Abstract
Problems associated with the stickiness of food in processing and storage practices along with its causative factors are outlined. Fundamental mechanisms that explain why and how food products become sticky are discussed. Methods currently in use for characterizing and overcoming stickiness problems in food processing and storage operations are described. The use of glass transition temperature-based model, which provides a rational basis for understanding and characterizing the stickiness of many food products, is highlighted.
1. INTRODUCTION
Some foods exhibit a marked tendency to adhere to a contact surface, which is generally known as stickiness. This stickiness can be perceived in the palate, teeth, and tongue when the food is being masticated (Jowitt, 1974) and can also be perceived on nonoral surfaces such as fingers and equipment surfaces. Although the stickiness to some extent may be a welcome sensory and processing attribute (Kilcast and Roberts, 1998), it is often a problem in manufacturing operations. Stickiness can cause lower product yield, operational problems, equipment wear, and fire hazards.
Although the problem of stickiness in food industries was recognized and a systematic approach to quantify it was initiated long ago (Szczesniak, 1963), methods developed to quantify it have been empirical in nature (Brennan and Mohamed, 1984). There is no general consensus as to what factors and forces are involved in stickiness. Some authors attribute it to adhesive force, some see it as being attributable to a combined effect of adhesive and cohesive forces, and others include viscosity and viscoelasticity as well. Typical examples of stickiness in some of the food industries are outlined below.
Cereal Industries
Stickiness associated with dough can have strongly negative effects on dough handling and breadmaking quality. Sticky dough causes low dough mixing tolerance, reduced dough strength, and reduced bread volume (Martin and Stewart, 1986; Graybosch, et al., 1993). Dough stickiness problem is of particular concern to large mechanized bakeries where it can result in costly disruptions to production schedules and in loss of product qualities (Huang and Hoseney, 1999). Genetically modified, disease-resistant wheat varieties are increasingly cultivated to produce higher grain yield and improve agronomic performance (Henry et al., 1989; Dhaliwal et al., 1990). However, flours made from these wheat varieties are reported to produce doughs with marked stickiness (Martin and Stewart, 1986). The study of stickiness and its quantification is an important issue in the cereal industries.
Confectionery Industries
Boiled sweets are typically sucrose-glucose or sucrose-invert syrups. The concentration of invert sugars in confectionery products is maintained at such a level that at ordinary temperature they are glassy solids. The problem with these products in a moist environment is that they become extremely sticky, subsequently leading to graining or fluidity (White and Cakebread, 1966). When the relative humidity of the storage environment is higher than the value at which the confectionery products can be kept safely, stickiness sets in. Adsorption of moisture by the confectionery products results in a lowered viscosity that leads not only to stickiness but also to the collapse of the product structure.
Dairy Industries
Stickiness and the subsequent caking of milk powders has already received much attention (Papadakis and Bahu, 1992; Chuy and Labuza; 1994; Lloyd et al, 1996; Rennie et al., 1999). Stickiness and collapse of structure are common phenomena in ice cream (White and Cakebread, 1966). Adhesion of milk constituents on the surface of processing equipment and subsequent fouling is a nuisance in most milk processing plants (Burton, 1968). In continuous cheesemaking machines, adhesion of coagulated milk proteins on surfaces (especially on stainless steel) has been a serious problem (Hegg et al, 1985). Adhesion of milk constituents, especially the proteins and minerals in reverse osmosis, ultrafiltration, or microfiltration membranes results in a persistent type of fouling that results in the need to clean at regular intervals (Marshall and Daufin, 1995).
Powder Industries
Although stickiness is an attribute in limited processes such as agglomeration, it is in general a negative attribute. In milk powder production, the powder turns to be lumpy when the equilibrium moisture and temperature matrix exceeds the one with respect to lactose (White and Cakebread, 1966; Chuy and Labuza, 1994). In fruit powders stickiness is a commonly encountered problem because of the presence of the low molecular weight sugars in high concentration (Brennan et al., 1971; Downton et al., 1982; Bhandari et al., 1997a). The presence of glassy glucose, fructose, and sucrose in spray and freeze-dried fruit powders makes these powders particularly susceptible to moisture and temperature.
Sticking on Packaging Materials
Adhesion or sticking of food materials in food cans and packages not only adversely affects consumers' confidence in the quality of the product but also results in lower product utility. Sticking of food in packaging causes further damage in packaging material, and disfigurement of the product surface. In general, the greater the extent of sticking of food in packages the higher the consumer antipathy to the product (Kilcast and Roberts, 1998).
Researchers have proposed various mechanisms to explain stickiness in food depending on the type of food products. These mechanisms also reflect the background of those who propose them: polymer physics, physical chemistry, rheology, engineering, and mechanics etc (Lai, 1986). There is no quantitative model that successfully and generally describes the mechanism of stickiness in food systems. Mechanisms based on interparticle attraction (Rumpf, 1962; Schubert, 1987; Peleg; 1993) successfully explain the unwanted agglomeration and loss of flowability in dry powders. Wetting and thermodynamic adsorption based on Young's force equation (Young, 1805) and Dupre's (Dupre, 1869) energy equation explain the sticky issue based on the surface energies. The mechanisms based on tack and rheology are used to describe pressure-sensitive adhesives (Hammond, 1989; Satas, 1989; Kokini et al., 1984) and have found use in the quantification of dough stickiness (Chen and Hoseney, 1995).
Instrumental methods for quantification of stickiness in food have been product-specific. Rotational sticky point testers based on Lazer et al. (1956) have been developed and adapted by drying industries (Downton et al., 1982; Wallack and King, 1988). Measurement of cohesive and adhesive forces based on Jenike's shear cell or similar devices has been widely used to characterize the degree of powder flowability by powder industries (Peleg, 1993; Pasley and Haloulos, 1994; Teunou et al., 1999). Probe methods that have been in application in pressure-sensitive adhesives are successfully adapted to measure the stickiness of wheat dough (Chen and Hoseney, 1995) and candy (Kilcast and Roberts, 1998). Recently, a glass transition temperature approach has emerged as a fundamental technique to characterize the stickiness in spray- and freeze-drying operations (Roos and Karel, 1991a; Truong et al., 1999).
In this context, Section 2 reviews the food-related and non-food related factors that contribute to stickiness. Section 3 reviews the mechanisms of stickiness that are relevant to food systems. Likewise, Section 4 reviews the techniques adapted by different food industries to characterize stickiness along with the instruments involved. Finally, Section 5 reviews the techniques that have been currently adapted to overcome the problem of stickiness in foods especially in drying and storage operations.
2. FACTORS AFFECTING STICKINESS
2.1. Viscosity
Many foods are in an amorphous or supercooled liquid state. Viscosity of the amorphous food system is so high that (1012–14 Pa.s) they are capable of supporting their weight against flow resulting from the force of gravity. On heating, the amorphous state yields to a viscous one where its viscosity decreases sharply to 106–8 Pa.s (Downton et al., 1982; Wallack and King, 1988) thereby causing stickiness. It was found that consistency of cooked rice was well-correlated negatively with its stickiness (Kumar et al., 1976). Variation of viscosity of carbohydrates (polymeric, oligomeric, and monomeric) or low-moisture synthetic polymers between glass transition and melting point temperature is given by the WLF (Wiliams-Landel-Ferry) equation (Williams et al., 1955). The reduced viscosity greatly enhances the molecular mobility of the system, which is linked to the textural problems like stickiness and adhesion. The lowered viscosity and subsequent softening is also linked to the liquid and solid bridges that causes caking in food particulate systems (Papadakis and Bahu, 1992; Peleg, 1993). Equation Equation1 is a modified version of the WLF equation that gives the temperature dependence of viscosity above the glass transition temperature (Section 3.4 deals more with glass transition temperature) (Soesanto and Williams, 1981; Angell et al., 1982). It is reported that the Equation Equation1 is applicable between Tg to Tg + 100°C, above which the Arrhenius type of dependence prevails (Roos, 1992).
2.2. Water
The interaction of water with solids is the prime cause of stickiness and caking in low-moisture foods. Properties like viscosity, surface tension, and good solvation all make water a suitable catalyst for stickiness, caking, and collapse of food and particulate systems. Besides, it is a part of atmosphere and any increase in the relative humidity of air is associated with the possibility of increased amount of water being adsorbed to the surface or absorbed in to the bulk (Griffith, 1991). At room temperature the critical relative humidity, the humidity at which the food powders exhibit caking, can be as low as 33% in the case of tea and whey powder (Teunou et al., 1999).
Water is a ubiquitous plasticizer (refers to the process of softening and increase in flexibility of a polymer brought about by the incorporation of a plasticizer, alternatively defined as a process of lowering the glass transition temperature) for low-moisture foods and biological materials (Slade and Levine, 1991). The increase of water content results in a sharp decrease in the glass transition temperature (Tg ). The Tg of food materials such as sugars, starch, gluten, gelatin, hemicellulose, and elastin decreases rapidly to about −10°C or so when moisture content increases to 30% by mass (Atkins, 1987). Furthermore, the rate of decrease of Tg attributable to water plasticization is ∼10°C per 0.01g of water/g of material (Slade et al., 1989). Plasticization of amorphous food powders and the subsequent depression of their glass transition temperature below ambient temperatures is related to undesired textural behavior such as caking and stickiness in food powders including sugar-rich foods (Slade and Levine, 1991), the mechanism of this effect may be through much lowered viscosity (Downton et al., 1982; Wallack and King, 1988) or the viscous effects of liquid bridges (Peleg, 1993).
