Abstract
The GAB (Guggenheim, Andersen, and de Boer) equation was adjusted to literature data of sorption of Amaranthus cruentus L. (M e vs. a w for adsorption and desorption) determined at 25, 30, 35, 40, 45, 50, 55, 65, 70, and 90°C, in the range of water activity from 0.029 to 0.979. To quantify the goodness of fit, the correlation coefficient (R 2), the sum of squares (RSS), the standard error of the estimate (S y ), the mean relative deviation (MRD) and the plots of residuals were analysed. The three theoretical parameters of the GAB model (M o , C, and K) gave a good correlation (R 2 > 0.9817, RSS < 0.0297, MRD < 0.138, S y < 0.0143, and random residuals‐plots) in the range of a w from 0.029 to 0.979, of interest in seed storage and processing. However this correlation does not consider the effect of temperature (T) on coefficient values. In a second stage, parameters M o and K were adjusted at each temperature. Very low variances were obtained in the range 25–65°C for desorption and in the range 25–55°C for adsorption. These results suggested that M o and K remain almost constant and a correlation with T is not justified. On the contrary sense, parameter C showed stronger variation with T. This was explained by the analysis of sensitivity for the influence of C on moisture content. On this basis, the relation C–T was proposed by an Arrhenius‐type relation [C = A.exp(B/T)] and this function was incorporated to the original GAB model to re‐estimate the parameters A, B, M o , and K. The developed modification provides a generalised and precise expression of GAB model for Amaranth.
Introduction
Amaranth genus (Amaranthaceae family) involves more than 50 species.Citation[1] Among edible ones it can be mentioned five American species: Amaranthus cruentus L., Amaranthus caudatus L., Amaranthus dibins martext thelling, Amaranthus hyponchondriacus L., and Amaranthus. mantegazzianus.Citation[1]
In the last years, this grain has been rediscovered because of its extraordinary differential properties for human consumption, like high lysine content, good balance in other aminoacids, high content of proteins (14–18%), vitamins and minerals and high proportion of squalene in the oil compared to other vegetable oils.Citation[2] The potential complementary nature of amaranth protein has been studied by combining amaranth with wheat, sorghum, and maize in compound flours. In the same sense its starch components are distinctive.Citation[3]
Amaranth grain can be used in breakfast cereals, soups, breads, cookies, pancakes, and as ingredient in confections.Citation[4] Citation[5] Also, the popped grain provides opportunities for processors to develop innovative products like candies and nougats.Citation[6] The composition of A. cruentus L. grains, based on 100 g (dry basis), is 16.8 g protein, 3.1 g ash, 7.7 g fat, 10.5 g water, and 73 g starch.Citation[6] Compared with other cereals like wheat, corn, rice, and oats, amaranth grains have higher content of proteins, fiber, calcium, and iron and provide more calories. The quality of proteins is very remarkable. The content of essential aminoacids of amaranth grains is comparable with the corresponding for soybeans (4 g isoleucine, 6 g leucine, 5.5 g lysine, 3.5 g treonine, 1 g tryptophane, 4.5 g valine, based on 100 g of protein).Citation[6]
Successful amaranth grain production requires a good knowledge of both pre‐harvest and post‐harvest characteristics to prevent quality losses. The crop should be harvested as soon as possible after a frost—usually about 10 days—to reduce grain loss from shatteringCitation[4] and must be dried below 10–12% moisture content for safe storage. Excessive thermal processing has been shown to reduce the quality of amaranth grain.Citation[3] Citation[7]
To optimise grain conditioning operations and equipment design, the characteristics of the relationship between equilibrium moisture content (EMC) and equilibrium relative humidity (ERH) and its dependence with temperature must be comprehended.
Moisture sorption isotherms describe the interaction between moisture content (M e ) and relative humidity, usually called water activity (a w ) in food science studies. Many theoretical, semi‐theoretical and empirical equations have been developed in order to model the sorptional equilibrium of grains.
