Abstract
Cellular stresses, including growth factor deprivation, inflammatory cytokines or viral infection promote RAX/PACTdependent activation of the double-stranded RNA-dependent protein kinase, PKR, to phosphorylate eIF2α, resulting in translation inhibition and apoptosis. In addition, PKR has been reported to regulate p53, STAT1 and NFκB. Here, we report that RAX/PACT interacts with the SUMO E2 ligase Ubc9 to stimulate p53-Ubc9 association and reversible p53 sumoylation on lysine 386. In addition, expression of RAX/PACT in a variety of cell lines promotes p53 stability and activity to increase p53 target gene expression. Significantly, while the expression of RAX/PACT, PKR or p53 alone has little effect on the cell cycle of p53-null H1299 cells, co-expression of p53 with either RAX/PACT or PKR promotes a 25–35% increase of cells in G1. In contrast, co-expression of RAX/PACT with the sumoylation-deficient p53(K386R) mutant or with the desumoylase SENP1 fails to induce such a G1 arrest. Furthermore, co-expression of p53, RAX/PACT and the dominantnegative PKR(K296R) mutant inhibits RAX/PACT-induced, p53-dependent G1 growth arrest and expression of RAX/PACT in pkr+/+ but not pkr-/- MEF cells promotes p53 and p21 expression following gamma irradiation. Significantly, p53 stability is decreased in cells with reduced RAX/PACT or PKR following doxorubicin treatment, and expression of exogenous RAX/ PACT promotes phosphorylation of wild-type but not p53(K386R) on serine 392. Collectively, results indicate that, in response to stress, the RAX/PACT-PKR signaling pathway may inhibit p53 protein turnover by a sumoylation-dependent mechanism with promotion of p53 phosphorylation and translational activation leading to G1 cell cycle arrest.
Introduction
Our laboratory and others have observed that a broad range of apoptosis-inducing cellular stresses may activate the interferon-inducible, dsRNA-dependent serine/threonine kinase, PKR, in a mechanism dependent on the cellular activator of PKR, RAX (PKR activator X), that leads to inhibition of cell growth and apoptosis.Citation1–Citation4 Murine RAX and its independently identified, 98% identical, human ortholog PACT (PKR activator) are the only known cellular activators of PKR.Citation1,Citation5,Citation6 RAX/PACT is comprised of three dsRNA binding domains where the first two N-terminal domains are necessary for its association with dsRNA and PKR, while the C-terminal third dsRNA binding domain is required for PKR activation.Citation7,Citation8 Canonically, activated PKR phosphorylates the α subunit of eukaryotic initiation factor eIF2 to inhibit global protein synthesis.Citation9–Citation12 Expression of exogenous RAX/PACT sensitizes cells to a broad range of stresses, including IL-3 withdrawal from factor-dependent hematopoietic cells, viral infection and inflammatory cytokine or chemotherapy treatment.Citation13,Citation14 In addition, knockdown of RAX/PACT by siRNA technology or forced expression of a non-phosphorylatable, dominant-negative RAX mutant can potently inhibit stress-induced PKR activation and promote clonal cell growth.Citation13 Thus, RAX/PACT functions as a necessary upstream activator of PKR in a stress → RAX/PACT → PKR signaling axis to promote apoptosis.
Although PKR has been primarily studied for its anti-viral and anti-proliferation effects via its capacity to inhibit protein synthesis, it has also been reported that several transcription factors, including p53, NFκB and STAT1, are functionally associated with PKR, indicating PKR's role in regulation of transcription in addition to translation.Citation10,Citation15–Citation23 Significantly, PKR is among the diverse stress-activated protein kinases that have been reported to phosphorylate p53. PKR can directly associate with the C terminus of p53, and this interaction does not require dsRNA.Citation21 PKR also phosphorylates p53 on serine 392 in vitro, and basal phosphorylation of mouse p53 on serine 18 is defective in pkr−/− murine embryonic fibroblast (MEF) cells.Citation21,Citation22 Furthermore, following genotoxic stress, induction of serine 18 phosphorylation and transcriptional upregulation of p21 is drastically reduced in pkr−/− cells.Citation22 Consequently, G1 arrest following DNA damage is impaired in pkr−/− cells.Citation22 In addition, PKR-overexpressing U937 cells have been observed to possess a constitutively higher level of p53 and are more sensitive to apoptosis following TNFα treatment.Citation23 Significantly, TNFα-induced apoptosis is inhibited by reduced p53 expression in PKR-overexpressing U937 cells, while, conversely, expression of wild-type p53 in PKR-deficient U937 cells restores the susceptibility of the cells to TNFα-induced apoptosis.Citation23 These findings may indicate that p53 induction is downstream of PKR signaling following TNFα-induced apoptosis. Collectively, these results demonstrate that PKR positively modulates p53 following cellular stress.
The importance of p53 in tumor suppression is well-established in a wide variety of species, including mouse and human.Citation24 It is clear that even minimal alterations in p53 expression or activity may have profound effects on cell survival, DNA damage repair, metabolic adaptation, cell death and senescence. Therefore, tight control of p53 function is essential for maintaining cell integrity, and virtually every aspect of p53 expression, localization, stability and activity is tightly regulated. The p53 tumor suppressor protein is subject to many post-translational modifications, including phosphorylation, acetylation, modification with ubiquitin and related proteins, such as SUMO and NEDD8.Citation25–Citation28 These modifications regulate p53 at a number of levels, including control of protein turnover, subcellular localization and changes in the ability to regulate gene expression.
Sumoylation of p53 on lysine 386 requires direct association with Ubc9, the SUMO E2-type conjugating enzyme.Citation29 Interestingly, reports indicate that interaction of Ubc9 and p53 may be regulated by p53 phosphorylation.Citation30 In addition, MDM2 can promote sumoylation of p53 in cooperation with p14ARF, a protein that binds MDM2 and inhibits ubiquitination.Citation31 However, the effect of sumoylation on p53 function has been controversial, with several studies reporting that sumoylation promotes p53 function as a transcription activator, while others have reported that it represses transcription.Citation32–Citation34 These diametric results may be reconciled if sumoylation can have either an inhibitory or activator function dependent upon either the promoter context or cell type studied. In addition, recent studies indicate that post-translational modification of SUMO-1 may regulate whether sumoylation will inhibit or stimulate p53, with SUMO-1 acetylation activating p53.Citation35,Citation36 Functionally, it has been proposed that sumoylation of p53 may regulate protein-protein interactions, half-life and/or subcellular localization.Citation29,Citation37
Now, we report that RAX/PACT, the only identified cellular activator of PKR, can form a complex with p53 and the sumo-conjugating enzyme Ubc9 to induce p53 sumoylation at lysine 386. This promotes sumoylation-dependent transcriptional activation of p53, leading to G1 cell cycle arrest. Importantly, RAX/PACT-dependent p53 activation and G1 arrest require PKR, and RAX/PACT promotes p53 phosphorylation on serine 392. Moreover, endogenous p53 stability is reduced in cells with decreased RAX/PACT or PKR expression following siRNA knockdown. Taken together these results suggest that the RAX/PACT-PKR stress-signaling pathway may dynamically regulate p53 stability and activation following stress by a mechanism dependent on p53 sumoylation and phosphorylation. These novel findings demonstrate the importance of RAX/PACT-PKR stress signaling beyond eIF2α-depedent translation regulation.
