, M.; Krishnaraj, R. N.; Sani, R. K. Fermentation Strategies in the Food and Beverage Industry. In Biomolecular Engineering Solutions for Renewable Specialty Chemicals, Krishnaraj, R.N. and Sani, R.K; Eds.; John Wiley and Sons Ltd., 2021; pp. 141–164. DOI: 10.1002/9781119771951.ch5.
- Jayachandran, M.; Xu, B. An Insight into the Health Benefits of Fermented Soy Products. Food Chem. 2019, 271, 362–371. DOI: 10.1016/j.foodchem.2018.07.158.
- Qiao, Y.; Zhang, K.; Zhang, Z.; Zhang, C.; Sun, Y.; Feng, Z. Fermented Soybean Foods: A Review of Their Functional Components, Mechanism of Action and Factors Influencing Their Health Benefits. Food Res. Int. 2022, 158, 111575. DOI: 10.1016/j.foodres.2022.111575.
- Vaikma, H.; Kaleda, A.; Rosend, J.; Rosenvald, S. Market Mapping of Plant-Based Milk Alternatives by Using Sensory (RATA) and GC Analysis. Fut. Foods. 2021, 4, 100049. DOI: 10.1016/j.fufo.2021.100049.
- Munekata, P. E. S.; Domínguez, R.; Budaraju, S.; Roselló-Soto, E.; Barba, F. J.; Mallikarjunan, K.; Roohinejad, S.; Lorenzo, J. M. Effect of Innovative Food Processing Technologies on the Physicochemical and Nutritional Properties and Quality of Non-Dairy Plant-Based Beverages. Foods. 2020, 9(3), 288. DOI: 10.3390/foods9030288.
- Zujko, M. E.; Witkowska, A. M. Antioxidant Potential and Polyphenol Content of Beverages, Chocolates, Nuts, and Seeds. Int. J. Food Prop. 2014, 17(1), 86–92. DOI: 10.1080/10942912.2011.614984.
- Böhme, K.; Calo-Mata, P.; Barros-Velázquez, J.; Ortea, I. Recent Applications of Omics-Based Technologies to Main Topics in Food Authentication. TrAC Trends Anal. Chem. 2019, 110, 221–232. DOI: 10.1016/j.trac.2018.11.005.
- Mielko, K. A.; Pudełko-Malik, N.; Tarczewska, A.; Młynarz, P. NMR Spectroscopy As a “Green Analytical method” in Metabolomics and Proteomics Studies. Sustain. Chem. Pharm. 2021, 22, 100474. DOI: 10.1016/j.scp.2021.100474.
- Emwas, A.-H.; Roy, R.; McKay, R. T.; Tenori, L.; Saccenti, E.; Gowda, G. A. N.; Raftery, D.; Alahmari, F.; Jaremko, L.; Jaremko, M., et al. NMR Spectroscopy for Metabolomics Research. Metabolites. 2019, 9 (7), 7. DOI: 10.3390/metabo9070123.
- Sadeghi, A.; Ebrahimi, M.; Hajinia, F.; Kharazmi, M. S.; Jafari, S. M. FoodOmics as a Promising Strategy to Study the Effects of Sourdough on Human Health and Nutrition, as Well as Product Quality and Safety; Back to the Future. Trends Food Sci. Technol. 2023, 136, 24–47. DOI: 10.1016/j.tifs.2023.03.026.
- Zhan, Q.; Thakur, K.; Zhang, W.-W.; Feng, J.-Y.; Zhang, J.-G.; Khan, M. R.; Wei, Z.-J. Metabolic Changes and Isoflavone Biotransformation in Natto for Improved Nutritional Distribution and Bioavailability. Food Biosci. 2024, 59, 103937. DOI: 10.1016/j.fbio.2024.103937.
- Van Kerrebroeck, S.; Comasio, A.; Harth, H.; De Vuyst, L. Impact of Starter Culture, Ingredients, and Flour Type on Sourdough Bread Volatiles as Monitored by Selected Ion Flow Tube-Mass Spectrometry. Food. Res. Int. 2018, 106, 254–262. DOI: 10.1016/j.foodres.2017.12.068.
- Huang, Y.-P.; Paviani, B.; Fukagawa, N. K.; Phillips, K. M.; Barile, D. Comprehensive Oligosaccharide Profiling of Commercial Almond Milk, Soy Milk, and Soy Flour. Food Chem. 2023, 409, 135267. DOI: 10.1016/j.foodchem.2022.135267.
- Valerón, N. R.; Mak, T.; Jahn, L. J.; Arboleya, J. C.; Sörensen, P. M. Characterization of Kokumi γ-Glutamyl Peptides and Volatile Aroma Compounds in Alternative Grain Miso Fermentations. LWT - Food Sci. Technol. 2023, 188, 115356. DOI: 10.1016/j.lwt.2023.115356.
- Nwodo, U. U.; Green, E.; Okoh, A. I. Bacterial Exopolysaccharides: Functionality and Prospects. Int. J. Mol. Sci. 2012, 13(11), 14002–14015. DOI: 10.3390/ijms131114002.
- De Vuyst, L.; de Vin, F.; Vaningelgem, F.; Degeest, B. Recent Developments in the Biosynthesis and Applications of Heteropolysaccharides from Lactic Acid Bacteria. Int. Dairy. J. 2001, 11(9), 687–707. DOI: 10.1016/S0958-6946(01)00114-5.