GAB (Guggenheim, Anderson, and de Boer) equation, derived from the model of BET (Brunauer, Emmet, and Teller) for physical adsorption, has been widely adopted, mainly for starchy products,Citation[8] cereals and oilseeds.Citation[9] Shatadal and Jayas,Citation[10] in a review of moisture sorption isotherms, recognized GAB equation as the most satisfactory theoretical isotherm equation. They found it suitable for describing the effect of temperature on the sorption behavior of several food components in the temperature range of 25–80°C and, remarking the popularity of GAB equation in Europe, suggested that more studies should be done to derive the parameters of GAB equation for different cereal grains.
Also, Van den Berg,Citation[11] from the analysis of approximately 75 equations, concluded that GAB equation should be used due its important advantages over the others, like: (a) it has a sound theoretical background, because derives from the Langmuir and BET theories of physical adsorption; (b) it provides a good description of almost all food isotherms in the wide range 0–0.9 of water activity; (c) it is a simple expression with only three parameters that can be used easily in engineering design; (d) its parameters have physical meaning to comprehend the complexity of water sorption; and (e) it is able to describe the effect of temperature on the isotherms by equating its parameters through the Arrhenius model.
ASAE Standard D254.5,Citation[12] after its revision,Citation[13] also includes the GAB equation as accepted prediction method. Guggenheim, Anderson, and de Boer equation has been also recommended by Bakker‐ArkemaCitation[14] for use in the simulation of drying.
Notwithstanding, this isotherm does not include the temperature term; then it can only describe the relationship between a w and moisture content at fixed temperatures. In that respect, trying to improve its performance, Iglesias and ChirifeCitation[15] modelled M o (monolayer moisture content) as an Arrhenius type relationship with temperature. In the same sense, Jayas and MazzaCitation[16] modified one parameter of the GAB model to incorporate the effect of temperature when studying water sorption by oats.
Calzetta Resio et al.Citation[17] used the GAB model to estimate the isosteric heat of sorption of amaranth starch in the range from 25 to 50°C. Pollio et al.Citation[18] also studied the sorption equilibrium of amaranth grains in order to predict the isosteric heat of sorption but at only three temperatures (35, 45, and 65°C). Lema et al.Citation[19] presented experimental data of adsorption and desorption of water over amaranth in the range 25 to 55°C. Tosi et al.Citation[1] reported data of water sorption on amaranth grains (A. cruentus L. variety) in the range from 40 to 90°C.
As very little information about sorptional equilibrium of amaranth grains is available in literature and the GAB model proved to be a valuable tool for the analysis of sorption and desorption on foods, the objectives of this work were: (i) to study the adjustment of GAB equation to sorption data of amaranth obtained from literature; (ii) to analyse the effect of temperature on GAB parameters; and (iii) to develop a simple modification of GAB equation that incorporates the above mentioned effect.
Materials and Methods
Sources of Sorption Data
Experimental data of water sorption (M e vs. a w ) of the species A. cruentus L. were taken from literatureCitation[1] Citation[18] Citation[19] for desorption/adsorption at 25, 30, 35, 40, 45, 50, 55, 65, 70, and 90°C in the range of water activity from 0.029 to 0.979. presents all the data sets with their individual temperature and water activity ranges. The total number of data points available was 147. All the data were original experimental points either cited precisely in tables or read from experimental points on figures. The collected data were obviously classified in two groups: adsorption and desorption; however some of them were not possible to be identified as desorption or adsorption data, then these points were considered as average results. summarizes the complete data sets and shows that—as drying has been generally of more interest of study than rehydration—a higher amount of data points for desorption are available in literature.
