Abstract
Drosophila has been the invertebrate model organism of choice for the study of innate immune responses during the past few decades. Many Drosophila–microbe interaction studies have helped to define innate immunity pathways, and significant effort has been made lately to decipher mechanisms of microbial pathogenesis. Here we catalog 68 bacterial, fungal, and viral species studied in flies, 43 of which are relevant to human health. We discuss studies of human pathogens in flies revealing not only the elicitation and avoidance of immune response but also mechanisms of tolerance, host tissue homeostasis, regeneration, and predisposition to cancer. Prominent among those is the emerging pattern of intestinal regeneration as a defense response induced by pathogenic and innocuous bacteria. Immunopathology mechanisms and many microbial virulence factors have been elucidated, but their relevance to human health conventionally necessitates validation in mammalian models of infection.
Introduction
We interact with microorganisms throughout our lives. Some microbes are beneficial for the human body, while others can be pathogenic. The skin and the mucosal surfaces are the primary sites of host–microbe interaction.Citation1,Citation2 The intestinal mucosa is one of the largest interfaces of the human body and is heavily colonized by numerous bacterial species,Citation1,Citation3 some of which protect the host by modulating immune responses to fight pathogens, while providing tolerance to non-pathogens.Citation3 Beneficial bacteria adhesion to the intestinal mucosa may prevent the attachment and compete for space and food with suspected pathogens, thus preventing the colonization and invasion of pathogenic bacteria.Citation4
Nevertheless, many bacteria, viruses, and fungi can cause dangerous infections especially under conditions that favor their growth and survival. Pneumonia and diarrhea together are the third cause of death among children under 5 years of age worldwide, accounting for 2 million deaths per year.Citation5 Food- and waterborne pathogens can cause acute or chronic infections to most individuals, while immunocompromised individuals due to skin burn, cancer treatment, or HIV infection, are highly susceptible to opportunistic pathogens. Also genetically predisposed individuals are more susceptible to infection, because conditions such the inflammatory bowel disease and cystic fibrosis, can alter the microbiota composition and host defense promoting the colonization and invasion of pathogenic bacteria.
Here we list 68 microbial species that have been studied in flies () and review some of the 43 human microbes that have been modeled in Drosophila melanogaster (), describing the lessons as well as the shortcomings in studying human microbes in flies. It appears that many human infectious agents can be effectively studied in Drosophila, in cases where the pathologies exhibited in flies reflect conserved aspects of human disease or physiology.
Table 1. Microbes studied in Drosophila melanogaster
Figure 1. Human microbes extensively studied in Drosophila. Human microbes studied during their interaction with Drosophila in wound (thoracic pricking), systemic (hemolymph injection), or intestinal (feeding) infection assays. While depicted in adult flies, many hemolymph and intestinal infections are studied in larvae. In addition, microbial virulence factors have been expressed in live Drosophila tissues or Drosophila tissue culture cells have been studied upon infection with various human microbes.
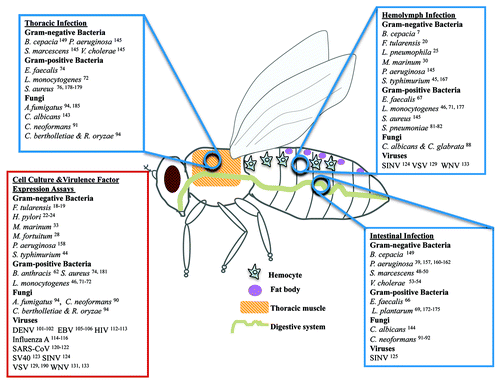
Due to space limitations we do not describe the significant work done in Drosophila with Pseudomonas entomophila, Erwinia carotovora, Beauveria bassiana, Drosophila viruses, and other non-human pathogens (), focusing instead on studies aiming to explore in depth human microbial pathogenesis.
D. melanogaster, a Simple Host for Studying Microbial Diseases
D. melanogaster is a simple model organism for studying diseases caused by a great number of bacteria, fungi, and viruses. It has a short generation time simpler but analogous organ structure compared with mammals, and can be expanded at low cost.Citation2,Citation134 Despite simplicity, many Drosophila defense mechanisms are highly conserved in mammals.Citation135 NFκB, JNK, and JAK-STAT signaling pathways are critical regulators of the immune responses in both flies and mammals.Citation135 Similarly to mammals one of the first lines of the Drosophila defense against microbes is mediated by barrier epithelia and their responses.Citation136 Infected tissue homeostasis and regeneration are also part of the defense response.Citation39,Citation137,Citation138
Systemically, Drosophila fights many microbes primarily via the production of conserved antimicrobial peptides by the fat body (an analog of the mammalian liver), by the deposition of melanin that traps microbes and via phagocytosis by the plasmatocytes, which are analogous to the mammalian macrophages.Citation135 Bacteria, fungi, and viruses induce Toll and Imd, the two highly conserved NFκB pathways of Drosophila, as well as the highly conserved in mammals' JAK-STAT pathway.Citation135 Viruses that infect Drosophila may also induce RNA interference and autophagy.Citation139 The many studies that have established the paradigm of innate immunity in flies provide one framework in which to analyze host-pathogen interactions with the added dimensions of specific virulence factor, regeneration and tolerance mechanisms.Citation135,Citation140-Citation142
Infections in flies enable the study of infected tissues and organs without the ethical concerns that accompany mammalian hosts. Moreover, flies are amenable to anti-infective treatments and a great number of genetic tools based on the Drosophila genome are now available.Citation2,Citation134 Prominent among those is the ability to conditionally inactivate every single gene using fly strains expressing gene specific RNAi constructs.Citation138
During the last years flies played a critical role in identifying virulence factors of various opportunistic pathogens.Citation16 Some microbes use to a large extent similar virulence mechanisms to infect flies and mammals, and many virulence factors effective against mammals are also responsible for pathogenicity in flies.Citation143,Citation144 As a result, a big array of microbes has been studied in fruit flies, including many important human-related microbes (). We discuss the most extensively studied of the human pathogens in the following sections ().