- Kaur, N.; Dey, P. Bacterial Exopolysaccharides as Emerging Bioactive Macromolecules: From Fundamentals to Applications. Res. Microbiol. 2023, 174(4), 104024. DOI: 10.1016/j.resmic.2022.104024.
- Angelin, J.; Kavitha, M. Exopolysaccharides from Probiotic Bacteria and Their Health Potential. Int. J. Biol. Macromol. 2020, 162, 853–865. DOI: 10.1016/j.ijbiomac.2020.06.190.
- Shukla, A.; Krina, M.; Jignesh, P.; Jaimin, P.; Meenu, S. Depicting the Exemplary Knowledge of Microbial Exopolysaccharides in a Nutshell. Eur. Polym. J. 2019, 119, 298–310. DOI: 10.1016/j.eurpolymj.2019.07.044.
- Bengoa, A. A.; Iraporda, C.; Acurcio, L. B.; de Cicco Sandes, S. H.; Costa, K.; Moreira Guimarães, G.; Esteves Arantes, R. M.; Neumann, E.; Cantini Nunes, Á.; Nicoli, J. R., et al. Physicochemical, Immunomodulatory and Safety Aspects of Milks Fermented with Lactobacillus Paracasei Isolated from Kefir. Food Res. Int. 2019, 123, 48–55. DOI: 10.1016/j.foodres.2019.04.041.
- Kim, D.-H.; Jeong, D.; Kang, I.-B.; Lim, H.-W.; Cho, Y.; Seo, K.-H. Modulation of the Intestinal Microbiota of Dogs by Kefir as a Functional Dairy Product. J. Dairy. Sci. 2019, 102(5), 3903–3911. DOI: 10.3168/jds.2018-15639.
- Riaz Rajoka, M. S.; Mehwish, H. M.; Fang, H.; Padhiar, A. A.; Zeng, X.; Khurshid, M.; He, Z.; Zhao, L. Characterization and Anti-Tumor Activity of Exopolysaccharide Produced by Lactobacillus Kefiri Isolated from Chinese Kefir Grains. J. Funct. Foods. 2019, 63, 103588. DOI: 10.1016/j.jff.2019.103588.
- Rahman, A.; Choudhary, M. I.; Wahab, A. Important 2D NMR Experiments. In Solving Problems with NMR Spectroscopy, 2nd ed.; Academic Press: United States, 2016; pp. 265–386. DOI: 10.1016/C2012-0-06253-9.
- Xiao, L.; Xu, D.; Tang, N.; Rui, X.; Zhang, Q.; Chen, X.; Dong, M.; Li, W. Biosynthesis of Exopolysaccharide and Structural Characterization by Lacticaseibacillus paracasei ZY-1 Isolated from Tibetan Kefir. Food Chem. Mol. Sci. 2021, 3, 100054. DOI: 10.1016/j.fochms.2021.100054.
- Guzel-Seydim,Z. B.; Gökırmaklı, C.; Greene, A. K. A Comparison of Milk Kefir and Water Kefir: Physical, Chemical, Microbiological and Functional Properties. Trends Food Sci. Technol. 2021, 113, 42–53. DOI: 10.1016/j.tifs.2021.04.041.
- Fels, L.; Jakob, F.; Vogel, R. F.; Wefers, D. Structural Characterization of the Exopolysaccharides from Water Kefir. Carbohydr. Polym. 2018, 189, 296–303. DOI: 10.1016/j.carbpol.2018.02.037.
- De Vuyst, L.; Van Kerrebroeck, S.; Harth, H.; Huys, G.; Daniel, H.-M.; Weckx, S. Microbial Ecology of Sourdough Fermentations: Diverse or Uniform? Food Microbiol. 2014, 37, 11–29. DOI: 10.1016/j.fm.2013.06.002.
- Brown, A. J. X. On an Acetic Ferment Which Forms Cellulose. J. Chem. Soc. Trans. 1886, 49, 432–439. DOI: 10.1039/CT8864900432.
- Blanco, A.; Monte, M. C.; Campano, C.; Balea, A.; Merayo, N.; Negro, C. Nanocellulose for Industrial Use: Cellulose Nanofibers (CNF), Cellulose Nanocrystals (CNC), and Bacterial Cellulose (BC). In Handbook of Nanomaterials for Industrial Applications. Micro and Nano Technologies, 1st ed.; Hussain, C.M., Ed.; Elsevier: The Netherlands, 2018; pp. 74–126.
- Reif, B.; Ashbrook, S. E.; Emsley, L.; Hong, M. Solid-State NMR Spectroscopy. Nat. Revs. Methods Primers. 2021, 1(1), 2. DOI: 10.1038/s43586-020-00002-1.
- Gupte, Y.; Kulkarni, A.; Raut, B.; Sarkar, P.; Choudhury, R.; Chawande, A.; Kumar, G. R. K.; Bhadra, B.; Satapathy, A.; Das, G., et al. Characterization of Nanocellulose Production by Strains of Komagataeibacter sp. Isolated from Organic Waste and Kombucha. Carbohyd. Polym. 2021, 266, 118176. DOI: 10.1016/j.carbpol.2021.118176.
- Feng, Y.; Zhang, M.; Mujumdar, A. S.; Gao, Z. Recent Research Process of Fermented Plant Extract: A Review. Trends Food Sci. Technol. 2017, 65, 40–48. DOI: 10.1016/j.tifs.2017.04.006.
