Abstract
The antioxidant activity of the aminodi(hetero)arylamines, prepared by C–N coupling of the methyl 3-aminothieno[3,2-b]pyridine-2-carboxylate with bromonitrobenzenes and further reduction of the obtained nitro compounds, was evaluated by chemical, biochemical and electrochemical assays. The aminodi(hetero)arylamine with the amino group ortho to the NH and a methoxy group in para, was the most efficient in radical scavenging activity (RSA, 63 µM) and reducing power (RP, 33 µM), while the aminodiarylamine with the amino group in para to the NH, gave the best results in β-carotene-linoleate system (41 µM) and inhibition of formation of thiobarbituric acid reactive substances in porcine brain cells homogenates (7 µM), with EC50 values even lower than those obtained for the standard trolox. This diarylamine also presented the lowest oxidation potential, lower than the one of trolox, and the highest antioxidant power in the electrochemical assays. The para substitution with an amino group enables higher antioxidant potential.
Introduction
The equilibrium between reactive oxygen species (ROS) production and antioxidant defences might be displaced either by the overproduction of ROS or by the loss of the cell antioxidant defencesCitation1. This disequilibrium is known as oxidative stress, and the excess of ROS may oxidize and damage cellular lipids, proteins and DNA, leading to their modification and inhibiting their normal functionCitation2. The discovery of the roles played by ROS on the cell has generated a great interest on compounds with antioxidant activity. There is no doubt about the beneficial effects of antioxidants on pathological conditions like ischemia-reperfusion, cancer and various types of inflammation, where ROS play a pivotal role in the disease onsetCitation3.
A few examples are the synthetic antioxidants edaravoneCitation4 and ebselenCitation5 () used for improving neurologic recovery following acute cerebral infarctions. Edaravone was the first drug developed for treating acute cerebral infarctions that acts as scavenger of free radicals while ebselen is still on clinical trials for acute cerebral infarction as well as for the treatment of free radical cornea injury. Although antioxidants are used as therapeutic drugs and as ingredients in dietary supplements with the purpose of maintaining health and preventing diseases, these claims are still subject to intense scientific debate.
Another application of antioxidants is as food additives such as BHT (butylated hydroxytoluene), BHA (butylated hydroxyanisole), TBHQ (tertiary-butylhydroquinone), PG (propyl gallate), OG (octyl gallate) and EQ (ethoxyquin). Nevertheless, some of these compounds have raised concerns on possible health problems they may promoteCitation6.
For these reasons, the continuous discovery of new synthetic products with antioxidant activity is of primary importance as they may substitute with advantage the currently used compounds. Diarylamines have been described as good antioxidants, especially as radical scavengers, with the ability to act as chain-breaking compounds transferring one hydrogen from the N–H group to peroxyl radicals (ROO), leading to the formation of an aminil radical (-N-)Citation7. Radical scavenging activity (RSA)Citation8,Citation9, redox propertiesCitation10,Citation11 and effects on mitochondrial bioenergeticsCitation12 of these compounds has been demonstrated by our and other research groups.
In the present work, the antioxidant activity of aminodi(hetero)arylamines in the thieno[3,2-b]pyridine, previously synthesized by usCitation13, was evaluated through their free radical scavenging effects, reducing power (RP), lipid peroxidation inhibition and electrochemical behavior. Electrochemical techniques, such as cyclic voltammetry (CV) and differential pulse voltammetry (DPV), have been applied as alternative tools for the evaluation of antioxidant activity, expressed in terms of “antioxidant power”, and provide a deeper insight into the redox-processes of oxidative stressors and antioxidantsCitation11,Citation14–16.
Materials and methods
Aminodi(hetero)arylamines
The aminodiarylamines 3 were previously synthesized by Calhelha et al.Citation13 by a palladium-catalyzed C–N Buchwald-Hartwig coupling of the methyl 3-aminothieno[3,2-b]pyridine-2-carboxylate 1 with bromonitrobenzenes, and further reduction of the obtained nitrodi(hetero)arylamines 2 ().
