Abstract
Cancer has become one of the leading causes of morbidity and mortality worldwide. This disease is classified broadly by tissue, organ, and system; different cancer types and subtypes require different treatments. Drug bioavailability, selectivity, and high dosage, as well as extended treatment, are significantly associated with the development of resistance – a complex problem in cancer therapy. It is expected that the combination of anticancer drugs and drug delivery systems, using polymers to increase the access of such agents to their site of action, will improve the efficacy of therapy. Polyethyleneimine (PEI) is a polymer used as a co-delivery system for anticancer drugs and gene therapy. PEI is also useful for other purposes, such as transfection and bio-adsorbent agents. In co-delivery, PEI can promote drug internalization. However, PEI with a high molecular weight is linked to higher cytotoxicity, thus requiring further evaluation of clinical safety. This review focuses on the utilization of PEI as a co-delivery system for anticancer therapy, as well as its potential to overcome resistance, particularly in the treatment of specific subtypes (eg, breast cancer). In conclusion, PEI has promising applications and is improvable for the development of anticancer drugs.
Introduction
Cancer is a disease that causes abnormal and uncontrolled cell growth with the potential to invade or metastasize to other parts of the body. It has become a major public health problem and a leading cause of mortality worldwide.Citation1 In women, the most prevalent type of cancer is breast cancer (a histopathologically and molecularly heterogeneous type). The classification of breast cancer into subtypes is based on the clinical stage, histopathologic features, hormone indicator, growth factor expression, biomarkers, and molecular profiling.Citation2 Despite research and technological developments, unfortunately, the variability of cancer poses a significant challenge to accurate diagnosis and treatment efficacy.Citation3,Citation4
There are numerous reports on the occurrence of drug resistance in cancer therapy.Citation5 Some anticancer drugs are characterized by low bioavailability, low selectivity, and the need for high dosage to reach their target, and are associated with the development of multi-drug resistance.Citation6 Several factors cause this resistance; for example, intracellular adenosine triphosphates (ATPs) play a significant role in promoting resistance to anticancer drugs. Also, extracellular ATPs can induce drug resistance. The avoidance or blockage of ATP internalization by combinational therapy may increase the cellular uptake of anticancer drugs.Citation7,Citation8 Nevertheless, combining two or more anticancer therapeutics leads to an increase in toxicity on healthy cells.
The use of nanoparticle-based (NP-based) drug delivery systems may reduce the occurrence of drug resistance. Different types of NPs (eg, organic, inorganic, and hybrid) have been used in anticancer therapy. Organic NPs have been investigated for an extended period of time and involve many materials. This type includes liposome-based, polymer-based, and dendrimer NPs.Citation9 Liposome-based NPs were the first nanomedicine to be approved for clinical use.Citation10 Polymer-based NPs are considerably more specific for drug delivery on resistant cancer cells than liposome-based NPs.Citation11 Various polymer-based NPs have been used in breast cancer therapy, including lipid combinations (eg, cholesterol) to form lipo-polymeric NPs.Citation12
Polyethyleneimine (PEI) is a cationic polymer containing repeating units of primary amino groups and ethylene (CH2CH2). PEI is commonly used as a transfection reagent and nanocarrier for drug delivery systems to enhance the efficacy of targeted drugs and gene therapy.Citation13 A Phase I clinical trial of PEI has been conducted for a DNA vaccine administered as a polymeric complex.Citation14 However, there is a lack of clinical studies on the use of PEI in the treatment of cancer. The safety of PEI has always been an important consideration for clinical use. PEI is one of the polymers used to coat the combination of anticancer drugs and gene therapy. Differences in its characteristics can affect the ability of PEI for drug delivery. The use of polymer-based nanocarriers as co-delivery systems for anticancer drugs and gene-targeted therapy has been reported. Nevertheless, there is a limited number of studies using PEI for co-delivery in cancer, particularly in the treatment of specific subtypes such as breast cancer.Citation15–Citation17 This review focuses on the utilization of PEI as a co-delivery system for anticancer drugs and gene therapy, as well as its potential to overcome resistance.
Methods
Relevant literature was collected from the PubMed database using the following terms: “co-delivery,” “cancer,” and “breast cancer”; only articles published in English were screened. The relevant research articles published from 2016 to 2021 were included according to the criteria original research articles, namely investigations on PEI combined with anticancer drugs for co-delivery cancer with or without other supportive components. Related articles on PEI as a co-delivery system for the treatment of cancer were selected, and review articles and non-cancer research publications were excluded. shows the flowchart of the methodology.
PEI Structure
PEI is also termed polyfunctional aziridine (polyaziridine) due to its origin (ie, polymerization process from the ring-opening of aziridine). The protonated amino nitrogen chain on every third atom renders this polymer as the highest positively charged density potential.Citation18 It was reported that PEI has two different structures: H(NHCH2CH2)nNH2 for branched PEI and (CH2CH2NH)n for linear PEI ().Citation19–Citation21
Figure 2 Structure of linear PEI (A) and branched PEI (B).