Results
RAX/PACT interacts with Ubc9, the SUMO E2 ligase.
In an effort to gain insight into RAX/PACT-PKR stress response signaling, we performed a two-hybrid screen to identify putative RAX/PACT-binding proteins by expressing RAX/PACT as a GAL4 binding domain fusion (BD-RAX) in yeast strain AH109. Importantly, expression of BD-RAX alone is not toxic, does not affect the mating efficiency of the strain and does not independently activate transcription of the reporter genes. The AH109 strain expressing BD-RAX was mated with yeast Y187 containing either a day 11 mouse embryo cDNA or a mouse testis cDNA-GAL4 activation domain fusion library. Colonies positive for activation of three reporter genes were isolated, and DNA from the resulting positive clones was sequenced. These screens identified seven putative RAX/PACT-interacting proteins: RAX/PACT (self-association), TRBP, JAZ, Ubc9, Tenr, AF4 and Pcbp1 ( and data not shown). Since Ubc9, the sole SUMO E2 conjugating enzyme and a key regulator of the sumoylation pathway, has been reported to regulate many of the same transcription factors as PKR, we chose to further investigate this interaction.
To verify the interaction between RAX/PACT and Ubc9, immunoprecipitation followed by western blotting was performed in H1299 cells. FLAG-tagged RAX/PACT was co-transfected with Ubc9 and immunoprecipitated using anti-FLAG agarose. Consistent with the yeast two-hybrid result, association between RAX/PACT and Ubc9 is detected by co-immunoprecipitation (). Furthermore, immunofluorescence (IF) staining of H1299 cells reveals that endogenous RAX/PACT is cytoplasmic, while Ubc9 is diffusely localized in both the nucleus and cytoplasm. Thus, co-localization of Ubc9 and RAX/PACT occurs predominantly in the cytoplasm (). Similarly, PKR and Ubc9 are observed to co-localize in the cytoplasm by IF staining, suggesting that any interaction does not involve translocation of the complex to the nucleus (data not shown).
RAX/PACT promotes sumoylation of p53.
Since p53 is a target of RAX/PACT-PKR signaling and p53 may be sumoylated, we hypothesize that RAX/PACT may facilitate interaction between p53 and Ubc9.Citation33,Citation38–Citation40 To test this, Ubc9, p53 and Flag-tagged-RAX plasmids were transiently co-transfected into H1299 cells, and complex formation was detected by immunoprecipitation with Ubc9 antibody, followed by western blotting with p53 antibody. Significantly, co-expression of RAX/PACT promotes increased p53 association with Ubc9 ().
To test whether RAX/PACT promotes p53 sumoylation, an in vivo sumoylation assay was performed.Citation38 As a positive control, MDM2 and ARF were utilized, since overexpression of MDM2 and/or ARF has been reported to stimulate p53 sumoylation.Citation31 Histidine-tagged SUMO-1 was co-expressed with p53, RAX/PACT, MDM2 and/or ARF in H1299 cells and any resulting SUMO-1 conjugated proteins isolated by Ni-NTA affinity chromatography under denaturing conditions. Following purification, sumoylated p53 was detected by western blotting using a p53-specific antibody. Results demonstrate that while expression of either MDM2 or ARF cannot stimulate p53 sumoylation alone (, lanes 4 and 5), RAX/PACT promotes p53 sumoylation in a dose-dependent manner (, lanes 6–8). Furthermore, co-expression of RAX/PACT with MDM2 and ARF significantly enhances p53 sumoylation, indicating a synergy between RAX/PACT, MDM2 and ARF to promote p53 sumoylation (, lanes 9–15). In addition, while MDM2 expression reduced p53 levels, co-expression with RAX/PACT stabilized p53 protein expression (, lanes 4 and 9 compared with lanes 10 and 11). Significantly, we did not observe RAX/PACT or PKR sumoylation in vitro, and RAX/PACT expression does not affect either p53-MDM2 interaction or p53 ubiquitination (data not shown).
The site of p53 sumoylation is reported to be lysine 386. To test whether RAX/PACT promotes p53 sumoylation at this site, wild-type p53 and the p53(K386R) mutant were compared (). Importantly, sumoylation does not occur on the p53(K386R) mutant, indicating that RAX/PACT-induced sumoylation requires an intact lysine 386 (, lanes 6 and 7 vs. lanes 8 and 9).
We also tested whether RAX/PACT-dependent p53 sumoylation can be reversed by the potent desumoylating protease, SENP1 (SUMO1/sentrin specific peptidase 1). Results reveal that when SENP1 is expressed in the presence of SUMO1, p53 and RAX/PACT, RAX/PACT can stimulate and SENP1 inhibits/reverses p53 sumoylation (). Taken together, these results indicate that RAX/PACT promotes reversible sumoylation of p53 at lysine 386.
To confirm these results, we tested whether RAX/PACT could enhance sumoylation of a p53-Ubc9 fusion plasmid where p53 has recently been demonstrated to be efficiently sumoylated.Citation41 Co-expression of HA-tagged SUMO1 promotes sumoylation of the p53-Ubc9 fusion protein (, lanes 1 and 2). Furthermore, p53 sumoylation occurs at lysine 386 and is dependent on Ubc9 activity, since neither the p53(K386R)-Ubc9 or the p53-Ubc9 (C93S) mutant can be efficiently sumoylated (, lanes 3–6). Significantly, co-expression of RAX/PACT stimulates p53-Ubc9 sumoylation, even in the absence of co-transfection with HA-SUMO1 (, lane 7). Moreover, co-expression of exogenous HA-SUMO1 and RAX further enhances p53-Ubc9 sumoylation (, lane 8).
RAX/PACT promotes p53 activation and G1 cell cycle arrest.
First, to test the effect of RAX/PACT expression on p53 activity, a p21-promoter firefly luciferase reporter assay was used. A Renilla luciferase expression plasmid was used to normalize expression data. Significantly, while RAX/PACT expression alone in p53-null H1299 cells fails to promote any luciferase activity, the co-expression of RAX/PACT and p53 increases p53-dependent luciferase activity about 5-fold compared with p53 expression alone. Furthermore, the reporter expression is RAX/PACT dosage-dependent (, upper part). In addition, U2OS cells that express endogenous p53 were also tested. Expression of RAX/PACT results in a dose-dependent increase in p53-dependent luciferase activity (by about 6-fold), indicating that RAX/PACT induces endogenous p53 transcriptional activity (, upper part). As a control for the specificity of the p53 reporter, luciferase activity was measured after expression of either p53 or MDM2. As expected, the expression of p53 increases and expression of MDM2 decreases p53 transcriptional activity (, upper part). Similar results were also observed using Saos2 cells or HeLa cells (data not shown). Taken together, these results indicate that RAX/PACT expression enhances p53 transcriptional activity.