Table 1. Sources of sorption data of amaranth seeds (Amaranthus cruentus L. variety) for the fitting of GAB equation
Table 2. Collection of sorption equilibrium values of amaranth seeds (Amaranthus cruentus L. variety)
The published data of water sorption on amaranth grains were obtained by static gravimetric methods with different atmospheres surrounding the product (). The experimental determination of isotherms using the static gravimetric method involved the exposition for long times of grain samples supported in small baskets into glass desiccators containing either saturated salt solutions or sulfuric‐acid solutions to maintain constant vapor pressure at constant temperatures. Lema et al.Citation[19] used saturated salts and amaranth grains with initial moisture content in the range 28–31% (d.b.) for desorption experiences. For adsorption, they used dry grains with moisture content between 2–4% (d.b.). Tosi et al.Citation[1] worked with natural dried grains which were exposed to saturated solutions of sulfuric acid that provided water activities between 0.2 and 0.8. These authors measured the a w level with an humidimeter (Hanna, HI 8564 Model). Pollio et al.Citation[18] obtained the isotherms by the gravimetric method using saturated salt solutions for dehydrating small samples of amaranth grains (harvested with 9% d.b. moisture content and hydrated to 21% d.b.) in vacuum desiccators. These researchers measured the corresponding a w levels with a hygrometer (Thermoconstanter Humidal TH2, Novasina AB, Zurich, Switzerland) while the moisture content was measured gravimetrically after vacuum drying. Equilibrium conditions were obtained when the change in sample mass among three successive measures was less than 0.001 gCitation[19] or 0.005 g.Citation[1] The moisture content of samples at this stage was determined by drying in oven either at 95°C during 48 hoursCitation[19] or at 130°C during 1 hourCitation[1] or at 70°C and 6.7 kPa over magnesium perchlorate.Citation[18] The time to reach equilibrium varied from 10 to 12 d depending on relative humidity and temperature.Citation[19] The differences among the reported data would be attributed to differences in grain maturity and history, and to the different techniques used for measuring EMC–ERH.Citation[9] Citation[21]
Mathematical Modelling and Fitting Method
The whole set of published data were modelled by the Guggenheim–Anderson–de Boer isotherm (GAB) that has the following form:
The standard error of the estimate (S y ) is the conditional standard deviation of the dependent variable and has the form:
The MRD in an absolute value that was used because gives a clear idea of the mean divergence of the estimated data respect to the measured data:
In general, low values of R 2, high values of RSS, S y , and MRD, and clear patterns in the residual plots mean that the model is not able to explain the variation in the experimental data.
shows the obtained values of the parameters M o , C, and K of EquationEq. (1) for the complete data set with the corresponding standards errors (ASE) and percent standard error (ASE%) of the parameters. This fitting can only be used to describe the average sorption behavior because it was originated from data of different sources, determined over a wide range of temperatures and with diverse experimental techniques (). Fitting individual data sets, more sensitive tests of the adequacy and characteristics of the GAB equation can be performed than fitting the whole data set, due to the great dissemination of the experimental points. shows the results of the fitting of the grouped data for desorption, adsorption and “average.” These results can describe the individual processes of desorption and adsorption, while the average can be used in general applications.Citation[23] From these results, it can also be noted that the hysteresis effect is significant over all the water activity range. shows the comparison between the curves for desorption, adsorption, and average predicted by the GAB equation at 50°C. Very similar graphs with comparable magnitude of hysteresis effect were obtained at 25, 30, 35, 40, 45, and 55°C, demonstrating that temperature has no strong influence on hysteresis. This behavior has also been observed by Sun and WoodsCitation[24] in a review of sorption data for wheat. On the other hand, these results confirm that the theoretical desorption curves are positioned above the adsorption curves for all the a w range. The regression for “average” data, as shown in for data at 50°C, lie between adsorption and desorption curves when a w is between 0.1 and 0.5.
Figure 2. The influence of different kinds of data on the isotherms at 50°C of Amaranthus cruentus L. grains predicted by GAB equation.