Furthermore, water can exist on particle surfaces as adsorbed mono/multi-layers or as capillary condensation. This can reduce surface micro-roughness of the particles thereby allowing them to come closer, which then increases the forces of attraction between the particles. This may be an important cause of caking of the particles in the presence of water (Iveson, 1997).
2.3. Temperature
The temperature of an amorphous or semicrystalline system determines whether the mobility of food molecules is in frozen state (glassy) or in viscous mobile (rubbery) one. Viscosity of food systems is a function of temperature. If the temperature of amorphous foods is higher than Tg , they will be transformed into a liquid-like, rubbery state that is associated with stickiness and caking (Roos and Karel, 1993). Roos and Karel (1993) reported that the critical viscosity of stickiness (Downton et al., 1982) is equivalent to the viscosity of the amorphous sugars at T-Tg values varying from 10 to 20°C, which means that the sticky point temperature is 10–20°C higher than the glass transition temperature. This implies that if the product temperature is less than the glass transition temperature, phenomena including stickiness and adhesion will not take place. It is also emphasized that for amorphous products of any kind the magnitude of T-Tg is a fundamental indicator of stickiness and adhesion (Slade and Levine, 1991).
2.4. Compression
Higher pressure or compression of a solid system stimulates stickiness and caking of food powders. If powders are exposed to sufficient external compression, they are inclined to cake (Griffith, 1991). This effect can be observed in rail or truck load of powders and also in stockpiles. The powder at the bottom of the stack is lumped or cemented. If the powder contains soluble components and the humidity and temperature of the surrounding is high, there is a marked influence of pressure on caking. There is also a time factor because pressure influence is not instantaneous. This is because pressure brings the particles very close to each other (decreased distance between the particles), and the surface area of contact increases (Schubert et al., 1975). The cohesiveness of a powder depends largely on the magnitude of the consolidation load applied, in general, the higher the consolidation load, the greater the cohesiveness of a powder (Teunou et al., 1999).
2.5. Food Ingredients
Amorphous low molecular weight sugars such as fructose, glucose, sucrose, and lactose have very high hygroscopicity and solubility. Even if sugars are in a crystalline form at room temperature, they are generally rendered amorphous as an effect of the size reduction operations (Kelley et al., 1974). The amorphous sugars are in a high-energy state compared with the corresponding crystalline form and hence are metastable. They have glass transition temperatures ranging between 5–100°C in their anhydrous state that are substantially depressed because of water platicization. As a result, the low molecular weight sugars are likely culprits of stickiness and caking in foods and food-based particulate systems. The hydrolyzed products of high DE (provides quantitative measure of starch polymer hydrolysis, a measure of reducing power compared with a dextrose standard of 100) starch derivatives are presumed to behave similarly to the low molecular weight sugars. Similarly, low molecular weight protein hydrolyzates presumably the amino acids were found to exhibit hygroscopicity and contribute to caking during storage of spray-dried fermented soy sauce powders (Hamano and Aoyama, 1974).
High molecular weight water compatible ingredients such as carbohydrates and proteins have a high glass transition temperature because Tg increases with increasing molecular weight (Fox and Flory; 1950; Roos and Karel, 1991). Hence, addition of ingredients like low DE maltodextrins is recommended both in drying (Bhandari et al., 1997b) and storage practices (Nisshin, 1981) to minimize stickiness and caking, respectively. In the presence of water different components of high molecular weight carbohydrates may behave differently. The starch of sticky or waxy rice, a rice variety which is popular in Japan, Korea, Taiwan, and Thailand (Juliano, 1993) has a high amylopectin/amylose ratio and it has been reported that amylopectin is responsible for stickiness in these rice varieties (Juliano, 1985a; Chen et al., 1999).
Full fat milk is much more difficult to spray dry and exhibits stickiness compared with skim milk. This is because milk fat has a low melting point, which raises the particle surface temperature during drying, impedes the diffusion of moisture from interior and evaporation from the surface of a drying drop (Stevenson et al., 1998). Furthermore, cohesion of whole milk powder of identical moisture content and at 30°C was found almost twice that of the skim milk powder and also that the flowability of whole milk powder was more sensitive to increase in temperature (Rennie et al., 1999). The liquefaction of fat above room temperature and subsequent softening of powder leads to increased contact area and formation of liquid bridges, thereby rendering the whole milk powders more cohesive. It was also reported that when whole milk powder was consolidated while the fat was liquid and then cooled to 5°C and subsequently tested, the cohesion was very high, which was a consequence of formation of solid bridges because of the fat crystallization (Buma, 1971).
A comparison of the degree of contribution of food compositional factors on stickiness is presented in Table .
Table 1. Factors Causing Stickiness and Their Relative Contribution
2.6. Particle Size
Particle size has a profound influence on the cohesive (cohesion is an internal property of material and a measure of force holding two similar particles/surfaces together) and adhesive (adhesion is an interfacial property and is a measure of force holding dissimilar particles/surfaces together) strength of a particulate system. Micro or nano size particles are highly influenced by electrostatic or molecular forces of attraction because of their very high surface to volume ratio. Van der Waals' forces f vdw can become significant for particles below 10 micron size where the interparticle distance becomes sufficiently small (Rumpf, 1962). The most typical characteristics of food particulate system compared with assemblies or large size bodies is that minor changes in the surface properties can bring about major changes in bulk properties. This is because the surface forces become dominant over gravitational forces in small particulates (Schubert, 1987). It is considered that powders with particle sizes larger than 200 micron are free-flowing while finer powders are susceptible to cohesion and their flowability is more difficult (Teunou et al., 1999). The cohesive or adhesive forces are inversely related to the particle size (Buma, 1971; Renne et al., 1999). Furthermore, if particles are of 1 micron size or finer, these molecular forces can appreciably deform them thereby creating more contact area that results in more intimate contact (Gay and Leibler, 1999). A diagram showing the strength of agglomerate bond as a function of particle size is given in Figure .
3. MECHANISMS OF STICKINESS
3.1. Interparticle Attraction
The factors rendering the particles adhesive or cohesive may be similar but higher cohesion is not necessarily associated with higher adhesion or vice versa. The extent of cohesive or adhesive behavior depends on the nature of the food and external factors like moisture and heat. According to Rumpf (1962), mechanisms of interparticle attraction have been divided in to four major groups: intermolecular and electrostatic forces, liquid bridges, solid bridges, and mechanical interlocking.
a. Intermolecular and Electrostatic Forces
Cohesion between two particles and adhesion between two surfaces without material bridges is primarily attributable to Fvdw and electrostatic forces. For particles smaller than 5 micron, these forces become dominant compared with the weight of the particles and they tend to form agglomerates without the need for solid and liquid bridges (Walton and Mumford, 1999). The Fvdw can be calculated for two spheres of diameter d 1 (m) and d 2 (m) separated from each other by distance x (m), as:
Electrostatic Forces
Electrostatic adhesion occurs where particles possess excess opposing charges. This situation may also arise when particles come in contact attributable to different contact potential values. Electrically nonconducting particles are capable of carrying excess electrical charges (Schubert, 1987). For an ideal electrical insulator the adhesion forces, F el,i,(N) can be calculated using Coulomb's law as:
With electrically conducting particles, electrostatic adhesion arises only when the particles make contacts attributable to contact potential. The adhesion force, Fel,c (N) attributable to contact potential between two rigid spheres is given by:
b. Liquid Bridges
Adhesion between two surfaces and cohesion between similar surfaces in powder material is a strong function of liquid bridges that holds the solid particles together. These liquid bridges can be divided in two major groups: mobile and immobile.
Mobile Liquid Bridges
Wet agglomerates or cakes are held together by forces originating from mobile liquid between the particles. Based on the extent of occupation of voidage by water, these bridges can further subdivided into three groups: pendular, funicular, and capillary.
Pendular State
According to Newitt and Conway-Jones (1958), the pendular state is the one in which liquid has occupied only a part of the total voidage between the particles. In this state the liquid bridges have strength resulting from the pressure drop developed through curvature of the liquid meniscus and also from the interfacial tension exerted by the liquid along the wetted perimeter. Hence, liquid bridges result in a combined pull of the solid particles by these two forces (Rumpf, 1962; Newitt and Conway-Jones, 1958; Papadakis and Bahu, 1992). Assuming perfect contact between two rigid spheres, and perfect wetting, the tensile strength, T (Pa) of agglomerate coming from pendular water is given by (Newitt and Conway-Jones, 1958):
Funicular and Capillary States
When the void space between powder particle is completely filled by water extending to the edge of the pore and forming a concave surface, a negative capillary pressure is exerted in the entire liquid space boosting the tensile strength of the wet agglomerate, which is referred to as a capillary state (Papadakis and Bahu, 1992). The tensile strength of capillary water is given by:
The funicular state is the transition between pendular and capillary state. In this state gas still occupies a small fraction in the water continuum. The tensile strength provided by the funicular water lies between those provided by the capillary and pendular water (Fig. ).