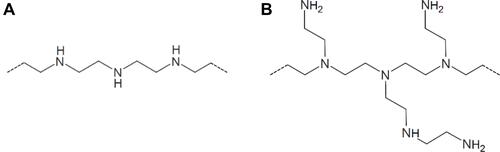
The different PEI compositions provide various properties and reactivities to particular structures.Citation21 In addition, the molecular weight of PEI varies broadly, and high-molecular-weight-PEI is associated with increased cytotoxicity. Also, the effects of PEI on DNA damage are considered based on the polymer/DNA (C/P) ratios. Thus, PEI with different structures and molecular weights in different C/P ratios will exert variable DNA-damaging effects, thereby causing cell death.Citation22 Previously, Wightman et al reported that, among all PEI/DNA complexes, linear-PEI exhibits better transfection efficiency than branch-PEI under salt conditions.Citation23 A subsequent report showed that branch-PEI is more toxic than linear-PEI; additionally, it is more effective for drug delivery because its structure exhibits better interaction with subcellular moieties.Citation24 However, the study of diverse aspects of PEI use is currently in progress.
PEI as a Drug Co-Delivery System
PEI has been utilized for several purposes and is one of the preferable options due to its efficiency and cost-effectiveness. It is used to transfect nucleic acids to the nucleus, and numerous reports showed that PEI is a potential co-delivery system for various types of combinations (eg, amine-functionalized biochar modified with PEI and PEI-functionalized magnetic Fe3O4 system). Both systems enhanced drug release and function.Citation25,Citation26 Moreover, PEI and its modification may be used for gene delivery using N-acetyl-L-leucine (N-Ac-L-Leu-PEI) to effectively deliver CpG oligodeoxynucleotides (CpG-ODN). This is a crucial component in resisting inflammation, it is involved in the bone resorption process, and enhances cell apoptosis.Citation27
Yoshitomi et al discovered an increase in the accumulation of astaxanthin in Haematococcus pluvialis cells following the addition of PEI. The polymer caused excessive production of reactive oxygen species and increased oxidative stress, thereby leading to the increment of astaxanthin accumulation.Citation28 The application of PEI is broad and versatile. Many research studies use PEI as a co-delivery system for anticancer drugs, cancer gene therapy, and distinct functions to enhance drug adsorption or accumulation.
PEI as a Co-Delivery System for Anticancer Drugs
The combination of anticancer drugs has been used to overcome drug resistance in cancer therapy. The goal of the combination of two anticancer drugs is to increase efficacy without inducing more adverse effects. Furthermore, polymers are used as drug delivery systems to promote the entry of combinations of anticancer drugs to their site of action, mainly in the cell nucleus. PEI is a cationic polymer that can enter the nucleus. Some research studies reported employing PEI as a co-delivery system for breast cancer therapy () and the treatment of other types of cancer (). It has been shown that this system increases the anticancer effects of agents by enhancing drug uptake, selectivity, stability, and biocompatibility.
Table 1 PEI as Co-Delivery System for Cancer Therapy
Table 2 PEI as Co-Delivery System for Breast Cancer Therapy
Li et al, Zhao et al, and Sharma et al used branch PEI as the co-delivery system for anticancer drugs, such as paclitaxel (PTX).Citation29–Citation31 These co-delivery systems increased therapeutic efficacy without causing more adverse reactions. The cytotoxicity of PTX and epirubicin was increased, and the presence of PEI in NPs enhanced drug uptake. Moreover, treatment of human embryonic kidney cell lines with NPs did not lead to significant cytotoxicity, highlighting the selectivity of NPs.
Another study combining poly(2-ethyl-2-oxazoline)-co-poly(ethyleneimine) (PEtOx-co-PEI) with poly(E-caprolactone) (PCL) as PEtOx-co-PEI-b-PCL block copolymers showed high stability and effective delivery of doxorubicin (DOX). These effects occurred because PEI as copolymers decreased the metabolic activity of breast cancer cell lines.Citation32 Zhou reported that the combination of grafted ruthenium pro-drug (Ru) with cholic acid (CA) and PEI–ZnO-SiO2 to form core-shell nanoparticles (CSNPs) (Ru–CA–PEI–ZnO–SiO2 CSNPs) demonstrated antitumor activity against HeLa cells.Citation33 The co-delivery system for anticancer drugs (ie, PTX, DOX, and Ru) resulted in excellent drug biocompatibility and may be a promising system for drug delivery in the treatment of cancer.
