Abstract
Signaling via the Ras/Erk pathway has long been recognized to be critical in lymphocyte development and function, yet the mechanisms that control the distinct functional outputs of this pathway in different cellular contexts remain poorly understood. Our recent results have demonstrated unexpected involvement of Ras/Erk signaling in the sensitization of B cells to apoptosis in order to eliminate autoreactive cells. Increases in cytosolic Ca2+ are necessary and sufficient to induce activation of this Ras/Erk pathway, and the biochemical events involved in its activation are different from the ones involved in diacylglycerol (DAG)-mediated Ras/Erk activation. Developmental regulation of upstream mediators of these distinct pathways contributes to their predominant activation at different stages of B cell development. These findings have revealed a mechanism by which antigen stimulation can activate distinct Ras/Erk pathways at different developmental stages to mediate appropriate functional outputs that control the selection, development and activation of B cells. Despite these recent findings, however, much remains to be learned about the molecular mechanisms that confer functional specificity to common Ras/Erk signaling modules.
The Ras pathway has long been recognized as an important pathway in lymphocyte development and activation.Citation1 Ras proteins were originally identified on the basis of their oncogenic potential, and have since been implicated in the regulation of important signaling pathways in many cell types. Ras proteins have intrinsic GTPase activity and exist in a dynamic equilibrium between the GTP-bound state (active) and the GDP-bound state (inactive); RasGTP production is catalyzed by Ras guanine nucleotide exchange factors (RasGEFs), while hydrolysis to RasGDP is accelerated by Ras GTPase-activating proteins (RasGAPs). Ras signaling typically involves activation of a GEF, such as SOS, RasGRF or RasGRP, which triggers RasGTP production. RasGTP then activates Raf proteins; activated Raf phosphorylates MEK and MEK phosphorylates Erk. Erk has the potential to phosphorylate many targets, making this a remarkably promiscuous pathway, and its activation has been implicated in a wide variety of cellular processes in multiple cell types. Therefore, the mechanisms that convey specificity to this pathway have been an active field investigation. Current models suggest that scaffolding of Ras/Erk signaling complexes plays a crucial role in determining the functional output of the signals.Citation2,Citation3 By bringing together the upstream activators of the pathway, the components of the Ras/Erk cascade and the appropriate substrates, scaffolds ensure that the pathway is activated by the proper stimuli and at the proper location to gain access to appropriate substrates while being sequestered away from other non-specific substrates.
Stimulation of lymphocytes via the antigen receptor triggers uniquely high levels of RasGTP production,Citation1,Citation4,Citation5 and recent work has elucidated the mechanisms by which such elevated levels of RasGTP are generated. Lymphocytes express RasGEFs from at least two different families, namely SOS and RasGRP. RasGRP proteins contain two Ca2+-binding EF hands and a diacylglycerol (DAG)-binding C1 domain; expression of RasGRP1 and RasGRP3 is largely restricted to neuronal and hematopoietic cells. DAG binding to RasGRP has been implicated in its activation, as has PKC-mediated phosphorylation of a threonine residue (T184 in RasGRP1, corresponding to T133 in RasGRP3).Citation6–Citation8 SOS proteins, in contrast, are ubiquitously expressed and their activation is regulated by recruitment to sites of receptor or adaptor phosphorylation. In addition, SOS proteins contain an allosteric pocket that, upon binding RasGTP, increases their GEF activity 75-fold.Citation9 A current model proposes that, upon antigenic stimulation, RasGRP is initially activated by DAG, leading to production of an initial pool of RasGTP; this RasGTP can then bind to the allosteric pocket of SOS, increase its catalytic activity and thereby drive a feed-forward loop for RasGTP production. The synergistic activation of these two GEFs would then account for the unusually high RasGTP production observed in lymphocytes.Citation10,Citation11
Although DAG production has been extensively associated with activation of Ras/Erk signaling in lymphocytes, Ras/Erk pathway activation in lymphocytes has been thought to be largely Ca2+-independent. This notion comes from early studies demonstrating that DAG analogs (such as PMA), but not Ca2+ ionophores, could potently induce the Ras activation in lymphocytes.Citation4 The involvement of RasGRP and PKCs in Ras/Erk activation in lymphocytes explains the early observations that showed that DAG analogs could potently induce Ras activation. However, in other cell types, perhaps most notably in the nervous system, it has become increasingly clear that Ca2+ can lead to Ras/Erk activation by means of Ca2+-responsive GEFs (in addition to RasGRP, neuronal cells express RasGRF, which is potently activated by Ca2+).Citation12