Modeling Human Microbial Diseases in D. melanogaster
Human wound, systemic, and intestinal infections can be easily recapitulated in Drosophila by pricking, injecting, and feeding flies, respectively, with the pathogens of interestCitation145 (). The method of thoracic or abdominal needle pricking involves the use of a metal needle dipped into a bacterial suspension.Citation145 If flies are pricked in the thorax, wounding is primarily imposed to the thoracic cuticular epithelium and the underlying muscle.Citation137,Citation145,Citation146 Upon inoculation at the wound site, the bacteria may proliferate locally and disseminate throughout the body of the fly, leading to both local and systemic tissue damage and immune response.Citation137,Citation145,Citation146 A second method is the “injector pumping” that produces primarily systemic inoculation by distributing microbes throughout the fly body.Citation145 Using this method adult flies or larvae can be easily injected with precise doses of the microbes of interest directly into the hemolymph, bypassing the wound site barrier.
Using Drosophila feeding assays to mimic mammalian intestinal infection various microbes can be introduced into the fly intestine.Citation145 This method provides the advantage, of the facile assessment of intestinal regeneration orchestrated by evolutionary conserved signaling pathways, including the JNK, Hippo, EGFR, and JAK-STAT signaling pathways.Citation147 Moreover, microbial genes can be individually studied by being expressed as transgenes in flies. This is a valuable technique necessary for studying human microbes that are unable to establish an infection in flies, expressing nonetheless virulence factors potentially harmful to both flies and mammals. Finally, infection of Drosophila hemocyte-like cell lines provides a means for high-throughput studies of microbe–immune cell interactions.
Many human bacterial, fungal, and viral pathogens have been studied in Drosophila. Some of them can be highly pathogenic in flies, while others are relatively harmless.Citation134,Citation148 In the following sections we focus on Drosophila studies describing mechanisms of pathogenesis as potential targets against human pathogens.
Lessons from Drosophila Studies of Human Pathogens
Gram-negative and gram-positive bacteria, fungi, and viruses are grouped in separate subsections for systematic purposes.
Gram-negative bacteria
Burkholderia cepacia
Colonization with bacterial species of the B. cepacia complex (Bcc) is associated with serious respiratory infections in immunocompromised patients, such as cystic fibrosis and wounded individuals.Citation6 B. cepacia complex does not appear to kill Drosophila in feeding assays.Citation149 However, in wound infection (pricking) assays it is highly lethal and appropriate for screening Bcc mutants for virulence attenuation.Citation149 Mutant flies for eiger, the Drosophila TNFα homolog, die faster than wild type flies when injected with B. cepacia.Citation7 On the contrary, there is no increase in the mortality of flies mutant for melanization, although melanization-deficient flies bear on average more bacteria.Citation8
Conclusion: TNFα pathway might act against Bcc wound infections in humans. Interestingly, melanization seems to reduce the ability of bacteria to grow (increases resistance) in flies, but also reduces the tolerance of flies to Bcc infection, presumably because melanization induces immunopathology.
Francisella tularensis
F. tularensis is the causative agent of tularemia which, is a zoonotic disease affecting many hosts including humans.Citation17 Most strains require biosafety level 3 handling due to the potential aerosol transmission. Flies and other arthropods, transmit F. tularensis to small mammals, such as rabbits.Citation150D. melanogaster has been established as a good arthropod model for studying tularemia.Citation150,Citation151 For example, out of 394 mutants assessed for defects in intracellular proliferation, 135 were defective is both Drosophila S2 cells and human macrophages.Citation18 Two virulence factors conserved in mammals, the PI4 kinase PI4KCA and the ubiquitin hydrolase USP22, are required for proliferation within the cytosol while a third, the ubiquitin ligase CDC27, is important for the escape of F. tularensis into the cytosol of the host cells.Citation19 In addition, 249 mutant strains of F. tularensis subsp. novicida, potentially relevant to mammalian cell pathogenesis, were tested in adult flies.Citation17 This subspecies is attenuated in virulence in mammals yet lethal to flies allowing experimentation in a reduced biosafety level environment. Twenty percent of the genes tested in mice also contributed to adult fly pathogenesis.Citation17 In a similar Transponson Site Hybridization (TraSH) screen the transcription factor oxyR and the DNA repair proteins uvrB, recB, and ruvC were found to contribute to virulence.Citation20 These virulence factors resist oxidative stress and counteract the melanization that Drosophila uses as an immune response to infection. On the other hand, F. tularensis subsp. novicida is very sensitive to the antimicrobial peptides produced by the Imd-regulated immune response of the infected flies,Citation20 despite the ability of Francisella lipid A and Kdo core but not of O-antigen to confer resistance against Drosophila antimicrobial peptides.Citation21
Conclusion: F. tularensis uses common and many virulence factors to proliferate within Drosophila and mouse cells. Nevertheless, the factors required for virulence in adult flies might be different from those inferred from in vitro or mouse studies, and further studies are necessary to validate their significance.
