Abstract
Differential Scanning Calorimetry (DSC) was used to obtain specific heat, heat of fusion, and protein denaturation temperatures of raw skipjack tuna. Thermal conductivity values of raw skipjack tuna over a range of temperatures were determined by the line heat source probe method. Statistical analysis showed that there was no significant difference between the specific heat of loin meat (3.536 kJ/kg K), red meat (3.505 kJ/kg K), and viscera (2.263 kJ/kg K). There were significant differences between the backbone and loin meat, backbone and red meat, and backbone and viscera on specific heat values. The protein denaturation temperature of loin meat was higher than that of red meat. There was no significant difference between thermal conductivity of raw loin meat (0.57 W/m K) and viscera (0.56 W/m K). In general, thermal conductivity increased with temperature.
NOMENCLATURE
cp | = |
Specific heat (kJ/kg K) |
q | = |
Energy, I 2 R w (W) |
k | = |
Thermal conductivity (W/m K) |
kc | = |
Estimated thermal conductivity (W/m K) |
t | = |
Time (min) |
H | = |
Enthalpy (kJ/kg) |
I | = |
Current, V r/R (A) |
Rw | = |
Resistance of heater wire (Ω/m) |
T | = |
Temperature (°C) |
Vr | = |
Voltage across resistor (V) |
Xw | = |
Mass fraction of water (-) |
INTRODUCTION
The thermal properties of food are of interest to food engineers in modeling food processes and in designing equipment. As computer technology continues to develop, the improved accuracy of mathematical models for food processes depends on the accuracy of property data. Thawing, precooking, and cooling are three processes used in the commercial tuna canning industry. To develop accurate mathematical models to find the optimal time and temperature conditions in these processes, thermal properties of tuna, for example, specific heat (c p), thermal conductivity (k), and enthalpy (H) must be known.
The solid pack style of canned tuna has dominated the market over the years. This style was made mostly from the albacore tuna, its delicate, white meat fetching the highest price. However, tuna canners have been forced to use other species since the decline of the albacore tuna off the Pacific Coast over the last 20 years, even though a significant amount of frozen Japanese albacore is imported for canning. Yellowfin and skipjack tuna have replaced the albacore as the main species canned in the United States, and it appears that the skipjack has rapidly replaced yellowfin as the largest single contributor of raw material to the tuna canning trade. Skipjack, being darker fleshed and stronger flavored, is less valuable other than species and little research has been done on this species.
Specific heat has been determined using microcalorimetry with internal heating and recently by means of Differential Scanning Calorimetry (DSC) Citation[1]. The advantage of DSC is that it works rapidly and simply, much valuable information can be obtained by a single thermogram, and a very small sample can yield accurate results Citation[2]. This method is also used to study the thermal properties of muscle proteins Citation[3] and protein denaturation Citation[4]. There have been many applications of DSC in polymer science and food science. The effects of process conditions on beef and vegetable protein denaturation have been investigated Citation4-5. Hastings et al. Citation[6] studied the protein denaturation of different varieties of fish muscle.
DSC has been used to study the thermally-induced transitions of rabbit and beef actin, myosin, and sacroplasmic proteins as a function of pH and ionic strength Citation[3]. The effects of process conditions on beef and vegetable protein denaturation have also been investigated. As the DSC measures net changes and can not differentiate between the endotherms caused by the activation energy required for denaturation and the exothermic process of aggregation, the measurements for meat do not agree on which protein is responsible for each transition noted. Transitions around 55°C and 80°C are accepted as myosin and actin, respectively Citation[7]. The transition around 67°C may be attributable to sarcoplasmic protein, connective tissue, or heavy meromyosin Citation[7]. Hasting et al. Citation[6] reported 8 protein transitions for purified cod muscle protein. Transition temperatures ranged from 27°C to 87°C.