Our recent results now demonstrate that Ca2+ can potently induce Erk activation in immature and transitional bone marrow B cells. Our unexpected findings linking Ca2+ to Erk activation were first obtained while examining a B cell line overexpressing Stim1. Stim1 is an ER transmembrane protein that contains a Ca2+-binding EF hand domain localized within the lumen of the ER. Upon ER Ca2+ store emptying, Stim1 becomes unbound from Ca2+, aggregates into punctate structures, and moves toward the plasma membrane,Citation13–Citation16 where it interacts directly or indirectly with plasma membrane channels (Orai proteins) and causes them to open.Citation17–Citation22 Extracellular Ca2+ then enters the cell following a ten thousand-fold gradient, a process known as store-operated Ca2+ entry (SOCE), thereby sustaining increases in cytoplasmic Ca2+ concentration. DT40 avian B cells that were made to overexpress Stim1 displayed augmented amplitude and duration of SOCE, which was sufficient to sensitize the cells to antigen receptor-induced apoptosis. In this Stim1-overexpressing cell, Ca2+-dependent Erk activation required PKCδ and RasGRP, but not SOS, proteins, and disrupting this pathway by means of RasGRP deletion was sufficient to rescue the cells from antigen receptor-induced apoptosis. Strikingly, this pathway is also active in primary B cells, where this Ca2+-Erk pathway is selectively activated at the bone marrow immature and transitional stages of B cell development. These developing B cells undergo apoptosis in response to antigen, one critical mechanism that eliminates self-reactive B cells to prevent later autoimmunity (). We also demonstated that PKCδ and RasGRP1 protein expression is highest at these developmental stages. Moreover, PKCδ-/- B cells display a competitive advantage over wild type cells at this developmental stage concomitant with a marked defect in activation of the Ca2+-Erk pathway, and the PKCδ-/- mice subsequently develop severe B cell autoimmunity. In contrast, developing bone marrow B cells that overexpress Stim1 display increased propensity to negative selection, an effect that depends on PKCδ.Citation23
The involvement of PKCδ in this pathway was unexpected and revealed additional mechanisms that modulate RasGRP1 function that were not previously known. We identified a new phosphorylation site on RasGRP1, S332, which is a putative PKCδ target site and selectively modulates the responsiveness of RasGRP1 to Ca2+. Phosphorylation at S332 is not required to activate Erk via the DAG pathway, but it is absolutely required for Ca2+-mediated Erk activation. Further, although phosphorylation of T184 on RasRGP1 (which corresponds to T133 on RasGRP3) had previously been identified and shown to be crucial for optimal Erk activation via the DAG pathway,Citation7,Citation8,Citation24 phosphorylation of this site was largely dispensable for Ca2+-dependent Erk activation. These results demonstrated that RasGRP1 activity can be modulated by multiple mechanisms. These mechanisms may involve Ca2+, DAG or both second messengers, and likely involve at least two phosphosites, T184 and S332. Different cellular perturbations at different developmental stages may therefore induce RasGRP1 activation by unique mechanisms that contribute to its functional specificity.
By homology modeling, we predicted that S332 lies in close proximity to the Ras-binding site within the CDC25 domain of RasGRP1. A phosphorylation event at this site may create electrostatic interactions that influence Ras binding. Indeed, it is possible that such interactions may change the affinity of different isoforms of Ras or Ras-like GTPases for RasGRP1. Because different GTPases have unique trafficking and localization properties, a phosphorylation event that alters the choice of Ras molecule to activate could very well have an impact on its functional output. Alternatively, phosphorylation of this site could establish electrostatic interactions with other charged residues within the CDC25 domain, particularly in flap2, which is predicted to abut the phosphosite. Such interactions could thereby coordinate the enzymatic activity of the exchange factor. Our results indicate that mutation of this site to alanine disrupts the responsiveness of RasGRP1 to Ca2+. However, a BCR-induced DAG signal is sufficient to trigger activity of this mutant, albeit at lower levels than wild type. These results demonstrate that mutation of this site does not sufficiently alter the conformation of RasGRP1 to disrupt the enzymatic activity of the protein, but rather modulates its responsiveness to Ca2+. However, based on the reduced activity observed in response to the BCR-induced DAG signal, it is possible that subtle conformational changes in the CDC25 domain induced by phospho-S332 may improve the enzymatic function of the protein.
It is noteworthy that a phosphomimetic mutation at this site, S332E, RasGRP1 behaves like WT RasGRP1, indicating that phospho-S332 is permissive for activation of both the Ca2+- and the DAG-induced Erk pathways. However, this phosphomimetic residue does not lead to constitutive activation of either pathway. Therefore, while phosphorylation of RasGRP1 on S332 is required for Ca2+-dependent Erk activation, it is not sufficient to activate the pathway, implying that other Ca2+-dependent events may be at play. As mentioned above, RasGRP proteins contain both a DAG-binding C1 domain and two Ca2+-binding EF hands. Mutagenesis studies have uncovered an important role for the C1 domain in DAG-mediate Erk activation, but the role of the EF hands is still not well understood.Citation7,Citation8,Citation24 Interestingly, and consistent with our results, one report demonstrated that RasGRP1 can sensitize a WEHI-231 immature B cell line to antigen receptor-induced apoptosis, and this effect seemed to depend on the EF hands.Citation25 This implies that Ca2+ binding to RasGRP1 via the EF hands may be important for its ability to mediate apoptotic signals. Combined with our findings, these results suggest that Ca2+ binding may either alter the conformation of RasGRP1 to facilitate phosphorylation of S332, or may synergize with this phosphorylation event to induce pro-apoptotic signals in B cells. However, at this point we cannot rule out that other Ca2+-dependent events are at play in this pathway, possibly involving additional Ca2+-modulated proteins.