Standards and reagents
Methanol was analytical grade from Panreac (Lisbon, Portugal). Acetonitrile, analytical grade (Fisher Scientific, Brebieres, France), was double distilled over sodium perchlorate monohydrate, purity grade, purchased from Fluka (Sigma Chemical Co., St. Louis, MO). Tetrabutylammonium perchlorate (TBAP), electrochemical grade, was purchased from Fluka and kept at 30 °C before use. Trolox (6-hydroxy-2,5,7,8-tetramethylchroman-2-carboxylic acid) was purchase from Sigma. 2,2-Diphenyl-1-picrylhydrazyl (DPPH) was obtained from Alfa Aesar (Ward Hill, MA). Water was treated in a Mili-Q water purification system (TGI Pure Water Systems, Greenville, SC).
Solutions of the compounds
Stock solutions of the tested aminodiarylamines were prepared in methanol and acetonitrile for antioxidant activity and electrochemical assays, respectively, and kept at −20 °C. Appropriate dilutions were prepared in the corresponding solvent prior to the assays.
Evaluation of antioxidant activity
DPPH radical-scavenging activity
This assay was performed in 96-well microplates using an ELX800 Microplate Reader (Bio-Tek Instruments, Bedfordshire, UK). Each one of the different concentrations of diarylamines solutions (30 µL) was mixed with methanolic solution containing DPPH radicals (6 × 10−5 mol/L; 270 µL). The mixture was shaken vigorously and left to stand for 60 min in the dark (until stable absorption values were obtained). The reduction of the DPPH radical was determined by measuring the absorption at 515 nm. The RSA was calculated as a percentage of DPPH discoloration using the equation: % RSA = [(ADPPH − AC)/ADPPH] × 100, where AC is the absorbance of the solution when the compound has been added at a particular concentration, and ADPPH is the absorbance of the DPPH solutionCitation8,Citation9. The concentration of diarylamine solution providing 50% of RSA (EC50) was calculated from the graph of RSA percentage against compound concentration. Trolox was used as standard.
Reducing power
This assay was also performed using the Microplate Reader described above. Each one of the different concentrations of diarylamines solutions (0.5 mL) was mixed with sodium phosphate buffer (200 mmol/L, pH 6.6, 0.5 mL) and potassium ferricyanide (1% w/v, 0.5 mL). The mixture was incubated at 50 °C for 20 min, and trichloroacetic acid (10% w/v, 0.5 ml) was added. The mixture (0.8 mL) was poured into the wells of a 48-well microplate, along with deionized water (0.8 mL) and ferric chloride (0.1% w/v, 0.16 mL), and the absorbance was measured at 690 nm (8,9). The concentration of diarylamine solution providing 0.5 of absorbance (EC50) was calculated from the graph of absorbance at 690 nm against compound concentration. Trolox was used as standard.
Inhibition of β-carotene bleaching
The antioxidant activity of the compounds was evaluated by the β-carotene linoleate model system (CLS). Two millilitres of a solution of β-Carotene in chloroform (0.2 mg/mL) were mixed with linoleic acid (40 mg) and Tween 80 emulsifier (400 mg). After chloroform evaporation (40 °C, under vacuum), distilled water (100 mL) was added and the mixture was vigorously shaking. Aliquots (4.8 mL) of this emulsion were transferred into test tubes containing each one of the concentrations of diarylamines solutions (0.2 mL). The tubes were shaken and incubated at 50 °C in a water bath. As soon as the emulsion was added to each tube, the zero time absorbance was measured at 470 nm (Analytikjena 200 spectrophotometer, Jena, Germany)Citation8,Citation9. β-Carotene bleaching inhibition was calculated using the following equation: (β-carotene content after 2 h of assay/initial β-carotene content) × 100. The concentration of diarylamine solution providing 50% antioxidant activity (EC50) was calculated by interpolation from the graph of β-carotene bleaching inhibition percentage (CLS) against extract concentration. Trolox was used as standard.