- Dai, J.; Sha, R.; Wang, Z.; Cui, Y.; Fang, S.; Mao, J. Edible Plant Jiaosu: Manufacturing, Bioactive Compounds, Potential Health Benefits, and Safety Aspects. J. Sci. Food Agric. 2020, 100(15), 5313–5323. DOI: 10.1002/jsfa.10518.
- de Sá, L. Z. M.; Castro, P. F.; Lino, F. M.; Bernardes, M. J.; Viegas, J. C.; Dinis, T. C.; Santana, M. J.; Romao, W.; Vaz, B. G.; Lião, L. M., et al. Antioxidant Potential and Vasodilatory Activity of Fermented Beverages of Jabuticaba Berry (Myrciaria Jaboticaba). J. Funct. Foods. 2014, 8, 169–179. DOI: 10.1016/j.jff.2014.03.009.
- Feng, F.; Zhou, Q.; Yang, Y.; Zhao, F.; Du, R.; Han, Y.; Xiao, H.; Zhou, Z. Characterization of Highly Branched Dextran Produced by Leuconostoc citreum B-2 from Pineapple Fermented Product. Int. J. Biol. Macromol. 2018, 113, 45–50. DOI: 10.1016/j.ijbiomac.2018.02.119.
- Ye, G.; Chen, Y.; Wang, C.; Yang, R.; Bin, X. Purification and Characterization of Exopolysaccharide Produced by Weissella Cibaria YB-1 from Pickle Chinese Cabbage. Int. J. Biol. Macromol. 2018, 120(A), 1315–1321. DOI: 10.1016/j.ijbiomac.2018.09.019.
- Liu, J.; Wang, X.; Pu, H.; Liu, S.; Kan, J.; Jin, C. Recent Advances in Endophytic Exopolysaccharides: Production, Structural Characterization, Physiological Role and Biological Activity. Carbohyd. Polym. 2017, 157, 1113–1124. DOI: 10.1016/j.carbpol.2016.10.084.
- Hu, X.; Pang, X.; Wang, P. G.; Chen, M. Isolation and Characterization of an Antioxidant Exopolysaccharide Produced by Bacillus sp. S-1 from Sichuan Pickles. Carbohyd. Polym. 2019, 204, 9–16. DOI: 10.1016/j.carbpol.2018.09.069.
- Yang, X.; Hu, W.; Xiu, Z.; Jiang, A.; Yang, X.; Ji, Y.; Guan, Y.; Feng, K. Microbial Dynamics and Volatilome Profiles During the Fermentation of Chinese Northeast Sauerkraut by Leuconostoc mesenteroides ORC 2 and Lactobacillus Plantarum HBUAS 51041 Under Different Salt Concentrations. Food Res. Int. 2020, 130, 108926. DOI: 10.1016/j.foodres.2019.108926.
- Du, R.; Yu, L.; Yu, N.; Ping, W.; Song, G.; Ge, J. Characterization of Exopolysaccharide Produced by Levilactobacillus brevis HDE-9 and Evaluation of Its Potential Use in Dairy Products. Int. J. Biol. Macromol. 2022, 217, 303–311. DOI: 10.1016/j.ijbiomac.2022.07.057.
- Xu, X.; Peng, Q.; Zhang, Y.; Tian, D.; Zhang, P.; Huang, Y.; Ma, L.; Qiao, Y.; Shi, B. A Novel Exopolysaccharide Produced by Lactobacillus Coryniformis NA-3 Exhibits Antioxidant and Biofilm-Inhibiting Properties in vitro. Food Nutr. Res. 2020, 64, 3744. DOI: 10.29219/fnr.v64.3744.
- Yu, L.; Ye, G.; Qi, X.; Yang, Y.; Zhou, B.; Zhang, Y.; Du, R.; Ge, J.; Ping, W. Purification, Characterization and Probiotic Proliferation Effect of Exopolysaccharides Produced by Lactiplantibacillus plantarum HDC-01 Isolated from Sauerkraut. Front. Microbiol. 2023, 14, 1210302. DOI: 10.3389/fmicb.2023.1210302.
- Curiel, J. A.; Coda, R.; Centomani, I.; Summo, C.; Gobbetti, M.; Rizzello, C. G. Exploitation of the Nutritional and Functional Characteristics of Traditional Italian Legumes: The Potential of Sourdough Fermentation. Int. J. Food Microbiol. 2015, 196, 51–61. DOI: 10.1016/j.ijfoodmicro.2014.11.032.
- Manini, F.; Casiraghi, M. C.; Poutanen, K.; Brasca, M.; Erba, D.; Plumed-Ferrer, C. Characterization of Lactic Acid Bacteria Isolated from Wheat Bran Sourdough. LWT - Food Sci. Technol. 2016, 66, 275–283. DOI: 10.1016/j.lwt.2015.10.045.
- Campo, E.; Del Arco, L.; Urtasun, L.; Oria, R.; Ferrer-Mairal, A. Impact of Sourdough on Sensory Properties and consumers’ Preference of Gluten-Free Breads Enriched with Teff Flour. J. Cereal Sci. 2016, 67, 75–82. DOI: 10.1016/j.jcs.2015.09.010.