For measuring the thermal conductivity of foods, the line source probe method is fast and simple, and requires a relatively small sample Citation[8]. The basic theory behind the probe method involves a line heat source that has been discussed previously by Wang et al. Citation[9]. This method has been applied in many different areas. There are research reports on such materials as soil, silicon rubber materials, and liquid chemicals. There are also studies on the thermal conductivity of foods. These include frozen foods, apple juice, food powders, and fruits and vegetables Citation[9]. Recently, thermal conductivity measurement for shrimp Citation[10] and albacore tuna Citation[1] have been reported. However, for albacore, limited data is available over wide temperature ranges. No information is available on protein denaturation enthalpy of tuna muscle. The objectives for this study were to determine the protein denaturation temperature and heat of fusion for skipjack tuna as well as specific heat and thermal conductivity over temperature ranges found during processing.
MATERIALS AND METHODS
Frozen skipjack tuna (Katsuwonus pelamis) was obtained from a commercial tuna processing plant. Lengths ranged from 50.4 to 55.9 cm. Moisture content of skipjack was determined by the oven-drying method [950.46B Citation[11]] and the average value was 70.8%. Whole fish were stored at −45°C until needed.
Specific Heat and Protein Denaturation
Four 2-cm thick steaks were cut from whole fish and held at 24 ± 2°C for thawing. After thawing, each piece was separated into four portions according to region: loin meat, red meat, viscera, and backbone. The skin portion was discarded. Individual portions were ground to a homogenous state by using Handy Chopper Plus (HC3000, Black & Decker, Brockville, Ontario). Each portion was used for specific heat measurement. Loin meat and red meat were used for protein denaturation temperature measurement.
Specific heat and protein denaturation data were determined using a Perkin-Elmer DSC 7 equipped with an Intracooler II refrigeration unit and dry box (Perkin-Elmer Corp., Norwalk, CT). Nitrogen gas was used to flush the sample holder and the dry box. The DSC was calibrated using both indium and dodecane.
Specific heat was determined for six individual (45–68 mg) samples of each region using stainless steel pans (Perkin-Elmer Corp., Norwalk, CT). Samples were scanned from 5 to 105°C at a heating rate at 5°C/min using an empty pan as the reference. Reference pans and sample pans were balanced to within 0.1 mg. Specific heat was calculated using the single curve method (Pyris, Perkin-Elmer Corp., Norwalk, CT). To ascertain the accuracy of the measurements, the specific heat of high-performance liquid chromatographic (HPLC) grade water was measured and found to be within ±2.4% of published values Citation[12].
For protein denaturation temperature measurements, six individual (45–68 mg) samples of loin meat and red meat were loaded into stainless steel pans and scanned from 5 to 105°C at a heating rate at 5°C/min. High-performance liquid chromatography (HPLC) water, with a weight identical to 70% of the loin meat weight, was loaded into the reference pan. This weight was selected because it reflected the approximate weight of water in the loin meat. Heat of fusion of loin meat and red meat was determined by scanning six samples from −30°C to 20°C at a heating rate of 5°C/min.
Thermal Conductivity Measurement
Whole fish were cut into four equal length pieces and held at 24 ± 2°C for thawing. After thawing, loin meat was cut into 2-cm thick steaks. Viscera was ground to a homogenous state. A controlled temperature water bath was used to bring samples to test temperatures for determining thermal conductivity values (k values) for the loin meat. A range of temperatures commonly found in processing was used.
The line heat source method was used to determine the thermal conductivity of tuna samples. A 1.25-mm diameter thermal conductivity probe Citation[12] was used with a Campbell Scientific 21 × datalogger (Salt Lake City, UT) to record temperature and voltage data at a sampling rate at 4 Hz. Voltage (V r) across a precision resistor (R = 1.02088 Ω) was measured, thus the current (I) to the heating element within the probe was determined. Another voltage measurement provided the voltage (V i) across the heating element. This was used to determine the energy (q) output by the heating element. This was used to determine the energy (q) output by the heating element. The resistance of heater wire was (R w = 223.097 Ω/m).