PEI as a Co-Delivery System for Gene Therapy
Recently, the use of gene-targeted therapy for the treatment of cancer has also been accepted. PEI is an established transfection reagent that can deliver nucleic acids to the nucleus. The utilization of PEI as a delivery system for gene-targeted therapy is the most coherent approach and expected to provide substantial scientific evidence. In study by Weecharangsan et al, PEI was used as a co-delivery system for a plasmid constructed with gene-targeted therapy or small interfering RNA (siRNA).Citation35 The findings indicated that the use of this system could reduce toxicity in normal cells, increase the efficiency of gene transfection, and enhance the inhibition of cancer cells. In vitro analysis showed that the combination of PEI with siRNA could increase the efficiency of gene transfection and reduce toxicity in normal cells.Citation34,Citation35 Furthermore, another research study showed high transfection rates in BFF, HEK293, and HepG2 cells following treatment with PEI/plasmid DNA (PEI/pDNA) and PEI/siRNA complexes. Thus, PEI complexes with pDNA and siRNA may provide a new cost-effective strategy for transfection. PEI/siRNA complexes also induced a reduction in tumor volume, as a result of efficient drug delivery.Citation36,Citation37 PEI is also useful for a DNA vaccination complex system, consisting of a tumor-specific antigen termed Dickkopf-1 (DKK-1) and the immune checkpoint termed programmed death-ligand 1 (PD-L1) complexed with poly-(lactic-co-glycolic) acid/ PEI (PLGA/PEI). The treatment led to significant inhibition of tumor growth and induction of a transient inflammatory reaction, as well as maintenance of stable body weight and health. These findings confirmed that this tumor vaccine was effective with limited toxicity.Citation38
Subsequent research studies used polyethylene glycol (PEG)- and PEI-complexed siRNA and marker peptides. The data showed that the combination could escape from lysosome activity and successfully release the gene. This combination could suppress tumor growth in folate receptor-positive ovarian cancer or similar cancer cells.Citation39,Citation40 GALA belongs to a group of pH-sensitive peptides that can penetrate cells and protect the drug from endocytosis by endosomes.Citation41 CREKA is a pentapeptide linear cysteine-arginine glutamic acid-lysine-alanine, utilized as a tumor-homing peptide. Using the nano-delivery system, CREKA showed high selectivity for triple-negative breast cancer (TNBC).Citation42 GALA- and CREKA-modified PEI with siRNA complexes showed excellent transfection efficiency with good biocompatibility and profiled redox-sensitive release property that is suitable for siRNA delivery system in tumor condition. Moreover, siRNA was protected from RNA enzymatic degradation by the complex. The complexes showed significant cytotoxicity against MDA-MB-231 cells via the BDR4-c-Myc and EGFR/PI3K/Akt pathway. Collectively, the available evidence indicates that this combination provides efficient delivery of siRNA for the treatment of TNBC.
PEI as a Co-Delivery System for Anticancer Drug and Gene Therapy
Recently, research studies combined anticancer drugs and gene therapy to overcome significant efficacy. It is expected that the use of a co-delivery system that effectively delivers the agent to the target will enhance the combined effect of drugs and gene-targeted therapy. PEI is a robust co-delivery system due to its ability to lead the anticancer drug into the cells and transfect nucleic acid-based therapy to the nucleus. illustrates PEI nanoparticle complexes with anticancer drugs and RNA interference (RNAi).
Figure 3 Scheme of nanoparticles containing PEI as drug and RNAi delivery.
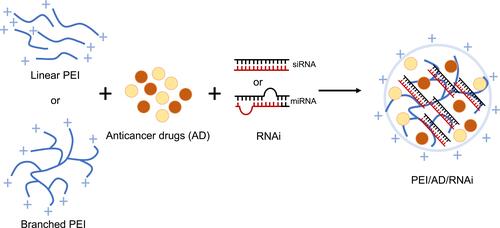
The formation of a co-polymer system by loading DOX into PEI along with pDNA, short hairpin RNA (shRNA), and siRNA showed higher cytotoxicity than treatment with DOX only. Drug release was examined under several pH conditions, and pH adjustment to 5.0 showed that >80% of DOX was released. The PEI complex has exhibited excellent biocompatibility, reliable delivery of DOX and DNA, and protection of DNA from degradation by DNase I.Citation43–Citation51 It is established that the intracellular uptake of PEI nanoparticles is dominated by clathrin- and caveolae-independent endocytosis and an energy-dependent process, as shown in .Citation49 The PEI co-delivery system has shown a good capability for the delivery of genes, and the transfection efficiency improved following the increase in weight ratios. Thus, the combination of DNA and DOX exerted synergistic apoptotic effects, and the PEI co-delivery system significantly reduced tumor volume in vivo.Citation50
Figure 4 Mechanism of action of PEI for drug and nucleic acid internalization via endocytosis.