Next, we tested whether RAX/PACT and p53 could synergize to regulate cell cycle progression by transiently transfecting p53-null H1299 cells with RAX/PACT and/or p53. Cells were simultaneously transfected with a Us9-GFP fusion protein in order to gate for positively transfected cells.Citation42 While the expression of RAX/PACT or p53 alone has no demonstrable effect, co-expression of p53 and RAX/PACT causes a ∼25–35% increase in the G1 population with a corresponding decrease in S and G2 phases (). Significantly, FACS analysis reveals that RAX/PACT can only promote G1 cell cycle arrest when co-expressed with wt p53 but not mutant p53(K386R) (85% vs. 65%; ). Furthermore, the expression of SENP1 abrogates this effect (63% vs. 85%; ). These data suggest that RAX/PACT-induced p53 sumoylation facilitates p53-dependent G1 cell cycle arrest.
Finally, to test whether RAX/PACT can effect expression of p53 target genes, quantitative real-time PCR was performed using RNA isolated from U2OS cells that express either a control siRNA or a RAX/PACT-specific siRNA. U2OS cells expressing siRNA to RAX/PACT display a > 90% reduction in RAX/PACT protein level by western blotting and a > 50% decrease in gene expression ( and E). Significantly, quantitative real-time PCR results indicate that cells with reduced RAX/PACT expression display a significant reduction in p21 (∼5-fold) and PUMA (∼50%) gene expression (). In addition, decreased RAX/PACT expression has little or minor effect on p53, BAX or MDM2 (). Taken together, these data indicate that RAX/PACT does not affect p53 gene expression but may be required for expression of p53 downstream targets such as p21 to regulate the cell cycle.
PKR is required for RAX/PACT-dependant p53 activation.
Since RAX/PACT is a well-characterized cellular activator of PKR during stress, we tested whether PKR may be required for RAX/PACT to stimulate p53 activity. First, we tested whether RAX/PACT, PKR and p53 synergize to regulate cell cycle progression by transiently transfecting p53-null H1299 cells with RAX/PACT, PKR and/or p53. Importantly, while expression of p53 or PKR alone has little effect, the co-expression of p53 and PKR promotes G1 cell cycle arrest (). In addition, co-expression of RAX/PACT, PKR and p53 promoted G1 cell cycle arrest to about the same degree as co-expression of PKR and p53 (). Significantly, the dominant-negative PKR(K296R) mutant (DN-PKR) was unable to cooperate with p53 to promote G1 arrest. Furthermore, co-expression of DN-PKR with RAX/PACT and p53 blocked the ability of RAX/PACT and p53 to promote G1 cell cycle arrest (). These results indicate that RAX/PACT requires PKR to promote p53-depedent cell cycle arrest.
Second, to further test whether PKR may be required for RAX/PACT-dependent p53 activation, Flag-tagged RAX/PACT was transfected into both pkr−/− or pkr+/+ MEF cells and stable clones selected. As expected, when RAX/PACT is expressed in pkr+/+ cells, the expression of p53 and p21 is increased compared with vector-only control cells (, lane 3 vs. lane 4). However, when RAX/PACT is expressed in pkr−/− cells, neither p53 nor p21 is upregulated (, lane 1 vs. lane 2). Furthermore, following gamma irradiation, pkr+/+ cells display an increase in p53 and p21 (, left part). In contrast, no increase in p53 or p21 expression is observed in pkr−/− cells following gamma irradiation, including in pkr−/− cells stably expressing exogenous RAX/PACT (, right part). Together, these data indicate that RAX/PACT activation of p53 following genotoxic stress is indirect and requires PKR.
RAX/PACT-PKR inhibits p53 turnover and promotes phosphorylation of p53 on serine 392 by a sumoylation-dependent mechanism.
Since RAX-PKR expression and p53sumoylation may regulate p53 stability, we next measured p53 turnover in U2OS cells that express a PKR siRNA following stress. Stable expression of PKR siRNA results in > 80% reduction of PKR expression in U2OS cells compared with control siRNA-expressing cells (). Since the level of endogenous p53 is low in U2OS cells, we also treated cells with doxorubicin for 4 h to induce p53 expression. Following doxorubicin treatment, U2OS cells expressing either control or PKR siRNA were then treated with cyclohexamide for various times to block new protein synthesis. Importantly, western blot analysis indicates that the p53 half-life is significantly shorter in the PKR-knockdown cells following doxorubicin treatment (5 min. vs. 15 min.), indicating that PKR is required to sustain p53 stability following stress (). Similar results were observed in U2OS cells with decreased endogenous RAX/PACT by siRNA knockdown (data not shown). Taken together, these results indicate that the RAX/PACT-PKR signaling can promote p53 stability following stress.
Since PKR has been reported to phosphorylate p53, we tested whether RAX/PACT expression may promote p53 phosphorylation. We analyzed H1299 lysates from cells co-transfected with RAX/PACT and p53 using a part of phospho-specific p53 antibodies. Significantly, we determined that RAX/PACT promotes phosphorylation of wild-type p53 to a greater degree than p53(K386R) on serine 392 (). Interestingly, no significant difference in p53 phosphorylation at other sites of phosphorylation was observed in these cells (data not shown). Furthermore, RAX/PACT expression promotes significantly increased p53 protein expression. Thus, RAX/PACT-PKR signaling may promote a sumoylation-dependent increase in p53 protein level that leads to increased p53 phosphorylation on serine 392.
Discussion
Findings reported here demonstrate that RAX/PACT is a multifunctional protein, which not only activates PKR to inhibit new protein synthesis, but also regulates post-translational modification and transcriptional activity of p53. Data indicate that RAX/PACT's role in regulating p53 is indirect, since RAX/PACT interacts directly with the SUMO E2 ligase Ubc9 to stimulate Ubc9-p53 association and reversible sumoylation of p53 on lysine 386. RAX/PACT appears to promote successive sumoylation-dependent p53 phosphorylation on serine 392, which increases the stability and transcriptional activity of p53 and leads to G1 cell cycle arrest. The nature of this mechanism for facilitating p53-mediated growth suppression is dynamic since expression of the desumoylating enzyme SENP1 reverses both RAX/PACT-dependent p53 sumoylation as well as the inhibitory effect on the cell cycle. Significantly, co-expression of a dominant-negative PKR mutant blocks RAX/PACT's ability to synergize with p53 to promote G1 cell cycle arrest, while cells expressing reduced PKR have decreased p53 half-life. Thus, we now propose that the RAX/PACT-PKR stress-signaling pathway may promote p53 protein stability, translational activation and G1 cell cycle arrest by a mechanism dependent on sequential sumoylation then phosphorylation of p53.