Helicobacter pylori
Helicobacter pylori is a causative agent of peptic ulcers, atrophic gastridis and gastric carcinoma.Citation152 Virulent strains can inject the CagA effector protein into the host cells.Citation22 Expression of this virulence factor in Drosophila, promotes apoptosis or tumorigenesis through the activation of the JNK signaling and the activation of receptor tyrosine kinase (RTK) pathway genes, such as Gab adapters.Citation22,Citation23 Similarly, Drosophila transgenic models show that CagA activates myosin regulatory light chain (MLC), leading to the rapid disruption of epithelial integrity.Citation24
Conclusion: JNK, RTKs, and MLC are activated in response to CagA in a tissue-dependent manner. Thus orthotopic activation of CagA in Drosophila stomach like tissues, for instance, midgut copper cells, might be recommended to validate these mechanisms of action.
Legionella pneumophila
L. pneumophila can cause severe pneumonia in humans called Legionnaire disease.Citation25 The bacteria direct the formation of their replication vacuole by injecting many effector proteins into the host cells via the Dot/Icm type IV secretion system,Citation153 a mechanism that is conserved in Drosophila.Citation25 In an RNA interference screen using Drosophila cells Legionella protein complex Cdc48/p97 was found necessary for the subcellular localization of bacterial effector proteins into the host cells.Citation153 Another screen, which combined bacterial mutagenesis with Drosophila cell RNA interference, uncovered the role in pathogenesis of bacterial effectors, previously considered as redundant for bacterial replication inside host cells.Citation154
Conclusion: The Dot/Icm system and the pertinent secreted effectors of L. pneumophila are pivotal for pathogenicity in both flies and mammals. Importantly, some bacterial effectors are required for full infectivity in Drosophila cells only in specific host genetic backgrounds.
Mycobacterium marinum and Mycobacterium fortuitum
M. marinum, a close relative to M. tuberculosis, causes human skin infections that may spread deeper, resulting in arthritis or osteomyelitis.Citation29 Injection of only 5 colony forming units of M. marinum suffices to kill 50% of flies.Citation30 Infected flies undergo a “wasting” process characterized by hyperglycemia and the loss of metabolic stores, similarly to what happens in humans. This process is partially induced by the transcription factor FOXO, which nevertheless does not affect bacterial load.Citation155 Thus FOXO controls fly tolerance to M. marinum infection. In addition, infection with M. marinum does not induce the expression of antimicrobial peptides by Drosophila, as it is customary during other bacterial infections.Citation30 This means that flies—similarly to human lung cells infected with M. tuberculosis—fail to recognize and clear the bacteria or that bacteria actively suppress immune responses.Citation30 Strikingly, host cell autophagy activation is necessary process for successful antimycobacterial drug action in infected flies and mammalian macrophages.Citation31 And the highly conserved ubiquitin ligase parkin contributes to host defense against Mycobacteria and other intracellular pathogens in flies and mice.Citation32 Moreover, lysosomal enzyme β-hexosaminidase is sufficient to control M. marinum growth in S2 cells and mouse macrophages.Citation33 Furthermore, fly cell infection with M. fortuitum, which is also pathogenic to humans, is a useful model for the identification of conserved host factors, for example the CD36 family gene peste, that are required for M. fortuitum recognition and uptake by fly and human cells.Citation28
Conclusion: Innate immunity and autophagy stimulants and anabolic and antimycobacterial drugs can be tested in flies against M. marinum and other mycobacterial infections.
Pseudomonas aeruginosa
P. aeruginosa is a major agent of lethal infections in cystic and burn wound patients.Citation38 Many of its virulence factors show exceptional conservation by contributing to pathogenesis in flies and mice.Citation156P. aeruginosa redox-active phenazine pyocyanin induces Drosophila intestinal stem cells overproliferation as a defense response to infection, which nevertheless may lead to tumor formation in genetically predisposed flies.Citation39 In an oral infection model, in which the bacteria spread systemically to kill the fly, the quorum sensing regulator RhlR is required for full virulence.Citation157 In a wound infection model, transgenic flies expressing Paraoxonase 1 (PON1) are more resistant to P. aeruginosa wound infection, because PON1 can neutralize the quorum sensing regulator LasI.Citation158
Interestingly, P. aeruginosa may interact with avirulent or beneficial bacteria in the fly alimentary canal to enhance its pathogenicity against Drosophila.Citation159 In the fly gut P. aeruginosa senses gram-positive bacteria peptidoglycan to induce its infectivity and virulence against eukaryotic and prokaryotic cells.Citation160 In addition, it may suppress the NFκB and JNK mediated innate immune response during wound infection but it may induce JNK signaling during intestinal infection to promote intestinal regeneration or tumor cell growth and dissemination.Citation38,Citation161,Citation162 P. aeruginosa actively limits the expression of Drosophila skeletal muscle genes at the site of wound infection and the expression of glutathione-S-transferase S1 (GstS1) in flies, a JNK-mediated response that is also conserved in mouse wound infections.Citation137 This wound site response is a resistance mechanism that inhibits bacterial growth and dissemination.Citation137 Interestingly, low expression levels of GstA4, the GstS1 homolog in mice and humans, proved later on to be a factor of susceptibility to wound infection in mice and humans.Citation163
Recently, formation of P. aeruginosa biofilms was noticed upon infection in the Drosophila crop.Citation40 In this model biofilm formation correlates negatively with the virulence of the different strains. That is, mutants with decreased biofilm formation are significantly more virulent than hyperbiofilm strains, because the former disseminate more easily to the fly hemolymph and immune response is decreased, facilitating the progression of infection.Citation40
The P. aeruginosa–fly model has still many aspects of infection to teach us because the Drosophila genotypic variation affects bacterial load and survival post-infection independently, suggesting that there are mechanisms of tolerance to infection which have not been studied.Citation164 Furthermore, evolutionary selection for traits that allow better survival of Drosophila to P. aruginosa infection reveal a correlation between organismal development and host defense, plus the importance of genes with dual involvement in developmental and immune pathways.Citation165 Thus pleiotropy might be a mechanism for the observed correlation.