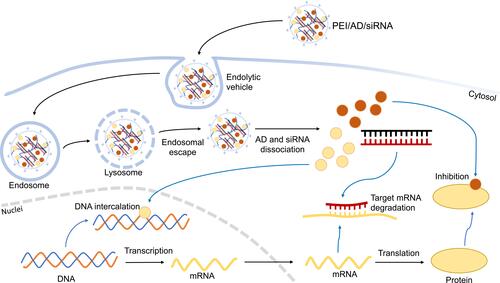
Research using a complex composed of docetaxel combined with PEI and pDNA yielded similar results. In vivo transfection analysis in tumor-bearing mice showed that this system significantly improved the transfection efficiency.Citation52,Citation53
Wang et al revealed that the co-loading of tumor necrosis factor-related apoptosis-inducing ligand plasmid (pTRAIL) and gambogic acid (GA) significantly enhanced cytotoxicity against MCF-7 and MDA-MB-231 cells.Citation54 The GA encapsulated into PEI-PLGA NPs (GA/pTRAIL-HA/PPNPs) showed high cytotoxicity, indicating the effectiveness of the combination that may lead to the selective accumulation of CD44 in MDA-MB-231 cells. In vivo observation of tumor-bearing nude BALB/c mice showed that both GA and pTRAIL separately loaded in NPs could significantly enhance tumor inhibition. Moreover, the combination of GA and pTRAIL yielded more significant results. The hyaluronic acid-PPNP co-delivery system for GA and pTRAIL represents a potential strategy for the treatment of TNBC through the combination of anticancer drugs and therapeutic DNA. Methotrexate (MTX) has been loaded to PEI NPs in combination with pDNA and siRNA.Citation55,Citation56 The cytotoxic effects of both PEI with pDNA and siRNA polyplexes on HeLa cells was observed. Linear-PEI did not show cytotoxicity, but demonstrated significant biocompatibility. Branch-PEI/pDNA complexes are less biocompatible, but induce more cytotoxicity than linear PEI.Citation56 Furthermore, PEI with siRNA polyplexes showed higher cytotoxicity and protein inhibition in HeLa cells versus PEI with pDNA. In vivo analysis showed that mice treated with M-MTX/siRNA had lower tumor weight, indicating that the delivery system is a more effective anticancer agent versus free MTX.
Other research studies have used PEI to deliver anticancer drugs along with pDNA and/or siRNA, as well as increase therapeutic responses. Drugs that have been used include retinoic acid, embelin, 4-(N)-stearoyl gemcitabine (GemC18), and DOX.Citation57–Citation62 The complex increased apoptosis in cancer cells, but had a minimal effect on nonmalignant cells. In vivo analysis of this system in tumor-bearing mice showed markedly low tumor volume and high therapeutic response.Citation57
The results of a tumor accumulation assay showed that PEG shells effectively protects siRNA from cell interaction and recognition by polymeric micelles in the reticuloendothelial system.Citation60 Delivery of DOX with CD44-specific-siRNA transfection led to the downregulation of CD44, matrix metallopeptidase 9 (MMP9), vascular endothelial growth factor, and C-X-C motif chemokine receptor 4 (CXCR4), thereby reducing the inhibition of apoptosis by CD44.Citation62 Taken together, the evidence shows that PEI can be used as a drug co-delivery system and gene-transfection reagent to overcome resistance in the treatment of cancer.
PEI as a Co-Delivery System for Breast Cancer Therapy
Breast cancer is one of the leading causes of cancer-related death among women. Previously, all patients with breast cancer were receiving the same treatment. More recently, research revealed the presence of different types of breast cancer that led to various clinical findings and the development of specific treatments. The classification of breast cancer is based on histological and molecular characteristics. The relevant molecular subtypes reported in breast cancer is triple-negative breast cancer (TNBC), that has specific treatment response behavior among all subtypes of breast cancer.
TNBC is negative for the typical receptors in breast cancer: estrogen receptors; progesterone receptors; and excess human epidermal growth factor receptor-2 (HER2).Citation63,Citation64 This type of breast cancer is more aggressive and associated with a poor prognosis versus other types of the disease. Owing to its specific features, TNBC does not respond to hormonal therapy or HER2-targeted medicine; hence, its treatment involves a combination of surgery, radiation therapy, and chemotherapy.
The development of drug resistance poses challenges to the treatment of breast cancer. Moreover, the development of high-efficiency/low-toxicity agents is crucial. Therefore, developing a suitable co-delivery system for breast cancer is also essential to enhance bioavailability and cellular uptake. The development of a potent anticancer agent with a multifunctional molecule characterized by high specificity and capability for delivery is challenging.
Based on the reviewed literature, PEI has been used in various research fields of cancer therapy as a polymeric complex along with anticancer drugs and/or genetic substances. Numerous types of cancer cells respond well to treatment with an NP complex composed of PEI. Thus, this approach could potentially improve cancer therapy.