The effect of p53 sumoylation has been controversial. Several studies indicate that sumoylation of p53 may promote its transcription factor activity, while others have reported that sumoylation represses transcription.Citation28,Citation32–Citation34 Results may potentially be reconciled by the recent finding that phosphorylation or acetylation of SUMO-1 may occur to regulate whether sumoylation can be inhibitory or stimulatory.Citation36 Thus, sumoylation of p53 may have either an inhibitory or activator function, depending upon whether SUMO-1 has been acetylated and the promoter context or cell type studied. Significantly, our data supports the notion that sumoylation promotes p53 stability, activation and G1 cell cycle arrest.
Our findings indicate that RAX/PACT enhances p53 activity in a PKR-dependent mechanism. Significantly, others have reported that p53 activation leading to G1 arrest is impaired in pkr−/− cells, and that increased PKR expression/activity will promote p53 stability.Citation23,Citation43 It has also been reported that PKR can phosphorylate p53 on serine 392, and that phosphorylation may inhibit the turnover of p53 leading to enhanced stability.Citation21,Citation30 Findings here both support and extend this mechanism, since RAX/PACT promotes increased p53 protein expression and serine 392 phosphorylation of wild-type but not mutant p53(K386R). Furthermore, RAX/PACT mediated p53 activation and cell cycle arrest requires both PKR activity and sumoylation of p53. Thus, we propose that RAX/PACT-PKR may promote p53 sumoylation followed by p53 phosphorylation at serine 392, leading to increased p53 stability and G1 cell cycle arrest. To test this hypothesis, further experimentation is required to determine whether p53 phosphorylation is dependent on p53 sumoylation.
One intriguing question is where, intracellularly, does RAX/PACT-PKR-dependent sumoylation of p53 occur: the nucleus or cytosol or both? Findings indicate that RAX/PACT and PKR exclusively co-localize in the cytoplasm where some fraction of p53 and Ubc9 also reside ( and data not shown). Thus, we favor a cytoplasmic site for sumoylation of p53. However, while we could not detect nuclear RAX/PACT or PKR, others have reported that PKR may translocate into the nucleus during stress where a majority of Ubc9 and p53 are located.Citation44–Citation46 Thus, it is possible that p53 sumoylation may occur in either the nucleus or cytoplasm or both locations, and the process may even be cell type-dependent. Further experiments will need to be performed to determine whether RAX/PACT-PKR transiently associates with p53 and Ubc9 only in the cytosol, or whether RAX/PACT-PKR-dependent sumoylation and activation of p53 occurs following PKR translocation to the nucleus.
In summary, results indicate the RAX/PACT-PKR stress response pathway may regulate p53 protein sumoylation. Importantly, the findings reported here might have wide-ranging implications beyond p53 sumoylation, since RAX/PACT-PKR may promote sumoylation of other downstream target proteins. This provides a novel mechanism by which RAX/PACT-PKR signaling may effect the subcellular localization, stability and protein-protein interactions of other downstream targets during cellular stress in addition to its role in eIF2α phosphorylation-dependent translation inhibition. Together, these findings demonstrate the intricate protein networks by which the RAX/PACT-PKR signaling axis may regulate cell growth and adaptation to stress. Since many cancers, such as AML, retain wild-type p53, future anticancer therapies that incorporate RAX-PKR activation may provide a novel mechanism to promote p53-dependent tumor suppression.
Materials and Methods
Cell lines and reagents.
H1299 (human non-small cell lung cancer, p53-null), Saos2 (human osteosarcoma, p53-null), U2OS (human osteosarcoma, wt p53) were obtained from American Type Culture Collection. The pkr−/− and pkr+/+ littermate MEFs were from Bryan R.G. Williams (Monash Institute for Medical Research). Cells were maintained in DMEM supplemented with 10% FBS at 37°C and 5% CO2 (Gibco, Invitrogen). To generate pkr−/− cells stably expressing exogenous Flag-RAX/PACT, a retroviral expression system was used as described in reference Citation12. U2OS with reduced endogenous RAX/PACT or PKR were generated using lentivirus particles containing shRNA (Santa Cruz Biotechnology) followed by selection in 2 µg/ml puromycin. Immunoblotting using antibodies to PKR, RAX/PACT (Abnova) or Flag (#F1804, Sigma-Aldrich) was used to measure expression levels in the cell lines generated.
Western blot and immunoprecipitation.
H1299 cells (1 × 106) were transfected with a total of 8 µg of expression plasmids using Lipofectamine 2000 (Invitrogen) in 60-mm plates and harvested at 24-h post-transfection. Cells were lysed in 200 µl of lysis buffer (10 mM TRIS-HCl, pH 7.5, 1 mM EDTA, 1% Triton X-100, 150 mM NaCl and protease inhibitors) by incubating on ice for 30 min, and the extracts were centrifuged at 13,000 rpm for 15 min to remove cell debris. Protein concentrations were determined by BCA assay, and samples were resolved by SDS-PAGE. For immunoprecipitation, cell lysate was prepared in 0.5% Triton X-100 lysis buffer and incubated with antibody for 4 h, followed by incubation with protein A beads (Invitrogen) for an additional 4 h. Immune complexes and whole lysates were resolved using SDS-PAGE, transferred to nitrocellulose membranes, and western blotting was performed using the indicated antibody. The following antibodies were used: DO-1 for p53 (Santa Cruz Biotechnology), RAX/PACT monoclonal antibody (Abnova) or a rabbit polyclonal serum for RAX/PACT, rabbit polyclonal antibody for Ubc9 (Santa Cruz Biotechnology), rabbit polyclonal antibody for MDM2 (Calbiochem) and rabbit polyclonal antibody for p21 (Santa Cruz Biotechnology).
Real-time qPCR.
Reverse transcription was performed using 2 µg of total RNA from U2OS cells stably expressing either control siRNA or RAX siRNA and High-Capacity cDNA Reverse Transcription Kits (Applied Biosystems). The primers and Probe for real-time PCR were designed using the Primer Express software and synthesized at Applied Biosystems. Each real-time PCR reaction was performed in a mix of 25 µl reaction mixture containing 50 ng of cDNA, 2x TaqMan Universal PCR Master Mix (Applied Biosystems) and 20x of primers and Probe. The reaction mixture was incubated for 10 min at 95°C and repeat 40 cycles (denaturing for 15 sec at 95°C and annealing and extending for 1 min at 60°C) using Applied Biosystems 7900HT Real-Time PCR System. All samples were tested in triplicate, and average values were used for quantification. Analysis was performed using SDS v2.3 software (Applied Biosystems) according to the manufacturer's instruction. Beta-actin was used as an endogenous reference. The comparative CT method (CT) was used for quantification of gene expression.
Immunofluorescence staining.