Conclusion: P. aeruginosa modulates the local host defense responses in a tissue-dependent manner and may contribute to epithelial inflammation and cancer in genetically predisposed organisms. Moreover, Drosophila studies show that there is an inverse correlation between biofilm formation and acute virulence and the ability of other microbial species to enhance P. aeruginosa virulence.
Salmonella Typhimurium
S. Typhimurium is highly virulent due to its many virulence factors.Citation43 It can cause inflammatory diarrhea (gastroenteritis) in calves and humans and a typhoid-like disease in mice.Citation43 AvrA is among the effector proteins that S. Typhimurium secretes into the mammalian cells. Expression of AvrA in Drosophila suppresses apoptosis by inhibiting the JNK pathway, a conserved mechanism used by S. Typhimurium to restrict its elimination.Citation44 Consistently, AvrA was found suppressing innate immune response and inflammation in the mouse intestine.Citation166
When injected into the hemocoel of Drosophila, S. Typhimurium is lethalCitation167 and similarly to most lethal infections it induces anorexia in flies. Anorexia in turn increases the fly’s tolerance to S. Typhimurium infection.Citation168 Similarly, during S. Typhimurium infection, eiger, the only known TNF family member in the fly is required in the fat body to reduce the bacterial load via melanization.Citation45 Eiger mutant flies nevertheless survive the infection better because they are anorexic.Citation45 However, the relationship between diet restriction and host defense is not universal and should be evaluated on a pathogen-specific basis. Furthermore, the Drosophila p38 mitogen-activated protein (MAP) kinase (Dmp38b), a homolog of the mammalian p38 MAP kinase family, protects the host against S. Typhimurium, because it increases the phagocytic capacity of hemocytes.Citation46
Conclusion: The JNK and the p38 MAP kinases may drive humoral and the cellular innate immune response respectively against S. Typhimurium, while the secreted effector protein AvrA may inhibit JNK to promote infection. Nevertheless, TNF pathway inhibition induces anorexia, which seems to contribute to host tolerance.
Serratia marcescens
S. marcescens is an entomopathogenic bacterium able to infect many hosts, including humans.Citation48 It is a significant cause of hospital-acquired infections with high mortality rates, especially in neonatal intensive care units as it may cause pneumonia, meningitis or other serious infections.Citation47Drosophila intestinal infection with S. marcescens causes a local immune response but bacteria can also traverse the intestinal epithelium and gain access to the host’s body cavity.Citation48 A genome-wide in vivo Drosophila RNAi screen using S. marcescens infected flies identified the JAK-STAT pathway as an important inducer of intestinal regeneration and a negative regulator of host defense to intestinal infection.Citation49 On the contrary, Imd/NFκB signaling activation upon infection induces host defense.Citation48,Citation49 Moreover, bacteria that escape to the hemolymph are contained by phagocytes.Citation48 Ingested bacteria that translocate to the hemolymph are detected by the systemic humoral immune system only when phagocytosis is blocked.Citation48 Importantly, flies lacking the gene subdued, a member of the mammalian calcium-activated chloride channels-TMEM16 family, accumulate more bacteria and succumb faster than wild-type flies upon S. marcescens oral infection, indicating a role of this gene in the Drosophila resistance to infection.Citation50
Conclusion: S. marcescens may cause intestinal pathologies and concomitant lethality, in accordance to the propensity of bacteria to damage mammalian epithelia. While phagocytosis and NFκB pathway induction promotes host defense, JAK-STAT pathway-induced intestinal regeneration appears to exacerbate infection.
Vibrio cholerae
V. cholerae is the etiological agent of cholera, a life-threatening diarrheal disease. Humans are usually infected through ingestion of contaminated water, because this bacterium primarily exists in marine environments. V. cholerae polysaccharide (VPS)-dependent biofilm is highly activated upon entry into the arthropod intestine and is specifically required for colonization of the arthropod rectum.Citation169 Interestingly, intestinal infection of D. melanogaster with V. cholera mimics to a great extent the human disease cholera.Citation52
KerV, a virulence factor conserved among pathogenic Proteobacteria, contributes to V. cholerae pathogenesis in Drosophila.Citation61 Furthermore, mutations in the pro-apoptotic Eiger/TNF signaling pathway increase the susceptibility of the fly to V. cholerae infection, suggesting that this pathway promotes host defense against this bacterium.Citation170 V. cholerae inhibits intestinal regeneration in infected flies, but Imd/NFκB pathway and mustard mutants counteract this inhibition, maintain higher levels of intestinal stem cell division, and survive better during V. cholerae infection.Citation53 Cholera toxin-driven inhibition of Rab11/exocyst-mediated trafficking of host proteins induces junctional damage, weight loss, and dye leakage in the Drosophila gut and other pathologies conserved in human intestinal epithelial cells, and ligated mouse ileal loops.Citation54
Conclusion: Suppression of intestinal stem cell division is likely a virulence strategy of V. cholerae because accelerated epithelial regeneration may protect the host against V. cholerae. Also the barrier-disrupting effects of cholera toxin may act in parallel with Cl− secretion to drive the pathophysiology of cholera.
