Abstract
The p42.3 gene was recently identified and characterized as having tumor-specific and mitosis phase-dependent expression in many types of cancer. This suggested that p42.3 antigen could be used as a target for vaccines against cancers. In this study, we immunized C57BL/6 mice with a DNA vaccine encoding p42.3. We used intramuscular injection with electroporation, either before or after challenge with tumor B16F10 cells. Vaccination with pcDNA3-p42.3 induced some degree of antitumor effect both therapeutically and prophylactically, as evaluated by the inhibition of tumor growth and decrease in tumor weight. Immunized mice showed a high level of specific cytotoxic activity against the p42.3 protein in vivo and had activated CD8 T cells that secreted IFN-γ, perforin, and granzyme B in response to stimulation with the antigen in vitro. Thus, this study presents the DNA vaccination against novel tumor target p42.3 as a promising antitumor modality.
Introduction
Melanoma is a malignant skin cancer that is among the most notoriously aggressive and treatment-resistant of human cancers.Citation1 Early stages of melanoma can be successfully treated by surgical excision while advanced stages are uniquely refractory to current therapies.Citation2 The mouse melanoma cell B16F10, derived from C57BL/6 mice, is one of most malignant cancers in mice and can serve as a good model of human melanoma to evaluate various treatments including vaccines.Citation3-Citation5 We use it here to test if the novel cancer antigen p42.3 (GenBank: DQ150361) can be used as a target for DNA vaccination.
The p42.3 gene was first identified and characterized in gastric cancer (GC) cell line BGC823. It was initially found to be expressed in primary GC tissues and embryonic tissues but not in the normal organ tissues of adults. After depletion of p42.3 expression in BGC823 cells by the RNAi technique, significant suppression of cell proliferation and tumorigenicity were detected, associated with a G2/M phase arrest.Citation6 A recent study suggested that p42.3 is involved in cell cycle regulation and tumorigenesis and is expressed at significantly higher mRNA levels in many other cancer cells including human melanoma.Citation7-Citation10 We have observed that p42.3 is also expressed at higher levels in human melanoma than in nevus (data not shown). Thus differential expression of p42.3 could not only be used to recognize the malignant phenotype of melanoma, but could also make it a vaccine target. Furthermore, a high degree of sequence conservation of p42.3 can be found among tumors from various mammal species by searching the NCBI GenBank, suggesting that the gene has important biological functions.Citation11 It is generally believed that it is a fetal protein, highly expressed at fetal stages and quickly shut down after birth, but undergoing reactivation at tumor stages. Accordingly, this gene may represent a novel candidate target for cancer vaccines.
DNA vaccines provide a promising approach for cancer immunotherapy, having significant advantages compared with traditional vaccines.Citation12,Citation13 DNA plasmids are safe, easy to manufacture, store and deliver and have a flexibility that can facilitate rapid evaluation of a series of target antigens.Citation14-Citation16 Moreover, DNA vaccines can be easily modified to enhance immunogenicity in various ways, such as by including CpG motifs in the plasmid to enhance adjuvanticity.Citation17 However, poor immunogenicity of DNA vaccines in large animals and humans compared with traditional protein-based vaccine has hindered progress. The poor responses may be related to inefficient plasmid uptake by the antigen presenting cells (APC), resulting in low levels of antigen expression.Citation18,Citation19 Electroporation (EP) enhances uptake and has emerged as a promising approach for the delivery of DNA vaccines. EP induces transiently enhanced cell membrane permeability, and creates a low level of inflammation that recruits APC to the injection site.Citation19,Citation20 DNA vaccine efficacy is enhanced 100–1000 fold, resulting in increased cellular and humoral immune responses at significantly lower doses.Citation21
Since the newly identified p42.3 tumor antigen is potentially a novel target, we subcloned the coding region into a DNA vaccine vector, and tested it in the B16F10 melanoma model. C57BL/6 mice were vaccinated by electroporation with a DNA vaccine encoding p42.3, either before or after challenge with B16F10 cells. Vaccination with pcDNA3-p42.3 induced antitumor effects both therapeutically and prophylactically, as revealed by inhibition of tumor growth and decrease in tumor weight. The anti-tumor effects were associated both with an elicited anti-p42.3 specific cytotoxic activity and with higher levels of IFN-γ, perforin, and granzyme-B-producing CD8+ T cells.