Cells cultured on chamber slides were fixed with acetone-methanol (1:1) for 3 min at room temperature, blocked with PBS plus 10% normal goat serum (PBS + 10% NGS) for 20 min and incubated with primary antibodies in PBS + 10% NGS for 2 h. The slides were washed with PBS + 0.1% Triton X-100, incubated with Alexa Fluor-goat anti-mouse IgG and/or Alexa Fluor-goat anti-rabbit IgG (Molecular Probes) in PBS + 10% NGS for 2 h, washed with PBS + 0.1% Triton X-100, and mounted. Each of the primary antibodies for p53 (DO-1), RAXPACT, Ubc9 and PKR was diluted 1:100 for double staining. Leica TCS SP2 AOBS Spectral Confocal Microscope and LCS (Leica Confocal Software) Version 2.61, Build 1537 was used for all IF staining data collection.
Luciferase reporter assay.
Cells (1 × 105/well) were plated in 12-well plates and transfected with 100 ng p53-responsive BP100-luciferase reporter plasmid and 10 ng Renilla luciferase reporter plasmid (Promega). In addition, p53 and/or Flag-RAX/PACT expression plasmid were transfected as indicated using Lipofectamine 2000 reagents (Invitrogen). Cells were analyzed for luciferase and Renilla luciferase expression after 24 h using the dual luciferase system (Promega). The ratio of p53 reporter/Renilla reporter activity was used as an indicator of p53 transcription activity. p53-TA (containing the wt p53 response elements) luciferase reporter vectors were obtained from BD Clontech.
Cell cycle analysis.
FACS analysis was performed on H1299 cells at University of Florida Flow Cytometry Core Facility using a FACSCAN flow cytometer (BD Biosystems). An integral membrane GFP marker, Us9-GFP, was used to test for the positively transfected cells in FACS analysis.Citation43 Cells were transfected with lipofectamine 2000 with Us9-GFP and other plasmid as indicated. Twenty-four hours post transfection cells were treated with trypsin, fixed and stained by propidium iodide. Cells expressing Us9-GFP and PI stained were gated and analyzed for cell cycle status using Cellquest version 3.3 software.
Yeast two-hybrid screen.
A two-hybrid screen for RAX/PACT-interacting proteins was performed using the Matchmaker two-hybrid system 3 (BD Biosciences, Clontech). Briefly, murine RAX/PACT was cloned as an Nde I-BamHI fragment into pGBKT7 to create the RAX-GAL4 DNA binding domain expression plasmid, pGBKT7-RAX. This plasmid was transformed into yeast strain AH109 (BD Biosciences) by LiCl method. Yeast were selected on SD/-Trp plates for stable integration of the RAX expression plasmid, and RAX expression was confirmed by western blotting. Yeast strain AH109pGBKT7-RAX was mated with Y187 pretransformed with either a day 11 mouse embryo cDNA or a mouse testis cDNA-GAL4 activation domain fusion library (BD Matchmaker pretransformed libraries, BD Biosciences). The mating mixture was plated on SD/-Leu/-Trp/-His, allowed to grow approximately 1 week and then replica plated to SD/-Leu/-Trp/-His/-Ade (QDO) plates containing X-α-Gal. Resultant colonies after ∼2 weeks of growth were picked and restreaked onto QDO plates twice to select single colonies. Plasmids were isolated from these colonies, interactions reconfirmed by retransformation and selection on QDO plates and cDNAs sequenced at the University of Florida DNA sequencing core facility.
In vivo sumoylation assays.
Briefly, 2 × 105 H1299 cells cultured in 6 cm plates were transfected with 1 µg each of Hexahistidine-tagged SUMO-1 and 1 µg of p53 expression plasmids using lipofectamine 2000 (Invitrogen). Twenty-four hours after transfection, cells were collected into two aliquots. Western blot from one aliquot was used to examine the expression of transfected proteins. The second aliquot was used for affinity purification of His-tagged proteins using Ni2+-NTA beads. The cell pellet was lysed in buffer A (6 M guanidinium-HCl, 0.1 M Na2HPO4/NaH2PO4, 0.01 M Tris-Cl, pH 8.0, 5 mM imidazole and 10 mM β-mercaptoethanol) and incubated with Ni2+-NTA beads (Qiagen) for 4 h at room temperature. The beads were washed with buffers A, B (8 M urea, 0.1 M Na2PO4/NaH2PO4, 0.01 M Tris-Cl, pH 8.0 and 10 mM β-mercaptoethanol), C (8 M urea, 0.1 M Na2PO4/NaH2PO4, 0.01 M Tris-Cl, pH 6.3 and 10 mM β-mercaptoethanol), and bound proteins were eluted with buffer D (200 mM imidazole, 0.15 M Tris-Cl, pH 6.7, 30% glycerol, 0.72 M β-mercaptoethanol and 5% SDS). The eluted proteins were analyzed by western blot for p53 by DO-1 antibody (Santa Cruz Biotechnology).
Disclosure of Potential Conflicts of Interest
No potential conflicts of interest were disclosed.
Figures and Tables
Figure 1 RAX/PACT interacts with the SUMO-conjugating enzyme Ubc9. (A) A two-hybrid screen identifies interaction between RAX/PACT and the SUMO conjugating enzyme, Ubc9. Top part: limiting dilution plate on SD-leu/-trp medium indicates presence of yeast two-hybrid binding domain and activation domain vectors. Lower part: RAX/PACT self-association and RAX/PACT interaction with Ubc9 are indicated by growth on SD-leu/-trp/-ade/-his plates. (B) Ubc9 and FLAG-tagged RAX/PACT were transiently transfected as indicated into H1299 cells. Following immunoprecipitation of Flagtagged RAX/PACT, western blotting using antibodies specific for Ubc9 demonstrated interaction between RAX/PACT and Ubc9. (C) Co-localization of Ubc9 and RAX/PACT in H1299 cells by immunofluorescence cell staining. Nuclei are indicated by DAPI staining.