- Bounaix, M. S.; Gabriel, V.; Morel, S.; Robert, H.; Rabier, P.; Remaud-Simeon, M.; Gabriel, B.; Fontagné-Faucher, C. Biodiversity of Exopolysaccharides Produced from Sucrose by Sourdough Lactic Acid Bacteria. J. Agric. Food Chem. 2009, 57(22), 10889–10897. DOI: 10.1021/jf902068t.
- Dertli, E.; Mayer, M. J.; Narbad, A. Impact of the Exopolysaccharide Layer on Biofilms, Adhesion and Resistance to Stress in Lactobacillus Johnsonii FI9785. BMC Microbiol. 2015, 15(1), 8. DOI: 10.1186/s12866-015-0347-2.
- Taylan, O.; Yilmaz, M. T.; Dertli, E. Partial Characterization of a Levan Type Exopolysaccharide (EPS) Produced by Leuconostoc mesenteroides Showing Immunostimulatory and Antioxidant Activities. Int. J. Biol. Macromol. 2019, 136, 436–444. DOI: 10.1016/j.ijbiomac.2019.06.078.
- Aburas, H.; İspirli, H.; Taylan, O.; Yilmaz, M. T.; Dertli, E. Structural and Physicochemical Characterisation and Antioxidant Activity of an α-D-Glucan Produced by Sourdough Isolate Weissella Cibaria MED17. Int. J. Biol. Macromol. 2020, 161, 648–655. DOI: 10.1016/j.ijbiomac.2020.06.030.
- Ahmed, R. Z.; Siddiqui, K.; Arman, M.; Ahmed, N. Characterization of High Molecular Weight Dextran Produced by Weissella Cibaria CMGDEX3. Carbohyd. Polym. 2012, 90(1), 441–446. DOI: 10.1016/j.carbpol.2012.05.063.
- Vermeulen, N.; Gänzle, M. G.; Vogel, R. F. Glutamine Deamidation by Cereal‐Associated Lactic Acid Bacteria. J. Appl. Microbiol. 2007, 103(4), 1197–1205. DOI: 10.1111/j.1365-2672.2007.03333.x.
- Yazar, G.; Tavman, Ş. Functional and Technological Aspects of Sourdough Fermentation with Lactobacillus Sanfranciscensis. Food Eng. Rev. 2012, 4(3), 171–190. DOI: 10.1007/s12393-012-9052-1.
- De Angelis, M.; Gallo, G.; Corbo, M. R.; McSweeney, P. L.; Faccia, M.; Giovine, M.; Gobbetti, M. Phytase Activity in Sourdough Lactic Acid Bacteria: Purification and Characterization of a Phytase from Lactobacillus Sanfranciscensis CB1. Int. J. Food Microbiol. 2003, 87(3), 259–270. DOI: 10.1016/S0168-1605(03)00072-2.
- Zhang, G.; Zhang, W.; Sun, L.; Sadiq, F. A.; Yang, Y.; Gao, J.; Sang, Y. Preparation Screening, Production Optimization and Characterization of Exopolysaccharides Produced by Lactobacillus Sanfranciscensis Ls-1001 Isolated from Chinese Traditional Sourdough. Int. J. Biol. Macromol. 2019, 139, 1295–1303. DOI: 10.1016/j.ijbiomac.2019.08.077.
- Llamas-Arriba, M. G.; Hernández-Alcántara, A. M.; Mohedano, M. L.; Chiva, R.; Celador-Lera, L.; Velázquez, E.; Prieto, A.; DueñDueñAs, M. T.; Tamame, M.; López, P. Lactic Acid Bacteria Isolated from Fermented Doughs in Spain Produce Dextrans and Riboflavin. Foods. 2021, 10(9), 2004. DOI: 10.3390/foods10092004.
- Nakata, H.; Imamura, Y.; Saha, S.; Lobo, R. E.; Kitahara, S.; Araki, S.; Tomokiyo, M.; Namai, F.; Hiramitsu, M.; Inoue, T., et al. Partial Characterization and Immunomodulatory Effects of Exopolysaccharides from Streptococcus Thermophilus SBC8781 During Soy Milk and Cow Milk Fermentation. Foods. 2023, 12 (12), 2374. DOI: 10.3390/foods12122374.
- Liu, J.; Wang, X.; Pu, H.; Liu, S.; Kan, J.; Jin, C. Recent Advances in Endophytic Exopolysaccharides: Production, Structural Characterization, Physiological Role and Biological Activity. Carbohydr. Polym. 2017, 157, 1113–1124. DOI: 10.1016/j.carbpol.2016.10.084.
- Yang, Y.; Feng, F.; Zhou, Q.; Zhao, F.; Du, R.; Zhou, Z.; Han, Y. Isolation, Purification and Characterization of Exopolysaccharide Produced by Leuconostoc Pseudomesenteroides YF32 from Soybean Paste. Int. J. Biol. Macromol. 2018, 114, 529–535. DOI: 10.1016/j.ijbiomac.2018.03.162.
- Lay, J. O.; Liyanage, R.; Borgmann, S.; Wilkins, C. L. Problems with the “Omics. Trends Anal. Chem. 2006, 25(11), 1046–1056. DOI: 10.1016/j.trac.2006.10.007.
- Rizo, J.; Guillén, D.; Farrés, A.; Díaz-Ruiz, G.; Sánchez, S.; Wacher, C.; Rodríguez-Sanoja, R. Omics in Traditional Vegetable Fermented Foods and Beverages. Crit. Rev. Food Sci. 2020, 60(5), 791–809. DOI: 10.1080/10408398.2018.1551189.