Immobile Liquid Bridges
These liquid bridges come in existence when viscous binders are introduced between the particles. The immobile liquid bridge creates a strong binding force between the particles through the viscous binder, which is far stronger than the mobile liquid bridges. Immobile liquid bridging can be observed in spray-drying. The thermoplastic materials (sugars) at or above glass transition form immobile liquid bridges ultimately leading to unwanted lumping. This type of liquid bridge retains a capacity to transform itself into solid bridges in subsequent drying. The mechanism of this type of bridge formation is viscous flow driven by surface energy (Downton et al., 1982; Wallack and King, 1988). Wallack and King (1988), through the independent measurement of sticky behavior and viscosity of sucrose/fructose mixture and instant coffee, found that Frenkel's (1945) equation relates the rate of bridge size increase and agglomerate strength to the material properties.
c. Solid Bridges
According to Rumpf (1962) agglomerates can be formed through solid bridges by: Sintering i.e., resulting from diffusion of molecules from one particle to another at the point of contact at elevated temperatures (Pietsch, 1997). Melting at the point of contact invariably leads to a bridge of molten material. In case of hard material with high melting points this bridge is localized at the roughness peaks. But, considerably large bridges are formed when a thermoplastic material moves under the influence of pressure. Crystallization of dissolved material i.e., when a moist material is granulated and then dried, the solid dissolved in the liquid crystallizes and forms solid bridges at the points of contact. The strength of bond depends on the nature of the solid and the rate of crystallization. Rapid crystallization invariably leads to small crystals with strong bonds. Dissolution and drying i.e. when the binder liquid (e.g., water) dissolves considerable amount of solids (e.g., sugars) at the time of formation of the liquid bridges, and the subsequent evaporation (drying) of the binder leads to formation of solid bridges (Newitt and Conway-Jones, 1958; Rumpf, 1962; Pietsch, 1997). Solid bridges are important during drying and production of instant food powders (Peleg, 1993; Iveson, 1997). Rumpf (1962) has also listed hardening of bonding agents and chemical reaction as important factors capable of forming solid bridges.
• | Mechanical interlocking: Fibrous, bulky, and flaky particles can interlock or fold about each other resulting in “form-closed” bonds (Pietsch, 1997). Such mechanical interlocking can be formed when temperature rises to reach a certain viscosity that causes the molecules at the interface begin to flow into each other. When the temperature decreases again, a merger can take place, which leads to the formation of mechanical interlocking/matting/meshing between the particles (Griffith, 1991). | ||||
• | Primary chemical bonds: Besides the physical bonding as discussed above scientists emphasize that if the contact distance between two particles or adhesive and adherend is smaller than 4 × 10−9 m, then chemical bonding (covalent, ionic, metallic, and hydrogen) can take place because of the primary valence forces. Cohesive (adhesive) force originated from chemical bonding is the strongest among all the bondings (Allen, 1993). The adhesion of milk protein on glass surfaces is believed to be because of the formation of chemical bonds (Matthews, 1969). |
3.2. Wetting and Thermodynamic Adsorption
The adhesion of fluids or semi-solid foods to dissimilar surfaces is not possible unless they have good spreadability or wettability to the adherend. Wettability reflects whether a liquid will spread on a surface as a continuous film or, conversely, retract as one or several drops. Good wettability means the food and the adherend have a strong mutual affinity and are likely to adhere well (Michalski et al., 1997). Complete wetting of the surface will occur when the surface energy of the adherend is greater than that of the adhesive (Saunders et al., 1992). This is attributable to the fact that low-energy materials absorb strongly to high-energy surfaces to lower the surface energy of the system.
The mechanism of thermodynamic adsorption is based on Young's force equation (Fowkes, 1964) and Dupre's energy equation (Michalski et al., 1997). Young's equation relates solid and liquid surface tensions γ s (N.m −1) and γl (N.m −1), solid-liquid interfacial tension γ sl (N.m −1) and the liquid contact angle θ at the solid /liquid/ air triple line. Similarly, Dupre's equation relates adhesive and adherend surface tensions to the work of adhesion, W a (J.m−2) (Equation Equation9).
Previously, an objection in the use of this theory was that the work of adhesion, when measured experimentally was found to be substantially higher compared with the same calculated theoretically (Michalski et al., 1997). But it was found that the difference was attributable to viscoelastic dissipation occurring at the site of adhesive fracture (Shanahan and Carre, 1995). Spreading of liquid on a viscoelastic solid causes dissipation through the formation of a wetting ridge on the solid at the triple line. The work of adhesion is now recognized as a function of the reversible work of adhesion and the irreversible deformation of the substrate (Shanahan and Carre, 1995; Michalski et al., 1997).
3.3. Tack and Sample Rheology
The energy required to separate two objects that are not permanently bound together is a measure of tack (Russel and Kim, 1999). Tackiness is the word often applied to characterize the surface adhesion of sticky material. As early as 1941 Green outlined tack as pull resistance between two surfaces and stated that cohesion, surface tension, and viscosity all contribute to the tack (Green, 1941). Naturally wettability and surface energies are important contributions to adhesion, but the time taken by a fluid or semi-solid food to wet the surface is influenced by viscosity. A material is sticky when the energy required to break its bond with a surface is as large as the interfacial energy that comes from the dissipation during the separation process, which in turn causes deformation and friction in the contact film. If a good contact is to be established between two surfaces, some softness of the material is essential. Hence, a material is tacky when it possesses a right balance between softness and ability to dissipate energy (Crevoisier et al., 1999). Wettability on the other hand, is related to the interfacial energy of a material. Green (1942) had expressed tack (N) as:
Foods are pressure-sensitive and may behave in a similar manner to pressure-sensitive adhesives. First, the greater the force used to apply the probes (Section 4.4 deals more with probes) to the surface of the food, the greater the tackiness will be (Hoseney and Smewing, 1999). Second, the force required to remove the probe (tackiness) is proportional to the rate at which it is withdrawn (Chen and Hoseney, 1995). Boyd and Sherman (1975) observed that subjective stickiness is analogous to the high crosshead speeds of Instron testing machine. Stickiness of sugar syrups was investigated by Brennan and Mohamed (1984) who found that viscosity and surface tension were directly proportional to the stickiness.
Not only is the bonding between adhesive and adherend essential for stickiness, but the mechanism of failure of this bond is also equally significant (Fig. ). The clean failure of adhesive and the adherend surface is termed adhesive failure while the failure within the adhesive with residue on the adherend surface is known as cohesive failure (Kilcast and Roberts, 1998). The adhesive industries have been able to successfully manipulate the failure mechanism so that only adhesive failure takes place. Cohesive failure may be dominant mode of failure where stickiness of the food is concerned.
Figure 3. Schematic presentation of failure mechanism between adhesive and adherend surfaces (adapted from Kilcast and Roberts, 1998).
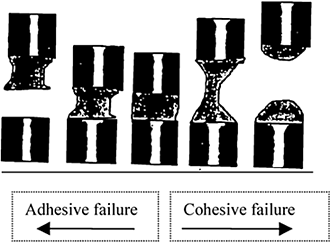
Research on pressure-sensitive adhesives has shown that the mode of failure depends on the rate of separation and the temperature. Higher rate of separation of the surfaces favors the adhesive failure while the slow rate the cohesive one (Kambe and Kamagata, 1969). It was also found that transition from adhesive to cohesive failure takes place when one moves toward the phase transition (Satas, 1989). Cereal scientists have noted similar effect of rate and temperature in failure mechanism. They further found that increase in moisture favors cohesive failure (Dhaliwal et al., 1990).
3.4. Glass Transition Approach
Recently glass transition temperature (Tg ), the temperature at which the switch between rubbery and glassy phases takes place, is being recognized as a fundamental parameter to explain and control stickiness (Roos and Karel, 1991; Bhandari and Howes, 1999) for amorphous and semicrystalline foods particularly in the case of low molecular weight carbohydrates.
The glass transition temperature is a fundamental property of a amorphous material. When a glassy material is subjected to moisture and heat and subsequently approaches the phase transition, the viscosity of the system decreases dramatically from 1012–14 Pa.s to 106–8 Pa.s. The lowered viscosity is unable to support the glassy microstructure giving way to structural collapse of freeze-dried products and stickiness of spray-dried ones (Wallack and King, 1988; Tsourouflis et al., 1976; Flink, 1983). If the glass transition temperature of a powder is lower than the storage temperature, the phenomena of stickiness, caking, and unwanted agglomeration becomes unavoidable.
Figure 4. Plots (semi-logarithmic) of sticky point temperature (Tst ) and glass transition temperature (Tg ) with moisture content for low moisture sucrose/fructose (7:1); data taken from Downton et al. (1982) and Roos and Karel (1991b). Solid line (_____) indicates variation of sticky point temperature with moisture content (dry basis), Dotted line (----) indicates variation of glass transition temperature with moisture content (db).