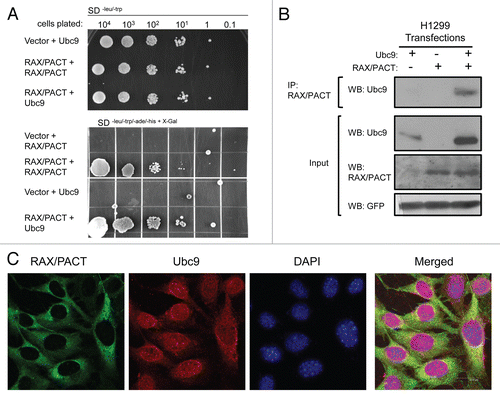
Figure 2 RAX/PACT enhances the association of p53 with Ubc9 to promote p53 sumoylation at lysine 386. (A) RAX/PACT expression promotes the association of p53 and Ubc9. Ubc9, p53 and Flag-tagged RAX/PACT were transiently transfected into H1299 cells. Immunoprecipitation was performed with anti-Ubc9 antibody, and co-immunoprecipitation was detected by western blotting. (B) RAX/PACT enhances p53 sumoylation in vivo. His-tagged SUMO-1 was co-transfected with p53 and the indicated expression plasmids into H1299 cells. Affinity chromatography was used to isolate His-SUMO-1 conjugated proteins, and the sumoylated p53 population was detected by western blotting with antibody to p53. Co-expression of MDM2 and ARF synergize with RAX/PACT to further enhance p53 sumoylation. (C) RAX/PACT mediates p53 sumoylation at lysine 386 since RAX/PACT does not promote sumoylation of the p53(K386R) mutant. (D) RAX/PACT-induced p53 sumoylation is reversible. Co-expression of the SUMO-specific protease SENP1 inhibits RAX/PACT-induced sumoylation of p53. (E) The expression plasmids for p53-Ubc9, p53 (K386R)-Ubc9, p53-Ubc9 (C93S) as well as HA-SUMO-1 and Flag-RAX were co-transfected with 0.1 µg of GFP in H1299 cells. SUMO-conjugated or non-conjugated p53 were detected by western blot. GFP was used as a transfection efficiency control.
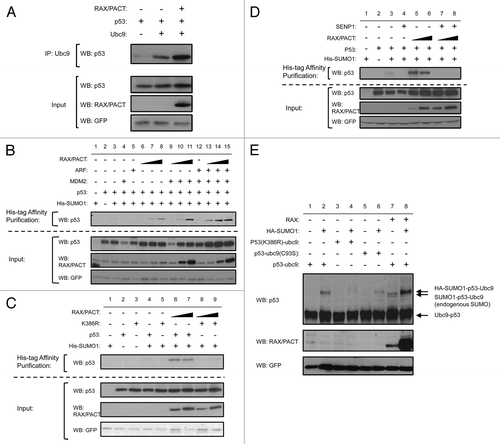
Figure 3 RAX/PACT increases the activity of p53. RAX/PACT promotes p53-dependent transcription in a dose-dependent manner. (A) H1299 cells (p53-null) and (B) U2OS cells expressing endogenous p53 were transiently transfected with a p53-inducible firefly luciferase plasmid, Renilla luciferase control plasmid and 0.1, 0.3 or 0.6 µg of the indicated expression constructs. Twenty-four hours after transfection, cells were lysed, and the firefly/Renilla luciferase ratio was measured by dual luciferase assay. The average of at least three similar dual luciferase assays and a representative western blot are shown. (C) Cell cycle analysis of H1299 cells 24 h after transfection with plasmids expressing either GFP alone, RAX/PACT and/or p53 as indicated. (D) U2OS cells stably expressing siRNA to RAX/PACT display a > 90% reduction in RAX/PACT protein by western blot. (E) Quantitative real-time PCR (qPCR) to measure gene expression of RAX/PACT, p53, MDM2 and the p53 targets p21, PUMA and BAX in U2OS cells stably expressing either siRNA to RAX/PACT or control siRNA.
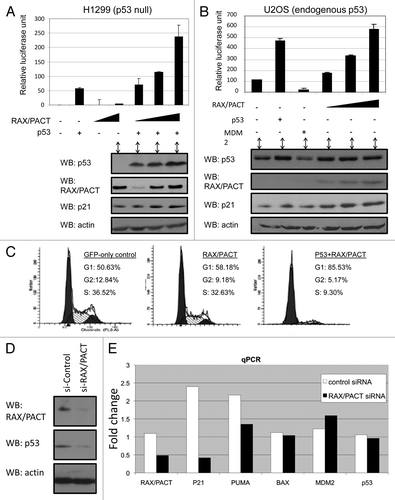
Figure 4 PKR promotes p53 activity. (A) Co-expression of PKR and p53 promotes G1 cell cycle arrest. Cell cycle analysis of H1299 cells 24 h after transfection with plasmids expressing either GFP alone, PKR and/or p53 as indicated. (B) Exogenous RAX/PACT expression increases the level of p53 and p21 in normal pkr+/+ MEF cells but not pkr−/− MEF cells. RAX/PACT expression and endogenous levels of PKR, p53, p21 and actin were determined by western blot using lysate of MEF cells from pkr−/− mice or paired pkr+/+ littermates stably expressing either vector control or Flag-RAX/PACT. (C) Left part: p53 and p21 levels are increased following 10 Gy of gamma irradiation. Right part: following gamma irradiation, pkr−/− MEF cells or pkr−/− MEF stably expressing Flag-RAX/PACT fail to upregulate p53 and p21 expression.
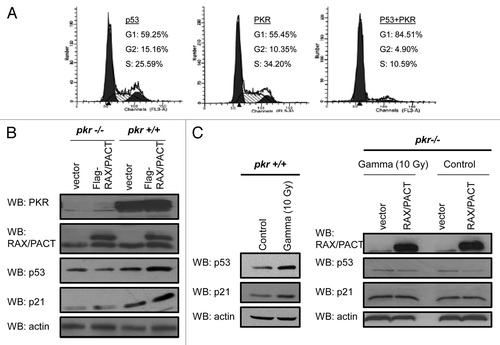
Figure 5 RAX/PACT-PKR promotes p53 stability following stress and p53 phosphorylation on serine 392. (A) PKR expression is reduced by > 80% in U2OS cells by siRNA knockdown. (B) The half-life of p53 is decreased in U2OS cells with reduced PKR following treatment with 5 µM doxorubicin. (C) RAX/PACT expression stimulates p53 phosphorylation at serine 392 when co-expressed with wild-type p53 but not mutant p53(K386R).
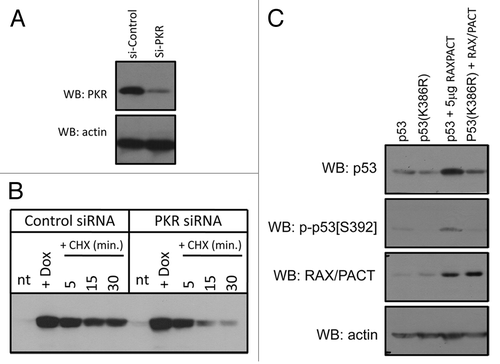
Table 1 RAX/PACT-dependent G1 cell cycle arrest is dependent on p53 sumoylation
Table 2 PKR synergizes with p53 to promote G1 cell cycle arrest
Acknowledgements
We would like to thank Jiandong Chen for the pCMV-p53, ARF and SENP1 expression plasmids, Daiqing Liao for the HA-SUMO1 expression plasmid, Lynn Enquist for the Us9-GFP expression plasmid and Rainer Niedenthal for the Ubc9-p53 fusion plasmids. Teng Hui was an HHMI-UF Science for Life award recipient. This work was supported by NIH/NHLBI grant 5R01HL054083.