Results
Inhibition of tumor growth by pcDNA3-p42.3 vaccination in B16F10 melanoma subcutaneous model
Because the p42.3 tumor antigen is expressed in various human tumor cells as well as the murine myeloma cell line B16F10 but not in normal mouse splenocytes (Fig. S1A and see ref. Citation7), it could serve as a target antigen for DNA vaccine development. To evaluate the antitumor effects of the DNA vaccine, we first subcloned the cDNA of p42.3 into pcDNA3 vector to create a DNA vaccine (designated as pcDNA3-p42.3). This construct was transfected into NIH-3T3 cells and cell lysate was subjected into western blot analysis with anti-p42.3 mAb to check for its expression (Fig. S1B). After the confirmation, C57BL/6 mice were immunized intramuscularly with the pcDNA3-p42.3 with or without electroporation four times at biweekly intervals, and with pcDNA3 or PBS as controls. Humoral immune responses were compared among these groups. We observed that a significantly higher level of anti-p42.3 specific antibody was achieved in the group immunized with pcDNA3-p42.3 with electroporation, suggesting that EP-aided DNA vaccination indeed gave enhanced immune responses (). To test if the responses included anti-tumor effects, B16F10 cells (1 × 105) were used to subcutaneously challenge the mice on day 7 after the final immunization (). The growth of melanoma in mice was monitored by measuring tumor size at the challenged site with an analytic clip. Tumor growth in the mice that had been immunized with pcDNA3-p42.3 was significantly retarded compared with the mice immunized with PBS or pcDNA3 (). The gross weight of tumor in the mice immunized with pcDNA3-p42.3 was also significantly less than that in the pcDNA3 or PBS group (). As shown in , 50 percent of mice immunized with pcDNA3-p42.3 survived more than 50 d after the implantation of B16F10 cells, whereas all mice of the control groups were dead within 40 d. Therefore, pre-immunization with pcDNA3-p42.3 could inhibit the growth of B16F10 melanoma cells upon subsequent challenge
Figure 1. Inhibition of tumor growth induced by pcDNA3-p42.3 vaccination in the B16F10 melanoma subcutaneous model. (A) C57BL/6 mice were immunized intramuscularly (i.m) with pcDNA3-p42.3 with or without electroporation (EP) four times at biweekly intervals, or with pcDNA3 or PBS as controls. Antibody levels in serum collected 7 d after final immunization were detected by ELISA. (B) Immunization schedule. C57BL/6 mice were immunized with 30 μl of pcDNA-p42.3 (1 mg/ml), pcDNA3 (1 mg/ml) or PBS four times every two weeks and were subcutaneously challenged with 1 × 105 B16F10 cells on day 7 after the final immunization. Tumor size (C, D, and E) was measured every 2 d. At day 22 after challenge, solid tumors were isolated from mice and weighed using an analytical balance (F). Another group of mice was monitored for survival (G). In (C), (D), and (F), each value represents the mean ± SEM from seven or eight mice in each group. Every experiment was done at least three times, and one representative experiment is shown. *p ≤ 0.05, **p ≤ 0.01 compared with other groups by the Student t-test. In (G), results are expressed as the percentages of surviving mice in groups of seven or eight mice. *p ≤ 0.05 compared with other groups by the log-rank test.
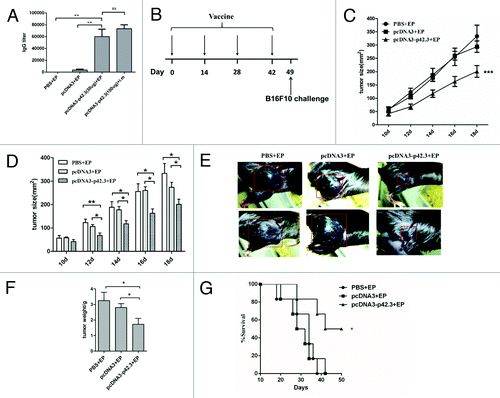
Inhibition of metastasis of B16F10 melanoma induced by pcDNA3-p42.3 vaccination
To investigate whether immunization with pcDNA3-p42.3 is effective in inhibiting the metastasis of B16F10 melanoma, we used a mouse multiple pulmonary melanoma metastases model. After four vaccinations with pcDNA3-p42.3, pcDNA3, or PBS, mice were challenged intravenously (i.v.) with 4 × 105 B16F10 cells and sacrificed 18 d after the challenge. As shown in and , the metastatic nodes of B16F10 in the lung were significantly decreased in mice immunized with pcDNA3-p42.3 compared with those of the control groups. These results suggested that vaccination with pcDNA3-p42.3 could inhibit metastasis of B16F10 in C57BL/6 mice.
