Abstract
This article reports a collection of data points on the cohesiveness of a whole milk powder. The results reveal how the cohesion (the result of inter-particle forces) of a whole milk powder is affected by relative humidity (0–70%) and temperature (20–60°C). The data were collected using a laboratory-scale vibrated fluidized bed, which minimized the duration of contact of the powder defined with humid air when being conditioned. The relationship between cohesion defined as the unconfined yield stress of a sample powder plug formed under specific conditions and powder stickiness was identified and discussed.
Introduction
Powder stickiness has been researched in recent years because of its importance in the food industry. The deposition of powder on to processing devices has caused concerns in terms of product degradation due to contamination, microbial activities, and fire hazards.Citation1 Citation2 Citation3 Citation4 Citation5 The relationship between the powder deposition (onto a stainless steel plate) rate and the powder cohesiveness (or stickiness) is evident.Citation4 In addition, the inter-particle sticking has increasingly been found to be a detrimental factor for storage and handling.Citation6 Citation7 Citation8 Inter-particle sticking causes particles to lump which sometimes leads to caking. This topic has also been “sensationalized” in some ways by the increasing recognition of perhaps the most important link between food science and engineering, i.e., material science. One of the significant developments is the glass-transition of sugar phases in foods. This transition relates directly to the sticking properties.Citation9 Citation10 Citation11 Citation12
For sugar-containing products especially those with very high sugar contents, there is a clear indication of the dependence of the stickiness of the powder on the glass-transition of amorphous sugars.Citation8 Citation13 The moisture-interactions with proteins or the basic moisture-nonreactive-surface interactions can also cause powder to stickCitation14 Citation15 but these aspects have not been addressed as rigorously as that for moisture-sugar interactions. There have been many studies in the classical powder technology discipline for nonfood materials, e.g., the capillary condensation phenomenon.
Whole milk powder has a typical composition of 36.6wt% lactose, 27.9wt% fat, 2.0wt% moisture, and 5.8wt% ash (on wet basis). The lactose (the milk sugar) is the major component, but fat and protein also are significant. Milkfat has a melting point ranging from some −20°C up to about 50°C depending on the molecular chain length.Citation16 Proteins, upon hydration, also become sticky. The extent to which these components affect the overall picture of sticking for whole milk powder is not known in a quantitative manner.
A number of methods for assessing powder stickiness have been reported previously, e.g., a mechanical stirring method for measuring sticking points,Citation17 a simple method of volume change,Citation13 a hardness test,Citation13 Citation14 Citation15 a traditional shear cell method as adopted from classical powder technologyCitation18 Citation19 Citation20 and the cohesion tests performed by the current authors in a previous study.Citation5 Wallack and KingCitation17 attributed the sticking phenomenon to a viscous flow mechanism. The traditional shear cell method can generate results useful for mass flow silo design. For milk powders, however, our experience (unpublished) is that the measurement is not stable as the sticky powder tends to create a wall slip, which fluctuates over time.Citation4 The method of volume change is more suitable for 100% amorphous materials. The hardness test may be suitable for partially amorphous products as well but has not been reported other than for an amorphous lactose product.Citation13 Cohesion is a measure of inter-particle forces thus reflecting the stickiness of the particle surfaces. The flowability defined by JenikeCitation18 of a powder is known to be a measure of how powder may be sticky or not. The cohesion value used in this study is the Unconfined Yield Stress (UYS), on which the flowability is inversely dependent. In other words, the larger the UYS, the poorer the flowability thus the greater the stickiness. This article reports the results of cohesion testing for a large temperature (20–60°C) and relative humidity range (0–35%RH for 50 and 60°C, and 0–70%RH at 20°C respectively) and the relationship between cohesion and powder stickiness has been discussed.
Materials and Methods
The laboratory procedures for sample preparation and testing have been reported in a previous article,Citation5 which describes extensive experimental work on the effect of moisture on cohesion at 20 ± 0.5°C. Here, sufficient details are provided so that the readers of the journal can easily follow.
In the current work, the unconfined yield stress (UYS) tests, as mentioned in the introduction, were conducted on a whole milk powder over a range of temperatures and relative humidities. The test procedure and the test equipment have been detailed previously.Citation5 Essentially this involves making powder plugs and then shearing the plugs.