References
- Ito T, Yang M, May WS. RAX, a cellular activator for double-stranded RNA-dependent protein kinase during stress signaling. J Biol Chem 1999; 274:15427 - 15432; PMID: 10336432; http://dx.doi.org/10.1074/jbc.274.22.15427
- Ito T, Jagus R, May WS. Interleukin 3 stimulates protein synthesis by regulating double-stranded RNA-dependent protein kinase. Proc Natl Acad Sci USA 1994; 91:7455 - 7459; PMID: 7519779; http://dx.doi.org/10.1073/pnas.91.16.7455
- Bennett RL, Blalock WL, May WS. Serine 18 phosphorylation of RAX, the PKR activator, is required for PKR activation and consequent translation inhibition. J Biol Chem 2004; 279:42687 - 42693; PMID: 15299031; http://dx.doi.org/10.1074/jbc.M403321200
- Chang KS, Cai Z, Zhang C, Sen GC, Williams BR, Luo G. Replication of hepatitis C virus (HCV) RNA in mouse embryonic fibroblasts: protein kinase R (PKR)-dependent and PKR-independent mechanisms for controlling HCV RNA replication and mediating interferon activities. J Virol 2006; 80:7364 - 7374; PMID: 16840317; http://dx.doi.org/10.1128/JVI.00586-06
- Patel CV, Handy I, Goldsmith T, Patel RC. PACT, a stress-modulated cellular activator of interferon-induced double-stranded RNA-activated protein kinase, PKR. J Biol Chem 2000; 275:37993 - 37998; PMID: 10988289; http://dx.doi.org/10.1074/jbc.M004762200
- Patel RC, Sen GC. PACT, a protein activator of the interferon-induced protein kinase, PKR. EMBO J 1998; 17:4379 - 4390; PMID: 9687506; http://dx.doi.org/10.1093/emboj/17.15.4379
- Peters GA, Hartmann R, Qin J, Sen GC. Modular structure of PACT: distinct domains for binding and activating PKR. Mol Cell Biol 2001; 21:1908 - 1920; PMID: 11238927; http://dx.doi.org/10.1128/MCB.21.6.1908-1920.2001
- Huang X, Hutchins B, Patel RC. The C-terminal, third conserved motif of the protein activator PACT plays an essential role in the activation of double-stranded-RNA-dependent protein kinase (PKR). Biochem J 2002; 366:175 - 186; PMID: 11985496
- Meurs E, Chong K, Galabru J, Thomas NS, Kerr IM, Williams BRG, et al. Molecular cloning and characterization of the human double-stranded RNA-activated protein kinase induced by interferon. Cell 1990; 62:379 - 390; PMID: 1695551; http://dx.doi.org/10.1016/0092-8674(90)90374-N
- Williams BR. PKR; a sentinel kinase for cellular stress. Oncogene 1999; 18:6112 - 6120; PMID: 10557102; http://dx.doi.org/10.1038/sj.onc.1203127
- Balachandran S, Roberts PC, Brown LE, Truong H, Pattnaik AK, Archer DR, et al. Essential role for the dsRNA-dependent protein kinase PKR in innate immunity to viral infection. Immunity 2000; 13:129 - 141; PMID: 10933401; http://dx.doi.org/10.1016/S1074-7613(00)00014-5
- Chen LL, Yang L, Carmichael GG. Molecular basis for an attenuated cytoplasmic dsRNA response in human embryonic stem cells. Cell Cycle 2010; 9:3552 - 3564; PMID: 20814227; http://dx.doi.org/10.4161/cc.9.17.12792
- Bennett RL, Blalock WL, Abtahi DM, Pan Y, Moyer SA, May WS. RAX, the PKR activator, sensitizes cells to inflammatory cytokines, serum withdrawal, chemotherapy and viral infection. Blood 2006; 108:821 - 829; PMID: 16861340; http://dx.doi.org/10.1182/blood-2005-11-006817
- Chen G, Ma C, Bower KA, Ke Z, Luo J. Interaction between RAX and PKR modulates the effect of ethanol on protein synthesis and survival of neurons. J Biol Chem 2006; 281:15909 - 15915; PMID: 16574643; http://dx.doi.org/10.1074/jbc.M600612200
- Wong AH, Tam NW, Yang YL, Cuddihy AR, Li S, Kirchhoff S, et al. Physical association between STAT1 and the interferon-inducible protein kinase PKR and implications for interferon and double-stranded RNA signaling pathways. EMBO J 1997; 16:1291 - 1304; PMID: 9135145; http://dx.doi.org/10.1093/emboj/16.6.1291
- D'Acquisto F, Ghosh S. PACT and PKR: turning on NFkappaB in the absence of virus. Sci STKE 2001; 2001:1; PMID: 11752660
- Bonnet MC, Weil R, Dam E, Hovanessian AG, Meurs EF. PKR stimulates NFkappaB irrespective of its kinase function by interacting with the IkappaB kinase complex. Mol Cell Biol 2000; 20:4532 - 4542; PMID: 10848580; http://dx.doi.org/10.1128/MCB.20.13.4532-4542.2000
- Gil J, Alcami J, Esteban M. Activation of NFkappaB by the dsRNA-dependent protein kinase, PKR involves the IkappaB kinase complex. Oncogene 2000; 19:1369 - 1378; PMID: 10723127; http://dx.doi.org/10.1038/sj.onc.1203448
- Zamanian-Daryoush M, Mogensen TH, DiDonato JA, Williams BR. NFkappaB activation by double-stranded-RNA-activated protein kinase (PKR) is mediated through NFkappaB-inducing kinase and IkappaB kinase. Mol Cell Biol 2000; 20:1278 - 1290; PMID: 10648614; http://dx.doi.org/10.1128/MCB.20.4.1278-1290.2000
- Frémont M, Vaeyens F, Herst CV, De Meirleir KL, Englebienne P. Double-stranded RNA-dependent protein kinase (PKR) is a stress-responsive kinase that induces NFkappaB-mediated resistance against mercury cytotoxicity. Life Sci 2006; 78:1845 - 1856; PMID: 16324719; http://dx.doi.org/10.1016/j.lfs.2005.08.024
- Cuddihy AR, Wong AH, Tam NW, Li S, Koromilas AE. The double-stranded RNA activated protein kinase PKR physically associates with the tumor suppressor p53 protein and phosphorylates human p53 on serine 392 in vitro. Oncogene 1999; 18:2690 - 2702; PMID: 10348343; http://dx.doi.org/10.1038/sj.onc.1202620
- Cuddihy AR, Li S, Tam NW, Wong AH, Taya Y, Abraham N, et al. Double-stranded-RNA-activated protein kinase PKR enhances transcriptional activation by tumor suppressor p53. Mol Cell Biol 1999; 19:2475 - 2484; PMID: 10082513