Figure 2. Inhibition of metastasis of B16F10 melanoma induced by pcDNA3-p42.3 vaccination. C57BL/6 mice immunized with pcDNA3-p42.3 vaccine, vector control pcDNA3 or PBS were challenged with B16F10 melanoma cells i.v.; 17 d after the challenge, mice were sacrificed and metastases in lung were photographed (A) and analyzed (B). Each value is the mean ± SEM from eight mice. Every experiment was done at least three times, and one representative experiment is shown. *p ≤ 0.05, **p ≤ 0.01 compared with other groups by the Student t-test.
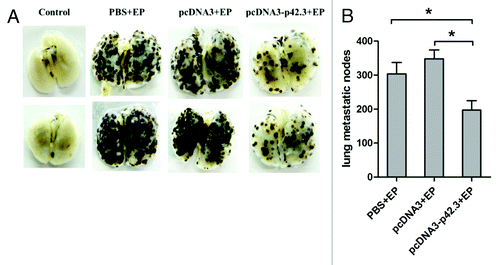
Therapeutic effects of pcDN3-p42.3 vaccination on B16F10 melanoma subcutaneous model
From the point of view of clinical application, it is important to examine the therapeutic effects of this vaccination strategy against established melanoma. Mice were first given 1 × 105 B16F10 cells 2 d before starting immunization (). As shown in , three immunizations of tumor-bearing mice with pcDNA3-p42.3 resulted in marked suppression of tumor growth and reduction of tumor weight compared with mice immunized with PBS or pcDNA3. Vaccination with pcDNA3-p42.3 also partially extended the survival time of the mice ().
Figure 3. Therapeutic effects of pcDN3-p42.3 vaccination on B16F10 the subcutaneous melanoma model. (A) C57BL/6 mice were first subcutaneously injected with 1 × 105 B16F10 cells, then after 2 d of incubation the mice were immunized three times at weekly intervals with 30 μl of pcDNA-p42.3 (1 mg/ml), or pcDNA3 (1 mg/ml) or PBS. Tumor size (B), (C) was measured every 2 d. At day 22, solid tumors were isolated from the mice and weighed (D). Another group of mice was monitored for survival (E). In (B), (C), and (D), each value represents the mean ± SEM from seven or eight mice in each group. Every experiment was done at least three times, and one representative experiment is shown. *p ≤ 0.05, **p ≤ 0.01 compared with other groups by the Student t-test. In (E), results are expressed as the percentages of surviving mice in groups of seven or eight mice. *p ≤ 0.05 compared with other groups by the log-rank test.
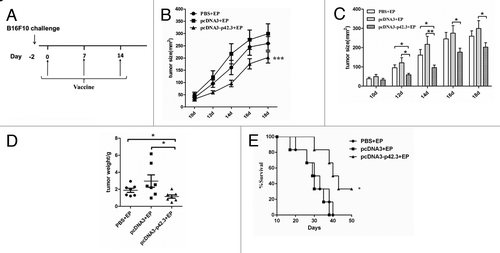
A critical role of antigen specific CD8+ T cells in the antitumor responses
DNA vaccine is known to induce CD8+ CTL activities that are important for the inhibition and clearance of melanoma. We used CD8 knockout mice to assess the importance of CD8 cells for protection here. As shown in , absence of CD8+ T cells completely abolished the protective responses against the B16F10 cell challenge that were otherwise induced by vaccinating 3 times with pcDNA3-p42.3 in wild type animals. Therefore, we examined whether activated CD8+ T cells were induced by the vaccination. CTL assays were performed to verify the specific CTL activity of CD8+ T cells in vivo. As showed in , p42.3-specific CTLs were significantly increased in mice immunized with pcDNA3-p42.3 compared with mice in the control group.