- Lacalle-Bergeron, L.; Izquierdo-Sandoval, D.; Sancho, J. V.; López, F. J.; Hernández, F.; Portolés, T. Chromatography Hyphenated to High Resolution Mass Spectrometry in Untargeted Metabolomics for Investigation of Food (Bio)markers. Trends Anal. Chem. 2021, 135, 116161. DOI: 10.1016/j.trac.2020.116161.
- Begou, O.; Gika, H. G.; Wilson, I. D.; Theodoridis, G. Hyphenated MS-Based Targeted Approaches in Metabolomics. Analyst. 2017, 142(17), 3079–3100. DOI: 10.1039/C7AN00812K.
- León, C.; Cifuentes, A.; Valdés, A. Chapter Twenty-Two - Foodomics Applications. Compr. Anal. Chem. 2018, 82, 643–685. DOI: 10.1016/bs.coac.2018.06.008.
- Pezzatti, J.; Boccard, J.; Codesido, S.; Gagnebin, Y.; Joshi, A.; Picard, D.; González-Ruiz, V.; Rudaz, S. Implementation of Liquid Chromatography–High Resolution Mass Spectrometry Methods for Untargeted Metabolomic Analyses of Biological Samples: A Tutorial. Anal. Chim. 2020, 1105, 28–44. DOI: 10.1016/j.aca.2019.12.062.
- Liu, X.; Locasale, J. W. Metabolomics: A Primer. Trends. Biochem. Sci. 2017, 42(4), 274–284. DOI: 10.1016/j.tibs.2017.01.004.
- Liland, K. H. Multivariate Methods in Metabolomics – from Pre-Processing to Dimension Reduction and Statistical Analysis. Trends Anal. Chem. 2011, 30(6), 827–841. DOI: 10.1016/j.trac.2011.02.007.
- Lindon, J. C.; Nicholson, J. K. Spectroscopic and Statistical Techniques for Information Recovery in Metabonomics and Metabolomics. Annu. Rev. Anal. Chem. 2008, 1(1), 45–69. DOI: 10.1146/annurev.anchem.1.031207.113026.
- Sun, J.; Xia, Y. Pretreating and Normalizing Metabolomics Data for Statistical Analysis. Genes Dis. 2023, 11(3), 100979. DOI: 10.1016/j.gendis.2023.04.018.
- Vignoli, A.; Ghini, V.; Meoni, G.; Licari, C.; Takis, P. G.; Tenori, L.; Turano, P.; Luchinat, C. High-Throughput Metabolomics by 1D NMR. Angew. Chem. Int. Ed. 2019, 58(4), 968–994. DOI: 10.1002/anie.201804736.
- Abadl, M. M. T.; Marzlan, A. A.; Sulaiman, R.; Abas, F.; Meor Hussin, A. S. Optimization of Coconut Milk Kefir Beverage by RSM and Screening of Its Metabolites and Peptides. Fermentation. 2023, 9(5), 430–435. DOI: 10.3390/fermentation9050430.
- Qadi, W. S. M.; Mediani, A.; Benchoula, K.; Wong, E. H.; Misnan, N. M.; Sani, N. A. Characterization of Physicochemical, Biological, and Chemical Changes Associated with Coconut Milk Fermentation and Correlation Revealed by 1H NMR-Based Metabolomics. Foods. 2023, 12(10), 1971. DOI: 10.3390/foods12101971.
- Gao, Y. X.; Xu, B.; Fan, H. R.; Zhang, M. R.; Zhang, L. J.; Lu, C.; Zhang, N. N.; Fan, B.; Wang, F. Z.; Li, S. 1H NMR-Based Chemometric Metabolomics Characterization of Soymilk Fermented by Bacillus subtilis BSNK-5. Food. Res. Int. 2020, 138, 109686. DOI: 10.1016/j.foodres.2020.109686.
- Park, K. Y.; Jeong, J. K.; Lee, Y. E.; Daily, J., III. Health Benefits of Kimchi (Korean Fermented Vegetables) As a Probiotic Food. J. Med. Food. 2014, 17(1), 6–20. DOI: 10.1089/jmf.2013.3083.
- Koo, O. K.; Lee, S. J.; Chung, K. R.; Jang, D. J.; Yang, H. J.; Kwon, D. Y. Korean Traditional Fermented Fish Products: Jeotgal. J. Ethn. Foods. 2016, 3(2), 107–116. DOI: 10.1016/j.jef.2016.06.004.
- Jung, M. Y.; Kim, T.-W.; Lee, C.; Kim, J. Y.; Song, H. S.; Kim, Y. B.; Ahn, S. W.; Kim, J. S.; Roh, S. W.; Lee, S. H. Role of Jeotgal, a Korean Traditional Fermented Fish Sauce, in Microbial Dynamics and Metabolite Profiles During Kimchi Fermentation. Food Chem. 2018, 265, 135–143. DOI: 10.1016/j.foodchem.2018.05.093.
- Seo, H.; Bae, J.-H.; Kim, G.; Kim, S.-A.; Ryu, B. H.; Han, N. S. Suitability Analysis of 17 Probiotic Type Strains of Lactic Acid Bacteria as Starter for Kimchi Fermentation. Foods. 2021, 10(6), 1435. DOI: 10.3390/foods10061435.