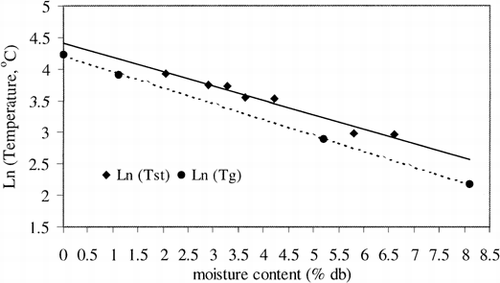
The literature values of sticky point temperature and glass transition temperature for low-moisture content sucrose: fructose (7:1) mixture has been shown in Figure . It can be seen that the semilogarithmic plots of sticky point temperature and glass transition temperature with moisture content run almost parallel to each other; the sticky point temperature is almost 4–11°C higher than the glass transition temperature (Tg end). Hence, glass transition temperature can be used as a reliable indicator of stickiness.
It has been shown that it is possible to switch on or off stickiness (Crevoisier et al., 1999). It is generally noticed that surface properties of a polymer change abruptly when it changes from structured smectic phase to disordered isotropic phase. This change in the surface properties is brought about by the bulk properties (e.g., viscosity) through the change of phase which can be manipulated for tuning of stickiness at will (Crevoisier et al., 1999). These authors also found that for a copolymer obtained by copolymerization of an acrylate monomer bearing a long perfluoro alkyl side chain (C2H4-C8F17) and a methacrylate monomer bearing a long alkyl chain (C17H35) the tack energy was zero at the smectic phase, while it was 50 J/m2 at the switch temperature (37°C) and decreased with increasing temperature. The surface wetting increased from 10% at smectic phase to nearly 100% in the isotropic phase. It is not difficult to see that thermodynamic and bulk properties of a substance undergo a sharp change at or around glass transition temperature. The sharp drop in viscosity because of the glass transition is responsible for rendering the product tacky or sticky. Hence, the glass transition concept can be successfully used as an approach for characterizing stickiness.
4. TEST METHODS
A brief review of various test methods that have been adapted for characterization of food stickiness is presented in this section. A subjective comparison of these methods in terms of the degree of automation, extent of application, and ability of the same to quantify stickiness is presented in Table .
Table 2. Comparison of Various Test Methods Currently Used to Assess Food Stickiness
4.1. Shear Cell Method
This method is specifically applied for quantification of cohesion and adhesion of powder or granular materials. Flowability of a powder is the ease at which it flows through a chute or hopper. And, it is possible to determine the flowability of a powder using shear cells such as the Jenike shear cell (Jenike, 1964) or rotational split-level shear cell (Peschl, 1989). Schematic diagrams of these shear testers are presented in Figure . A shear cell is a box-like structure that is split in half horizontally and is equipped with a provision of applying various normal stresses (σ) and shear stresses (τ) on the top of it. The powder to be tested is preconsolidated with the maximum applicable consolidation load. If a sample is tested at different normal load, one can obtain a particular shear stress that causes failure (initiation of flow). When the shear stress is plotted as a function of consolidation (normal) stress, a failure curve is obtained which is known as yield locus curve (Fig. ).
Figure 5. Schematic diagram of a) split type rotational shear cell and b) Jenike shear cell used for measuring cohesive and adhesive force of particulates (adapted from Peschl (1989) and Jenike (1962), respectively).
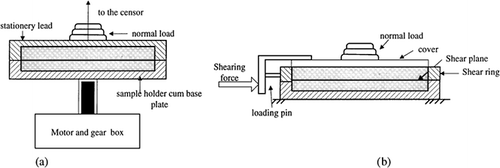
Figure 6. Yield loci of noncohesive a) and cohesive b) powders. OC represents the cohesive force and OT represents the tensile strength (adapted from Peleg, 1993; Peschl, 1989).
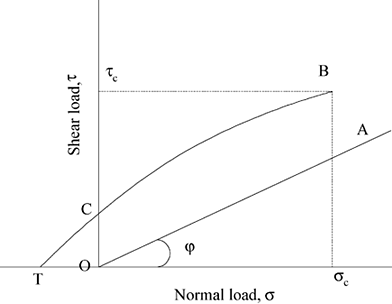
Figure shows the yield loci of two samples. The yield loci of the sample A passes through the origin signifying that the shear stress of a free-flowing powder at zero consolidating load is zero. The yield locus of sample B doesn't pass through the origin, which means that for such powders it is essential to apply a finite shearing stress to cause flow. The intercept OC is known as cohesive force. The higher the force of cohesion, the less free-flowing the powder becomes. It has been found that a cohesive food powder has a cohesion of the order of 785–1177 Pa (determined under the consolidation load of 9.81 kPa) (Peleg, 1993).
The flowability index of a powder is extracted from information obtained through its yield locus and is expressed as a function of unconfined yield strength (fc ) and major and minor consolidation stresses (Fig. and ). Peschl (1989) defined absolute flowability (dimensionless) based on Jenike's approach, which is a function of major and minor consolidation loads, unconfined yield strength, and specific gravity of the powder thereby making it possible to compare the extent of flowability of different powders through the same parameter (Equation Equation11).
Figure 7. Schematic diagram of Mohr Circles. σ1 and σ2: major and minor consolidating stresses, fc : unconfined yield strength, OC and OT: cohesive force and tensile strength of the powder, respectively (adapted from Peschl, 1989).
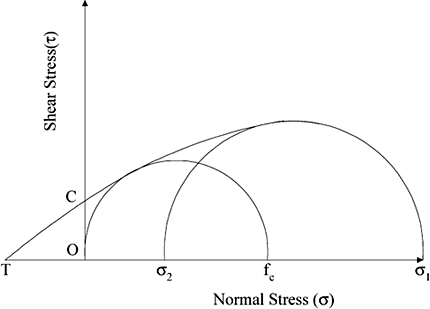
This method is successfully adapted by industries for characterizing the flow behavior of powders through chutes and hoppers. It has also been widely used to characterize the caking properties of the dry particulates. However, it is confined to dry particulate systems. It is not possible to use the shear cells for semi-solid foods. Furthermore, it is very hard to adapt it to high temperature and high humidity systems.
4.2. Sticky Point Temperature Method
A sticky point tester was developed by Lazar et al. (1956) to assess the effects of temperature on the powder and subsequently modified and used by Downton et al. (1982) and Wallack and King (1988). The sticky point tester used by Wallack and King (1988) consists of a test tube in which a powder with known moisture content is placed. This test tube can be rendered airtight using a rotating mercury seal. The tube is then immersed in a controlled temperature bath and the bath temperature is raised slowly. The powder within the test tube is intermittently stirred either by hand or through a machine-driven impeller that is imbedded in the sample. When temperature is slowly raised, a temperature is reached at which the force experienced to drive the impeller is maximum; this is called sticky point temperature. The sticky point temperature is a function of moisture content. This test is repeated for other moisture contents and corresponding sticky points are determined. Finally, the sticky point temperatures are plotted as a function of moisture content. The drying industry uses this sticky point versus moisture content curve to identify a safe drying regime. Schematic diagram of this sticky point tester is provided in Figure . Recently additional improvements to this tester by introducing double and curved blades have been reported (Pasley and Haloulos, 1994).
Figure 8. Schematic diagram of sticky point testing apparatus (adapted from Wallack and King, 1988).
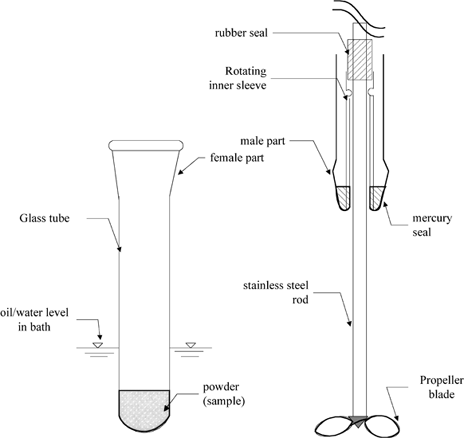
Although the information based on this test is very important for determination of safe drying regime, it fails to provide information regarding the stickiness status (flowability status) of a powder below the sticky point temperature (Peleg, 1993). Its use is confined to empirically determine the safe drying regime, especially in spray-drying operations.
4.3. Optical Probe Method
An instrument using an optical probe was recently reported by Lockemann (1999). This method exploits the change in optical properties of a free-flowing powder when its flow pattern gets altered. A free-flowing sample is filled in a glass test tube. This test tube is mounted to a rotating drive tilted at a certain angle. A thermometer is introduced inside the test tube to monitor the sample temperature. The test tube is then immersed in temperature programmable oil bath. The temperature is slowly raised and the test tube is rotated at a slow speed. The sample gets continuously tumbled within the test tube.
The motion of the test sample is monitored and recorded with the help of an optical fiber sensor that has combined illuminator-receiver system. The reflectance of light from the sample surface is continuously recorded. The sticky point temperature is the one in which the flow pattern of the sample undergoes a qualitative change. The signal recording system gives a sharp rise in reflectance, which is indication of the sticky point. Schematic diagram of this instrument is provided in Figure .