Preparation of the consolidated plugs for the UYS test involved placing approximately 6 g of the specially prepared powder into the consolidation die, shown in Fig. , and vibrating the die to pack down the powder. Once the powder had been compacted for approximately 15–30 s, the consolidation plunger of 250 g mass (this weight gives a consolidation stress of 7.8 kPa) was placed on the powder in the die. The powder was left for 5 min to consolidate and was then tested. Typically the consolidation pressures used were very low (7.8 kPa) to represent the low levels of consolidation that would occur in the wall deposition process during the spray drying process.Citation4
When testing, the plunger was removed and the consolidation die was dismantled, yielding a cylindrical plug of powder, as shown in the centre of Fig. . Figure also indicates that it was not always possible to obtain perfect shearing; therefore the UYS results were sometimes scattered. The cylinder was axially loaded by using a loading frame that gradually increased the load on the plug. The load at which the powder failed was defined as the UYS (in Pascals). The loading of the powder was measured using a balance (Sartorius, E 5500 S) which was connected to a computer (Commodore, XT compatible) for data acquisition.
Humidification of the powder was achieved by placing about 20 g of whole milk powder in a vigorously vibrated fluidised bed. As cohesion was considered to be a surface property, it was necessary to change only the moisture content at the surface of the particles. Fluidization, i.e., a dynamic method, was used to achieve this goal of altering only the surface moisture content of the particles in a reasonably short time (in contrast to the equilibrium method).Citation21
The vibrated fluidized bed (shown in Fig. ) was constructed of glass (50 mm diameter tube, 250 mm in length), and the distributor plate was made of sintered glass with a porosity grade of 1 (the porosity of sintered glass is graded on a scale from 0 to 4, with 0 being the finest). This bed was housed in a metal cage with springs on each corner. The bed was vibrated using an unbalanced rotating wheel, which vigorously shook the cage and the attached fluidized bed. This vibration prevented channelling of the gas stream through the powder bed and allowed continuous and uniform fluidization. The particles were clearly fluidized even without the gas flowing through the bed. In general, to produce an increase in powder moisture content, a humidified air stream was used; alternatively, a dried air stream was used to dry the powder. Sometimes a mixture of these two air streams was used to obtain intermediate values of surface moisture content. This procedure has been described by Keey.Citation22 To control the degree of humidification of the powder, different fluidization times were used (from 5 to 30 min). After humidification, the sample was placed immediately either into a consolidation die (Fig. ) or into a sealed container (with little free headspace after being filled with powder) for measuring the relative humidity between powder particles. Due to the slow diffusion of the moisture into the particle, the surface relative humidity only reduces very slowly after some 5 min of settling time after fluidization. Thus for the time when the plugs are being tested the relative humidity is representative of the particle surface value.Citation21 In other words, the relative humidity in the air is representative of the value next to the particle approximately 5 min after humification. The slow decrease would take minutues to make a real difference. The corresponding surface (liquid) water content could then be worked out using the previously established equilibrium sorption isotherm.Citation21
The relative humidity was measured using a Tri-Sense hygrometer (model no. 37000-00, Cole-Parmer Instrument Company, Chicago, Illinois, USA). The procedure of placing the powder, after humidification or drying, into a sealed container was a sub-sampling process that ran in conjunction with the cohesion testing. The hygrometer probe was then placed into the container and the relative humidity of the powder was measured over 15 min. The most important relative humidity value was that taken after about 5 min as this corresponded to the relative humidity between particles when the powder cohesion was tested.
In the current study, in order to create powder samples with a large range of temperatures and relative humidities, several treatment techniques were used. For example, a heated vacuum oven was used to obtain very low humidities for the full temperature range (from 30 to 75°C). An incubation box was used to obtain low humidities (22 and 34% RH) for the full temperature range. In this case, the RHs measured at the room temperature were converted to the high temperature values.
The vibrated-fluidized bed was also used to obtain high moisture, low temperature results. As mentioned earlier, the approach was to modify only the surface moisture content of the powder. By using a vibrated-fluidized bed with fluidizing air of various humidities and temperatures, we hoped to test a large range of powder moisture contents and temperatures. The problem with this technique was the treatment of whole milk powders at high temperature and moisture content. The vibrated-fluidized bed was very useful in modifying the moisture content/humidity at the powder surface. However, as heating the powder using this technique was not effective, the powder was preheated in an oven to 60°C, the desired temperature for the experiments. The powder was placed in airtight bags to avoid any extra contact with the ambient humidity. The fluidized bed was also preheated with 15 L min−1 of dry air (RH < 5%) which was passed through a heating tube. This procedure resulted in the air entering the bed being at more than 100°C; this process normally took at least 90 min.
After both the fluidised bed and the powder had been preheated to the necessary level, the powder was added to the bed. Wet air (RH > 80%; 20 L min−1) was used to fluidize the powder for 15 min; typically the water column was heated to 60°C (however, it would range between room temperature and 75°C depending upon the required temperature of the experiment). The fluidized bed was vibrated to reduce channelling of the powder and to improve the particle-air contact. After the 15 min of fluidization, the powder was added to the consolidation die and consolidated with a 7.8 kPa consolidation pressure for either 1 or 10 min. When the powder was consolidated for 1 min, the UYS of the plug was measured immediately. When the plug was consolidated for 10 min, the consolidation plunger was removed and the plug was allowed to “dry” for at least 60 min, before the UYS was measured.