Recently, PEI was utilized as a co-delivery system for the anticancer drug DOX, and in vitro study on MCF-7 cells confirmed an improvement in the anticancer effect.Citation32 As a gene therapy co-delivery system, PEI can efficiently deliver siRNA to the target, and induce an increase in cytotoxicity and tumor growth suppression.Citation40,Citation57 Moreover, as the co-delivery system for the combination of drug and gene therapy, PEI has improved the cytotoxic effect on breast cancer cell lines and reduced tumor volume in a xenograft model.Citation43,Citation45,Citation47,Citation50,Citation54,Citation59,Citation60,Citation62 Taken together, PEI shows excellent ability as a co-delivery system in breast cancer therapy. Further investigation is warranted to improve cancer therapy, particularly for breast cancer.
Conclusion
PEI is a cationic polymer utilized as a co-delivery system for drugs and gene therapy. The PEI co-delivery system improves the anticancer effect by enhancing drug selectivity for malignant cells, uptake, stability, and biocompatibility. PEI has been used for the delivery of anticancer drugs, such as DOX, PTX, epirubicin, Ru, docetaxel, MTX, retinoic acid, GemC18, perfluorodecalin, GA, and embelin. PEI co-delivery of a plasmid constructed with gene-targeted therapy, pDNA, or siRNA reduced toxicity in normal cells, increased the efficiency of gene transfection, and improved the inhibition of cancer cells. For the treatment of breast cancer, the combination of drugs with PEI induced higher cytotoxicity against breast cancer cell lines versus treatment with the drugs only. Moreover, it significantly reduced the tumorigenic ability in a xenograft model. Thus, PEI has promising applications and is improvable for the development of anticancer drugs.
Acknowledgment
This research study was funded by the Indonesian Ministry of Research, Technology and Higher Education, under The World Class Research Scheme.
Disclosure
The authors report no conflicts of interest in this work.
References
- Hassanpour SH, Dehghani MA. Review of cancer from perspective of molecular. J Cancer Res Pract. 2017;4:127–129. doi:10.1016/j.jcrpr.2017.07.001
- Zubair M, Wang S, Ali N. Advanced approaches to breast cancer classification and diagnosis. Front Pharmacol. 2021;11:1–24. doi:10.3389/fphar.2020.632079
- Meacham CE, Morrison SJ. Tumour heterogeneity and cancer cell plasticity. Nature. 2013;501:328–337. doi:10.1038/nature12624
- Fisher R, Pusztai L, Swanton C. Cancer heterogeneity: implications for targeted therapeutics. Br J Cancer. 2013;108:479–485. doi:10.1038/bjc.2012.581
- Vasan N, Baselga J, Hyman DM. A view on drug resistance in cancer. Nature. 2019;575(7782):299–309. doi:10.1038/s41586-019-1730-1
- Senapati S, Mahanta AK, Kumar S, Maiti P. Controlled drug delivery vehicles for cancer treatment and their performance. Signal Transduct Target Ther. 2018;3(1):1–19. doi:10.1038/s41392-017-0004-3
- Mokhtari RB, Homayouni TS, Baluch N, et al. Combination therapy in combating cancer systematic review: combination therapy in combating cancer background. Oncotarget. 2017;8(23):38022–38043.
- Wang X, Zhang H, Chen X. Drug resistance and combating drug resistance in cancer. Cancer Drug Resist. 2019;2(2):141–160. doi:10.20517/cdr.2019.10
- Yao Y, Zhou Y, Liu L, et al. Nanoparticle-based drug delivery in cancer therapy and its role in overcoming drug resistance. Front Mol Biosci. 2020;7:1–14. doi:10.3389/fmolb.2020.00193
- Zylberberg C, Matosevic S. Pharmaceutical liposomal drug delivery: a review of new delivery systems and a look at the regulatory landscape. Drug Deliv. 2016;23(9):3319–3329. doi:10.1080/10717544.2016.1177136
- Amreddy N, Babu A, Muralidharan R, et al. Recent Advances in Nanoparticle-Based Cancer Drug and Gene Delivery. Vol. 137. 1st ed. Elsevier Inc.; 2018. doi:10.1016/bs.acr.2017.11.003
- Sharma S, Pukale SS, Sahel DK, et al. Folate-targeted cholesterol-grafted lipo-polymeric nanoparticles for chemotherapeutic agent delivery. AAPS Pharm Sci Tech. 2020;21(7):1–21. DOI:10.1208/s12249-020-01812-y
- Park K. Biomaterials for Cancer Therapeutics. Woodhead Publishing; 2014. doi:10.1533/9780857096760
- Meleshko AN, Petrovskaya NA, Savelyeva N, Vashkevich KP, Doronina SN, Sachivko NV. Phase I clinical trial of idiotypic DNA vaccine administered as a complex with polyethylenimine to patients with B-cell lymphoma. Hum Vaccines Immunother. 2017;13(6):1398–1403. doi:10.1080/21645515.2017.1285477
- Sun H, Yarovoy I, Capeling M, Cheng C. Polymers in the co-delivery of siRNA and anticancer drugs for the treatment of drug-resistant cancers. Top Curr Chem. 2017;375(2):1–30. doi:10.1007/s41061-017-0113-z
- Hashemi M, Shamshiri A, Saeedi M, Tayebi L, Yazdian-Robati R. Aptamer-conjugated PLGA nanoparticles for delivery and imaging of cancer therapeutic drugs. Arch Biochem Biophys. 2020;691:108485. doi:10.1016/j.abb.2020.108485
- Ryu JH, Yoon HY, Sun IC, Kwon IC, Kim K. Tumor-targeting glycol chitosan nanoparticles for cancer heterogeneity. Adv Mater. 2020;32(51):1–40. doi:10.1002/adma.202002197
- Yuan W, Li H. Polymer-Based Nanocarriers for Therapeutic Nucleic Acids Delivery. Elsevier Inc.; 2017. doi:10.1016/b978-0-323-46143-6.00014-2
- Merck KGaA. Polyethylenimine, branched; 2021. Available from: https://www.sigmaaldrich.com/ID/en/substance/polyethyleniminebranched1234525987068. Accessed September 11, 2021.