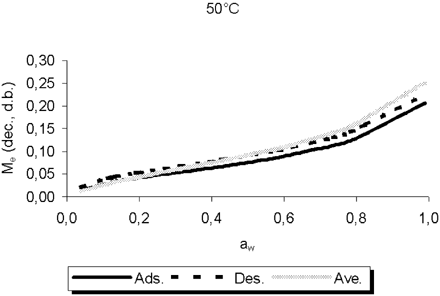
Table 3. Parameters for the fitting of GAB equation for water sorption of amaranth grains for the whole data set in the range of temperature from 25 to 90°C and water activity from 0.029 to 0.979
Table 4. Fitted parameters for GAB equation for desorption, adsorption, and average in the range of temperature from 25 to 90°C and water activity from 0.029 to 0.979
Besides, GAB equation was fitted for desorption and adsorption at each individual temperature. The original data in which desorption or adsorption were not identified (termed here as “average”) were assumed to be desorptive for low water activities and adsorptive at high water activities.Citation[23] Then, these points were included into the subgroups adsorption or desorption at their corresponding temperature. The results of these adjustments are presented in . As can be seen, R 2 values are very high and RSS, S y , and ASE% (except for C) are low for all the temperatures and both for desorption and adsorption. Values at 70 and 90°C were not adjusted because each set consisted of only four data. These results clearly demonstrate the adequacy of the GAB model to describe M e vs. a w relationship for amaranth seeds.
Table 5. Fitted parameters of GAB equation for desorption and adsorption at different temperatures from 25 to 90°C and water activity from 0.029 to 0.979
Comparison with Published Data for Other Starchy Foods
Calzetta Resio et al.,Citation[17] working in the range of 25–50°C with amaranth starch isolated from amaranth seeds, reported values of M o and C varying from 0.102 to 0.09 and from 16.8 to 9.7, respectively, while K varied between 0.81 and 0.80, showing no clear variation trend with temperature. These values are not very different from those obtained here, in spite of the differences in composition and structure of the tested materials. This could mean that the starchy components of the seed determine, to a great extent, its sorption characteristics.
Continuing the analysis of the adequacy of GAB model through the study of the residual plots, and show clear patterns, in agreement with the behavior observed by Chen and JayasCitation[9] for high‐protein and high‐starch materials. These authors explained this phenomenon by the sigmoid shape of the sorption curve that cannot be adequately tracked by most adsorption models.
Figure 3. Residual plot from the adjustment of GAB equation [EquationEq. (1)] for desorption data of Amaranthus cruentus L. at eight temperatures.
![Figure 3. Residual plot from the adjustment of GAB equation [EquationEq. (1)] for desorption data of Amaranthus cruentus L. at eight temperatures.](/cms/asset/42b9121f-c0d5-4bd2-bce4-f8b9d795a578/ljfp_a_10345201_o_f0003.gif)
Figure 4. Residual plot from the adjustment of GAB equation [EquationEq. (1)] for adsorption data of Amaranthus cruentus L. at seven temperatures.
![Figure 4. Residual plot from the adjustment of GAB equation [EquationEq. (1)] for adsorption data of Amaranthus cruentus L. at seven temperatures.](/cms/asset/55c708f7-9126-417e-bc8c-74dcd94859e4/ljfp_a_10345201_o_f0004.gif)
Lomauro et al.Citation[8] confirmed that nearly 80% of the isotherms of starchy foods can be described by the GAB equation. These authors have reported values of the GAB parameters for starchy foods () comparable to those observed in this work for amaranth.
Table 6. Constants for GAB isotherms of starchy foods reported by Lomauro et al.Citation[8]
In a similar fashion, Tolaba et al.,Citation[25] in a study of water sorption on quinoa grains (with characteristics of composition analogous to those of amaranth), found comparable values for K, which varies slightly between 0.6 and 0.8, while M o showed a moderate dependence with temperature. These results confirm the similitude in sorption behaviour among products with similar compositions.