- Yeung MC, Lau AS. Tumor suppressor p53 as a component of the tumor necrosis factor-induced, protein kinase PKR-mediated apoptotic pathway in human promonocytic U937 cells. J Biol Chem 1998; 273:25198 - 25202; PMID: 9737981; http://dx.doi.org/10.1074/jbc.273.39.25198
- Van Dyke T. p53 and tumor suppression. N Engl J Med 2007; 356:79 - 81; PMID: 17202460; http://dx.doi.org/10.1056/NEJMcibr066301
- Carter S, Vousden KH. Modifications of p53: competing for the lysines. Curr Opin Genet Dev 2009; 19:18 - 24; PMID: 19179064; http://dx.doi.org/10.1016/j.gde.2008.11.010
- Hock A, Vousden KH. Regulation of the p53 pathway by ubiquitin and related proteins. Int J Biochem Cell Biol 2010; 42:1618 - 1621; PMID: 20601087; http://dx.doi.org/10.1016/j.biocel.2010.06.011
- Ménendez S, Goh AM, Camus S, Ng KW, Kua N, Badal V, et al. MDM4 downregulates p53 transcriptional activity and response to stress during differentiation. Cell Cycle 2011; 10:1100 - 1108; PMID: 21422812; http://dx.doi.org/10.4161/cc.10.7.15090
- Stindt MH, Carter S, Vigneron AM, Ryan KM, Vousden KH. MDM2 promotes SUMO-2/3 modification of p53 to modulate transcriptional activity. Cell Cycle 2011; 10:3176 - 3188; PMID: 21900752; http://dx.doi.org/10.4161/cc.10.18.17436
- Stehmeier P, Muller S. Regulation of p53 family members by the ubiquitin-like SUMO system. DNA Repair (Amst) 2009; 8:491 - 498; PMID: 19213614; http://dx.doi.org/10.1016/j.dnarep.2009.01.002
- Lin JY, Ohshima T, Shimotohno K. Association of Ubc9, an E2 ligase for SUMO conjugation, with p53 is regulated by phosphorylation of p53. FEBS Lett 2004; 573:15 - 18; PMID: 15327968; http://dx.doi.org/10.1016/j.febslet.2004.07.059
- Chen L, Chen J. MDM2-ARF complex regulates p53 sumoylation. Oncogene 2003; 22:5348 - 5357; PMID: 12917636; http://dx.doi.org/10.1038/sj.onc.1206851
- Bischof O, Schwamborn K, Martin N, Werner A, Sustmann C, Grosschedl R, et al. The E3 SUMO ligase PIASy is a regulator of cellular senescence and apoptosis. Mol Cell 2006; 22:783 - 794; PMID: 16793547; http://dx.doi.org/10.1016/j.molcel.2006.05.016
- Gostissa M, Hengstermann A, Fogal V, Sandy P, Schwarz SE, Scheffner M, et al. Activation of p53 by conjugation to the ubiquitin-like protein SUMO-1. EMBO J 1999; 18:6462 - 6471; PMID: 10562558; http://dx.doi.org/10.1093/emboj/18.22.6462
- Wu SY, Chiang CM. Crosstalk between sumoylation and acetylation regulates p53-dependent chromatin transcription and DNA binding. EMBO J 2009; 28:1246 - 1259; PMID: 19339993; http://dx.doi.org/10.1038/emboj.2009.83
- Matic I, Macek B, Hilger M, Walther TC, Mann M. Phosphorylation of SUMO-1 occurs in vivo and is conserved through evolution. J Proteome Res 2008; 7:4050 - 4057; PMID: 18707152; http://dx.doi.org/10.1021/pr800368m
- Cheema A, Knights CD, Rao M, Catania J, Perez R, Simons B, et al. Functional mimicry of the acetylated C-terminal tail of p53 by a SUMO-1 acetylated domain, SAD. J Cell Physiol 2010; 225:371 - 384; PMID: 20458745; http://dx.doi.org/10.1002/jcp.22224
- Carter S, Bischof O, Dejean A, Vousden KH. C-terminal modifications regulate MDM2 dissociation and nuclear export of p53. Nat Cell Biol 2007; 9:428 - 435; PMID: 17369817; http://dx.doi.org/10.1038/ncb1562
- Rodriguez MS, Desterro JM, Lain S, Midgley CA, Lane DP, Hay RT. SUMO-1 modification activates the transcriptional response of p53. EMBO J 1999; 18:6455 - 6461; PMID: 10562557; http://dx.doi.org/10.1093/emboj/18.22.6455
- Kwek SS, Derry J, Tyner AL, Shen Z, Gudkov AV. Functional analysis and intracellular localization of p53 modified by SUMO-1. Oncogene 2001; 20:2587 - 2599; PMID: 11420669; http://dx.doi.org/10.1038/sj.onc.1204362
- Muller S, Berger M, Lehembre F, Seeler JS, Haupt Y, Dejean A. c-Jun and p53 activity is modulated by SUMO-1 modification. J Biol Chem 2000; 275:13321 - 13329; PMID: 10788439; http://dx.doi.org/10.1074/jbc.275.18.13321
- Jakobs A, Koehnke J, Himstedt F, Funk M, Korn B, Gaestel M, et al. Ubc9 fusion-directed SUMOylation (UFDS): a method to analyze function of protein SUMOylation. Nat Methods 2007; 4:245 - 250; PMID: 17277783; http://dx.doi.org/10.1038/nmeth1006
- Kalejta RF, Brideau AD, Banfield BW, Beavis AJ. An integral membrane green fluorescent protein marker, Us9-GFP, is quantitatively retained in cells during propidium iodide-based cell cycle analysis by flow cytometry. Exp Cell Res 1999; 248:322 - 328; PMID: 10094838; http://dx.doi.org/10.1006/excr.1999.4427
- Cox ML, Meek DW. Phosphorylation of serine 392 in p53 is a common and integral event during p53 induction by diverse stimuli. Cell Signal 2010; 22:564 - 571; PMID: 19932175; http://dx.doi.org/10.1016/j.cell-sig.2009.11.014
- Blalock WL, Bavelloni A, Piazzi M, Tagliavini F, Faenza I, Martelli AM, et al. Multiple forms of PKR present in the nuclei of acute leukemia cells represent an active kinase that is responsive to stress. Leukemia 2011; 25:236 - 245; PMID: 21072047; http://dx.doi.org/10.1038/leu.2010.264
- Bergeron J, Benlimame N, Zeng-Rong N, Xiao D, Scrivens PJ, Koromilas AE, et al. Identification of the interferon-inducible double-stranded RNA-dependent protein kinase as a regulator of cellular response to bulky adducts. Cancer Res 2000; 60:6800 - 6804; PMID: 11156368
- Hakki M, Marshall EE, De Niro KL, Geballe AP. Binding and nuclear relocalization of protein kinase R by human cytomegalovirus TRS1. J Virol 2006; 80:11817 - 11826; PMID: 16987971; http://dx.doi.org/10.1128/JVI.00957-06