Inhibition of lipid peroxidation using thiobarbituric acid reactive substances
Porcine brains were dissected and homogenized with a polytron in ice-cold Tris–HCl buffer (20 mM, pH 7.4) to produce a 1:2 (w/v) brain tissue homogenate which was centrifuged at 3000g for 10 min. An aliquot (0.1 mL) of the supernatant was incubated with the compound concentration (0.2 mL) in the presence of FeSO4 (10 µM; 0.1 mL) and ascorbic acid (0.1 mM; 0.1 mL) at 37 °C for 1 h. The reaction was stopped by the addition of trichloroacetic acid (28% w/v, 0.5 mL), followed by thiobarbituric acid (TBA, 2%, w/v, 0.38 mL), and the mixture was then heated at 80 °C for 20 min. After centrifugation at 3000g for 10 min to remove the precipitated protein, the color intensity of the malondialdehyde (MDA)–TBA complex in the supernatant was measured by its absorbance at 532 nm. The inhibition ratio (%) was calculated using the following formula: Inhibition ratio (%) = [(A − B)/A] × 100, where A and B were the absorbance of the control and the diarylamine solution, respectivelyCitation8,Citation9. The concentration of diarylamine solution providing 50% of lipid peroxidation inhibition (EC50) was calculated from the graph of antioxidant activity percentage against compound concentration. Trolox was used as standard.
Evaluation of oxidation potentials and antioxidant power
Instrumentation
CV and DPV measurements were performed on an Autolab PGSTAT 302 potentiostat/galvanostat using a closed standard three electrode cell. A platinum disk (BAS, Ø = 0.071 cm2) was used as the working electrode and a Pt foil as the counter electrode. All potentials are referred to an Ag/AgCl 3 M KCl reference electrode (Methrom, Herisau, Switzerland). Prior to use, the working electrode was polished in an aqueous suspension of 0.3 µm alumina (Beuhler, Lake Bluff, IL) on a Master-Tex (Beuhler) polishing pad, then rinsed with water. Subsequently, in a chemical treatment, the electrode was sonicated in 6 M HCl for 1 min, and then in methanol. This cleaning procedure was applied always before any electrochemical measurements.
Procedure
The diarylamines and the standard trolox were studied in acetonitrile solutions containing 1 mM of the compound and 0.1 M TBAP as the supporting electrolyte. All the solutions were analyzed immediately after preparation and the electrochemical responses recorded after the Pt electrode immersion, to minimize adsorption of species onto the electrode surface prior to the run. The voltammetric profiles were then acquired in different intervals in the range between −0.5 and 1.2 V for compounds 3a–c or −0.5 and 1.6 V for compounds 2a–c, using scan rates from 0.01 to 1 V/s. The first and subsequent cycles were recorded in order to access any adsorption phenomena common in organic compoundsCitation11. The antioxidant power was evaluated by DPV using the following operating conditions: 0.06 V pulse amplitude and 0.02 V/s as scan rate.
A calibration curve was prepared by plotting the concentration of trolox solutions between 0.05 and 2.00 mM against the current intensity of the respective DPV signals measured at peak maxima. The analytical signal of compounds (current density) was measured, converted to equivalents of trolox (mM), and expressed in terms of equivalents of trolox per mM of diarylamine. The sum of the values calculated at peak maxima for each electrochemical oxidation process was used to express the Total Electrochemical Antioxidant Power of the compound.
Results and discussion
Antioxidant activity
The evaluation of the antioxidant activity of the aminodi(hetero)arylamines 3a–c was performed using different methods: scavenging effect on DPPH radicals, RP assay, inhibition of lipid peroxidation using the CLS and TBA reactive substances (TBARS) assay. The results are displayed in . Furthermore, the antioxidant trolox was used as standard, and its results were compared with those of the compounds in terms of EC50 ().
Figure 3. (A) Scavenging activity on DPPH radicals (RSA), (B) RP (Abs at 690 nm), (C) CLS and (D) TBARS assay using brain homogenized tissue for compounds 3a–c (3a ▴; 3b ▪; 3c •). Results are the mean ± SD of three independent experiments.
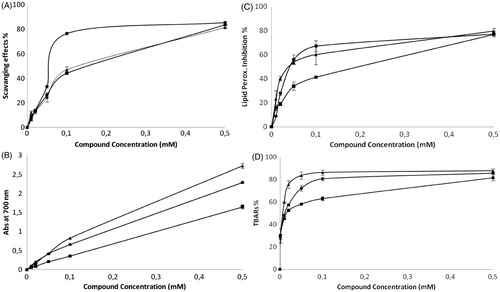
Table 1. EC50 valuesa (µM) obtained for the antioxidant activity of the diarylamines 3a–c and the standard trolox (mean ± SD; n = 3).