Another mechanism that may play an important role in establishing specificity in these pathways is control of subcellular localization of signaling complexes by Ca2+ and DAG. It is widely recognized that gradients of these second-messengers can recruit proteins to subcellular locations where the second-messengers are generated. As mentioned above, developing B cells that are susceptible to negative selection display increased amplitude and duration of SOCE and lower levels of DAG in response to antigen stimulation as compared with their mature counterparts.Citation26–Citation29 It is therefore possible that these second messengers compete to recruit active Erk complexes to particular subcellular compartments where their downstream substrates will vary. While speculative, this hypothesis is consistent with previous reports that demonstrate that RasGRP proteins can relocalize within cells in response to stimulation.Citation30 In addition, the fact that Ca2+ and DAG induce Erk activation via distinct biochemical pathways that rely upon proteins that contain Ca2+ and DAG binding domains suggests that these second messengers may act, not only to promote activation of one pathway, but also to simultaneously suppress activation of the second pathway, a model that makes a lot of sense when diametrically opposite cell fates are at stake (e.g., proliferation vs. apoptosis). Based on this, it is not far-fetched to imagine that, while Ca2+-induced phosphorylation of S332 and possibly Ca2+ binding to the EF hands of RasGRP1 promote apoptosis, DAG binding to the C1 domain might alter its conformation and/or localization sufficiently to disrupt signaling from this Ca2+-activated complex, while promoting signaling from a newly-formed DAG-dependent complex. This idea becomes even more compelling when we consider the fact that PKCδ is a non-classical PKC, which contains only a DAG binding C1 domain but no ability to bind Ca2+, yet it is this PKC isoform that is involved in RasGRP1 modulation downstream of Ca2+ elevations. Therefore, it is possible that other Ca2+ dependent events (perhaps Ca2+ binding to RasGRP1 and/or phosphorylation of S332 RasGRP1) act to promote the PKCδ-RasGRP1 interaction, while DAG binding could sufficiently alter the conformation and localization of these proteins to disrupt their interaction. This idea is also consistent with published observations that suggest that retention of PKCδ at the plasma membrane by DAG may be a mechanism to inhibit its proapoptotic functions.
The subcellular localization of Ras/Erk signaling modules is increasingly viewed as a crucial mechanism that confers specificity to these signaling pathways. This model proposes that scaffold proteins that localize to different subcellular compartments recruit Ras/Raf/Mek/Erk signaling modules to a particular location where the signaling cascade can be activated by appropriate stimuli and simultaneously gain access to appropriate substrates. This model for signaling specificity is not exclusive to MAPK signaling, and one other well characterized example of functionally distinct signaling complexes that contain the same effector molecules are the mTOR-containing complexes mTORC1 and mTORC2.Citation31–Citation33 Examples of Ras/Erk scaffolds are the KSR proteins, CNK, Sef and MP-1,2,Citation34–Citation38 but it is unclear whether any of these proteins play a role in the induction of either of these Erk pathways in B cells. Studies in T cells have shown that Ras/Erk signaling can occur at the plasma membrane as well as in endomembranes, most prominently in the Golgi, and RasGRP1 has also been found to shuttle between these compartments in response to stimulation.Citation30,Citation39,Citation40 In DT40 B cells, RasGRP1 has been found to accumulate at the plasma membrane in response to BCR crosslinking, and this effect seems to require EF1, but not Ca2+.Citation41 Previous data demonstrate that PLCγ is recruited to the site of antigen stimulation, and that a gradient of DAG from this site is generated and contributes to the recruitment of proteins toward this site.Citation42,Citation43 In contrast, the migration of Stim1-containing punctae toward the plasma membrane in cells stimulated with thapsigargin or soluble antibody is not constrained to a particular section of the membrane, implying that CRAC channels likely open throughout the plasma membrane.Citation13,Citation15 Although this does not appear to be the case when the stimulation depends on cell-cell contact and an immunological synapse is formed, in these cases Stim1 punctae have been shown to accumulate not only at the synapse, but also at the distal cap of the cells.Citation44,Citation45 These observations suggest that perhaps spatial sequestration of DAG and Ca2+ plays an important role in controlling the localization of Erk signaling: DAG-mediated restriction of Ras/Erk modules to sites of antigen stimulation might protect cells from apoptosis, while Ca2+-mediated Ras/Erk signaling in endomembranes might confer pro-apoptotic signals (). However, this is in direct contrast with what has been observed in T cell selection: in this system, it was found that thymic selection threshold correlated with compartmentalized Ras/MAPK signaling, and plasma membrane localization of the signals was correlated with thymic negative selection, while localization to endomembranes correlated with positive selection.Citation46 It will therefore be essential to further investigate how the subcellular localization of these signaling modules is controlled by Ca2+ and DAG, how these changes in location alter their functional output, what scaffolds control their spatial distribution, and what signals are triggered downstream of Erk at these different locations to mediate proliferation or apoptosis.
Another possible means of regulation of these pathways is by MAPK phosphatases (MKPs). These proteins belong to the dual-specificity family of protein phosphatases (DUSPs), which have the ability to dephosphorylate both phosphotyrosine and phosphoserine/phosphothreonine residues within the same substrate.Citation47 There are ten members of the DUSP family known to act as MKPs, each of which displays unique substrate specificity for Erk, JNK or p38. Expression of these proteins is tissue-specific and developmentally regulated. In some cases, they can act as inducible/early response genes, thereby triggering negative feedback loops over activated MAPKs. Recent studies have uncovered important roles for DUSP1, DUSP2 and DUSP10 in the control of inflammatory responses,Citation48–Citation54 but how these proteins affect lymphocyte development is a question that remains largely unexplored. Interestingly, publicly available data through the Immunological Genome Project website (www.immgen.org/index_content.html) demonstrates that DUSP2, DUSP6 and DUSP10 expression is substantially regulated during B cell development and is much higher in immature and transitional bone marrow cells than in any other B cell compartment. Because this is the same stage where Ca2+-dependent Erk activation occurs, it is provocative to speculate that the expression of these particular DUSPs could shape the outcome of an Erk response by preferentially inhibiting Erk signaling from one, but not the other Erk pahway. It will therefore be interesting to examine whether B cell development and/or function is affected in mice lacking these proteins, and whether the subcellular localization of these phosphatases could relate to the differential outcome of Erk signaling downstream of Ca2+ or DAG.