Gram-positive bacteria
Bacillus anthracis
Bacillus anthracis is the etiological agent of anthrax, and can infect many mammals, including humans.Citation62 There are three factors secreted by this bacterium which contribute to its high virulence: the lethal factor (LF), theoedema factor (EF), and the protective antigen (PA).Citation62 PA contributes to the entrance of LF and EF into the host cells.Citation62 Expression of LF and EF in Drosophila during development, cooperatively inhibit the last step of endocytosis, namely endocytic recycling, by blocking the Rab11/Sec15 exocyst.Citation62 The role of LF and EF in endocytosis proved to be conserved in a human cell line.Citation62 Another Bacillus anthracis-secreted factor the hemolytic/cytolytic protein anthrolysin O binds and kills mouse and human macrophage-like, but not Drosophila S2 cells, because flies contain mainly ergosteror instead of cholesterol in their cell membranes.Citation171
Conclusion: Endocytic recycling and cell membrane cholesterol are targets of B. anthracis toxins in flies and probably in humans.
Enterococcus faecalis
Enterococci, including E. faecalis, are commensal organisms of the gastrointestinal tract. Interestingly, E. faecalis appears to naturally colonize the Drosophila intestine and is the leading cause of many nosocomial infections. E. faecalis strains that express the virulence factor cytolysin are significantly more virulent to both flies and mammals.Citation66 Septic injury with E. faecalis activates phagocytosis in addition to the antimicrobial peptide production in Drosophila.Citation74E. faecalis phagocytosis is regulated by the receptor Eater and is critical for the Drosophila host defense.Citation74E. faecalis quorum regulatory system genes LrgAB and SprE, and bacteriocin EF1097 were found to contribute to infection toxicity in Drosophila.Citation67
Conclusion: E. faecalis shows exceptional similarities in natural colonization of Drosophila and humans, a property that places Drosophila in a suitable position to assess its quorum sensing factors that relate to pathogenicity.
Lactobacillus plantarum
L. plantarum is a gram-positive commensal bacterium in humans suggested to protect the intestinal epithelium barrier function.Citation68 Recent studies demonstrate that L. plantarum can colonize germ-free Drosophila larval gut and remains associated with it long after the initial colonization.Citation69 A mechanism used by L. plantarum to establish itself in the gut is the recognition by PGRP-LE and the subsequent lack of inhibition of the Imd/NFκB pathway.Citation172 On the contrary, PGRP-LE senses entomopathogenic Erwinia carotovora and induces the Imd/NFκB pathway to defend the host from infection.Citation172
Several L. plantarum strains stimulate larval development upon nutrient scarcity and adults emerge faster than in the germ-free flies.Citation69 Importantly, colonization with L. plantarum protects the fly from virulent P. aeruginosa and S. marcescens oral infection.Citation173 In addition, expression of human PON1, previously found to inhibit P. aeruginosa quorum sensing, is shown to increase L. plantarum colonization in the fly gut;Citation174 yet another mechanism to inhibit P. aeruginosa infection.
Interestingly, NADPH oxidase 1-dependent ROS generation and consequent cellular proliferation in intestinal stem cells are induced upon ingestion of L. rhamnosus and L. plantarum in mice and Drosophila respectively.Citation175 Although in disparate phylogenic clades, L. rhamnosus and L. plantarum seemingly have evolved the ability to induce cellular ROS and intestinal generation within their adapted host.
Conclusion: Unlike pathogenic bacteria, L. plantarum colonization is induced by PON1 and does not induce PGRP-LE mediated defense response. Due to its ability to naturally colonize, induce intestinal regeneration and facilitate larval development, L. plantarum studies in flies can be directly relevant to human health.
Listeria monocytogenes
L. monocytogenes, is an opportunistic anaerobic intracellular pathogen that causes listeriosis, which is presented by non-specific flu-like symptoms and gastroenteritis.Citation70 In a Drosophila cell culture RNAi screen many host factors were identified required for intracellular pathogenesis and factors that specifically affect access to the cytosol by L. monocytogenes.Citation176 Induction of autophagy in Drosophila requiring the autophagy-related factors Atg5 and Atg1 is crucial to prevent the intracellular growth of L. monocytogenes and promote host survival.Citation71Drosophila genes conferring tolerance to infection were found to be specific to the different stages of infection.Citation8 For example, p38 MAPK-dependent phagocytic encapsulation of bacteria resulted in enlarged phagocytes that trap L. monocytogenes conferring tolerance to infection.Citation46
L. monocytogenes virulence genes are expressed at 25 °C, and not only at temperatures higher than 30 °C as previously thought.Citation72 Moreover, similar bacterial genes, such as actA and prfA, are used in Drosophila and mammalian cells for the intracellular replication and cell to cell spreading of L. monocytogenes.Citation72 In addition, flies infected with L. monocytogenes exhibit a shift in their metabolism manifested primarily as changes in their lipid, carbohydrate, and amino acid levels.Citation177
Conclusion: Genetic screens in Drosophila identify host autophagy, phagocytosis, and bacterial factors required for resistance and tolerance to L. monocytogenes infection, as well as the metabolic changes in the host during infection.
Staphylococcus aureus
S. aureus has been characterized as a nosocomial pathogen, but can also infect healthy individuals.Citation75S. aureus infections can be life-threatening because they can cause pneumonia and necrotizing fasciitis.Citation75Drosophila is used for studying the virulence determinants of S. aureus strains,Citation178,Citation179 and the response to antibiotic treatment upon infection.Citation180Drosophila infection by S. aureus can be controlled by phagocytosis mediated by the Eater receptor.Citation74 Toll pathway recognizes peptidoglycan from many gram-positive bacteria and contributes to resistance against S. aureus.Citation181 Wound infection of Drosophila with S. aureus shows that d-alanylation of wall teichoic acid alters peptidoglycan recognition by the Toll innate immune pathwayCitation76 because d-alanylated wall teichoic acid binds covalently to peptidoglycan.Citation77,Citation78
Conclusion: Drosophila models of S. aureus infection show the interplay of peptidoglycan recognition and evasion of this recognition by d-alanylated wall teichoic acid bound to peptidoglycan.