The RSA values of methanolic solutions of the aminodi(hetero)arylamines 3a–c were examined and compared (). Results are expressed as a percentage of the ratio of the decrease in the absorbance at 515 nm to the absorbance of DPPH solution in the absence of diarylamines at 515 nm. From the analysis of , we can conclude that these diarylamines scavenging effects on DPPH radicals increase with the concentration and the results are very good for all the compounds specially for aminodi(hetero)arylamine 3c (NH2 in the ortho and OMe in the para position relative to the NH; 85.62 ± 0.23% at 0.5 mM). The RSA slightly decreases for compounds 3b (NH2 in the ortho position; 83.37 ± 0.22% at 0.5 mM) and 3a (NH2 in the para position; 81.53 ± 0.44% at 0.5 mM). Nevertheless, at 0.1 mM the RSA of diarylamines 3b and 3c (<50%) is significantly lower than the RSA of diarylamine 3a (∼77%).
The RP of compounds 3a–c was examined as a function of their concentration in methanolic solutions (). The RP of the compounds increases with an increase in concentration. A high value of absorbance at 690 nm is related to a high RP. For diarylamines 3a and 3c the absorbance values at 690 nm are the highest (2.73 ± 0.07 and 2.29 ± 0.03 at 0.5 mM, respectively) while for compound 3b are the lowest (1.65 ± 0.05). Despite the highest absorbance observed for diarylamine 3a at the highest concentration tested (), compound 3c revealed the lowest EC50 value ().
The β-carotene undergoes a rapid decolorization in the absence of an antioxidant since the free linoleic acid radical attacks the β-carotene molecule, which loses the double bonds and, consequently loses its orange color. For compound 3a (NH2 in the para position; 79.83 ± 2.19% at 0.5 mM), the percentages of β-carotene bleaching inhibition are the highest while for compound 3b are the lowest ().
The TBARS assay measures the MDA formed as the split product of an endoperoxide of unsaturated fatty acids resulting from an oxidation of a lipid substrate. The MDA reacts with TBA to form a pink pigment that is measured spectrophotometrically at 532 nm. The substrate is oxidized with the addition of an iron ion and the extent of the oxidation is determined by an addition of TBA and spectrophotometric measurement of the product. Oxidation is inhibited by the addition of an antioxidant and therefore a reduction in the absorbance is observed. The results are typically quantified in terms of percentage of inhibition of the oxidation. The inhibition of lipid peroxidation by the three diarylamines using the TBARS assay was high (; >80%), but the highest value was obtained for compound 3a (87.89 ± 1.83 % at 0.5 mM).
The DPPH scavenging activity and RP of the nitrodi(hetero)arylamines 2a–c were also assessed, but these compounds did not show any activity. Probably, the presence of nitro groups (electro-withdrawing groups) in the phenyl ring stabilizes the N–H, avoiding the formation of the aminil radical (-N-) involved in antioxidant potential.
For an overview of the results, presents the EC50 values (mM) for the different antioxidant activity assays performed for compounds 3a–c and the standard trolox. Diarylamine 3c (NH2 in the ortho and OMe in the para position relative to the NH) was the most efficient in RSA (62.62 ± 0.77 µM) and RP (33.18 ± 2.85 µM), while compound 3a (NH2 in the para position) gave the best results in CLS (41.30 ± 3.44 µM) and TBARS (7.27 ± 0.22 µM). Unless for CLS assay, the mentioned EC50 values were lower than those obtained for the standard trolox.
Oxidation potentials and antioxidant power
The cyclic voltammograms (CV) observed for the diarylamines and for the standard trolox are shown in . Compounds 3b and 3c revealed an electrochemical behavior with two major quasi-reversible oxidation processes, while diarylamine 3a shows three major irreversible oxidation processes. The nitrodi(hetero)arylamies 2 exhibit a similar behavior in comparison to the structural equivalent aminodi(hetero)arylamines 3, however at a significantly higher potential (). This can be justified due to the electro-withdrawing effect of the nitro group. Those electrochemical profiles are different from the one obtained for the amino precursor 1 (data not shown), leading us to associate the observed oxidation processes of the di(hetero)arylamines with the different substitutions in the phenyl ring. Furthermore, the presence of an amino group in the para position promotes significant changes in the electrochemical profile, with the appearance of a new oxidation process at lower potential, 0.5 V, and the inactivation of the electrode surface. This change did not occur if the para position is occupied by the nitro group, 2a. In fact, with the continuous cycling, the electrochemical activity for the compound 3a almost vanished, , as observed in our previous workCitation5 attributed to the adsorption of electroinactive species onto the electrode surface, requiring the physical polishing of the platinum electrode. For the other compounds, this behavior is not observed and after the second cycle the voltamogram reaches a repeatable electroactive profile. It seems that the presence of the amino/nitro group in the ortho position prevents somehow the low oxidative process/adsorption on the electrode surface, possible due to some steric hindrance caused by the substituent.