Consolidation for 10 min was performed to investigate the nature of the bonding. It was found that at high moisture contents the UYS decreased markedly. It was assumed that this was due to excess moisture softening the particles, allowing them to yield at a far lower stress. To test this, the plug was allowed to dry; it was assumed that the softened particles would then contact with a larger surface area and would produce a relatively higher UYS.
When a powder sample was being consolidated, the rest of the powder was placed in a sealed, insulated container. A temperature/humidity probe was placed into this container to measure the humidity and temperature of the air surrounding the powder.
Results and Discussion
Most of the powder samples were treated in the fluidized bed for 15 min and were consolidated for approximately 1 min. Both halves of the sheared plugs tended to be in uniform wedges, as shown in Fig. . All the data points obtained in this study are plotted in Fig. . To put the data points in perspective, rough boundaries corresponding to different relative humidity ranges (i.e., Zones III, IV, and V) are drawn. Zones I and II illustrate the results from the previous tests on samples with different relative humidities but at the same temperature (i.e., 20°C).Citation5
Between Zones I and II (at RH ≈ 50%), the cohesion (i.e., the UYS) increased dramatically with increasing relative humidity, indicating the onset of significant stickiness. Below this critical relative humidity value, the UYS did not change much at all. Figure shows the following results for Zones III, IV, and V.
i. | In each narrow relative humidity range, the UYS increased with temperature. | ||||
ii. | In the region where these zones apparently converged (at around 40°C), there were data points where very high relative humidity (about 50–90% RH) did not yield large UYS values. No large UYS values were found for this temperature region because no data points at the stickiness transition were collected. |
A more meaningful diagram is that given in Fig. , in which the mean values of UYS shown in Fig. and those reported previously by Rennie et al.Citation5 are plotted as a function of relative humidity. The boarder lines indicate the error ranges. This figure shows that similar behavior to that shown at 20°C was found for higher temperatures. However, the onset of a significant increase in UYS was shifted towards lower relative humidity. It is expected that increasing temperature will result in greater stickiness because of liquefaction of the constituent fats, which results in softening of the powder surface or makes the surface viscous. This softening of the powder will also result in an increase in inter-particle contact area, giving higher cohesion.
Figure 5. The temperature and relative humidity dependence of the cohesion of whole milk powder; the lines are indication of the expected error ranges.
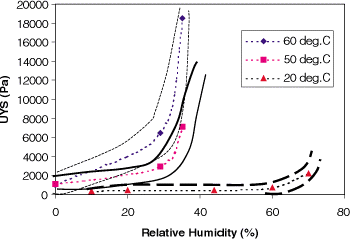
Powder that was treated using high humidity and temperature air for fluidization and that was then removed from the fluidized bed after 15 min of fluidization seemed to be more granular/powdery in texture before being packed into the consolidation die. There were large lumps of “macro” agglomerated powder, with some lumps measuring up to 10 mm in length. This indicated that the powder had already become quite sticky. The powder consolidated for 1 min yielded at a pressure generally far below that of the powder conditioned at low temperature and moisture content. In addition, the shear plane was not as defined as for the other powders and the plug usually disintegrated, as shown in Fig. . It is interesting to note that the resulting powder was quite “clumpy” relative to the powder shown in Fig. . These clumps were numerous and quite large and there did not appear to be much individual powder in the disintegrated mass. One explanation for the low UYS value is that the humidification with high relative humidity air produced large aggregates and a powder plug with larger particles was sheared which (instead of increasing the UYS) reduced the UYS. In other words, the powder surface was indeed sticky but because the lumps had already formed before UYS testing, the UYS did not reflect the true stickiness. Further, the low UYS value did not mean that the powder was not sticky. It was then thought that if the powder plug was dried the viscous contacts would form solid bridges to give a larger UYS. However, the samples that were consolidated for 10 min and then “dried” for 70 min behaved similarly, although there were some subtle differences. The disintegrated powder was again lumpy, but was also more granular—there was more individual powder in this mass. The UYS for these powders appeared to be at least the same magnitude if not less than that of the powder conditioned at low temperature and humidity. Visual observation confirmed that the powder surfaces must have been sticky before the powder was placed in the die. However, the plug lost its structural integrity because the particles were able to flow against each other when humidified at very high relative humidities or because larger aggregates were formed which changed the samples altogether even when the plugs were dried.
Conclusions
It is difficult to study the stickiness of milk powder particles because the processes of humidification and heating must be rapid to prevent secondary effects. In this study, many data points on cohesion as function of temperature and relative humidity were collected using a tedious sample preparation procedure and careful experimentation. Although the data were not comprehensive, based on the existing results, a quantitative temperature and relative humidity dependence of the cohesion (as the UYS) was identified. The results will be useful for identifying conditions under which sticking will occur in a milk powder plant or in the storage and transportation of milk powders.