Temperature Dependence of the Guggenheim, Anderson, and de Boer Equation
shows that M o varied between 0.070 (at 25°C) and 0.044 (at 65°C) for desorption (with standard deviation of 0.013), while for adsorption it varied between 0.052 (at 25°C) and 0.049 (at 55°C) (with standard deviation of 0.014). Parameter K presented a slight variation between 0.676 (at 25°C) and 0.858 (at 65°C) for desorption (with standard deviation of 0.105) and between 0.778 (at 25°C) and 0.787 (at 55°C) for adsorption (with a standard deviation of 0.125).
Analysing the parameters M o and K, it can be noted that both show a slight variation along the temperature range. Both for adsorption and desorption, the percent standard errors of estimation (ASE, %) of the parameters M o and K are notably low compared with those corresponding to C. In virtue of this, both M o and K can be considered approximately constant in the range of temperature from 25 to 65°C. Then, it is not justified to explore a correlation with temperature. In this sense, Van den Berg,Citation[11] Maroulis et al.Citation[26] and Kiranoudis et al.Citation[27] have also proposed to consider M o constant with temperature and these authors analysed the effect of temperature only on the other parameters. Besides, Van den BergCitation[11] studying the water sorption isotherms of various foods and related products—including starchy foods—informed that the influence of temperature on the isotherm is described by C and—to a lesser extent—by K.
Respect to the values of C of this work, it can be observed in that this parameter presents an evident dependence on temperature with a variable behavior along the temperature range. In order to study the relation C vs. T, the sensitivity of M e respect to C was analysed through the partial derivation of EquationEq. (1) respect to C, following the procedure proposed by Gely and Giner:Citation[28]
Figure 5. Test of sensitivity of M e against C through the partial derivative, for water desorption of Amaranthus cruentus L. seeds.
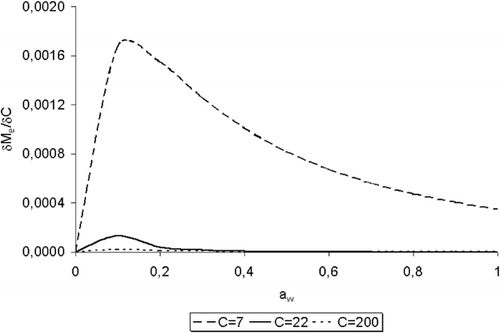
Figure 6. Test of sensitivity of M e against C through the partial derivative, for water adsorption of Amaranthus cruentus L. seeds.
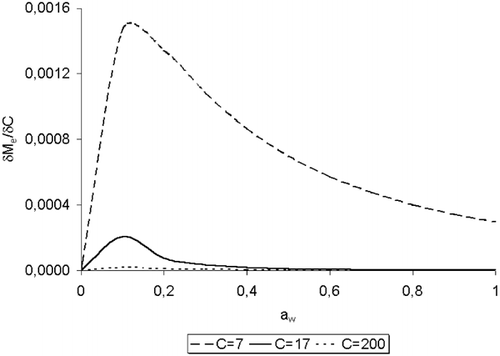
In this sense, an Arrhenius‐type expressionCitation[9] Citation[11] Citation[26] Citation[27] Citation[29] was proposed to describe the C–T relationship:
Table 7. Parameters of the generalized GAB isotherm for sorption of water of Amaranthus cruentus L. seeds
The residual plots ( and ) were uniformly scattered, remarking the goodness of fit of this proposed modification.
Figure 7. Residual plot from the generalised GAB equation [EquationEq. (7)] for water desorption on Amaranthus cruentus L. in the range from 25 to 90°C.
![Figure 7. Residual plot from the generalised GAB equation [EquationEq. (7)] for water desorption on Amaranthus cruentus L. in the range from 25 to 90°C.](/cms/asset/96eabe75-9d1d-4752-a02b-a65cfe3db039/ljfp_a_10345201_o_f0007.gif)
Figure 8. Comparison between experimental and calculated [by EquationEq. (7)] moisture content for desorption of water of Amaranthus cruentus L. at eight temperatures.