- Song, H. S.; Lee, S. H.; Ahn, S. W.; Kim, J. Y.; Rhee, J.-K.; Roh, S. W. Effects of the Main Ingredients of the Fermented Food, Kimchi, on Bacterial Composition and Metabolite Profile. Food. Res. Int. 2021, 149, 110668. DOI: 10.1016/j.foodres.2021.110668.
- Tomita, S.; Nakamura, T.; Okada, S. NMR- and GC/MS-Based Metabolomic Characterization of Sunki, an Unsalted Fermented Pickle of Turnip Leaves. Food Chem. 2018, 258, 25–34. DOI: 10.1016/j.foodchem.2018.03.038.
- Tomita, S.; Watanabe, J.; Kuribayashi, T.; Tanaka, S.; Kawahara, T. Metabolomic Evaluation of Different Starter Culture Effects on Water-Soluble and Volatile Compound Profiles in Nozawana Pickle Fermentation. Food Chem. Mol. Sci. 2021, 2, 100019. DOI: 10.1016/j.fochms.2021.100019.
- Gaudioso, G.; Weil, T.; Marzorati, G.; Solovyev, P.; Bontempo, L.; Franciosi, E.; Bertoldi, L.; Pedrolli, C.; Tuohy, K. M.; Fava, F. Microbial and Metabolic Characterization of Organic Artisanal Sauerkraut Fermentation and Study of Gut Health-Promoting Properties of Sauerkraut Brine. Front. Microbiol. 2022, 13, 929738. DOI: 10.3389/fmicb.2022.929738.
- Barbosa, J.; Teixeira, P. Development of Probiotic Fruit Juice Powders by Spray-Drying: A Review. Food Rev. Int. 2017, 33(4), 335–358. DOI: 10.1080/87559129.2016.1175016.
- Agatha, R.; Maryati, Y.; Susilowati, A.; Aspiyanto, A.; Devi, A. F.; Mulyani, H.; Budiari, S.; Filailla, E.; Rahmawati, D.; Artanti, N. Effect of Type and Concentration of Encapsulating Agents on Physicochemical, Phytochemical, and Antioxidant Properties of Red Dragon Fruit Kombucha Powdered Beverage. Indones. J. Chem. 2016, 23(1), 7–15. DOI: 10.14203/inajac.v23i1.474.
- Mohsin, A. Z.; Mat nor, N. A.; Muhialdin, B. J.; Mohd Roby, B. H.; Abadl, M. M.; Marzlan, A. A.; Hussain, N.; Meor Hussin, A. S. The Effects of Encapsulation Process Involving Arabic Gum on the Metabolites, Antioxidant and Antibacterial Activity of Kombucha (Fermented Sugared Tea). Food Hydrocoll. Health. 2022, 2, 100072. DOI: 10.1016/j.fhfh.2022.100072.
- Pauzi, N.; Man, S.; Nawawi, M. S. A. M.; Abu-Hussin, M. F. Ethanol Standard in Halal Dietary Product Among Southeast Asian Halal Governing Bodies. Trends Food Sci. Technol. 2019, 86, 375–380. DOI: 10.1016/j.tifs.2019.02.042.
- Landis, E. A.; Fogarty, E.; Edwards, J. C.; Popa, O.; Eren, A. M.; Wolfe, B. E.; Traxler, M. F. Microbial Diversity and Interaction Specificity in Kombucha Tea Fermentations. mSystems. 2022, 7(3), e00157–22. DOI: 10.1128/msystems.00157-22.
- Harrison, K.; Navarro, R.; Jensen, K.; Cayler, W.; Nielsen, T.; Live, C. C. Probiotic, or Neither? Microbial Composition of Retail-Available Kombucha and “Hard” Kombucha in the Pacific Northwest of the United States. Beverages. 2023, 9(3), 3. DOI: 10.3390/beverages9030059.
- Lutchmedial, M.; Ramlal, R.; Badrie, N.; Chang-Yen, I. Nutritional and Sensory Quality of Stirred Soursop (Annona Muricata L.) Yoghurt. Int. J. Food Sci. Nutr. 2004, 55(5), 407–414. DOI: 10.1080/09637480400002800.
- George, V. C.; Kumar, D. N.; Suresh, P. K.; Kumar, R. A. Antioxidant, DNA Protective Efficacy and HPLC Analysis of Annona Muricata (Soursop) Extracts. J. Food Sci. Technol. 2015, 52(4), 2328–2335. DOI: 10.1007/s13197-014-1289-7.
- Jayabalan, R.; Malbaša, R. V.; Lon ́ Car, E. S.; Vitas, J. S.; Sathishkumar, M. A Review on Kombucha Tea—Microbiology, Composition, Fermentation, Beneficial Effects, Toxicity, and Tea Fungus. Compr. Rev. Food Sci. 2014, 13(4), 538–550. DOI: 10.1111/1541-4337.12073.
- Tan, W. C.; Muhialdin, B. J.; Meor Hussin, A. S. Influence of Storage Conditions on the Quality, Metabolites, and Biological Activity of Soursop (Annona Muricata. L.) Kombucha. Front. Microbiol. 2020, 11. DOI: 10.3389/fmicb.2020.603481.
- Mohd Roby, B. H.; Muhialdin, B. J.; Abadl, M. M. T.; Mat nor, N. A.; Marzlan, A. A.; Lim, S. A. H.; Mustapha, N. A.; Meor Hussin, A. S. Physical Properties, Storage Stability, and Consumer Acceptability for Sourdough Bread Produced Using Encapsulated Kombucha Sourdough Starter Culture. J. Food Sci. 2020, 85(8), 2286–2295. DOI: 10.1111/1750-3841.15302.