Thermal conductivity was calculated using Equation Equation1 below Equation1:
Before each experiment, the thermal conductivity probe was calibrated using olive oil. The experimental value obtained was compared with the published value of olive oil and a correction factor C was obtained.
At an ambient temperature of 23°C, the thermal conductivity of loin meat was measured perpendicular to the fiber of the muscle. The line heat source probe was inserted into a loin steak sample for no more than 45 s. Ground viscera was placed into a 30-mL beaker, into which the probe was placed for measurement.
RESULTS AND DISCUSSION
Specific Heat
Values of specific heat ranged from 3.192 to 3.699 kJ/kg K for loin meat, 3.180 to 3.667 kJ/kg K for red meat, 3.162 to 3.624 kJ/kg K for viscera, and 1.838 to 2.596 kJ/kg K for backbone over a temperature range of 10 to 105°C (Fig. ). The average values of specific heat over this temperature range were 3.536, 3.505, 3.446, and 2.263 kJ/kg K for loin meat, red meat, viscera, and backbone, respectively. The specific heat value for loin meat was found to be slightly higher than red meat and viscera, and much higher than backbone. The specific heat for loin meat increased with temperature over the range of 10–65°C but decreased from 65 to 80°C followed by an increase. For red meat, specific heat increased up to 70°C and then decreased until 85°C was reached. This was followed by an increase. For viscera, specific heat increased with temperature, but had a slight decrease at 100°C. For backbone, specific heat increased with temperature except at 65 and 95°C.
Figure 1. Mean specific heat of skipjack loin meat, red meat, viscera, and backbone over the temperature range of 10–105°C n = 6. Standard deviations for this data were too small to be shown on plot.
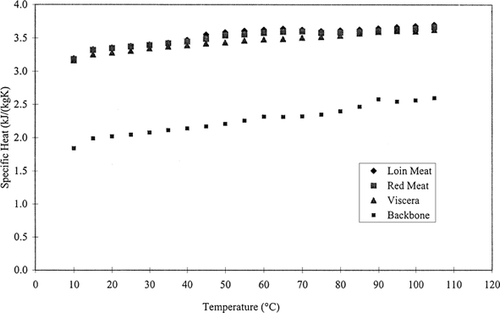
Data were statistically analyzed with the SAS GLM linear model Citation[13]. Statistical analysis showed at p < 0.05 level, a significant difference exists between backbone and loin meat, red meat, and viscera. There were no significant differences between loin meat, red meat, or viscera.
A comparison with the data from Perez-Martin et al. Citation[1] study on albacore (Table ) showed the specific heat value of skipjack loin meat to be 9% higher than albacore at 25°C. Specific heat values of skipjack loin meat at different temperatures were also higher. These differences can be attributed to differences in composition, especially the lower moisture content of albacore (67.3%) Citation[1].
Table 1. Comparison of Specific Heat of Loin Meat of Skipjack and Albacore Muscle
Protein Denaturation Analysis
Figure shows a typical DSC thermogram for skipjack tuna loin muscle, and Table gives three protein denaturation temperatures for loin and red meat. Red meat has higher transition temperatures than loin meat. On the basis of our results, and assuming that tuna DSC profiles follow a similar pattern to those of cod, the peaks on the thermogram can be assigned to be: (Protein 1) composite of the second (major) myosin transition and the first sarcoplasmic transition; (Protein 2) second sarcoplasmic transition; and (Protein 3) third sarcoplasmic transition or actin transition.
Table 2. Protein Denaturation Temperature
Figure 2. DSC thermogram for protein denaturation. Peaks 1, 2, and 3 represent the three protein denaturation temperatures discussed in text.