Streptococcus pneumoniae
S. pneumoniae is a human pathogen that can cause serious pathologies, including community-acquired pneumonia and meningitis.Citation80 Flies injected with 3000 bacterial cells into the hemolymph are usually killed within 2 d.Citation81 However, flies challenged with a lethal dose after being primed with heat-killed bacteria resist infection.Citation81 Phagocyte activation is critical for immune priming.Citation81 Nevertheless, this long-lasting effect is not universal and needs to be evaluated individually for each microbial species.Citation182 Furthermore, flies infected with S. pneumoniae lose circadian rhythms several days before dying.Citation82 Consistently, flies lacking the central clock proteins timeless or period have higher sensitivity to S. pneumoniae but also to L. monocytogenes infection.Citation82 Interestingly, survival during a L. monocytogenes infection is determined by phagocytosis and melanization; while only phagocytosis determines survival during a S. pneumoniae infection.Citation183 A trade-off in phagocytosis is evident, because increased phagocytosis is beneficial to the host during S. pneumoniae infection but detrimental during L. monocytogenes infection.Citation183 This might be because the former is an extracellular and the latter an intracellular pathogen.
Conclusion: Drosophila phagocytes are protective and exhibit an immunological memory, while circadian rhythms modulate the Drosophila defense against S. pneumoniae. Whether similar mechanisms take place in humans will be important to explore.
Fungi
Aspergillus fumigatus
A. fumigatus is the major cause of invasive aspergillosis in immunocompromised individuals and adult flies, although other Aspergillus species are also pathogenic.Citation83 The virulence of A. fumigatus has a multifactorial nature.Citation184 In 1996 Lemaitre, Hoffmann, and collaborators found that the Toll pathway is required in Drosophila to respond to A. fumigatus infection.Citation185 While non-pathogenic to wild-type flies, this fungus is lethal to Toll-deficient flies,Citation83,Citation185 which can also be used to screen for antifungal drugs combinatorially in vivo. For example, combinatorial treatments with voricanazole and terbinafine have been shown to have a synergistic effect against infection.Citation84 In addition, Toll-deficient flies have been used in combination with zebrafish to show that A. fumigatus secondary metabolites contribute to fungal virulence and phagocyte function respectively.Citation186
Conclusion: Drug screens in immunocompromised flies against various strains of A. fumigatus can reveal the efficacy of combinatorial drug treatments.
Candida albicans and Candida glabrata
C. albicans is the predominant fungal pathogen in humans causing invasive infections and most commonly death in immunocompromised patients.Citation187C. albicans, and to a lesser extend the microbiologically disctinct Candida glabrata, can cause superficial infections in several organs using tissue site-specific virulence factors, but also bloodstream infections in immunocompromised and inflammatory bowel diseases patients.Citation188 In immunocompromised patients the systemic dissemination is thought to occur from the gut to the bloodstream.Citation144
The pathogenicity of C. albicans can be studied by systemically infecting Toll-deficient flies or by feeding wild-type Drosophila larvae, because in both systems the virulence ranking of several clinical strains is the same between mice and Drosophila.Citation143,Citation144 Drosophila intestinal infection with C. albicans results in an extensive JNK-mediated death of gut cells and the expression of antimicrobial peptides in the fat body.Citation144 Moreover, Candida pathogens secrete aspartyl proteinases (SAPs), which are critical molecules that allow them to degrade barrier tissues by hydrolysing proteins such as collagen, fibronectin and keratin in order to obtain nutrition at the site of the infection.Citation87 In addition, the secretion of SAP4 and SAP6 from Candida is necessary for the activation of systemic Toll-dependent immunity.Citation144 Although Toll pathway controls fungal infection with both C. albicans and C. glabrata, the two species differ in their ability to activate protective melanization.Citation88
Conclusion: Toll-dependent defense responses contribute to resistance although to a different extent against systemic C. albicans and C. glabrata. SAP proteases of C. albicans compromise the intestinal barrier function and contribute to pathology.
Cryptococcus neoformans
C. neoformans is another opportunistic fungal pathogen that can cause serious infections in immunocompromised patients, such as those with HIV/AIDS.Citation90 In addition, systemic Cryptococcus infection is associated with meningoencephalitis.Citation90Drosophila S2 cells can be used in combination with RNA interference technology for identifying host defense factors and mechanisms, for example, the exploitation of host autophagy by C. neoformans to survive and disseminate upon infection.Citation90 Moreover, Toll pathway is critical for host defense when C. neoformans is introduced into the hemolymph of Drosophila, but Toll and Imd pathways are dispensable for host defense against intestinal infections.Citation91 Further studies showed that there are alternative, NFκB-independent, immune responses acting in the Drosophila intestine against many intestinal pathogens.Citation92
Conclusion: Alternative routes of infection reveal the existence of intestinal defense pathways other than the Imd and Toll as critical for host defense, while host cell autophagy contributes to pathogenesis.
Cunninghamella bertholletiae and Rhizopus oryzae
C. berthollethiae and R. oryzae are filamentous fungi that cause invasive mucormycosis, and are associated with high rates of mortality, especially in immunocompromised patients, such as those with hematological malignancies.Citation93 In a Drosophila model of mucormycosis the virulence of C. bertholletiae isolates is affected by iron content the nutrient media in which fungi grow.Citation93 Similarly, corticosteroid drugs and deferoxamide that affect iron availability in the host also affect wild-type Drosophila infection with C. bertholletiae.Citation94 In addition, tarcolimus and posaconazole have been shown to have combinatorial efficacy against R. oryzae in flies and mice.Citation99
Conclusion: Drosophila models of infection show that iron availability in the growth media and iron availability in the host affect the virulence of C. bertholletiae isolates. Tarcolimus and posaconazole show promise in combinatorial treatments against R. oryzae.