Figure 9. Schematic diagram of optical probe used for sticky point determination free-flowing particulates (Adapted from Lockemann, 1999). 1, illuminator-receiver; 2, light pipe; 3, fiber-optic sensor; 4, test-tube; 5, oil or water bath (oil or water); 6, rotating drive; 7, temperature probe; and 8, tube mount.
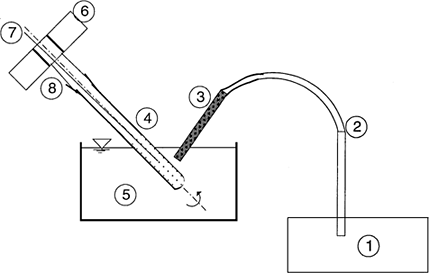
This instrument is novel and may prove very useful for online monitoring of stickiness in powders as a function of moisture and temperature. So far, it has only been used to determine the sticky point temperature of solid β-carotene. It needs to be tested with particulates that tend to be transparent on softening and melting. Like other sticky point temperature methods it can not be used to quantify the stickiness of a powder system below the sticky point temperature.
4.4. Tackmeter Method
The tack test involves the contact of a probe with adhesive material and measuring the tensile strength of the bond. One of the earliest tackmeters was used to study the tackiness of different inks applied on top of the other. A probe is brought in contact with the ink surface and subsequently pulled back and the force needed to separate the probe from the ink surface and the time taken for failure to occur is recorded (Green, 1941). Schematic diagram of Green's type tack meter is presented in Figure .
Figure 10. Schematic diagram of tack meter used for measurement of ink tackiness (Adapted from Green, 1941). a) 1, constant temperature bath; 2, sample holder; 3, tack probe; 4, support; 5, probe holder lever; 6, position knob; 7, load lever; 8, loads; and 9, stand. b) tack probe in action.
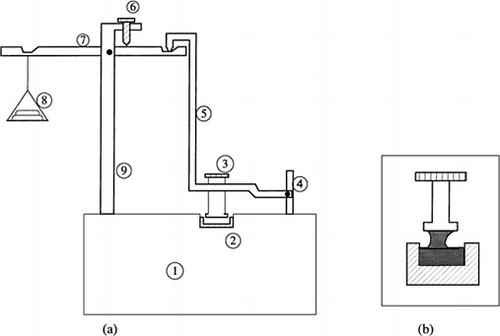
Research on pressure-sensitive adhesives has made intensive use of flat-faced probes. During the separation of probe from adhesives force and time of separation are recorded and area under the curve is determined (tack) (Kilcast and Roberts, 1998). The tack values of doughs have been found to increase with both the contact force and contact time. The increase in contact force, rate of withdrawal and contact time all seem to have positive correlation with tack energy (Saunders et al., 1992). The increase in temperature casts profound influence both in tack and the failure mechanisms.
Special tackmeters (such as: TA.XT2, Stable Micro Systems, England) have been developed (Chen and Hoseney, 1995) and widely being used to measure wheat dough stickiness and can work in a lower load range. However, the use of tackmeters has been confined to semi-solid foods and paste systems.
4.5. Peel Method
Peel tests have often been used for testing the strength of adhesives. Two peeling modes (i.e., 90 and 180°) are most often used for this purpose. The 180° peeling mode is easier to perform but if the adhesives are sensitive to bend- ing, structural damage may occur when the adhesive bends through 180°. A bend test through 90° requires a relatively complex holding system that maintains the peeling angle at 90° throughout the experiment (Kilcast and Roberts, 1998).
These peeling tests are less applicable to quantify the food adhesion. Rosinski et al. (1989) used 180° peeling test to study the adhesion of meat on packaging surface using an Instron-testing machine and reported that the degree of adhesion of meat on different can be determined. These peel tests are mainly confined to adhesive and tape industries and seem unlikely to be embraced by the food industries.
4.6. Weighing
Weighing of the adhered food materials on a surface is the simplest way of assessing stickiness. Adhesion of oils and emulsions on various packaging materials and surfaces like stainless steel and glasses have been studied by Michalski et al. (1999). An inclined surface was designed and the samples were allowed to flow down the inclined surfaces on which adhesion was being studied. It was found that both the viscosity of the foods and the surface engergies of the adherend influenced the amount of food adhesion (Michalski et al., 1999).
Although this test method is simple, it is difficult to say quantitatively which force, adhesive or cohesive, is dominant. A monolayer of food sticks on the adherend surface because of adhesive force and the remaining mass may simply remain there because of the cohesive force.
4.7. Glass Transition Temperature Method
The sticky point test described before at first instance appears to be somewhat empirical in nature. But, in reality, it is based on the softening of powders above their glass transition temperature when the glassy phase yields to a rubbery one. From the work of Schubert (1987) it is known that the magnitude of Van der Waals and electrostatic attraction is not as high as the attraction force that comes from liquid bridges. According to Rumpf (1962) liquid bridges offer strong bonding that has a significant practical significance in particulate aggregates having a size of 1 mm or less. Hence, formation of liquid bridges should be prevented if caking and sticking of powders is to be avoided. In reality, liquid bridging can only occur when at least some of the particle surface is viscous (or if there is a presence of an external moisture or a second liquid phase). The surface of particle can become viscous either because of the adsorption of moisture from the surroundings or plasticization by the moisture already present. The plasticization of the particle surface accelerates once the glass transition temperature is exceeded (Peleg, 1993).
Work by Slade et al. (1993) explains that unwanted agglomeration and caking of food powders in storage can be avoided simply by storing them below their glass transition temperature provided that adsorption of moisture from the environment is avoided. The sticky problem in drying can best be explained from the notion of glass transition. By determining the glass transition temperature of a system, it is possible to predict a bulk temperature and moisture matrix, which renders the product sticky. Furthermore, a safe drying regime can be designed by manipulating the glass transition temperature by simply controlling the drying parameters or introducing some drying aids.
The instruments that are used to measure the glass transition temperature are based on three basic measurement techniques viz. calorimetric, molecular mobility, and mechanical-thermal (Blanshard, 1993). Calorimetric methods make use of changes in specific heat capacity (Cp ). The differential scanning calorimeter (DSC) is extensively used to determine Tg of food materials. A detailed treatment of measurement of Tg using DSC is found elsewhere (Rahman, 1995). Despite the high performance of DSC in food systems, it has been reported that with increasing molecular weight and structural complexity, the measurement of T g with DSC becomes more problematic (Blanshard, 1993). A molecular mobility technique uses NMR (nuclear magnetic resonance) to track the phase change. The merit of NMR method lies in the fact that it can give not only information regarding mobility of the entire molecule, but also the mobility of individual groups or region within a food structure (Blanshard, 1993). Mechanical-thermal methods measure the changes in loss modulus, elastic modulus, and their ratio as a function of temperature. A sharp change in these properties is indicative of glass transition temperature (Rahman, 1995).
Although the glass transition temperature is a fundamental property directly related to food stickiness, techniques to measure the Tg itself require complex instruments and a high level of professional expertise. Current research on glass transition temperature shows that it may be possible to quantify stickiness in a broad range of food products. But, to this date, it has only been used to characterize low molecular weight carbohydrate systems. There is a real need to develop simple methods and instruments for measuring glass transition temperature for foods.
4.8. Other Methods
The Instron universal tester has been used by some researchers to study the stickiness of sugar syrups (Brennan and Mohamed, 1984). It involves the contact of crosshead or crosshead attached to a flat plate (resembling a flat-faced tackmeter) to a food surface at a low speed. It is then allowed to rest for some time and finally withdrawn from the food surface at greater speed. The force required for separating the crosshead from the food sample correlates with stickiness. Juliano (1985b) used an Instron for characterization of stickiness of cooked rice. Kumar et al. (1976) studied the stickiness of cooked rice by shaking and tapping a sieve with a suitable opening and allowing the cooked rice to pass through the sieve opening. The higher the amount of rice retained on the sieve, the greater the stickiness. A Tensipresser is used for characterization of stickiness in waxy and nonwaxy rice having various amylose contents (Okadome et al., 1999). Through its use, it is reported that the waxy rice (0.2–1.5% amylose by dry basis) is correlated highly with adhesiveness (stickiness). Furthermore, a salient feature of this device is that it is able to differentiate the decrease in stickiness of cooked rice with gradual increase in amylose content and also that surface stickiness of a single kernel of cooked rice can be measured with ease (Okadome et al., 1999). The working principle of this device is similar to a texturometer or an Instron universal tester.
Contact angle is the measure of wettability and it can ultimately be linked with the degree of adhesion on a surface. For good adhesion, the contact angle of the fluid and the rigid phase must be zero, or nearly so. The contact angle is, thus, a useful inverse measure of the wettability of a surface, in the sense that smaller contact angles are obtained with highly (or easily) wettable surfaces. Furthermore, lower the contact angles and higher the wettability more force will be required to separate these surfaces (Baier, 1970). The tilted plane technique of contact angle measurement was adapted by Lai (1986) to measure the extent of stickiness of the food products such as salad dressing and tomato sauce on various packaging materials.
5. EXAMPLES OF TECHNIQUES FOR MINIMIZING STICKINESS
5.1. Spray-Drying Operations
Various methods have been used to overcome the sticky problem in spray-drying operations; some common ones are given below.