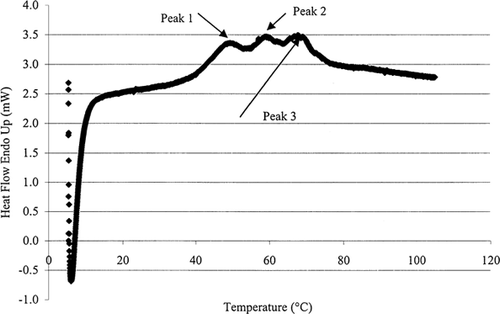
The moisture content of loin meat and read meat was found to be 71.6 and 69.9%, respectively. Heat of fusion was found to be 187.76 kJ/kg K for loin meat and was 166.45 kJ/kg K for red meat. These results are consistent with the relationship between moisture content of food material and the value of heat of fusion.
Thermal Conductivity
At an ambient temperature of 24°C, the thermal conductivity of raw skipjack loin meat was 0.57 ± 0.01 W/m K. The thermal conductivity of viscera was 0.56 ± 0.01 W/m K. Statistical analysis showed that there were no significant difference between loin meat and viscera.
Salvadori and Mascheroni Citation[14] proposed the general correlation for meat products when heat transfer is perpendicular to the fiber and the muscle temperature is above its freezing point.
Based on our experiments for loin meat, at a moisture content of 71.6% and temperature of 23°C, the estimated thermal conductivity value for loin meat using Equation Equation3 is:
For viscera with a moisture content of 70.5%, and the same temperature, the estimated thermal conductivity value using Equation Equation3 is:
The small difference between the estimated value and experimental value maybe considered negligible and Equation Equation3 used in the temperature range of 20 to 60°C but above this temperature the predicted values did not agree with experimental data, which increased at a greater rate (Fig. ).
Figure 3. Thermal conductivity of skipjack tuna loin muscle at various temperatures (n = 10 for all measurement except at a temperature of 91.6°C where n = 1).
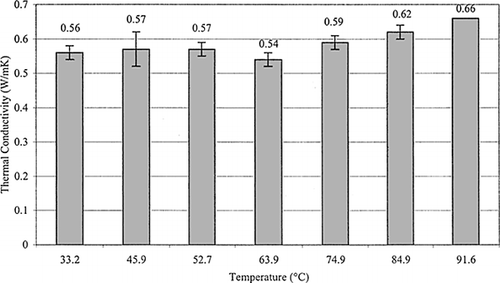
During commercial tuna processing, tuna are precooked to 60–65°C center or backbone temperature while the tuna surface may reach a maximum of 102°C. Based on this information, thermal conductivity values over a range of processing temperatures were needed as well as at an ambient temperature value. Thermal conductivity was measured over a temperature range of 30–90°C, at 10°C intervals. Results (Fig. ) showed that the thermal conductivity value of loin meat ranged from 0.56 W/m K to 0.66 W/m K from 33.22°C to 91.60°C. The k value increased with temperature but at 63.91°C it decreased from 0.57 W/m K to 0.54 W/m K and then increased afterward. Experiment data were statistically analyzed with the SAS GLM linear model Citation[13] and showed that there were no significant differences between k values at the different temperatures.
The thermal conductivity for loin meat was higher than the reported value for raw albacore with a perpendicular heat flux, but was close to the reported value with heat parallel to fibers Citation[1]. The differences can be attributed to the composition difference of the species.
CONCLUSIONS
Specific heat values of skipjack tuna loin meat were slightly higher than that of red meat, viscera, and backbone over the temperature range of 10–105°C, but were not significantly different from red meat and viscera. This can be attributed to the higher moisture content of loin meat. Proteins denatured at 42.56°C, 56.40°C, and 68.69°C for loin meat and 43.23°C, 57.10°C, and 67.88°C for red meat. These are associated with: 1) composite of the second (major) myosin transition and the first sarcoplasmic transition; 2) the second sarcoplasmic transition; and 3) the third sarcoplasmic transition or actin transition.