Viruses
Dengue virus (DENV)
Dengue virus can cause dengue fever which can develop into dengue hemorrhagic fever and dengue shock syndrome.Citation101,Citation102 Infection of Drosophila S2 cells with four DENV serotypes (DENV1–4) induces an RNAi response. Knocking down the RNAi pathway results in 10- to 100-fold enhancement of replication of all strains tested.Citation101 In addition, a genome-wide RNA interference screen in Drosophila cells identified candidate host factors implicated in the propagation of DENV.Citation102 Eighty-two of these have human homologs, while 42 were previously known to affect virus replication in human cells.Citation102
Conclusion: An RNAi response is triggered by DENV to control infection. Additional factors conserved between Drosophila and humans have been found to control infection and those could be further explored in mammals.
Epstein–Barr virus (EBV)
Epstein–Barr virus is associated with many different cancers,Citation105,Citation106 but also with several autoimmune diseases.Citation107 Viral gene expression in Drosophila is used to identify host cell proteins that can modulate the functions of EBV immediate-early genes BRLF1 and BZLF1, which are essential for the EBV replication.Citation105,Citation106 BRLF1 expression in fly tissues inhibits known tumor suppressor genes and as a consequence induces overproliferation.Citation106 Furthermore, many Drosophila genes with known human homologs are required for EBV induced cell proliferation.Citation106
Conclusion: Drosophila is a model host system for identifying human genes, such as tumor suppressors that are targeted by BRLF1 and are relevant to EBV-mediated tumorigenesis.
Human immunodeficiency virus (HIV)
HIV is the cause of the acquired immunodeficiency syndrome (AIDS) and there is no vaccine against it. High HIV-1 replication in the host-cells is achieved by accessory proteins, including the viral protein U (Vpu).Citation112 Vpu expression in the Drosophila fat body results in the inhibition of Cactus degradation counteracting Toll pathway activation.Citation112 In addition, Vpu expression in the Drosophila wing primordia triggers apoptosis via JNK pathway signaling.Citation113
Conclusion: Vpu inhibits Toll and induces JNK pathway, depending on the tissue in which it is expressed. Thus orthotopic expression of viral proteins in immune cells and barrier epithelia might be required for the study of responses elicited by Vpu.
Influenza A virus
Influenza is caused by negative-strand RNA viruses of the family Orthomyxoviridae. It is highly contagious and sometimes deadly.Citation114 Using a modified virus able to replicate in Drosophila cells 3 genes and their human homologs (ATP6 V0D1, COX6A1, and NXF1) were found to control viral replication.Citation114 In addition, expression of the influenza virus M2 gene in Drosophila led to the identification of V1V0 ATPase as a potentiator of M2-mediated aberrant cell development to the host cell.Citation115,Citation116
Conclusion: Adaptation of the virus for growth in Drosophila cells facilitates the identification of host genes that affect influenza A virus replication and aberrant host cell programming.
SARS coronavirus (SARS-CoV)
The severe acute respiratory syndrome–coronavirus (SARS-CoV) is the etiological agent of the 2003 atypical pneumonia outbreak.Citation120,Citation121 The SARS-CoV3a locus encodes a 274 a.a. potassium channel protein, which is detected in the patient’s cells.Citation122 This protein is usually localized on the cell surface of virus-infected cells.Citation122 Drosophila expressing the 3a protein is suitable for the investigation of its apoptotic function and genetic interaction with host factors, such as cytochrome c.Citation120,Citation122 Likewise, expression of the SARS-CoV Membrane (M) structural protein in Drosophila induces apoptosis via the inhibition of the AKT pathway.Citation121
Conclusion: Drosophila transgenic models of SARS-CoV indicate genetic interactions of the viral apoptotic proteins 3a and M with cytochrome c and the AKT pathway, respectively.
Simian vacuolating virus 40 (SV40)
Simian vacuolating virus 40 belongs to the family of DNA tumor viruses.Citation123 Such viruses induce host cell proliferation in order to promote their replication.Citation123 Expression of the viral oncogene tumor antigen ST in Drosophila tissues and mammalian cells leads to its interaction with PF2A and the induction of centromere duplication.Citation123
Conclusion: The interaction of tumor antigen ST with PF2A and the concomitant centromere duplication may drive oncogenesis by SV40.
Sindbis virus (SINV)
Sindbis virus is a mosquito-borne alphavirus that can cause fever, arthralgia and rush in humans.Citation124 Natural resistance-associated macrophage protein (NRAMP), a host cell surface iron transporter with 12 transmembrane domains, is used by SINV to enter Drosophila cells in culture and in adult flies.Citation124 Consistently, SINV entry and infection of the mammalian cells is mediated by the NRAMP homolog, NRAMP2.Citation124
Interestingly, arboviruses and food nutrients induce the ERK pathway, which in turn restricts viral infection in the Drosophila intestine. That is, SINV and vesicular stomatitis virus become infective upon genetic or pharmacological inhibition of the ERK pathway. Strikingly, vertebrate insulin, which activates ERK in the mosquito gut during a blood meal, restricts viral infection of the insect intestinal epithelium.Citation125
Conclusion: NRAMP family proteins are used by the SINV α virus to enter Drosophila and mammalian cells. ERK pathway induction is pivotal for Drosophila and mosquito host intestinal defense.