• | Introduction of cold air: Cold air is introduced at the bottom of a drying chamber (Lazar et al., 1956). A limited amount of air can only be introduced because the cooling process increases the relative humidity of the air, which is capable of reviving the sticky problem again (Bhandari et al., 1997a). | ||||
• | Control of wall temperature: Application of this technique to orange juices, black currants, and raspberries (Bhandari, et al., 1993) are reported to minimize the wall deposition. High wall temperature (>sticky point) not only causes stickiness and wall deposition of thermoplastic powders but also discoloration, self-heating and burning of deposited powder. However, low wall temperature (<sticky point) leads to heat loss and underdried products. | ||||
• | Scraped surface drying chamber: It has been used by Karatas and Esin (1990) for tomato paste to continuously remove the wall depositions that improved the yield and quality. | ||||
• | Low temperature drying (Birs dryer): Air of 30°C and 3% relative humidity is used in association with a very large spray-drying tower. This is a humidity-driven rather than temperature-driven process. Today this dryer is no longer in use (Hayashi, 1989). | ||||
• | Drying aids: Addition of high molecular weight additives, in the feed, before drying has long been practiced. The additives raise the glass transition temperature of the drying particle and also reduce hygroscopicity and thermoplasticity of the resultant mixture. Maltodextrin has been used widely for many juices such as orange (Gupta, 1978), black currants, and raspberry (Bhandari et al., 1993), honey, and pineapple (Bhandari et al., 1997b). The use of additives is the most common way of spray-drying sugar-rich foods. | ||||
• | Control of Drying Parameters: Based on the knowledge of glass transition temperature and drying kinetics (drying rate, particle temperature, and skin formation etc.), it is possible to define a safe drying regime at which a successful drying operation can be accomplished. Fundamental studies to optimize the drying operation as a function of glass transition temperature and drying parameters are still awaited (Truong et al., 1999). |
5.2. Storage of Food Powders
• | Storage below Tg : The surface of food powders is amorphous in nature. Also, the surface of crystalline particles becomes amorphous when it is finely divided. These particulates undergo a phase change when they reach Tg . Moisture acts as a plasticizer effectively depressing the glass transition temperature. The combined effect of temperature and moisture of a food powder and its surrounding is responsible for stickiness and caking. Hence, storage of the food powders below their Tg is recommended to avoid stickiness and caking during storage and transportation (Roos and Karel, 1991; Peleg, 1993). The temperature during storage and transportation frequently jumps above the Tg and makes the foods quite vulnerable to stickiness and unwanted agglomeration. Regarding food powders with high-fat content, it is desirable to store them below the melting point of the fat to prevent fat induced caking. | ||||
• | Addition of flow conditioners: Flow conditioners and anticaking agents are chemically (or practically) inert, finely divided particulates that are added to host particles to improve their flowability or tendency to cake. These conditioners are effective when added up to 2%. The main food grade conditioners are silicon dioxide, silicates, phosphates, salts of stearic acid, starches, and modified carbohydrates (Peleg et al., 1973; Peleg and Hollenbach, 1984). Flow conditioners inhibit the caking of food powders and improve the flowability of host powders through various mechanisms including physical separation, interruption of liquid bridges, lubrication, competitive adsorption of water (increased availability of sites for water sorption through coating action) and cancellation of molecular and electrostatic charges (Peleg and Mannheim, 1977; Peleg and Holenbach, 1984; Lai et al., 1999). They are effective, partly, because of their low particle size (<5 μm). Except the chemicals mentioned above various substances like oils in raisins (Kostaropoulos et al., 1997) and low DE maltodextrin in sugars (Nisshin, 1981) etc are also recommended as anti-caking or antisticking agents. Commonly used flow conditioners in food systems are listed in Table . |
Table 3. Commonly Used Flow Conditioners and Anticaking Agents in Food System
5.3. Dough and Cooked Rice Stickiness
It has been reported that ferulic acid esterified to a mixed-linkage β-glucan chain of about 71 anhydroglucose units is a major cause of dough stickiness. This compound is a low molecular entity and is water-soluble (Huang and Hoseney, 1999). It can be inferred that saponification of ester bond of this compound and increase of water-insoluble proteins can lower the dough stickiness. Furthermore, shortening of the dough mixing time and reducing the amount of water required can minimize the stickiness of dough.
Because high amylopectin/amylose ratio is associated with cooked rice stickiness, selection of rice with desired amylose content or rice with a desired amylopectin/amylose ratio will be a better way for ensuring consumer satisfaction. Furthermore, any means of reducing water requirements or minimizing the cooking time may be conducive for minimizing rice stickiness.
6. CONCLUSIONS
Stickiness is a prevalent problem in food processing operations that causes reduced product recovery, operational problems, wearing-out of equipments, frequent plant shutdowns, and fire hazards. Literature on the causes, mechanisms, and testing methods of food stickiness has largely been product-specific. No single mechanism or test method has been successful enough to generalize and characterize the problem of food stickiness. Because all the mechanisms and test methods have been limited in one or two products, glass transition temperature-based mechanism and test methods seem to be generally applicable where the stickiness and caking of low molecular weight carbohydrate system are involved.
Acknowledgments
REFERENCES
- Angell , C. A. , Stell , R. C. and Sichina , W. 1982 . Viscosity-Temperature Function for Sorbital from Combined Viscosity and Differential Scanning Calorimetry Studies . J. Physical Chem. , 86 : 1540 – 1542 .
- Allen , K. W. 1993 . Current Theories of Adhesion and Their Relevance to Adhesive Technology . J. Physics IV , 3 : 1511 – 1516 .
- Atkins , A. G. 1987 . “ The Basic Principles of Mechanical Failure in Biological Systems ” . In Food Structure and Behavior Edited by: Blanshard , J. M.V. and Lillford , P. J. 149 – 176 .
- Baier , R. E. 1970 . “ Surface Properties Influencing Biological Adhesion ” . In Adhesion in Biological Systems Edited by: Manly , R. S. 15 – 45 . New York : Academic Press .
- Bhandari , B. R. , Senoussi , A. , Dumoulin , E. D. and Lebert , A. 1993 . Spray Drying of Concentrated Fruit Juices . Drying Technol. , 11 ( 5 ) : 1081 – 1092 .
- Bhandari , B. R. , Datta , N. and Howes , T. 1997a . Problems Associated with Spray Drying of Sugar-Rich Foods . Drying Technol. , 15 ( 2 ) : 671 – 684 .
- Bhandari , B. R. , Datta , N. , Crooks , R. , Howes , T. and Rigby , S. 1997b . A Semi-Empirical Approach to Optimize the Quantity of Drying Aids Required to Spray Dry Sugar-Rich Foods . Drying Technol. , 15 ( 10 ) : 2509 – 2525 .
- Bhandari , B. R. and Howes , T. 1999 . Implication of Glass Transition for the Drying and Stability of Foods . J. Food Eng. , 40 ( 1–2 ) : 71 – 79 .
- Blanshard , J. M.V. 1993 . “ The Glass Transition, its Nature and Significance in Food Processing ” . In Glassy State in Foods Edited by: Blanshard , J. M.V. and Lillford , P. J. 18 – 48 . England : Nottingham University Press .
- Boyd , J. V. and Sherman , P. 1975 . The Mechanics of Stickiness Evaluation in Foods. A Comparison of Oral and Instrumental Methods of Evaluation in Selected Foods . Biorheology , 12 : 317 – 322 .
- Brennan , J. G. , Herrera , J. and Jowitt , R. 1971 . A Study of Some of the Factors Affecting the Spray Drying of Concentrated Orange Juice, on a Laboratory Scale . Food Technol. , 6 : 295 – 307 .
- Brennan , J. G. and Mohamed , A. M.A. 1984 . “ Relating Sensory Stickiness and Physical Properties of Foods ” . In Engineering and Food Edited by: MacKenna , B. M. Vol. 1 , 489 – 498 .
- Buma , T. J. 1971 . Free Fat in Spray Dried Whole Milk 5. Cohesion: Determination, Influence of Particle Size, Moisture Content and Free Fat Content . Netherlands milk Dairy J. , 25 : 107 – 122 .
- Burton , H. 1968 . Deposits From Whole Milk in Heat Treatment Plant-A Review and Discussion . J. Dairy Res. , 35 : 317 – 330 .
- Chen , J. J. , Lu , S. and Lii , C. Y. 1999 . Effect of Milling on the Physico-Chemical Characteristics of Waxy Rice in Taiwan . Cereal Chem. , 76 ( 5 ) : 796 – 799 .
- Chen , W. Z. and Hoseney , R. C. 1995 . Development of an Objective Method for Dough Stickiness . Lebensm.-Wiss.-u.-Technol. , 28 ( 5 ) : 467 – 473 .
- Chuy , L. E. and Labuza , T. P. 1994 . Caking and Stickiness of Dairy-Based Food Powders as Related to Glass Transition . J. Food Sci. , 59 ( 1 ) : 43 – 46 .