- Merck KGaA. Polyethylenimine, linear; 2021. Available from: https://www.sigmaaldrich.com/ID/en/substance/polyethyleniminelinear123459002986?context=product. Accessed September 11, 2021.
- Polymer Properties Database. Polyethyleneimine (Polyaziridine); 2021. Available from: https://polymerdatabase.com/PolymerBrands/Polyethyleneimine.html. Accessed March 23, 2022.
- Gholami L, Sadeghnia HR, Darroudi M, Kazemi Oskuee R. Evaluation of genotoxicity and cytotoxicity induced by different molecular weights of polyethylenimine/DNA nanoparticles. Turkish J Biol. 2014;38(3):380–387. doi:10.3906/biy-1309-51
- Wightman L, Kircheis R, Rössler V, et al. Different behavior of branched and linear polyethylenimine for gene delivery in vitro and in vivo. J Gene Med. 2001;3(4):362–372. doi:10.1002/jgm.187
- Omidi Y, Kafil V, Cytotoxic impacts of linear and branched polyethylenimine nanostructures in A431 cells. BioImpacts. 2011;1(1):23–30. doi:10.5681/bi.2011.004
- Li T, Tong Z, Gao B, Li YC, Smyth A, Bayabil HK. Polyethyleneimine-modified biochar for enhanced phosphate adsorption. Environ Sci Pollut Res. 2020;27(7):7420–7429. doi:10.1007/s11356-019-07053-2
- Rouhani P, Singh RN. Polyethyleneimine-FUNCTIONALIZED MAGnetic Fe 3 O 4 and nanodiamond particles as a platform for amoxicillin delivery. J Nanosci Nanotechnol. 2020;20(7):3957–3970. doi:10.1166/jnn.2020.17896
- Wang H, Yu W, Zheng Y, Chen Z, Lin H, Shen Y. N -acetyl-l-leucine-polyethyleneimine-mediated delivery of CpG oligodeoxynucleotides 2006 inhibits RAW264.7 cell osteoclastogenesis. Drug Des Devel Ther. 2020;Volume 14:2657–2665. doi:10.2147/DDDT.S241826
- Yoshitomi T, Shimada N, Iijima K, Hashizume M, Yoshimoto K. Polyethyleneimine-induced astaxanthin accumulation in the green alga Haematococcus pluvialis by increased oxidative stress. J Biosci Bioeng. 2019;128(6):751–754. doi:10.1016/j.jbiosc.2019.06.002
- Li Y, Guo M, Lin Z, et al. Polyethylenimine-functionalized silver nanoparticle-based co-delivery of paclitaxel to induce HepG2 cell apoptosis. Int J Nanomedicine. 2016;11:6693–6702. doi:10.2147/IJN.S122666
- Zhao MD, Li JQ, Chen FY, et al. Co-delivery of curcumin and paclitaxel by “core-shell” targeting amphiphilic copolymer to reverse resistance in the treatment of ovarian cancer. Int J Nanomed. 2019;14:9453–9467. doi:10.2147/IJN.S224579
- Sharma N, Kumari RM, Gupta N, Syed A, Bahkali AH, Nimesh S. Poly-(lactic-co-glycolic) acid nanoparticles for synergistic delivery of epirubicin and paclitaxel to human lung cancer cells. Molecules. 2020;25:18. doi:10.3390/molecules25184243
- Ozturk N, Kara A, Gulyuz S, et al. Exploiting ionisable nature of PEtOx-co-PEI to prepare pH sensitive, doxorubicin-loaded micelles. J Microencapsul. 2020;37:467–480. doi:10.1080/02652048.2020.1792566
- Zhou W. Cholic acid-functionalized mesoporous silica nanoparticles loaded with ruthenium pro-drug delivery to cervical cancer therapy. J Inorg Organomet Polym Mater. 2021;31(1):311–318. doi:10.1007/s10904-020-01710-7
- Wu Z, Zhan S, Fan W, et al. Peptide-mediated tumor targeting by a degradable nano gene delivery vector based on pluronic-modified polyethylenimine. Nanoscale Res Lett. 2016;11(1):1–13. doi:10.1186/s11671-016-1337-5
- Weecharangsan W, Opanasopit P, Niyomtham N, Yingyongnarongkul BE, Kewsuwan P, Lee RJ. Synergistic inhibition of human carcinoma cell growth via co-delivery of p53 plasmid DNA and bcl-2 antisense oligodeoxyribonucleotide by cholic acid-modified polyethylenimine. Anticancer Res. 2017;37(11):6335–6340. doi:10.21873/anticanres.12085