Figure 4. A critical role of antigen specific CD8+ T cells in the antitumor responses. (A) C57BL/6 WT mice and CD8-KO mice were first subcutaneously incubated with 1 × 105 B16F10 cells, then after 2 d of incubation mice were immunized three times at weekly intervals with 30 μl of pcDNA-p42.3 (1 mg/ml) or PBS. Tumor size was measured every 3 d. Each value represents the mean ± SEM *** p ≤ 0.0001 compared with control group by two-way ANOVA test. (B) Analysis of in vivo cytotoxic lysis on day 7 after the final immunization. In vivo CTL assays were as described in Materials and Methods. (C) The percentage of specific lysis summarized as the mean ± SEM of three independent experiments. Data shown are representative from three independent experiments. *p ≤ 0.05, **p ≤ 0.01, ***p ≤ 0.0001 compared with other groups by the Student t-test.
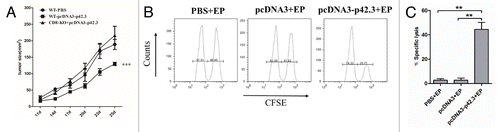
Functional analyses of p42.3-specific CD8+ T cells induced by vaccination with pcDNA3-p42.3
To test if the induced CD8+ T cell is also multi-functional, we analyzed cytokine expression by intracellular staining of activated CD8+ T cells. The production of intracellular factors including perforin, granzyme B, IFN-γ and TNF-α was analyzed by flow cytometry (). Production of IFN-γ, perforin, and granzyme B was significantly induced in CD8+T cells of mice vaccinated with pcDNA3-p42.3 (); the percentage of TNF-α positive CD8+ T cells was also slightly higher (). Thus, activated multi-functional CD8+ T cells specific for the p42.3 antigen were strongly induced by vaccination with pcDNA3-p42.3.
Figure 5. Functional analyses of p42.3-specific CD8+ T cells induced by vaccination with pcDNA3-p42.3. Spleen cells were isolated 7 d after the last vaccination and analyzed for function of CD8+ T cells. Percentages of CD8 T cells positive for perforin or granzyme B (A) and for IFN-γ or TNF-α (B) were analyzed by FACS. (C) The percentages of positive-stained cells are summarized as the means ± SEM from three independent experiments. Every experiment was done at least three times, and one representative experiment is shown. *p ≤ 0.05, **p ≤ 0.01 compared with other groups by the Student t-test.
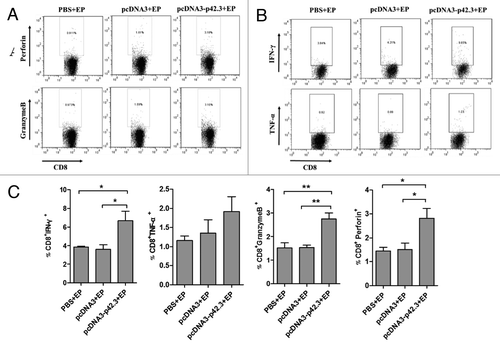
Discussion
In this study, we demonstrated not only that prior DNA immunization with pcDNA3-p42.3 rendered mice significantly resistant to challenge with B16F10 cells, but also that treatment of tumor-bearing mice with the pcDNA3-p42.3 vaccine resulted in marked suppression of tumor growth. The effects were specific in that the pcDNA3 vaccine vector itself had no significant antitumor action. Thus immunization using pcDNA3-p42.3 was effective against the malignant B16F10 melanoma both preventively and therapeutically.
The anti-tumor effects of the vaccination were accompanied by the induction of multi-functional CD8+ T cells () that mediated a strong p42.3 specific cytotoxic activity in vitro (). Cytotoxic CD8+T cells (CTL) are generally considered as the main effectors of immunity against tumors and are known to act by specific recognition of specific tumor antigens; p42.3 in this case.Citation22 By releasing various molecules such as cytokines, perforin and granzyme B, these effector T cells are capable of inducing apoptotic death of tumor cells.Citation23 The strong induction of cytotoxic T cells is consistent with the inherent capacity of DNA vaccines to induce strong cellular immune responses in addition to humoral responses, in particular favoring induction of killer cytotoxic CD8+T lymphocytes.Citation16
Cellular uptake of DNA is a significant barrier to transfection in vivo, and hence to DNA vaccine efficacy. Electroporation appears to be able to overcome this barrierCitation24 and electroporation technology has provided a breakthrough for DNA vaccine development. For example, electroporation of a hepatitis B virus (HBV) surface antigen DNA vaccine has induced potent CTL responses in mice and rabbits,Citation25 and the immunogenicity of a potent HIV gag DNA vaccine was increased in mice.Citation24 In this study, we used the Inovio’s Cellectra device to efficiently deliver the DNA vaccine into mice. The efficiency was demonstrated as lower DNA plasmid dose required and higher immune responses induced (). Moreover, EP has been applied to clinical trials and the exciting findings were obtained with results similar to the pre-clinical animal model.Citation21 EP appears to enhance the immunogenicity of DNA vaccine in the human as well.Citation26 A number of other strategies, including plasmid design and optimization, immunoregulatory and molecular adjuvants, prime-boost strategies, and strategies to break the immunosuppressive regulation were used to enhance the immunogenicity and efficacy of DNA vaccines.Citation27 IL-15 enhances in vivo antitumor activities of tumor-reactive CD8+ T cells. The use of CD40-expressing or IL-15 plasmid to enhance immune responses of DNA vaccine has been reported previouslyCitation28-Citation30 Therefore, antitumor effect of this DNA vaccine could be augmented by adding these molecular adjuvants. Moreover, combination of DNA vaccines and traditional chemotherapy should be also considered as a combinational therapy during the antitumor treatments.Citation31
In summary, vaccination with pcDNA3-p42.3 induced effective anti-tumor responses both therapeutically and prophylactically. This study provides the first evidence that the p42.3 could be used as a potential novel target for cancer vaccine development. Further optimization may in future lead to clinical immunotherapy against malignant tumors.