- Chong, S.-G.; Ismail, I. S.; Ahmad Azam, A.; Tan, S.-J.; Shaari, K.; Tan, J.-K. Nuclear Magnetic Resonance Spectroscopy and Liquid Chromatography–Mass Spectrometry Metabolomics Studies on Non-Organic Soybeans versus Organic Soybeans (Glycine Max), and Their Fermentation by Rhizopus Oligosporus. J. Sci. Food Agric. 2023, 103(6), 3146–3156. DOI: 10.1002/jsfa.12355.
- Kamal, G. M.; Uddin, J.; Muhsinah, A. B.; Wang, X.; Noreen, A.; Sabir, A.; Musharraf, S. G. 1H NMR-Based Metabolomics and 13C Isotopic Ratio Evaluation to Differentiate Conventional and Organic Soy Sauce. Arabian J. Chem. 2022, 15(1), 103516. DOI: 10.1016/j.arabjc.2021.103516.
- Dimidi, E.; Cox, S. R.; Rossi, M.; Whelan, K. Fermented Foods: Definitions and Characteristics, Impact on the Gut Microbiota and Effects on Gastrointestinal Health and Disease. Nutrients. 2019, 11(8), 8. DOI: 10.3390/nu11081806.
- Motonaga, M.; Watanabe, H.; Tayama, Y.; Shimizu, R.; Sanoh, S.; Kotake, Y.; Kitamura, S.; Ohta, S.; Sugihara, K. Comparison of the Components of Three Types of Miso (Fermented Soybean Paste) by 1H NMR Metabolomic Analysis. BPB Reports. 2021, 4(5), 148–154. DOI: 10.1248/bpbreports.4.5_148.
- Ferri, M.; Graen-Heedfeld, J.; Bretz, K.; Guillon, F.; Michelini, E.; Calabretta, M. M.; Lamborghini, M.; Gruarin, N.; Roda, A.; Kraft, A., et al. Peptide Fractions Obtained from Rice By-Products by Means of an Environment-Friendly Process Show in vitro Health-Related Bioactivities. PLOS ONE. 2017, 12(1), e0170954. DOI: 10.1371/journal.pone.0170954.
- Donkor, O. N.; Henriksson, A.; Vasiljevic, T.; Shah, N. P. Probiotic Strains as Starter Cultures Improve Angiotensin‐Converting Enzyme Inhibitory Activity in Soy Yogurt. J. Food Sci. 2005, 70(8), m375–m381. DOI: 10.1111/j.1365-2621.2005.tb11522.x.
- Trimigno, A.; Bøge Lyndgaard, C.; Atladóttir, G. A.; Aru, V.; Balling Engelsen, S.; Harder Clemmensen, L. K. An NMR Metabolomics Approach to Investigate Factors Affecting the Yoghurt Fermentation Process and Quality. Metabolites. 2020, 10(7), 293. DOI: 10.3390/metabo10070293.
- Wongsa, P.; Yuenyongrattanakorn, K.; Pongvachirint, W.; Auntalarok, A. Improving Anti-Hypertensive Properties of Plant-Based Alternatives to Yogurt Fortified with Rice Protein Hydrolysate. Heliyon. 2022, 8(10), e11087. DOI: 10.1016/j.heliyon.2022.e11087.
- Lu, Y.; Hu, F.; Miyakawa, T.; Tanokura, M. Complex Mixture Analysis of Organic Compounds in Yogurt by NMR Spectroscopy. Metabolites. 2016, 6(2), 19. DOI: 10.3390/metabo6020019.
- Lu, Y.; Ishikawa, H.; Kwon, Y.; Hu, F.; Miyakawa, T.; Tanokura, M. Real-Time Monitoring of Chemical Changes in Three Kinds of Fermented Milk Products During Fermentation Using Quantitative Difference Nuclear Magnetic Resonance Spectroscopy. J. Agric. Food Chem. 2018, 66(6), 1479–1487. DOI: 10.1021/acs.jafc.7b05279.
- Muhialdin, B. J.; Kadum, H.; Zarei, M.; Meor Hussin, A. S. Effects of Metabolite Changes During Lacto-Fermentation on the Biological Activity and Consumer Acceptability for Dragon Fruit Juice. LWT - Food Sci. Technol. 2020, 121, 108992. DOI: 10.1016/j.lwt.2019.108992.
- Muhialdin, B. J.; Kadum, H.; Meor Hussin, A. S. Metabolomics Profiling of Fermented Cantaloupe Juice and the Potential Application to Extend the Shelf Life of Fresh Cantaloupe Juice for Six Months at 8 °C. Food Control. 2021, 120, 107555. DOI: 10.1016/j.foodcont.2020.107555.
- Muhialdin, B. J.; Meor Hussin, A. S.; Kadum, H.; Abdul Hamid, A.; Jaafar, A. H. Metabolomic Changes and Biological Activities During the Lacto-Fermentation of Jackfruit Juice Using Lactobacillus Casei ATCC334. LWT - Food Sci. Technol. 2021, 141, 110940. DOI: 10.1016/j.lwt.2021.110940.