An additional layer of complexity in the regulation of these pathways relates to the unique biochemical and developmental roles of the different RasGRP proteins. RasGRP1 and RasGRP3 are both expressed in B cells, yet we have found that their expression is regulated very differently during stages of B cell development. Neither of these RasGRP proteins is expressed early in B cell development. RasGRP1 expression is robustly upregulated in immature and transitional B cells, and substantially downregulated in mature B cells. In contrast, RasGRP3 expression levels are relatively low in immature and transitional B cells,Citation23 but are very high in mature B cells.Citation23 Interestingly, although PKCδ is highly expressed in early B cell development, its expression is further increased at the immature and transitional stages, followed by a substantial decrease in mature B cells.Citation23,Citation55 The pattern of expression of these proteins is intriguing and suggests unique functions of the different RasGRP isoforms during development. Indeed, the B cell phenotypes of RasGRP-deficient mice are consistent with this idea. Although it is not yet clear if these phenotypes are B cell intrinsic, RasGRP1-deficient mice develop B cell lymphoproliferation and autoantibody production, while RasGRP3-deficient mice do not.Citation56–Citation58 These characteristics of the RasGRP1-/- mice are similar to what is observed in PKCδ-/- mice. Further, RasGRP1/RasGRP3 double deficient mice do not show B cell lymphoproliferation, suggesting that RasGRP3 is required for the peripheral expansion of RasGRP1-deficient cells.Citation56 These data suggest that different biochemical mechanisms are at play for these two exchange factors to mediate different roles during development, where RasGRP1 could play a role in B cell negative selection, while RasGRP3 may predominantly be involved in proliferative Erk signaling in mature B cells.
How might such an observation relate to the biochemical regulation of RasGRP signaling vis-à-vis the different RasGRP isoforms? One interesting observation is that, although serine 332 is conserved between RasGRP1 and RasGRP3, arginine 335 is not. This residue makes S332 a PKCδ consensus target site on RasGRP1 but not on RasGRP3. Further, this arginine is predicted to lie close enough to the putative phosphate on S332 to establish electrostatic interactions that may significantly change the Ras-binding pocket of RasGRP1 relative to RasGRP3. Indeed, differences in the ability of these two RasGRP isoforms to mediate nucleotide exchange of different GTPases have been documented,Citation59,Citation60 and justify the need to explore the mechanisms by which such specificity is obtained and its functional consequences. It is also not known what role SOS proteins may have during B cell development, and how the interplay between these two families of exchange factors may be regulated during B cell selection checkpoints.
The implication that Ras/Erk signaling may participate in B cell negative selection is interesting, given that recent reports implicate Erk as an important regulator of cell death in neurons and other cell types.Citation61–Citation63 In those systems, it has been observed that differences in duration and localization of Erk signaling correlate with distinct neuronal cell fates, such as proliferation, differentiation or apoptosis. Interestingly, many of the stimuli that can induce such responses, such as NGF or EGF, also induce activation of PLC and subsequent DAG production and Ca2+ entry. Moreover, neurons express proteins from another RasGEF family, namely RasGRF proteins, which are highly sensitive to regulation by Ca2+ via their Ca2+-calmodulin-binding IQ motif.Citation12,Citation64,Citation65 Therefore, it is possible that neurons display analogous Ras/Erk regulatory mechanisms to the one we have uncovered in B cells.
Our recently published findings shed light on the mechanisms by which developmental regulation of SOCE and DAG production alters the functional output of antigen encounter in B cells. They implicate biochemically and functionally distinct regulatory pathways upstream of Ras/Erk in determining B cell fate during development, and raise many more questions concerning the regulation of these pathways. How is the specificity achieved? How does subcellular location of Ras/Erk signaling complexes relate to our findings? What is the role of scaffolding proteins, and which scaffolds are important for each of these pathways? What are the downstream effectors of Erk signaling that determine the proliferation vs. apoptosis decision? To what extent does the developmental control of protein expression alter the configuration of these pathways during development? These are all intriguing and important questions that need to be addressed in order to fully understand the mechanisms that confer functional specificity during B cell selection. Because development of appropriate lymphocyte repertoires is crucial to prevent autoimmune and lymphoproliferative disorders, understanding these mechanisms will be essential in efforts to therapeutically target one pathway with relatively minor or no consequences on closely related but functionally distinct pathways.
Figures and Tables
Figure 1 Antigen encounter triggers receptor editing or apoptosis in developing cells, but leads to activation and proliferation in mature B cells. Precursor B cells undergo VDJ recombination in order to generate a B cell repertoire that is immensely diverse, where each B cell carries a recombined receptor with unique specificity. Because many of these receptors recognize self, antigen encounter during the immature or transitional stages of B cell maturation leads to receptor editing or apoptosis in order to eliminate these autoreactive cells. Once B cells mature, antigen encounter generally triggers proliferative responses leading to an immune response.