Materials and Methods
Animals, cell line and reagents
Female C57BL/6 mice of 8–10 weeks of age were purchased from the Animal Institute of Chinese Medical Academy and kept in SPF condition. All animal experiments were approved by the Animal Experiments Committee of China Agricultural University. C57BL/6 CD8 knockout (CD8-KO) mice were kindly provided by Richard Flavell (Yale University School of Medicine). B16F10 cell line (ATCC CRL-6475) and NIH-3T3 cell line (ATCC CRL-1658) were cultured at 37°C in 5% CO2 in complete media consisting of DMEM, 100 U/ml penicillin, 100 μg/ml streptomycin, and 10% fetal bovine serum (FCS). Anti-p42.3 monoclonal antibody was produced in Dr. Lu’s Laboratory and p42.3 CTL epitopes for H-2b mouse and human (HLA-A and HLA-B) were predicted using online database tools (IEDBa, BIMAS, syfpeithi) according to a previous protocol.Citation32 The p42.3-derived CTL peptide p27–35 (AFLQSLRTL) and p76–84 (APASGYLTF) were synthesized by GL Biochem Co., Ltd.
Plasmid preparation and immunization with EP
Plasmids pcDNA3-p42.3, proVAX-IL-15, pcDNA3-CD40 and pcDNA3-IL-17A were constructed in lab, maxi-prepared by the alkaline method, and subsequently purified by Qiagen Maxi-prep kit (Qiagen Inc.) and diluted in saline solution.
The C57BL/6 mice were randomly divided into three groups and immunized intramuscularly with 30 μg plasmid (1 μg/μl) or 30 μl PBS as control via electroporation (Cellatron, Inovio Inc.) on days 0, 14, 28, and 42.
Expression and purification of protein p42.3 and ELISA
Subcloning of plasmid pET28a-p42.3 was performed in Dr. Lu’s lab. Protein p42.3 was purified from pET28a-p42.3 transformed E.coli BL21 (DE3) according to a previous protocolCitation33 using BioLogic DuoFlow system (Bio-Rad). The concentration of purified protein was analyzed by bicinchoninic acid (BCA; Thermo Scientific) and verified by Western Blot using anti-his tag antibody and anti-p42.3 monoclonal antibody.
The detection of anti-p42.3-specific antibody in sera was done by ELISA. The 96-well microtiter plates were coated with 2 μg/mL of purified protein p42.3 at 4 °C overnight at 100 μL per well. These wells were subsequently blocked with 5% of BSA-PBST for 1 h at 37 °C, and reacted with serial dilutions of mouse sera. After three washes, 1:4000 diluted goat anti-mouse IgG conjugated with horseradish peroxidase was added to the wells and the plates were incubated at 37 °C for 1 h. Then, after three washes, 100 μl of TMB dissolved in 0.025 M phosphate-citrate buffer was added to each well and the reaction was stopped by the addition of 50 μl of 2 M H2SO4. The optical density (OD) at 450/620nm was then analyzed with a plate reader (Bio-Rad). The titer of anti-p42.3 antibody from the immunized mice was determined as the maximum dilution with an OD ratio greater than or equal to 2.