Figure 4. Electrochemical responses for 1 mM nitrodi(hetero)arylamines 2, the corresponding aminodi(hetero)arylamines 3 and trolox in 0.1 M TBAP/CH3CN solutions, with Pt electrodes: (left) cyclic voltammogram at 0.1 Vs−1 between −0.5 and 1.2/1.5 V; (right) differential pulse voltammogram obtained with 0.06 V pulse amplitude at 0.02 V.s−1.
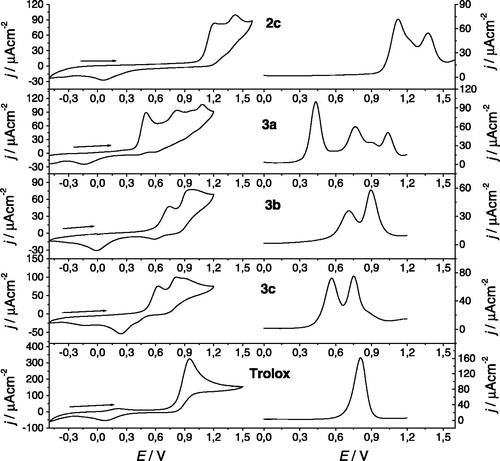
Figure 5. Cyclic voltammograms of 1 mM aminodi(hetero)arylamine 3a in 0.1 M TBAP/CH3CN solution, with Pt electrodes, between −0.5 and 1.2 V at 0.1 Vs−1: (A) first scan; (B) after several scans.
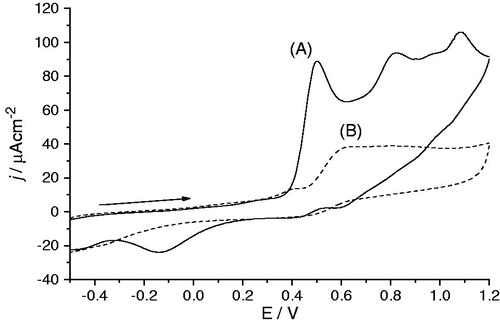
Peak potentials for the different compounds are presented in . The aminodi(hetero)arylamine 3a presented the lowest oxidation potentials, even lower than the one of trolox. Comparing compounds 3b and 3c, or 2b and 2c, the presence of a methoxy group (2c/3c), decreases the oxidation potentials probably due to a resonance stabilization of the oxidized species through the electro-donating effect. This effect was also observed by us in a previous study with diarylamines in the 2,3-dimethylbenzo[b]thiophene seriesCitation11. The dynamic behavior of the oxidation processes, scan rate dependency, revealed a semi-diffusion controlled process for the second peak of compound 3a and first peak of compounds 2a–c, 3b and 3c (considered in as Ep2), with a linear response of the anodic current density versus ν1/2, as predicted by the Randles--Sevcik equationCitation17. All the other anodic processes show a decline in the current densities for high scan rates, typical of a slow electron transfer mechanism. The presence of the amino group in the para position seems to be important for the antioxidant properties since it introduces an oxidation process at lower potentials.
Table 2. Peak potentials obtained for the electrochemical oxidation processes (CV and DPV) of the nitrodi(hetero)arylamines 2, the corresponding aminodi(hetero)arylamines 3, and the standard trolox.
The DPV, , follow a pattern similar to that of CV, but enable an easier evaluation of the antioxidant properties of the compounds. Based on the linear relationship of current density with the concentration of the electrochemical standard trolox, , diarylamine 3a gave the lowest antioxidant potentials and the highest ‘‘electrochemical antioxidant power” (EAP), , measure as trolox equivalents.