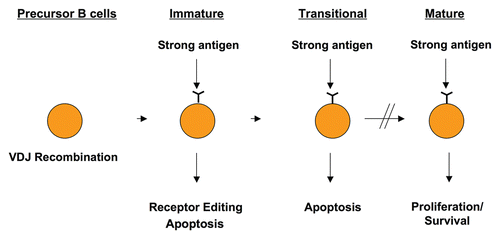
Figure 2 Model for activation of functionally distinct Ras/Erk pathways. The model depicts spatial sequestration of Ca2+- or DAG-dependent signaling complexes as a potential means to couple Ras/Erk signals to appropriate downstream effectors. Ca2+-dependent Erk activation involves PKCδ-mediated phosphorylation of RasGRP1 on S332 and its activation is favored in immature and transitional B cells. DAG-dependent Erk activation may be favored in mature B cells, and likely requires activity of RasGRP3 and phosphorylation of T133 (corresponding to T184 on RasGRP1). Developmental regulation of RasGRP1, RasGRP3 and PKCδ protein levels, as well as changes in the amplification or negative regulation of Ca2+ and DAG, may contribute to the preferential activation of these pathways at different stages in B cell development.
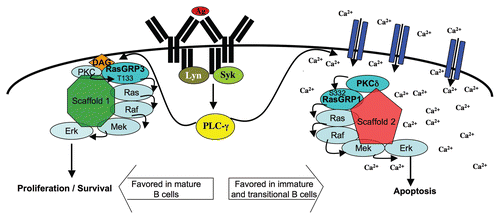
Extra View to: Limnander A, Depeille P, Freedman TS, Liou J, Leitges M, Kurosaki T, et al. STIM1, PKCδ and RasGRP set a threshold for proapoptotic Erk signaling during B cell development. Nat Immunol 2011; 12:425 - 433; PMID: 21441934; http://dx.doi.org/10.1038/ni.2016
References
- Genot E, Cantrell DA. Ras regulation and function in lymphocytes. Curr Opin Immunol 2000; 12:289 - 294; PMID: 10781411; http://dx.doi.org/10.1016/S0952-7915(00)00089-3
- Kolch W. Coordinating ERK/MAPK signalling through scaffolds and inhibitors. Nat Rev Mol Cell Biol 2005; 6:827 - 837; PMID: 16227978; http://dx.doi.org/10.1038/nrm1743
- Murphy LO, Blenis J. MAPK signal specificity: the right place at the right time. Trends Biochem Sci 2006; 31:268 - 275; PMID: 16603362; http://dx.doi.org/10.1016/j.tibs.2006.03.009
- Downward J, Graves JD, Warne PH, Rayter S, Cantrell DA. Stimulation of p21ras upon T-cell activation. Nature 1990; 346:719 - 723; PMID: 2201921; http://dx.doi.org/10.1038/346719a0
- Roose J, Weiss A. T cells: getting a GRP on Ras. Nat Immunol 2000; 1:275 - 276; PMID: 11017094; http://dx.doi.org/10.1038/79713
- Aiba Y, Oh-hora M, Kiyonaka S, Kimura Y, Hijikata A, Mori Y, et al. Activation of RasGRP3 by phosphorylation of Thr-133 is required for B cell receptormediated Ras activation. Proc Natl Acad Sci USA 2004; 101:16612 - 16617; PMID: 15545601; http://dx.doi.org/10.1073/pnas.0407468101
- Oh-hora M, Johmura S, Hashimoto A, Hikida M, Kurosaki T. Requirement for Ras guanine nucleotide releasing protein 3 in coupling phospholipase C-gamma2 to Ras in B cell receptor signaling. J Exp Med 2003; 198:1841 - 1851; PMID: 14676298; http://dx.doi.org/10.1084/jem.20031547
- Zheng Y, Liu H, Coughlin J, Zheng J, Li L, Stone JC. Phosphorylation of RasGRP3 on threonine 133 provides a mechanistic link between PKC and Ras signaling systems in B cells. Blood 2005; 105:3648 - 3654; PMID: 15657177; http://dx.doi.org/10.1182/blood-2004-10-3916
- Margarit SM, Sondermann H, Hall BE, Nagar B, Hoelz A, Pirruccello M, et al. Structural evidence for feedback activation by Ras.GTP of the Ras-specific nucleotide exchange factor SOS. Cell 2003; 112:685 - 695; PMID: 12628188; http://dx.doi.org/10.1016/S0092-8674(03)00149-1
- Das J, Ho M, Zikherman J, Govern C, Yang M, Weiss A, et al. Digital signaling and hysteresis characterize ras activation in lymphoid cells. Cell 2009; 136:337 - 351; PMID: 19167334; http://dx.doi.org/10.1016/j.cell.2008.11.051
- Roose JP, Mollenauer M, Ho M, Kurosaki T, Weiss A. Unusual interplay of two types of Ras activators, RasGRP and SOS, establishes sensitive and robust Ras activation in lymphocytes. Mol Cell Biol 2007; 27:2732 - 2745; PMID: 17283063; http://dx.doi.org/10.1128/MCB.01882-06
- Cullen PJ, Lockyer PJ. Integration of calcium and Ras signalling. Nat Rev Mol Cell Biol 2002; 3:339 - 348; PMID: 11988768; http://dx.doi.org/10.1038/nrm808
- Liou J, Kim ML, Heo WD, Jones JT, Myers JW, Ferrell JE Jr, et al. STIM is a Ca2+ sensor essential for Ca2+-store-depletion-triggered Ca2+ influx. Curr Biol 2005; 15:1235 - 1241; PMID: 16005298; http://dx.doi.org/10.1016/j.cub.2005.05.055