Tumor challenge
For the subcutaneous tumor model, each mouse was subcutaneously (s.c.) inoculated with 1 × 105 of B16F10 cells into the flank on day 7 after the final immunization. After measurable (4–5 mm) tumors had grown (between 5 and 10 d after tumor challenge), tumor growth was then measured using calipers every 2 d and was recorded as the greatest longitudinal diameter (length) and the greatest transverse diameter (width). Tumor size based on caliper measurements were calculated by the modified ellipsoidal formula: Area = π/4 (length × width). Mice were sacrificed when tumor sizes reached about 20–25mm in average diameter. Then solid tumors were isolated from the mice and weighed. For the pulmonary metastatic melanoma model, each mouse was inoculated with 4 × 105 of B16F10 cells into the lateral vein of the tail on day 7 after the final immunization. Mice were sacrificed 15 to 19 d after tumor inoculation and pulmonary nodules were enumerated using a dissecting microscope (Olympus).
In vivo cytotoxic assay
Splenocytes from naive C57BL/6 mice were pulsed with 10−6 M p42.3-derived peptides and labeled with a high concentration of CFSE (15 μM) to create CFSEhigh target cells. A portion of the same splenocytes was alternatively labeled with a low concentration of CFSE (1μM; CFSElow cells) without peptide pulse as a non-target control. The target and control cells were mixed in a 1:1 ratio and injected into immunized mice at 2 × 107 total cells per mouse via the tail vein on day 7 after the final immunization. Eight hours later lymph nodes and the spleen of injected mice were isolated and the target and control cells were analyzed by their differential CFSE fluorescent intensities using a FACSCalibur (BD Biosciences). Specific lysis was calculated using the following formula: Percentage specific lysis = (1- [ratio unprimed/ratio primed] × 100), where ratio = percentage CFSElow/percentage CFSEhigh.
Flow cytometry
Single-cell suspensions were prepared from mouse spleens on day 7 after the final immunization, the cells were then stimulated in 96-well plates with p42.3 peptide and anti-CD28 (5 μg/mL) mAb in the presence of BD GolgiPlug (BD Biosciences) for 6 h at 37 °C and 5% CO2. The cells were blocked with Mouse BD Fc Block (BD Biosciences) for 30 min at 4 °C, and fixed with 4% paraformaldehyde at 4 °C for 15 min. The cells were permeabilized with 0.1% saponin (Sigma-Aldrich) at 4 °C for 10 min, then immunostained for 30 min at 4 °C with pairs of labeled antibodies: anti-CD8-APC and anti-granzymeB-PE; anti-CD8-APC and anti-IFN-γ-PE; anti-CD8-APC and anti-perforin-PE; anti-CD8-APC and anti- TNF-α-PE. Data were acquired and analyzed using a FACS Calibur with Cell Quest software (BD Biosciences). All fluorescent-conjugated anti-mouse monoclonal antibodies were purchased from eBioscience.
Statistical analysis
Results are presented as means with standard error of the mean (SEM). Student’s t-test analysis was used for data analysis. Survival was analyzed using the log-rank test. Analyses were performed with GraphPad Prism 5 software (GraphPad). A value of p ≤ 0.05 was considered statistically significant.
Additional material
Download Zip (103.1 KB)Acknowledgments
This work was supported in part by Chinese National Natural Science Foundation (30930068) and the National High-Tech 863 Project of China (2010AA022907) to BW. We thank Dr Douglas Lowrie for his critical review of the manuscript and Dr. Jane QL Yu and Mr Zhonghuai He for their assistance in this work.
Conflict of Interest
No competing financial interests exist.