- Forcato DO, Fili AE, Alustiza FE, et al. Transfection of bovine fetal fibroblast with polyethylenimine (PEI) nanoparticles: effect of particle size and presence of fetal bovine serum on transgene delivery and cytotoxicity. Cytotechnology. 2017;69(4):655–665. doi:10.1007/s10616-017-0075-6
- Tong WY, Alnakhli M, Bhardwaj R, et al. Delivery of siRNA in vitro and in vivo using PEI-capped porous silicon nanoparticles to silence MRP1 and inhibit proliferation in glioblastoma. J Nanobiotechnol. 2018;16(1):1–17. doi:10.1186/s12951-018-0365-y
- Guo S, Xiao P, Li B, et al. Co-immunizing with PD-L1 induces CD8+ DCs-mediated anti-tumor immunity in multiple myeloma. Int Immunopharmacol. 2020;84:106516. doi:10.1016/j.intimp.2020.106516
- Wang Y, Sun G, Gong Y, Zhang Y, Liang X, Yang L. Functionalized folate-modified graphene Oxide/PEI siRNA nanocomplexes for targeted ovarian cancer gene therapy. Nanoscale Res Lett. 2020;15(1). doi:10.1186/s11671-020-3281-7
- Zhang C, Yuan W, Wu Y, Wan X, Gong Y. Co-delivery of EGFR and BRD4 siRNA by cell-penetrating peptides-modified redox-responsive complex in triple negative breast cancer cells. Life Sci. 2021;266:118886. doi:10.1016/j.lfs.2020.118886
- Nishimura Y, Takeda K, Ezawa R, Ishii J, Ogino C, Kondo A. A display of pH-sensitive fusogenic GALA peptide facilitates endosomal escape from a bio-nanocapsule via an endocytic uptake pathway. J Nanobiotechnol. 2014;12(1):2–7. doi:10.1186/1477-3155-12-11
- Zhou Z, Qutaish M, Han Z, et al. MRI detection of breast cancer micrometastases with a fibronectin-targeting contrast agent. Nat Commun. 2015;6:1–11. doi:10.1038/ncomms8984
- Ng KE, Amin MCIM, Katas H, et al. pH-responsive triblock copolymeric micelles decorated with a cell-penetrating peptide provide efficient doxorubicin delivery. Nanoscale Res Lett. 2016;11(1). doi:10.1186/s11671-016-1755-4
- Jia H, Chen S, Zhuo R, Feng J, Zhang X. Polymeric prodrug for bio-controllable gene and drug co-delivery. Sci China Chem. 2016;59(11):1397–1404. doi:10.1007/s11426-016-0230-9
- Ebrahimian M, Taghavi S, Mokhtarzadeh A, Ramezani M, Hashemi M. Co-delivery of doxorubicin encapsulated PLGA nanoparticles and Bcl-xL shRNA using alkyl-modified PEI into breast cancer cells. Appl Biochem Biotechnol. 2017;183(1):126–136. doi:10.1007/s12010-017-2434-3
- Wang T, Yu X, Han L, Liu T, Liu Y, Zhang N. Tumor microenvironment dual-responsive core–shell nanoparticles with hyaluronic acid-shield for efficient co-delivery of doxorubicin and plasmid DNA. Int J Nanomed. 2017;12:4773–4788. doi:10.2147/IJN.S134378
- Chen L, Ji F, Bao Y, et al. Biocompatible cationic pullulan-g-desoxycholic acid-g-PEI micelles used to co-deliver drug and gene for cancer therapy. Mater Sci Eng C. 2017;70:418–429. doi:10.1016/j.msec.2016.09.019
- Li Y, Zhang X, Zhang J, et al. Synthesis and characterization of a hyperbranched grafting copolymer PEI-g-PLeu for gene and drug co-delivery. J Mater Sci Mater Med. 2018;29(5). doi:10.1007/s10856-018-6057-1
- Sun X, Li M, Yang Y, Jia H, Liu W. Carrier-free nanodrug-based virus-surface-mimicking nanosystems for efficient drug/gene co-delivery. Biomater Sci. 2018;6(12):3300–3308. doi:10.1039/c8bm01033a
- Feng L, Yan S, Zhu Q, et al. Targeted multifunctional redox-sensitive micelle co-delivery of DNA and doxorubicin for the treatment of breast cancer. J Mater Chem B. 2018;6(20):3372–3386. doi:10.1039/c8tb00748a