- Roos J, DiGregorio PJ, Yeromin AV, Ohlsen K, Lioudyno M, Zhang S, et al. STIM1, an essential and conserved component of store-operated Ca2+ channel function. J Cell Biol 2005; 169:435 - 445; PMID: 15866891; http://dx.doi.org/10.1083/jcb.200502019
- Zhang SL, Yu Y, Roos J, Kozak JA, Deerinck TJ, Ellisman MH, et al. STIM1 is a Ca2+ sensor that activates CRAC channels and migrates from the Ca2+ store to the plasma membrane. Nature 2005; 437:902 - 905; PMID: 16208375; http://dx.doi.org/10.1038/nature04147
- Baba Y, Hayashi K, Fujii Y, Mizushima A, Watarai H, Wakamori M, et al. Coupling of STIM1 to store-operated Ca2+ entry through its constitutive and inducible movement in the endoplasmic reticulum. Proc Natl Acad Sci USA 2006; 103:16704 - 16709; PMID: 17075073; http://dx.doi.org/10.1073/pnas.0608358103
- Luik RM, Wu MM, Buchanan J, Lewis RS. The elementary unit of store-operated Ca2+ entry: local activation of CRAC channels by STIM1 at ER-plasma membrane junctions. J Cell Biol 2006; 174:815 - 825; PMID: 16966423; http://dx.doi.org/10.1083/jcb.200604015
- Wu MM, Buchanan J, Luik RM, Lewis RS. Ca2+ store depletion causes STIM1 to accumulate in ER regions closely associated with the plasma membrane. J Cell Biol 2006; 174:803 - 813; PMID: 16966422; http://dx.doi.org/10.1083/jcb.200604014
- Spassova MA, Soboloff J, He LP, Xu W, Dziadek MA, Gill DL. STIM1 has a plasma membrane role in the activation of store-operated Ca(2+) channels. Proc Natl Acad Sci USA 2006; 103:4040 - 4045; PMID: 16537481; http://dx.doi.org/10.1073/pnas.0510050103
- Hauser CT, Tsien RY. A hexahistidine-Zn2+-dye label reveals STIM1 surface exposure. Proc Natl Acad Sci USA 2007; 104:3693 - 3697; PMID: 17360414; http://dx.doi.org/10.1073/pnas.0611713104
- Liou J, Fivaz M, Inoue T, Meyer T. Live-cell imaging reveals sequential oligomerization and local plasma membrane targeting of stromal interaction molecule 1 after Ca2+ store depletion. Proc Natl Acad Sci USA 2007; 104:9301 - 9306; PMID: 17517596; http://dx.doi.org/10.1073/pnas.0702866104
- Park CY, Hoover PJ, Mullins FM, Bachhawat P, Covington ED, Raunser S, et al. STIM1 clusters and activates CRAC channels via direct binding of a cytosolic domain to Orai1. Cell 2009; 136:876 - 890; PMID: 19249086; http://dx.doi.org/10.1016/j.cell.2009.02.014
- Limnander A, Depeille P, Freedman TS, Liou J, Leitges M, Kurosaki T, et al. STIM1, PKCdelta and RasGRP set a threshold for proapoptotic Erk signaling during B cell development. Nat Immunol 2011; 12:425 - 433; PMID: 21441934; http://dx.doi.org/10.1038/ni.2016
- Roose JP, Mollenauer M, Gupta VA, Stone J, Weiss A. A diacylglycerol-protein kinase C-RasGRP1 pathway directs Ras activation upon antigen receptor stimulation of T cells. Mol Cell Biol 2005; 25:4426 - 4441; PMID: 15899849; http://dx.doi.org/10.1128/MCB.25.11.4426-41.2005
- Guilbault B, Kay RJ. RasGRP1 sensitizes an immature B cell line to antigen receptor-induced apoptosis. J Biol Chem 2004; 279:19523 - 19530; PMID: 14970203; http://dx.doi.org/10.1074/jbc.M314273200
- Benschop RJ, Brandl E, Chan AC, Cambier JC. Unique signaling properties of B cell antigen receptor in mature and immature B cells: implications for tolerance and activation. J Immunol 2001; 167:4172 - 4179; PMID: 11591737
- Gross AJ, Lyandres JR, Panigrahi AK, Prak ET, DeFranco AL. Developmental acquisition of the Lyn-CD22-SHP-1 inhibitory pathway promotes B cell tolerance. J Immunol 2009; 182:5382 - 5392; PMID: 19380785; http://dx.doi.org/10.4049/jimmunol.0803941
- Hoek KL, Antony P, Lowe J, Shinners N, Sarmah B, Wente SR, et al. Transitional B cell fate is associated with developmental stage-specific regulation of diacylglycerol and calcium signaling upon B cell receptor engagement. J Immunol 2006; 177:5405 - 5413; PMID: 17015726
- King LB, Norvell A, Monroe JG. Antigen receptor-induced signal transduction imbalances associated with the negative selection of immature B cells. J Immunol 1999; 162:2655 - 2662; PMID: 10072508
- Perez de Castro I, Bivona TG, Philips MR, Pellicer A. Ras activation in Jurkat T cells following low-grade stimulation of the T-cell receptor is specific to N-Ras and occurs only on the Golgi apparatus. Mol Cell Biol 2004; 24:3485 - 3496; PMID: 15060167; http://dx.doi.org/10.1128/MCB.24.8.3485-96.2004