- Ciz-K . 1973 . Use of Starch for Preventing Agglomeration of Icing Sugar . Industries-Alimentaires-et-Agricoles , 90 ( 7/8 ) : 969 – 972 .
- Crevoisier , G. , Fabre , P. , Corpart , J-M. and Leibler , L. 1999 . Switchable Tackiness and Wettability of a Liquid Crystalline Polymer . Science , 285 : 1246 – 1249 .
- Dhaliwal , A. S. , Mares , D. J. and Marshall , D. R. 1990 . Measurement of Dough Surface Stickiness Associated with the 1B/1R Chromosome Translocation in Bread Wheats . J. Cereal Sci. , 12 ( 2 ) : 113 – 122 .
- Downton , G. E. , Flores-Luna , J. L. and King , C. J. 1982 . Mechanisms of Stickiness in Hygroscopic, Amorphous Powders . Ind. Eng. Chem. Fundam. , 21 ( 4 ) : 447 – 451 .
- Flink , J. M. 1983 . “ Structure and Structure Transitions in Dried Carbohydrate Materials ” . In Physical Properties of Foods Edited by: Peleg , M. and Bagley , E. B. 473 – 521 . Westport, CT : AVI .
- Fowkes , F. M. 1964 . Attractive Forces at Interfaces . Ind. Eng. Chem. , 56 ( 12 ) : 40 – 52 .
- Fox , T. G. and Flory , P. J. 1950 . Second Order Transition Temperatures and Related Properties of Polystyrene . J. Appl. Phys. , 21 : 581 – 591 .
- Frenkel , J. 1945 . Viscous Flow of Crystalline Bodies under the Action of Surface Tension . J. Phys. (USSR) , 9 ( 5 ) : 385 – 391 .
- Gay , C. and Leibler , L. 1999 . On Stickiness . Phys. Today , 52 ( 11 ) : 48 – 52 .
- Girifalco , L. A. and Good , R. J. 1957 . A Theory for the Estimation of Surface and Interfacial Energies. I. Derivation and Application to Interfacial Tension . J. Phys. Chem. , 61 ( 7 ) : 904 – 909 .
- Graybosch , R. A. , Peterson , C. J. , Hansen , L. E. , Worrall , D. , Shelton , D. R. and Lukszewski , A. 1993 . Comparative Flour Quality and Protein Characteristics of 1BL/1RS and 1AL/1RS Wheat Rye Translocation Lines . J. Cereal Sci. , 17 ( 2 ) : 95 – 106 .
- Green , H. 1941 . The Tackmeter, an Instrument for Analyzing and Measuring Tack . Ind. Eng. Chem., Anal. , 13 : 632 – 639 .
- Green , H. 1942 . What is Tack? . Paper Trade J. , 114 ( 6 ) : 39 – 42 .
- Griffith , E. J. 1991 . Cake Formation in Particulate Systems Weinheim, , Federal Republic of Germany : VCH Publishers, Inc. . D-6940
- Gupta , A. S. Spray Drying of Orange Juice . US Patent 4,112,130 . 1978 .
- Hamano , M. and Aoyama , Y. 1974 . Caking Phenomena in Amorphous Food Powders . Trends Food Sci. Technol. , 6 : 149 – 155 .
- Hammond , F. H. 1989 . “ Tack ” . In Handbook of Pressure Sensitive Adhesive Technology Edited by: Satas , D. 38 – 60 . New York : Chapman and Hall .
- Hegg , P. H. , Castberg , H. B. and Lundh , G. 1985 . Fouling of Whey Proteins on Stainless Steel at Different Temperatures . J. Dairy Res. , 52 : 213 – 218 .
- Hayashi , H. 1989 . Drying Technologies of Foods—Their History and Future . Drying Technol. , 7 ( 2 ) : 315 – 369 .
- Henry , R. J. , Martin , D. J. and Stewart , B. G. 1989 . Cell-Wall Polysaccharides of Rye-Derived Wheats: Investigations of the Bio-chemical Causes of Dough Stickiness . Food Chem. , 34 ( 4 ) : 309 – 316 .
- Hoseney , R. C. and Smewing , J. 1999 . Instrumental Measurement of Stickiness of Doughs and Other Foods . J. Texture Stud. , 30 : 123 – 136 .
- Huang , W. N. and Hoseney , R. C. 1999 . Isolation and Identification of Wheat Flour Compound Causing Sticky Dough . Cereal Chem. , 76 ( 2 ) : 276 – 281 .
- Iveson , S. 1997 . Fundamentals of Granule Consolidation and Deformation , PhD Thesis St. Lucia, , Australia : Department of Chemical Engineering, The University of Queensland .
- Jenike , A. W. 1964 . Storage and Flow of Solids , Bulletin 123 Utah Engineering Experimental Station, University of Utah .
- Jowitt , R. 1974 . The Terminology of Food Texture . J. Texture Stud. , 5 : 351 – 358 .
- Juliano , B. O. 1985a . “ Polysaccharides, Proteins, and Lipids of Rice ” . In Rice Chemistry and Technology Edited by: Juliano , B. O. 59 – 174 . St. Paul, Minnesota : American Association of Cereal Chemists, Inc. .
- Juliano , B. O. 1985b . “ Criteria and Tests for Rice Grain Qualities ” . In Rice Chemistry and Technology Edited by: Juliano , B. O. 443 – 524 . St. Paul, Minnesota : The American Association of Cereal Chemists, Inc. . 1985b
- Juliano , B. O. 1993 . Rice in Human Nutrition. FAO Food and Nutrition Series, 26 Rome : Food and Agriculture Organization of the United Nations .
- Kambe , H. and Kamagata , K. 1969 . A Method of Measuring Tackiness . J. Appl. Polym. Sci. , 13 : 493 – 504 .
- Karatas , S. and Esin , A. 1990 . A Laboratory Scrapped Surface Drying Chamber for Spray Drying of Tomato Paste . Lebensm-Wiss-u-Technol. , 23 ( 4 ) : 354 – 357 .
- Kelley , F. H.C. , Mak , F. K. and Shah , D. 1974 . Some Hygroscopic Properties of Refined Sugar . Int. Sugar J. , 76 ( 912 ) : 361 – 363 .
- Kilcast , D. and Roberts , C. 1998 . Perception and Measurement of Stickiness in Sugar-Rich Foods . J. Texture Stud. , 29 ( 1 ) : 81 – 100 .
- Kokini , J. L. , Poole , M. , Mason , P. , Miller , S. and Stier , E. F. 1984 . Identification of Key Textural Attributes of Fluid and Semi-Solid Foods using Regression Analysis . J. Food Sci. , 49 ( 1 ) : 47 – 51 .
- Kostaropoulos , A. E. , Mandala , J. , Spiess , W. E.L. and Saravacos , G. D. 1997 . Factors Influencing the Friction of Raisins during Processing and Handling . J. Food Eng. , 33 : 385 – 393 .
- Kumar , B. M. , Upadhyay , J. K. and Bhatacharya , K. R. 1976 . Objective Tests for the Stickiness of Cooked Rice . J. Texture Stud. , 7 : 271 – 278 .
- Lai , C. C. 1986 . “ Sticky Problems in Food Packaging ” . In Food Product-Package Compatibility Proceedings Edited by: Gray , J. I. , Harte , B. R. and Miltz , J. 258 – 269 . East Lansing, MI : School of Packaging, Michigan State University .
- Lai , C. C. , Gilbert , S. G. and Mannheim , C. H. 1999 . Effect of Flow Conditioners on Water Sorption and Flow Properties of Egg Powder . J. Food Eng. , 5 : 321 – 333 .
- Lazar , W. E. , Brown , A. H. , Smith , G. S. and Wong , F. F. 1956 . Lindquist F.E. Experimental Production of Tomato Powder by Spray Drying . Food Technol. , : 129 – 134 .
- Lloyd , R. J. , Chen , X. D. and Hargreaves , J. B. 1996 . Glass Transition and Caking of Spray Dried Lactose . Int. J. Food Sci. Technol. , 31 : 305 – 311 .
- Lockemann , C. A. 1999 . A New Laboratory Method to Characterize the Sticking Property Free Flowing Solids . Chem. Eng. Process , 38 : 301 – 306 .
- Marshall , A. D. and Daufin , G. 1985 . “ Physico-Chemical Aspects of Membrane Fouling by Dairy Fluids ” . In Fouling and Cleaning in Pressure Driven Membrane Processes 8 – 35 . International Dairy Federation .
- Martin , D. J. and Stewart , B. J. 1986 . Dough Mixing Properties of a Wheat-Rye Derived Cultivar . Euphytica , 35 : 225 – 232 .
- Matthews , D. B. 1969 . Electrochemical Studies on the Adsorption of Amino Acids in Relation to Compatibility of Prosthetic Materials and Human Body . J. Biomed. Material Res. , 3 : 475 – 496 .
- Michalski , M. C. , Desobry , S. and Hardy , J. 1997 . Food Material Adhesion: A Review . Crit. Rev. Food Sci. Nutr. , 37 ( 7 ) : 591 – 619 .
- Michalski , M. C. , Desobry , S. , Babak , V. and Hardy , J. 1999 . Adhesion of Food Emulsions to Packaging and Equipment Surfaces. Colloids Surf., A: Physicochem . Eng. Aspects , 149 : 107 – 121 .