![Figure 8. Comparison between experimental and calculated [by EquationEq. (7)] moisture content for desorption of water of Amaranthus cruentus L. at eight temperatures.](/cms/asset/1e1217ad-4f22-4867-a4ae-7031765d8134/ljfp_a_10345201_o_f0008.gif)
The following graphs ( and ) were drawn to compare the experimental and calculated [through EquationEq. (7)] values for desorption and adsorption of water from Amaranth grains. In both cases, it can be observed that the points are regularly distributed around a line at 45°.
Figure 9. Residual plot from the generalised GAB equation [EquationEq. (7)] for water adsorption on Amaranthus cruentus L. in the range from 25 to 90°C.
![Figure 9. Residual plot from the generalised GAB equation [EquationEq. (7)] for water adsorption on Amaranthus cruentus L. in the range from 25 to 90°C.](/cms/asset/03a4209f-601c-4e5b-8aa5-6bd6a323b43b/ljfp_a_10345201_o_f0009.gif)
Figure 10. Comparison between experimental and calculated [by EquationEq. (7)] moisture content for adsorption of water of Amaranthus cruentus L. at seven temperatures.
![Figure 10. Comparison between experimental and calculated [by EquationEq. (7)] moisture content for adsorption of water of Amaranthus cruentus L. at seven temperatures.](/cms/asset/03b1f54c-a481-4833-9d9f-04d864075d3d/ljfp_a_10345201_o_f0010.gif)
In spite of the overall accuracy of EquationEq. (7) over the whole temperature range, the fitting of any data set for a unique temperature to the three‐parameter version always delivers more accurate results. This is shown in that presents the data sets for 40°C and the values predicted by the three‐parameter [EquationEq. (1)] and four‐parameter [EquationEq. (7)] versions of GAB equation.
Figure 11. Data sets of moisture sorption isotherms of Amaranthus cruentus L. grains at 40°C and predicted curves by the three‐parameter [EquationEq. (1)] and four‐parameter [EquationEq. (7)] versions of GAB equation. Key: Des., desorption; GAB‐3PAR Des., GAB‐4PAR Des.: GAB model for desorption with three and four parameters, respectively Ads., GAB‐3PAR Ads., GAB‐4PAR Ads.: GAB model for adsorption with three and four parameters, respectively.
![Figure 11. Data sets of moisture sorption isotherms of Amaranthus cruentus L. grains at 40°C and predicted curves by the three‐parameter [EquationEq. (1)] and four‐parameter [EquationEq. (7)] versions of GAB equation. Key: Des., desorption; GAB‐3PAR Des., GAB‐4PAR Des.: GAB model for desorption with three and four parameters, respectively Ads., GAB‐3PAR Ads., GAB‐4PAR Ads.: GAB model for adsorption with three and four parameters, respectively.](/cms/asset/ee449ae3-2b05-4594-9eea-372713332297/ljfp_a_10345201_o_f0011.gif)
Conclusions
From the analysis of the present work, the following conclusions can be drawn:
Guggenheim, Anderson, and de Boer isotherm describes closely the sorption data of water on Amaranthus cruentus L. seeds in the temperature range from 25°C to 90°C.
Monolayer moisture content (M o ) shows a slight decrease with the increase of temperature, and can be considered constant in the range from 25°C to 90°C.
Parameter K also presents a very slow variation with temperature; consequently, it can be set constant in the range of analysis.
Both for desorption and adsorption, the parameter C shows a strong dependence on temperature.
The generalised GAB expression with four parameters—that considers the influence of temperature—describes adequately the sorption data in the range from 25 to 90°C.