- Delgoffe GM, Pollizzi KN, Waickman AT, Heikamp E, Meyers DJ, Horton MR, et al. The kinase mTOR regulates the differentiation of helper T cells through the selective activation of signaling by mTORC1 and mTORC2. Nat Immunol 2011; 12:295 - 303; PMID: 21358638; http://dx.doi.org/10.1038/ni.2005
- Kim DH, Sarbassov DD, Ali SM, King JE, Latek RR, Erdjument-Bromage H, et al. mTOR interacts with raptor to form a nutrient-sensitive complex that signals to the cell growth machinery. Cell 2002; 110:163 - 175; PMID: 12150925; http://dx.doi.org/10.1016/S0092-8674(02)00808-5
- Sarbassov DD, Ali SM, Kim DH, Guertin DA, Latek RR, Erdjument-Bromage H, et al. Rictor, a novel binding partner of mTOR, defines a rapamycin-insensitive and raptor-independent pathway that regulates the cytoskeleton. Curr Biol 2004; 14:1296 - 1302; PMID: 15268862; http://dx.doi.org/10.1016/j.cub.2004.06.054
- Therrien M, Wong AM, Kwan E, Rubin GM. Functional analysis of CNK in RAS signaling. Proc Natl Acad Sci USA 1999; 96:13259 - 13263; PMID: 10557308; http://dx.doi.org/10.1073/pnas.96.23.13259
- Therrien M, Michaud NR, Rubin GM, Morrison DK. KSR modulates signal propagation within the MAPK cascade. Genes Dev 1996; 10:2684 - 2695; PMID: 8946910; http://dx.doi.org/10.1101/gad.10.21.2684
- Therrien M, Wong AM, Rubin GM. CNK, a RAF-binding multidomain protein required for RAS signaling. Cell 1998; 95:343 - 353; PMID: 9814705; http://dx.doi.org/10.1016/S0092-8674(00)81766-3
- Schaeffer HJ, Catling AD, Eblen ST, Collier LS, Krauss A, Weber MJ. MP1: a MEK binding partner that enhances enzymatic activation of the MAP kinase cascade. Science 1998; 281:1668 - 1671; PMID: 9733512; http://dx.doi.org/10.1126/science.281.5383.1668
- Torii S, Kusakabe M, Yamamoto T, Maekawa M, Nishida E. Sef is a spatial regulator for Ras/MAP kinase signaling. Dev Cell 2004; 7:33 - 44; PMID: 15239952; http://dx.doi.org/10.1016/j.devcel.2004.05.019
- Bivona TG, Perez De Castro I, Ahearn IM, Grana TM, Chiu VK, Lockyer PJ, et al. Phospholipase Cgamma activates Ras on the Golgi apparatus by means of RasGRP1. Nature 2003; 424:694 - 698; PMID: 12845332; http://dx.doi.org/10.1038/nature01806
- Mor A, Campi G, Du G, Zheng Y, Foster DA, Dustin ML, et al. The lymphocyte function-associated antigen-1 receptor costimulates plasma membrane Ras via phospholipase D2. Nat Cell Biol 2007; 9:713 - 719; PMID: 17486117; http://dx.doi.org/10.1038/ncb1592
- Tazmini G, Beaulieu N, Woo A, Zahedi B, Goulding RE, Kay RJ. Membrane localization of RasGRP1 is controlled by an EF-hand, and by the GEF domain. Biochim Biophys Acta 2009; 1793:447 - 461; PMID: 19168098
- Spitaler M, Emslie E, Wood CD, Cantrell D. Diacylglycerol and protein kinase D localization during T lymphocyte activation. Immunity 2006; 24:535 - 546; PMID: 16713972; http://dx.doi.org/10.1016/j.immuni.2006.02.013
- Quann EJ, Merino E, Furuta T, Huse M. Localized diacylglycerol drives the polarization of the microtubule-organizing center in T cells. Nat Immunol 2009; 10:627 - 635; PMID: 19430478; http://dx.doi.org/10.1038/ni.1734
- Barr VA, Bernot KM, Shaffer MH, Burkhardt JK, Samelson LE. Formation of STIM and Orai complexes: puncta and distal caps. Immunol Rev 2009; 231:148 - 159; PMID: 19754895; http://dx.doi.org/10.1111/j.1600-065X.2009.00812.x
- Barr VA, Bernot KM, Srikanth S, Gwack Y, Balagopalan L, Regan CK, et al. Dynamic movement of the calcium sensor STIM1 and the calcium channel Orai1 in activated T-cells: puncta and distal caps. Mol Biol Cell 2008; 19:2802 - 2817; PMID: 18448669; http://dx.doi.org/10.1091/mbc.E08-02-0146
- Daniels MA, Teixeiro E, Gill J, Hausmann B, Roubaty D, Holmberg K, et al. Thymic selection threshold defined by compartmentalization of Ras/MAPK signalling. Nature 2006; 444:724 - 729; PMID: 17086201; http://dx.doi.org/10.1038/nature05269
- Patterson KI, Brummer T, O'Brien PM, Daly RJ. Dual-specificity phosphatases: critical regulators with diverse cellular targets. Biochem J 2009; 418:475 - 489; PMID: 19228121