References
- Flaherty KT, Hodi FS, Fisher DE. From genes to drugs: targeted strategies for melanoma. Nat Rev Cancer 2012; 12:349 - 61; http://dx.doi.org/10.1038/nrc3218; PMID: 22475929
- Vidwans SJ, Flaherty KT, Fisher DE, Tenenbaum JM, Travers MD, Shrager J. A melanoma molecular disease model. PLoS One 2011; 6:e18257; http://dx.doi.org/10.1371/journal.pone.0018257; PMID: 21479172
- Pan Z-K, Weiskirch LM, Paterson Y. Regression of established B16F10 melanoma with a recombinant Listeria monocytogenes vaccine. Cancer Res 1999; 59:5264 - 9; PMID: 10537307
- Zhao F, Dou J, He XF, Wang J, Chu L, Hu W, et al. Enhancing therapy of B16F10 melanoma efficacy through tumor vaccine expressing GPI-anchored IL-21 and secreting GM-CSF in mouse model. Vaccine 2010; 28:2846 - 52; http://dx.doi.org/10.1016/j.vaccine.2010.01.057; PMID: 20153795
- van den Boorn JG, Konijnenberg D, Tjin EP, Picavet DI, Meeuwenoord NJ, Filippov DV, et al. Effective melanoma immunotherapy in mice by the skin-depigmenting agent monobenzone and the adjuvants imiquimod and CpG. PLoS One 2010; 5:e10626; http://dx.doi.org/10.1371/journal.pone.0010626; PMID: 20498710
- Xu X, Li W, Fan X, Liang Y, Zhao M, Zhang J, et al. Identification and characterization of a novel p42.3 gene as tumor-specific and mitosis phase-dependent expression in gastric cancer. Oncogene 2007; 26:7371 - 9; http://dx.doi.org/10.1038/sj.onc.1210538; PMID: 17525738
- Mao L, Sun W, Li W, Cui J, Zhang J, Xing R, Lu Y. Cell cycle-dependent expression of p42.3 promotes mitotic progression in malignant transformed cells. Mol Carcinog Forthcoming 2012; http://dx.doi.org/10.1002/mc.21982; PMID: 23192843
- Jung Y, Lee S, Choi H-S, Kim S-N, Lee E, Shin Y, et al. Clinical validation of colorectal cancer biomarkers identified from bioinformatics analysis of public expression data. Clin Cancer Res 2011; 17:700 - 9; http://dx.doi.org/10.1158/1078-0432.CCR-10-1300; PMID: 21304002
- Zhang J, Lu C, Shang Z, Xing R, Shi L, Lv Y. p42.3 gene expression in gastric cancer cell and its protein regulatory network analysis. Theor Biol Med Model 2012; 9:53; http://dx.doi.org/10.1186/1742-4682-9-53; PMID: 23228105
- Cui Y, Su W-Y, Xing J, Wang Y-C, Wang P, Chen X-Y, et al. MiR-29a inhibits cell proliferation and induces cell cycle arrest through the downregulation of p42.3 in human gastric cancer. PLoS One 2011; 6:e25872; http://dx.doi.org/10.1371/journal.pone.0025872; PMID: 21998710
- Hutchins JR, Toyoda Y, Hegemann B, Poser I, Hériché J-K, Sykora MM, et al. Systematic analysis of human protein complexes identifies chromosome segregation proteins. Science 2010; 328:593 - 9; http://dx.doi.org/10.1126/science.1181348; PMID: 20360068
- Fioretti D, Iurescia S, Fazio VM, Rinaldi M. DNA vaccines: developing new strategies against cancer. J Biomed Biotechnol 2010; 2010:174378; http://dx.doi.org/10.1155/2010/174378; PMID: 20368780
- Donnelly JJ, Wahren B, Liu MA. DNA vaccines: progress and challenges. J Immunol 2005; 175:633 - 9; PMID: 16002657
- Signori E, Iurescia S, Massi E, Fioretti D, Chiarella P, De Robertis M, et al. DNA vaccination strategies for anti-tumour effective gene therapy protocols. Cancer Immunol Immunother 2010; 59:1583 - 91; http://dx.doi.org/10.1007/s00262-010-0853-x; PMID: 20390416
- Alam S, McNeel DG. DNA vaccines for the treatment of prostate cancer. Expert Rev Vaccines 2010; 9:731 - 45; http://dx.doi.org/10.1586/erv.10.64; PMID: 20624047
- Kutzler MA, Weiner DB. DNA vaccines: ready for prime time?. Nat Rev Genet 2008; 9:776 - 88; http://dx.doi.org/10.1038/nrg2432; PMID: 18781156
- Rice J, Ottensmeier CH, Stevenson FK. DNA vaccines: precision tools for activating effective immunity against cancer. Nat Rev Cancer 2008; 8:108 - 20; http://dx.doi.org/10.1038/nrc2326; PMID: 18219306