The thermal conductivity for loin meat had no significant difference from viscera. Moisture content of these two portions of fish was not significantly different. Thermal conductivity increased with temperature over a range of 33.22–91.60°C except at 63.91°C. The decrease can be attributed to the fish muscle protein denaturation at that temperature range.
The values of specific heat, protein denaturation, and thermal conductivity determined in this work will be of interest for mathematical modeling of thermal processes and aid in the determination of optimal thermal processing conditions for commercial tuna canning.
ACKNOWLEDGMENTS
The authors acknowledge the financial support of Star-Kist Seafood Inc., the United States Department of Agriculture National Needs Fellowship Program, and North Carolina SeaGrant. In addition, the authors wish to thank Ms. Penny Amato for her assistance with the DSC equipment and valuable discussion and Ms. Heather Stewart for her help with the line source heat probe.
REFERENCES
- Perez-Martin , R. I. , Gallardo , J. M. , Banga , J. R. and Casares , J. 1989 . Determination of Thermal Conductivity, Specific Heat and Thermal Diffusivity of Albacore (Thunnus alalunga) . Z. Lebnsm Unters Forsch , 189 : 525 – 529 .
- McNaughton , J. L. and Mortimer , C. T. 1975 . Differential Scanning Calorimetry , IRS Physical Chemistry Series, 2 Vol. 10 , London : Butterworths .
- Wright , D. J. , Leach , I. B. and Wilding , P. 1977 . Differential Scanning Calorimetry Studies of Muscle and Its Constituent Proteins . J. Sci. Food Agr. , 28 : 557
- Arntifield , S. D. and Murray , E. D. 1981 . The Influence of Processing Parameters on Food Protein Functionality. 1. Differential Scanning Calorimetry as an Indicator of Protein Denaturation . Can. Inst. Food Sci. Technol. J. , 14 : 289
- Quinn , J. R. , Raymond , D. P. and Harwalker , V. R. 1980 . Differential Scanning Calorimetry of Meat Proteins as Affected by Processing Treatment . J. Food Sci. , 45 : 1146
- Hastings , R. J. , Rodger , G. W. , Park , R. , Mattews , A. D. and Anderson , E. M. 1985 . Differential Scanning Calorimetry of Fish Muscle: The Effect of Processing and Species Variation . J. Food Sci. , 50 : 503 – 510 .
- Findlay , C. J. and Barbut , S. 1990 . “ Thermal Analysis of Meat ” . In Thermal Analysis of Foods New York : Elsevier Applied Science .
- Sweat , V. E. 1985 . “ Thermal Conductivity of Food: Present State of the Data ” . In ASHRAE Transactions , Part 2B Vol. 91 , 299 U.S.
- Wang , J. J. and Hayakawa , K. I. 1993 . Maximum Slope Method for Evaluating Thermal Conductivity Probe Data . J. Food Sci. , 58 : 1340 – 1345 .
- Karunakar , B. , Mishra , S. K. and Sukumar , B. 1998 . Specific Heat and Thermal Conductivity of Shrimp Meat . J. Food Eng. , 37 ( 3 ) : 345 – 351 .
- 1995 . “ A.O.A.C. ” . In Official Methods of Analysis , 16th Ed. Washington, DC : Association of Official Analytical Chemists .
- Weast , R. C. 1977 . Handbook of Chemistry and Physics Cleveland : CRC Publisher .
- Rahman , M. S. 1995 . Food Properties Handbook 225–273, 275, 283 – 338 . Boca Raton, FL : CRC Press Inc. .
- 1996 . “ SAS ” . In SAS User's Guide: Statistics Cary, NC : SAS Institute, Inc. .
- Salvadori , V. O. and Masheroni , R. H. 1991 . Prediction of Freezing and Thawing Time of Foods by Means of a Simplified Analytical Method . J. Food Eng. , 13 : 67