- Chi H, Barry SP, Roth RJ, Wu JJ, Jones EA, Bennett AM, et al. Dynamic regulation of pro- and anti-inflammatory cytokines by MAPK phosphatase 1 (MKP-1) in innate immune responses. Proc Natl Acad Sci USA 2006; 103:2274 - 2279; PMID: 16461893; http://dx.doi.org/10.1073/pnas.0510965103
- Hammer M, Mages J, Dietrich H, Servatius A, Howells N, Cato AC, et al. Dual specificity phosphatase 1 (DUSP1) regulates a subset of LPS-induced genes and protects mice from lethal endotoxin shock. J Exp Med 2006; 203:15 - 20; PMID: 16380512; http://dx.doi.org/10.1084/jem.20051753
- Jeffrey KL, Brummer T, Rolph MS, Liu SM, Callejas NA, Grumont RJ, et al. Positive regulation of immune cell function and inflammatory responses by phosphatase PAC-1. Nat Immunol 2006; 7:274 - 283; PMID: 16474395; http://dx.doi.org/10.1038/ni1310
- Lang R, Hammer M, Mages J. DUSP meet immunology: dual specificity MAPK phosphatases in control of the inflammatory response. J Immunol 2006; 177:7497 - 7504; PMID: 17114416
- Salojin KV, Owusu IB, Millerchip KA, Potter M, Platt KA, Oravecz T. Essential role of MAPK phosphatase-1 in the negative control of innate immune responses. J Immunol 2006; 176:1899 - 1907; PMID: 16424221
- Zhang Y, Blattman JN, Kennedy NJ, Duong J, Nguyen T, Wang Y, et al. Regulation of innate and adaptive immune responses by MAP kinase phosphatase 5. Nature 2004; 430:793 - 797; PMID: 15306813; http://dx.doi.org/10.1038/nature02764
- Zhao Q, Wang X, Nelin LD, Yao Y, Matta R, Manson ME, et al. MAP kinase phosphatase 1 controls innate immune responses and suppresses endotoxic shock. J Exp Med 2006; 203:131 - 140; PMID: 16380513; http://dx.doi.org/10.1084/jem.20051794
- Mecklenbräuker I, Saijo K, Zheng NY, Leitges M, Tarakhovsky A. Protein kinase Cdelta controls self-antigen-induced B-cell tolerance. Nature 2002; 416:860 - 865; PMID: 11976686; http://dx.doi.org/10.1038/416860a
- Coughlin JJ, Stang SL, Dower NA, Stone JC. RasGRP1 and RasGRP3 regulate B cell proliferation by facilitating B cell receptor-Ras signaling. J Immunol 2005; 175:7179 - 7184; PMID: 16301621
- Dower NA, Stang SL, Bottorff DA, Ebinu JO, Dickie P, Ostergaard HL, et al. RasGRP is essential for mouse thymocyte differentiation and TCR signaling. Nat Immunol 2000; 1:317 - 321; PMID: 11017103; http://dx.doi.org/10.1038/80799
- Layer K, Lin G, Nencioni A, Hu W, Schmucker A, Antov AN, et al. Autoimmunity as the consequence of a spontaneous mutation in Rasgrp1. Immunity 2003; 19:243 - 255; PMID: 12932358; http://dx.doi.org/10.1016/S1074-7613(03)00209-7
- Rebhun JF, Castro AF, Quilliam LA. Identification of guanine nucleotide exchange factors (GEFs) for the Rap1 GTPase. Regulation of MR-GEF by M-Ras-GTP interaction. J Biol Chem 2000; 275:34901 - 34908; PMID: 10934204; http://dx.doi.org/10.1074/jbc.M005327200
- Stope MB, Vom Dorp F, Szatkowski D, Bohm A, Keiper M, Nolte J, et al. Rap2B-dependent stimulation of phospholipase C-epsilon by epidermal growth factor receptor mediated by c-Src phosphorylation of RasGRP3. Mol Cell Biol 2004; 24:4664 - 4676; PMID: 15143162; http://dx.doi.org/10.1128/MCB.24.11.4664-76.2004
- Cagnol S, Chambard JC. ERK and cell death: mechanisms of ERK-induced cell death-apoptosis, autophagy and senescence. FEBS J 2010; 277:2 - 21; PMID: 19843174; http://dx.doi.org/10.1111/j.1742-4658.2009.07366.x
- Cheung EC, Slack RS. Emerging role for ERK as a key regulator of neuronal apoptosis. Sci STKE 2004; 2004:45
- Subramaniam S, Zirrgiebel U, von Bohlen, Und Halbach O, Strelau J, Laliberte C, et al. ERK activation promotes neuronal degeneration predominantly through plasma membrane damage and independently of caspase-3. J Cell Biol 2004; 165:357 - 369; PMID: 15123736; http://dx.doi.org/10.1083/jcb.200403028
- Fam NP, Fan WT, Wang Z, Zhang LJ, Chen H, Moran MF. Cloning and characterization of Ras-GRF2, a novel guanine nucleotide exchange factor for Ras. Mol Cell Biol 1997; 17:1396 - 1406; PMID: 9032266
- Farnsworth CL, Freshney NW, Rosen LB, Ghosh A, Greenberg ME, Feig LA. Calcium activation of Ras mediated by neuronal exchange factor Ras-GRF. Nature 1995; 376:524 - 527; PMID: 7637786; http://dx.doi.org/10.1038/376524a0