- Markkinen, N.; Pariyani, R.; Jokioja, J.; Kortesniemi, M.; Laaksonen, O.; Yang, B. NMR-Based Metabolomics Approach on Optimization of Malolactic Fermentation of Sea Buckthorn Juice with Lactiplantibacillus plantarum. Food Chem. 2022, 366, 130630. DOI: 10.1016/j.foodchem.2021.130630.
- Colosimo, R.; Gabriele, M.; Cifelli, M.; Longo, V.; Domenici, V.; Pucci, L. The Effect of Sourdough Fermentation on Triticum Dicoccum from Garfagnana: 1H NMR Characterization and Analysis of the Antioxidant Activity. Food Chem. 2020, 305, 125510. DOI: 10.1016/j.foodchem.2019.125510.
- Brigante, F. I.; García, M. E.; López Radcenco, A.; Moyna, G.; Wunderlin, D. A.; Baroni, M. V. Identification of Chia, Flax and Sesame Seeds Authenticity Markers by NMR-Based Untargeted Metabolomics and Their Validation in Bakery Products Containing Them. Food Chem. 2022, 387, 132925. DOI: 10.1016/j.foodchem.2022.132925.
- Marques, V. D.; Franzolin, M. R.; Sanabani, S. S.; Vigerelli, H.; Piazza, R. M. F.; Pimenta, D. C.; Venâncio, T.; Neves, I. V.; de Sousa Silva, H. G. A New Class of Antimicrobial Molecules Derived from Kefir, Effective Against Pseudomonas aeruginosa and Methicillin Resistant Staphylococcus Aureus (MRSA) Strains. Sci. Rep. 2020, 10(1), 17434.
- Ullah, I.; Hu, Y.; You, J.; Yin, T.; Xiong, S.; Din, Z.; Huang, Q.; Liu, R. Influence of Okara Dietary Fiber with Varying Particle Sizes on Gelling Properties, Water State and Microstructure of Tofu Gel. Food Hydrocoll. 2019, 89, 512–522. DOI: 10.1016/j.foodhyd.2018.11.006.
- Ge, X.; Tang, N.; Huang, Y. ;.; Chen, X.; Dong, M.; Rui, X.; Zhang, Q.; Li, W. Fermentative and Physicochemical Properties of Fermented Milk Supplemented with Sea Buckthorn (Hippophae Elaeagnaceae L.). LWT - Food Sci. Technol. 2022, 153, 112484. DOI: 10.1016/j.lwt.2021.112484.
- Xiao, R.; Liu, M.; Tian, Q.; Hui, M.; Shi, X.; Hou, X. Physical and Chemical Properties, Structural Characterization and Nutritional Analysis of Kefir Yoghurt. Front. Microbiol. 2022, 13, 1107092. DOI: 10.3389/fmicb.2022.1107092.
- Santos, M. H. S. Biogenic Amines: Their Importance in Foods. Int. J. Food Microbiol. 1996, 29(2), 213–231. DOI: 10.1016/0168-1605(95)00032-1.
- Mannaa, M.; Seo, Y.-S.; Park, I. Addition of Coriander During Fermentation of Korean Soy Sauce (Gangjang) Causes Significant Shift in Microbial Composition and Reduction in Biogenic Amine Levels. Foods. 2020, 9(10), 1346. DOI: 10.3390/foods9101346.
- He, L.; He, T.; Farrar, S.; Ji, L.; Liu, T.; Ma, X. Antioxidants Maintain Cellular Redox Homeostasis by Elimination of Reactive Oxygen Species. Cell. Physiol. Biochem. 2017, 44(2), 532–553. DOI: 10.1159/000485089.
- Hu, Y.; Liu, X.; Wu, X.; Zhang, Z.; Wu, D.; Chen, C.; Su, W.; Zhang, L.; Li, J.; Wang, H.-M. D. Several Natural Phytochemicals from Chinese Traditional Fermented Food-Pickled Raphanus Sativus L.: Purification and Characterization. Food Chem. X. 2022, 15, 100390. DOI: 10.1016/j.fochx.2022.100390.
- Catzeddu, P.; Fois, S.; Tolu, V.; Sanna, M.; Braca, A.; Vitangeli, I.; Anedda, R.; Roggio, T. Quality Evaluation of Fresh Pasta Fortified with Sourdough Containing Wheat Germ and Wholemeal Semolina. Foods. 2023, 12(14), 2641. DOI: 10.3390/foods12142641.
- Münger, L. H.; Trimigno, A.; Picone, G.; Freiburghaus, C.; Pimentel, G.; Burton, K. J.; Pralong, F. P.; Vionnet, N.; Capozzi, F.; Badertscher, R., et al. Identification of Urinary Food Intake Biomarkers for Milk, Cheese, and Soy-Based Drink by Untargeted GC-MS and NMR in Healthy Humans. J. Proteome Res. 2017, 16 (9), 3321–3335. DOI: 10.1021/acs.jproteome.7b00319.
- Saha, D.; Bhattacharya, S. Hydrocolloids as Thickening and Gelling Agents in Food: A Critical Review. J. Food Sci. Technol. 2010, 47(6), 587–597. DOI: 10.1007/s13197-010-0162-6.
- Radziej, S.; Scherb-Forster, J.; Schlicht, C.; Eisenreich, W. Fast Identification of Food Thickeners by Nontargeted NMR-Spectroscopy. J. Agric. Food Chem. 2021, 69(12), 3761–3775. DOI: 10.1021/acs.jafc.0c07760.