Acknowledgments
The authors from the University of Auckland thank the New Zealand Dairy Board and the New Zealand Dairy Research Institute for providing the financial assistance for this work to be carried out at the university. The authors also thank the technicians at the Department of Chemical and Materials Engineering, who helped enormously during the course of this project.
References
- Papadakis , S.E. and Bahu , R.E. 1992 . The sticky issues of drying . Drying Technology , 10 : 817 – 837 .
- Chen , X.D. , Lake , R. and Jebson , S. 1993 . Study of milk powder deposition on a large industrial drier . Transactions of the Institution of Chemical Engineers, Part C , 71 : 180 – 185 .
- Chong , L.V. , Shaw , I.R. and Chen , X.D. 1996 . Exothermic reactivities of skim and whole milk powders as measured using a novel procedure . Journal of Food Engineering , 30 : 185 – 196 . [CROSSREF]
- Rennie , P.R. Cohesion and Adhesion Properties of Milk Powders as Influenced by Temperature and Water Content . PhD thesis . Auckland, , New Zealand : Department of Chemical and Materials Engineering, The University of Auckland .
- Rennie , P.R. , Chen , X.D. , Hargreaves , C. and Mackereth , A.R. 1999 . A study of the cohesion of dairy powders . Journal of Food Engineering , 39 : 277 – 284 . [CROSSREF]
- Aguilera , J.M. , Levi , G. and Karel , M. 1993 . Effect of water content on the glass transition and caking of fish protein hydrolyzates . Biotechnology Progress , 9 : 651 – 654 . [CSA]
- Aguilera , J.M. , de Valle , J.M. and Karel , M. 1995 . Caking phenomenon in amorphous food powders . Trends in Food Science and Technology , 6 : 149 – 155 . [CSA] [CROSSREF]
- Bhandari , B.R. and Howes , T. 1999 . Implication of glass transition for the drying and stability of dried foods . Journal of Food Engineering , 40 : 71 – 79 . [CROSSREF]
- Roos , Y. and Karel , M. 1991b . Plasticizing effect of water on thermal behaviour and crystallisation of amorphous food models . Journal of Food Science , 56 : 38 – 43 .
- Roos , Y. and Karel , M. 1991c . Water and molecular weight effects on glass transitions in amorphous carbohydrates and carbohydrate solutions . Journal of Food Science , 56 : 1676 – 1681 .
- Roos , Y. and Karel , M. 1992 . A research note: crystallisation of amorphous lactose . Journal of Food Science , 57 : 775 – 777 .
- Jouppila , K. and Roos , Y.H. 1994b . Glass transitions and crystallisation in milk powders . Journal of Dairy Science , 77 : 2907 – 2915 .
- Lloyd , R.J. , Chen , X.D. and Hargreaves , J.B. 1996 . Glass transition and caking of spray dried lactose . International Journal of Food Science and Technology , 31 : 305 – 311 . [CSA] [CROSSREF]
- Buma , T.J. 1971a . Free fat in spray-dried whole milk 5. Cohesion; Determination, influence of particle size, moisture content and free-fat content . Netherlands Milk and Dairy Journal , 25 : 107 – 122 .
- Buma , T.J. 1971b . Free fat in spray-dried whole milk. 3. Particle size. Its estimation, influence of processing parameters and its relation to free-fat content . Netherlands Milk and Dairy Journal , 25 : 53 – 72 .
- Chen , X.M. Layer Crystallisation of Milkfat in Shear Field . ME thesis . Department of Chemical and Materials Engineering, The University of Auckland .
- Wallack , D.A. and King , C.J. 1988 . Sticking and agglomeration of hygroscopic, amorphous carbohydrate and food powders . Biotechnology Progress , 4 : 31 – 35 .
- Jenike , A.W. 1964 . Storage and Flow of Solids In Bulletin of the University of Utah, 53, 26, Bulletin No. 123 of the Utah Engineering Experiment Station, Town of Publication .
- Jones , T.M. 1968 . Measuring cohesion in powders . Manufacturing Chemist and Aerosol News , 39 : 38 – 40 .
- Head , K.H. 1982 . Manual of Soil Laboratory Testing Vol. 2 , 581 – 585 . London : Pentech Press .
- Chen , X.D. , Rennie , P.R. and Mackereth , A.R. 1998 . A study of the cohesiveness of whole milk powder prepared using a rapid humidification technique . Chemeca'98, Rotorua, New Zealand, on CD rom ,
- Keey , R.B. 1992 . Drying of Loose and Particulate Materials New York : Hemisphere Publishing Corporation .