Notation
A, B | = |
parameters of EquationEq. (6) |
ASE | = |
standard error of estimation of parameter |
a w | = |
water activity |
C | = |
parameter of GAB equation |
df | = |
degrees of freedom |
EMC | = |
equilibrium moisture content |
ERH | = |
equilibrium relative humidity |
K | = |
parameter of GAB equation |
m | = |
number of data points |
M e | = |
dimensionless equilibrium moisture content |
[Mcirc] e | = |
estimated value |
M o | = |
mono‐layer moisture content |
MRD | = |
mean relative deviation |
P* | = |
mean relative deviation modulus |
R 2 | = |
correlation coefficient |
RSS | = |
sum of squares |
S y | = |
standard deviation of estimate |
T | = |
temperature |
References
- Tosi , E. , Masciarelli , R. and Ciappini , M. 1994 . Humedades de equilibrio del amaranto (Amaranthus cruentus) . La Alimentación Latinoamericana , 203 : 73 – 76 .
- Sun , H. , Wiesenborn , D. , Rayas‐Duarte , P. , Mohamed , A. and Hagen , K. 1995 . Bench‐scale processing of amaranth seed for oil . JAOCS , 72 ( 12 ) : 1551 – 1555 .
- Kauffmanm , C. S. and Weber , L. E. 1990 . “ Grain amaranth ” . In Advances in New Crops Edited by: Janick , J. and Simon , J. E. 127 – 139 . Portland , OR : Timber Press .
- Baltensperger , D. D. , Lyon , D. J. , Nelso , L. A. and Corr , A. “ Amaranth grain production in Nebraska ” . Nebraska Cooperative Extension 1995, NF91‐35
- Ayo , J. A. 2001 . The effect of amaranth grain flour on the quality of bread . Int. J. Food Prop. , 4 ( 2 ) : 341 – 351 .
- Genta , H. D. , Jandula , A. M. and Alvarez , H. 1997 . Determinación de la calidad nutricional de golosinas de soja y amaranto . Información Tecnológica , 8 ( 4 )
- Bressani , R. and Elias , L. G. 1986 . “ Development of 100% amaranth foods ” . In Third Amaranth Conference Emmaus , PA : Rodale Press, Inc. .
- Lomauro , C. J. , Bakshi , A. S. and Labuza , T. P. 1985 . Evaluation of food moisture sorption isotherm equations. Part II: milk, coffee, tea, nuts, oilseeds, spices and starchy foods . Lebensm.‐Wiss. U.‐Technol. , 18 : 118 – 124 .
- Chen , C. and Jayas , D. S. 1998 . Evaluation of the GAB equation for the isotherms of agricultural products . T. ASAE , 41 ( 6 ) : 1755 – 1760 .
- Shatadal , P. and Jayas , D. S. 1990 . Moisture sorption isotherms of grains and oilseeds . Postharvest News and Information , 1 ( 6 ) : 447 – 451 .
- Van Den Berg , C. “ Description of water activity of foods for engineering purposes by means of the GAB model of sorption ” . In Engineering and Food Engineering Sciences in the Food Industry Edited by: McKenna , B. Vol. 1 , 311 – 321 . 1984 : Elsevier Applied Science Pub. .
- 1999 . ASAE Standards, Standards Engineering Practices Data St. Joseph , MI : ASAE . ASAE
- Sokhansanj , S. and Yang , W. 1996 . Revision of the ASAE standard D245.4. Moisture relationships of grains . T. ASAE , 39 ( 2 ) : 639 – 642 .
- Bakker‐Arkema , F. W. 1985 . “ Heat and mass transfer aspects and modeling of dryers—a critical evaluation ” . In Concentration and Drying of Foods Edited by: McCarthy , Q. 165 – 202 . NY : Elsevier Appl. Sci. .
- Iglesias , H. A. and Chirife , J. 1984 . Correlation of BET monolayer moisture content in foods with temperature . J. Food Tech. , 19 : 503 – 506 .