- Newitt , D. M. and Conway-Jones , J. M. 1958 . A Contribution to the Theory and Practice of Granulation . Trans. Ins. Chem. Eng. , 36 : 422 – 441 .
- Nisshin Seito , K. K. Japanese Patent 5,646,760 . 1981 .
- Okadome , H. , Toyoshima , H. and Ohtsubo , K. 1999 . Multiple Measurements of Physical Properties of Individual Cooked Rice Grains with a Single Apparatus . Cereal Chem. , 76 ( 6 ) : 855 – 860 .
- Onwulata , C. I. , Konstance , R. P. and Holsinger , V. H. 1996 . Flow Properties of Encapsulated Milkfat Powders as Affected by Flow Agents . J. Food Sci. , 61 ( 6 ) : 1211 – 1215 .
- Papadakis , S. E. and Bahu , R. E. 1992 . The Sticky Issue of Drying . Drying Technol. , 10 ( 4 ) : 817 – 837 .
- Pasley , H. and Haloulos , P. 1994 . “ Stickiness- A Comparison of Test Methods and Characterization Parameters ” . In Drying '94 Edited by: Rudolph , V. , Keey , R. B. and Mujumdar , A. S. 165 – 172 .
- Peleg , M. , Mannheim , C. H. and Passy , N. 1973 . Flow Properties of Some Food Powders . J. Food Sci. , 38 : 959 – 964 .
- Peleg , M. 1977 . Flowability of Food Powders and Methods for its Evaluation- a Review . J. Food Process Eng. , 1 : 303 – 328 .
- Peleg , M. and Mannheim , C. H. 1977 . The Mechanism of Caking of Powdered Onion . Food Process Eng. , 1 : 3 – 11 .
- Peleg , M. and Hollenbach , A. M. 1984 . Flow Conditioners and Anticaking Agents . Food Technol. , : 93 – 102 .
- Peleg , M. 1992 . On the use of the WLF Model in Polymers and Foods . Crit. Rev. Food Sci. Nutr. , 32 ( 1 ) : 59 – 66 .
- Peleg , M. 1993 . “ Glass Transition and Physical Stability of Food Powders ” . In Glassy State in Foods Edited by: Blanshard , J. M.V. and Lillford , P. J. 18 – 48 . England : Nottingham University Press .
- Peschl , I. A.S.Z. 1989 . Quality Control of Powders for Industrial Application . Powder Handling Process , 1 ( 4 ) : 357 – 364 .
- Pietsch , W. B. 1997 . “ Size Enlargement by Agglomeration ” . In Handbook of Powder Science and Technology Edited by: Fayed , M. E. and Otten , L. 202 – 252 . New York : Chapman and Hall .
- Rahman , S. 1995 . Food Properties Handbook Boca Raton, FL : CRC Press .
- Rennie , P. R. , Chen , X. D. , Hargreaves , C. and Mackereth , A. R. 1999 . A Study of the Cohesion of Dairy Powders . J. Food Eng. , 39 : 277 – 284 .
- Roos , Y. H. 1992 . “ Phase Transitions and Transformations in Food Systems ” . In Handbook of Food Engineering Edited by: Heldman , D. R. and Lund , D. B. New York : Marcel Dekker .
- Roos , Y. and Karel , M. 1991a . Phase Transition of Mixtures of Amorphous Polysaccharides and Sugars . Biotechnol. Prog. , 7 ( 1 ) : 49 – 53 .
- Roos , Y. and Karel , M. 1991b . Plasticizing Efffect of Water on Thermal Behavior and Crystallization of Amorphous Food Model . J. Food Sci. , 56 ( 1 ) : 38 – 43 .
- Roos , Y. and Karel , M. 1993 . “ Effects of Glass Transitions on Dynamic Phenomena in Sugar Containing Food Systems ” . In Glassy States in Foods Edited by: Blanshard , J. M.V. and Lillford , P. J. 207 – 222 . England : Nottingham University Press .
- Rosinski , M. J. , Barmore , C. R. , Dick , R. L. and Action , J. C. 1989 . Film Sealant and Vacuum Effects on two Measures of Adhesion at the Sealant-Meat Interface in a Cook-in Packaging System for Processed Meat . J. Food Sci. , 54 ( 4 ) : 863 – 866 .
- Rumpf , H. 1962 . “ The Strength of Granules and Agglomerates ” . In Agglomeration Edited by: Knepper , W. , Ed. 379 – 418 .
- Russel , T. P. and Kim , H. C. 1999 . Tack—a Sticky Subject . Science , 285 : 1219 – 1220 .
- Satas , D. 1989 . “ Peel ” . In Handbook of Pressure Sensitive Adhesive Technology Edited by: Satas , D. , Ed. 61 – 95 . New York : Chapman and Hall .
- Saunders , S. R. , Hamann , D. D. and Lineback , D. R. 1992 . A Systems Approach to Food Material Adhesion . Lebensam-Wiss-u-Technol. , 25 : 309 – 315 .
- Schubert , H. , Herrmann , W. and Rumpf , H. 1975 . Deformation Behavior of Agglomerates under Tensile Stress . Powder Technol. , 11 : 121 – 131 .
- Schubert , H. 1987 . Food Particle Technology. Part I: Properties of Particles and Particulate Food Systems . J. Food Eng. , 6 : 1 – 32 .
- Shanahan , M. E.R. and Carre , A. 1995 . Viscoelastic Dissipation in Wetting and Adhesion Phenomena . Langmuir , 11 : 1396 – 1402 .
- Slade , L. , Levine , H. and Finley , J. W. 1989 . “ Protein Water Interactions: Water as a Plasticizer of Gluten and Other Protein Polymers ” . In Protein Quality and the Effects of Processing Edited by: Dixon-Phillips , R. and Finley , J. W. 9 – 124 . New York : Marcel Dekker .
- Slade , L. and Levine , H. 1991 . Beyond Water Activity: Recent Advances Based on an Alternative Approach to the Assessment of Food Quality and Safety . Crit. Rev. Food Sci. Nutr. , 30 ( 2–3 ) : 115 – 360 .
- Slade , L. , Levine , H. , Ievolella , J. and Wang , M. 1993 . The Glassy State Phenomenon in Applications for Food Industry: Application of Food Polymer Science Approach to Structure-Function Relationships of Sucrose in Cookie and Cracker Systems . J. Sci. Food Agric. , 63 : 133 – 176 .
- Soesanto , T. and Williams , M. C. 1981 . Volumetric Interpretation of Viscosity for Concentrated and Dilute Sugar Solutions . J. Phys. Chem. , 85 ( 22 ) : 3338 – 3341 .
- Stevenson , M. J. , Chen , X. D. and Fletcher , A. 1998 . “ The Effect of Fat Content of the Drying of Milk Products ” . In Drying' 98; Proceedings of the 11th International Drying Symposium (IDS'98) Vol. B , 1200 – 1206 . Halkidiki, , Greece
- Szczesniak , A. S. 1963 . Classification of Textural Characteristics . J. Food Sci. , 28 ( 4 ) : 385 – 389 .
- Teunou , E. , Fitzpatrick , J. J. and Synnott , E. C. 1999 . Characterization of Food Powder Flowability . J. Food Eng. , 39 : 31 – 37 .
- Truong , V. , Bhandari , B. R. , Howes , T. and Adhikari , B. 1999 . Glass Transition Temperature Approach to Describe the Stickiness of Sugar-Rich Foods during Spray Drying . Food Res. Int. , submitted
- Tsourouflis , S. , Flink , J. M. and Karel , M. 1976 . Loss of Structure in Freeze Dried Carbohydrate Solutions. Effect of Temperature, Moisture Contents and Composition . J. Sci. Food Agric. , 27 ( 6 ) : 509 – 519 .
- Van Oss , C. J. , Good , R. J. and Busscher , H. J. 1990 . Estimation of the Polar Surface Tension Parameters of Glycerol and Formamide, for use in Contact Angle Measurements on Polar Solids . J. Dispersion Sci. Technol. , 11 ( 1 ) : 75 – 81 .
- Wallack , D. A. and King , C. J. 1988 . Sticking and Agglomeration of Hygroscopic, Amorphous Carbohydrate and Food Powders . Biotechnol. Prog. , 4 ( 1 ) : 31 – 35 .
- Walton , D. E. and Mumford , C. J. 1999 . Spray Dried Products-Characterization of Particle Morphology . Chem. Eng. Res. Des. , 77 ( A1 ) : 21 – 38 .
- White , G. W. and Cakebread , S. H. 1966 . The Glassy State in Certain Sugar-Containing Food Products . J. Food Technol. , 1 : 73 – 82 .
- Williams , M. L. , Landel , R. F. and Ferry , J. D. 1995 . Temperature Dependence of Relaxation Mechanisms in Amorphous Polymers and Other Glass-Forming Liquids . J. Am. Chem. Soc. , 77 : 3701 – 3707 .
- Zisman , W. A. 1963 . The Influence of Constitution on Adhesion . Ind. Eng. Chem. , 55 : 19 – 38 .