- Saade F, Petrovsky N. Technologies for enhanced efficacy of DNA vaccines. Expert Rev Vaccines 2012; 11:189 - 209; http://dx.doi.org/10.1586/erv.11.188; PMID: 22309668
- Demoulin S, Herfs M, Delvenne P, Hubert P. Tumor microenvironment converts plasmacytoid dendritic cells into immunosuppressive/tolerogenic cells: insight into the molecular mechanisms. J Leukoc Biol 2013; 93:343 - 52; http://dx.doi.org/10.1189/jlb.0812397; PMID: 23136258
- van Drunen Littel-van den Hurk S, Hannaman D. Electroporation for DNA immunization: clinical application. Expert Rev Vaccines 2010; 9:503 - 17; http://dx.doi.org/10.1586/erv.10.42; PMID: 20450325
- Sardesai NY, Weiner DB. Electroporation delivery of DNA vaccines: prospects for success. Curr Opin Immunol 2011; 23:421 - 9; http://dx.doi.org/10.1016/j.coi.2011.03.008; PMID: 21530212
- van Duikeren S, Fransen MF, Redeker A, Wieles B, Platenburg G, Krebber W-J, et al. Vaccine-induced effector-memory CD8+ T cell responses predict therapeutic efficacy against tumors. J Immunol 2012; 189:3397 - 403; http://dx.doi.org/10.4049/jimmunol.1201540; PMID: 22914049
- Restifo NP, Dudley ME, Rosenberg SA. Adoptive immunotherapy for cancer: harnessing the T cell response. Nat Rev Immunol 2012; 12:269 - 81; http://dx.doi.org/10.1038/nri3191; PMID: 22437939
- Widera G, Austin M, Rabussay D, Goldbeck C, Barnett SW, Chen M, et al. Increased DNA vaccine delivery and immunogenicity by electroporation in vivo. J Immunol 2000; 164:4635 - 40; PMID: 10779767
- Luxembourg A, Hannaman D, Ellefsen B, Nakamura G, Bernard R. Enhancement of immune responses to an HBV DNA vaccine by electroporation. Vaccine 2006; 24:4490 - 3; http://dx.doi.org/10.1016/j.vaccine.2005.08.014; PMID: 16140436
- Low L, Mander A, McCann K, Dearnaley D, Tjelle T, Mathiesen I, et al. DNA vaccination with electroporation induces increased antibody responses in patients with prostate cancer. Hum Gene Ther 2009; 20:1269 - 78; http://dx.doi.org/10.1089/hum.2009.067; PMID: 19619001
- Gill DR, Pringle IA, Hyde SC. Progress and prospects: the design and production of plasmid vectors. Gene Ther 2009; 16:165 - 71; http://dx.doi.org/10.1038/gt.2008.183; PMID: 19129858
- Di Carlo E, Comes A, Basso S, De Ambrosis A, Meazza R, Musiani P, et al. The combined action of IL-15 and IL-12 gene transfer can induce tumor cell rejection without T and NK cell involvement. J Immunol 2000; 165:3111 - 8; PMID: 10975824
- Klebanoff CA, Finkelstein SE, Surman DR, Lichtman MK, Gattinoni L, Theoret MR, et al. IL-15 enhances the in vivo antitumor activity of tumor-reactive CD8+ T cells. Proc Natl Acad Sci U S A 2004; 101:1969 - 74; http://dx.doi.org/10.1073/pnas.0307298101; PMID: 14762166
- Xu H, Zhao G, Huang X, Ding Z, Wang J, Wang X, et al. CD40-expressing plasmid induces anti-CD40 antibody and enhances immune responses to DNA vaccination. J Gene Med 2010; 12:97 - 106; http://dx.doi.org/10.1002/jgm.1412; PMID: 19950201
- Zitvogel L, Apetoh L, Ghiringhelli F, Kroemer G. Immunological aspects of cancer chemotherapy. Nat Rev Immunol 2008; 8:59 - 73; http://dx.doi.org/10.1038/nri2216; PMID: 18097448
- Lundegaard C, Lund O, Keşmir C, Brunak S, Nielsen M. Modeling the adaptive immune system: predictions and simulations. Bioinformatics 2007; 23:3265 - 75; http://dx.doi.org/10.1093/bioinformatics/btm471; PMID: 18045832
- Zhou G, Parthasarathy G, Somasundaram T, Ables A, Roy L, Strong SJ, et al. Expression, purification from inclusion bodies, and crystal characterization of a transition state analog complex of arginine kinase: a model for studying phosphagen kinases. Protein Sci 1997; 6:444 - 9; http://dx.doi.org/10.1002/pro.5560060222; PMID: 9041648