Conclusions
The aminodi(hetero)arylamine 3c with the amino group ortho to the NH and a methoxy group in para, was the most efficient in RSA (63 µM) and RP (33 µM), while compound 3a with the amino group in para to the NH, gave the best results in CLS (41 µM) and TBARS (7 µM), with EC50 values even lower than those obtained for the standard trolox. The di(hetero)arylamine 3a also presented the lowest oxidation potential, lower than the one of trolox, and the highest antioxidant power in the electrochemical assays. The amino group in the para position relative to the NH, enables a higher antioxidant potential. Furthermore, it should be mentioned that the absence of antioxidant activity observed in the nitrodi(hetero)arylamines 2 is in agreement with the high oxidation potential induced by the nitro group and verified in electrochemical analyses.
Declaration of interest
The authors report no declarations of interest.
The authors are grateful to FCT and FEDER (European Fund for Regional Development)-COMPETE/QREN/EU for financial support through the research unities PEst-C/QUI/UI686/2011 and PEst-OE/AGR/UI0690/2011, the research project PTDC/QUI-QUI/111060/2009 and the post-Doctoral grant attributed to R.C.C. (SFRH/BPD/68344/2010).
References
- Halliwell B. Reactive species and antioxidants. Redox biology is a fundamental theme of aerobic life. Plant Physiol 2006;141:312–22
- Valko M, Leibfritz D, Moncol J, et al. Free radicals and antioxidant in normal physiological function and human disease. Int J Biochem Cell Biol 2007;39:44–84
- Halliwell B. The wandering of a free radical. Free Rad Biol Med 2009;46:531–42
- Toyoda K, Fujii K, Kamouchi M, et al. Free radical scavenger, edaravone, in stroke with internal carotid artery occlusion. J Neurol Sci 2004;221:11–17
- Green AR, Ashwood T, Odergren T, Jackson DM. Nitrones as neuroprotective agents in cerebral ischemia, with particular reference to NXY-059. Pharmacol Therapeut 2003;100:195–214
- Carocho M, Ferreira ICFR. A review on antioxidants, prooxidants and related controversy: natural and synthetic compounds, screening and analysis methodologies and future perspectives. Food Chem Toxicol 2013;51:15–25
- Thomas JR. The identification of radical products from the oxidation of diphenylamine. J Amer Chem Soc 1960;82:5955–6
- Ferreira ICFR, Queiroz M-JRP, Vilas-Boas M, et al. Evaluation of the antioxidant properties of diarylamines in the benzo[b]thiophene series by free radical scavenging activity and reducing power. Bioorg Med Chem Lett 2006;16:1384–7
- Queiroz M-JRP, Ferreira ICFR, Calhelha RC, Estevinho LM. Synthesis and antioxidant activity evaluation of new 7-aryl or 7-heteroarylamino-2,3-dimethylbenzo[b]thiophenes obtained by Buchwald–Hartwig C–N cross-coupling. Bioorg Med Chem 2007;15:1788–94
- Esteves MA, Narender N, Marcelo-Curto MJ, Gigante B. Synthetic derivatives of abietic acid with radical scavenging activity. J Nat Prod 2001;64:761–6
- Abreu RMV, Falcão S, Calhelha RC, et al. Insights in the antioxidant activity of diarylamines from the 2,3-dimethylbenzo[b]thiophene through the redox profile. J Electroanal Chem 2009;628:43–7
- Pinto-Basto D, Silva JP, Queiroz M-JRP, et al. Di(hetero)arylamines in the benzo[b]thiophene series as novel potent antioxidants. Mitochondrion 2009;9:17–26
- Calhelha RC, Ferreira ICFR, Peixoto D, et al. Aminodi(hetero)arylamines in the thieno[3,2-b]pyridine series: synthesis, effects in human tumor cells growth, cell cycle analysis, apoptosis and evaluation of toxicity using non-tumor cells. Molecules 2012;17:3834–43
- Korotkova EI, Karbainov YA, Shevchuk A. Study of antioxidant properties by voltammetry. J Electroanal Chem 2002;518:56–60
- Blasco AJ, Rogerio MC, González MC, Escarpa, A. Electrochemical Index” as a screening method to determine “total polyphenolics” in foods: a proposal. Analyt Chim Acta 2005;539:237–44
- Chevion S, Roberts MA, Chevion M. The use of cyclic voltammetry for the evaluation of antioxidant capacity. Free Rad Biol Med 2000;28:860–70
- Bard AJ, Faulkner LR. Electrochemical methods, fundamentals applications. NY: Wiley; 2001