- Tian G, Pan R, Zhang B, et al. Liver-targeted combination therapy basing on glycyrrhizic acid-modified DSPE-PEG-PEI nanoparticles for co-delivery of doxorubicin and Bcl-2 siRNA. Front Pharmacol. 2019;9:1–13. doi:10.3389/fphar.2019.00004
- Dong S, Zhou X, Yang J. TAT modified and lipid – PEI hybrid nanoparticles for co-delivery of docetaxel and pDNA. Biomed Pharmacother. 2016;84:954–961. doi:10.1016/j.biopha.2016.10.003
- Pang ST, Lin FW, Chuang CK, Yang HW. Co-delivery of docetaxel and p44/42 MAPK siRNA using PSMA antibody-conjugated BSA-PEI layer-by-layer nanoparticles for prostate cancer target therapy. Macromol Biosci. 2017;17(5):1–9. doi:10.1002/mabi.201600421
- Wang S, Shao M, Zhong Z, et al. Co-delivery of gambogic acid and TRAIL plasmid by hyaluronic acid grafted PEI-PLGA nanoparticles for the treatment of triple negative breast cancer. Drug Deliv. 2017;24(1):1791–1800. doi:10.1080/10717544.2017.1406558
- Costa D, Valente AJM, Queiroz JA, Sousa Â. Finding the ideal polyethylenimine-plasmid DNA system for co-delivery of payloads in cancer therapy. Colloids Surfaces B Biointerfaces. 2018;170:627–636. doi:10.1016/j.colsurfb.2018.06.063
- Hao F, Lee RJ, Yang C, et al. Targeted co-delivery of siRNA and methotrexate for tumor therapy via mixed micelles. Pharmaceutics. 2019;11(2):1–19. doi:10.3390/pharmaceutics11020092
- Thapa B, Kc R, Bahniuk M, et al. Breathing new life into TRAIL for breast cancer therapy: co-delivery of pTRAIL and complementary siRNAs using lipopolymers. Hum Gene Ther. 2019;30(12):1531–1546. doi:10.1089/hum.2019.096
- Magalhães M, Jorge J, Gonçalves AC, et al. miR-29b and retinoic acid co-delivery: a promising tool to induce a synergistic antitumoral effect in non-small cell lung cancer cells. Drug Deliv Transl Res. 2020;10(5):1367–1380. doi:10.1007/s13346-020-00768-7
- Xu Y, Liu D, Hu J, Ding P, Chen M. Hyaluronic acid-coated pH sensitive poly (β-amino ester) nanoparticles for co-delivery of embelin and TRAIL plasmid for triple negative breast cancer treatment. Int J Pharm. 2020;573:118637. doi:10.1016/j.ijpharm.2019.118637
- Norouzi P, Amini M, Dinarvand R, Arefian E, Seyedjafari E, Atyabi F. Co-delivery of gemcitabine prodrug along with anti NF-κB siRNA by tri-layer micelles can increase cytotoxicity, uptake and accumulation of the system in the cancers. Mater Sci Eng C. 2020;116:111161. doi:10.1016/j.msec.2020.111161
- Joshi U, Filipczak N, Khan MM, Attia SA, Torchilin V. Hypoxia-sensitive micellar nanoparticles for co-delivery of siRNA and chemotherapeutics to overcome multi-drug resistance in tumor cells. Int J Pharm. 2020;590:119915. doi:10.1016/j.ijpharm.2020.119915
- Vahidian F, Safarzadeh E, Mohammadi A, et al. siRNA-mediated silencing of CD44 delivered by Jet Pei enhanced Doxorubicin chemo sensitivity and altered miRNA expression in human breast cancer cell line (MDA-MB468). Mol Biol Rep. 2020;47(12):9541–9551. doi:10.1007/s11033-020-05952-z
- Nedeljkovi M. Mechanisms of chemotherapy resistance in triple-negative breast cancer — how we can rise to the challenge. Cells. 2019;8(9):957.
- Bai X, Ni J, Beretov J, Graham P, Li Y. Triple-negative breast cancer therapeutic resistance: where is the Achilles’ heel? Cancer Lett. 2020;497:100–111. doi:10.1016/j.canlet.2020.10.016
- Chen G, Wang K, Wu P, et al. Development of fluorinated polyplex nanoemulsions for improved small interfering RNA delivery and cancer therapy. Nano Res. 2018;11(7):3746–3761. doi:10.1007/s12274-017-1946-z