- Jayas , D. S. and Mazza , G. 1993 . Comparison of five, three‐parameter equations for description of adsorption data of oats . T. ASAE , 36 ( 1 ) : 119 – 125 .
- Calzetta Resio , A. , Aguerre , R. J. and Suarez , C. 1999 . Analysis of the sorptional characteristics of amaranth starch . J. Food Engng. , 42 : 51 – 57 .
- Pollio , M. L. , Tolaba , M. P. and Suárez , C. 1998 . Measuring and modeling grain sorption equilibria of amaranth grains . Cereal Chem. , 75 ( 3 ) : 297 – 300 .
- Lema , A. , Palumbo , D. , Adaro , J. and Lara , M. 2001 . “ Equilibrio de Sorción de Amaranto ” . In Memorias del VIII Congreso Latinoamericano de Transferencia de Calor y Materia LATCYM'2001 184 – 189 . Veracruz , , México ISBN 698‐5401‐00‐4
- Wilkinson , L. 1990 . SYSTAT: The System for Statistics Evanston , IL : SYSTAT, Inc. .
- Brooker , D. V. , Bakker‐Arkema , F. W. and Hall , C. W. 1981 . Drying Cereals Grains Westport , Connecticut : The Avi Pub. Co. Inc. .
- Sopade , P. A. 2001 . Criteria for an appropriate sorption model based on statistical analysis . Int. J. Food Prop. , 4 ( 3 ) : 405 – 418 .
- Sun , D. W. and Woods , J. L. 1994 . The selection of sorption isotherm equations for wheat based on the fitting of available data . J. Stored Prod. Res. , 30 ( 1 ) : 27 – 43 .
- Sun , D. W. and Woods , J. L. 1993 . The moisture content/relative humidity equilibrium relationship of wheat—a review . Dry. Technol. , 11 ( 7 ) : 1523 – 1551 .
- Tolaba , M. P. , Peltzer , M. , Enriquez , N. and Pollio , M. L. 2001 . “ Grain sorption equilibria of Quinoa grains ” . In CD‐ROM Proc. III Congreso Iberoamericano de Ingeniería de Alimentos “CIBIA'2001” y I Congreso Español de Ingeniería de Alimentos 1A – 06 . Valencia , , España
- Maroulis , Z. B. , Tsami , E. and Marinos‐Kouris , D. 1988 . Application of the GAB model to the moisture sorption isotherms for dried fruits . J. Food Engng. , 7 : 63 – 78 .
- Kiranoudis , C. T. , Maroulis , Z. B. , Marinos‐Kouris , D. and Tsamparlis , M. 1997 . Design of tray driers for food dehydration . J. Food Engng. , 32 : 269 – 291 .
- Gely , M. C. and Giner , S. A. 2000 . Water‐corn equilibrium: Temperature dependency of the G.A.B. model parameters and calculation of the heat of sorption . Dry. Technol. , 18 ( 7 ) : 1449 – 1464 .
- Rapusas , R. S. , Driscoll , R. H. and Buckle , K. A. 1993 . Moisture desorption characteristics of raw onion slices . Food Aust. , 45 ( 6 ) : 278 – 284 .
- Gely , M. C. and Giner , S. A. 1999 . “ Comportamiento del Modelo G.A.B. para Sorción de Agua en Trigo ” . In CD‐ROM Proceedings del VIII Congreso Argentino de Ciencia y Tecnología de Alimentos y I Simposio Internacional de Tecnología Alimentaria A2 – 13 . Rafaela , , Argentina
- Silakul , T. and Jindal , V. K. 2002 . Equilibrium moisture content isotherms of mungbean . Int. J. Food Prop. , 5 ( 1 ) : 25 – 35 .
- Kuye , A. and Sanni , L. O. 2002 . Modelling sorption isotherms of lafun and soyflour using a spreadsheet . Int. J. of Food Prop. , 5 ( 2 ) : 599 – 610 .