Vesicular stomatitis virus (VSV)
Vesicular stomatitis virus, a member of rhabdovirus family,Citation189 is a highly promising agent for cancer treatment, since it selectively infects and kills cancer cells.Citation128 Recognition of this single stranded RNA virus by the Drosophila pattern recognition receptor Toll-7, similarly to mammalian TLR7,Citation130 results in the activation of antiviral autophagy, which is NFκB-independent.Citation129 Consistent with this, flies deficient for Toll-7 are more susceptible to VSV infection.Citation129 Other studies in both adult flies and Drosophila S2 cells also show that activation of autophagy in Drosophila decreases the replication of VSV.Citation190 Interestingly, the host cells recognize a preformed component of the virus and induce autophagy before the initiation of viral replication.Citation190
Conclusion: Similarly to mammalian TLR7, Drosophila Toll-7 induces autophagy to suppress VSV infection in an NFκB-independent manner. Nevertheless, Toll-7 recognizes the viral capsid, as opposed to viral RNA recognition by the mammalian TLR7. Thus, similarly to Toll, Toll-7 pathway appears conserved in mammals, but only downstream of the receptor.
West Nile virus (WNV)
West Nile virus is emerging as a highly virulent human pathogen.Citation131,Citation132 It belongs to neurotropic mosquito-borne flavivirusesCitation131,Citation132 causing fever, meningitis and encephalitis. Similarly to VSV infection, WNV infection induces RNAi as a defense mechanism in Drosophila.Citation133 WNV infection of adult Drosophila also supports the idea of a triggered protective RNAi response upon infection.Citation133 Importantly, non-coding WNV and other flavivirus RNA can suppress the RNAi defense mechanism in mammalian and Drosophila cells.Citation131
Conclusion: WNV can induce and suppress RNAi in Drosophila and mammals.
Shortcomings of Drosophila Models of Microbial Infections
Drosophila can be used to investigate many mechanisms underlying microbial infections in humans, but there are also limitations in its use due to the evolutionary distance between flies and mammals. Thus a gold standard in studying human pathogens in flies is to verify findings in mammalian models of infection. Focusing on conserved aspects of host immunity and physiology increases the chance that any mechanism of pathogenesis identified in Drosophila will have a direct impact in humans. For example, the Drosophila melanization, while clearly contributing to host defense, it does not appear conserved in mammals. Thus, caution should be taken when interpreting findings related to the fly melanization in terms of human infectious diseases.
Some aspects of wound healing and inflammation cannot be modeled in Drosophila, because particular cells and tissues found in mammals are missing from flies. For example, flies lack an adaptive immune response as we know it in humans, thus they are inappropriate for studying the impact of the known adaptive immunity on tissue repair and inflammation.Citation191 Also fibrosis and scarring cannot be easily investigated in Drosophila because there are no myofibroblasts and no connective tissues to induce fibrosis.Citation191 Additionally, flies lack structural orthologs of many mammalian effector molecules, including chemokines, which are crucial for cell communication and regulation of inflammation during infection.Citation138,Citation191 Furthermore, due to the absence of lamina propria from the Drosophila intestine, which includes connective tissue, myofibroblasts, and immune cells, it is only possible to study regenerative inflammatory signals of the intestinal epithelium, trachea, and muscle.Citation2 For example, the local tissue-emanating signals in Drosophila that control regeneration of the intestinal epithelium upon damage or infection.Citation147
Additional limitations may also be posed by the wrong choice of infection methods. For example, when Drosophila is injected directly into the hemolymph with various bacteria, flies can be killed even by bacterial strains that are considered nonpathogenic in mammals.Citation192 Thus, this technique might fail to distinguish between virulent and non-virulent bacteria,Citation192 in which case pathogenicity cannot be studied and alternative modes of infection should be tried. Accordingly, infection modes that mimic intestinal or wound infections might be more appropriate for highly virulent microbes, such as P. aeruginosa, E. faecalis, and S. aureus that initially exert their virulence locally on soft tissues. Importantly, while major differences in host survival to infection and bacterial load are mostly independent of the general genetic background, less extensive differences are not.Citation145 In the latter case isogenic fly strains should be compared or more than one wild-type and mutant fly strains for the same gene should be assessed.
Finally, while some mammalian viruses can be recognized by and can enter Drosophila cells, others need to be previously modified. Therefore in many cases only viral proteins can be assessed via transgene expression in fly tissues. While transgenic flies can produce valuable results they do not necessarily recapitulate the complexity of the whole virus and can only provide insights on specific aspects of the infection.
Conclusions
A better understanding of host–microbe interactions is critical for the development of successful treatments. Drosophila represents a very useful invertebrate model host for studying many human microbes. Similarly to humans, host–pathogen interactions in flies are far more complex than the induction of distinct immune responses directed against gram-negative or gram-positive bacteria and fungi or viruses. This is because microbial strain-specific virulence factors—identified in Drosophila and other hosts—and host factors control, not only innate immune responses, but also muscle homeostasis, intestinal regeneration, predisposition for cancer, and tolerance to infection. Prominent among those is the role of intestinal regeneration as a protective response induced by pathogens, such as P. aeruginosa, but also beneficial bacteria, such as L. plantarum. Interestingly, V. cholerae appears to have the ability to suppress regeneration and S. marcescens appears to benefit from the induction of regeneration. Clearly, future studies can shed more light into this exciting area of research.
Regarding the modeling of disease in flies, the route of infection plays a pivotal role in the interaction. Microbial injection into the hemolymph, for example, bypasses many of the host barrier defenses, and it might be appropriate to study systemic infections, but not highly virulent microbes able to bypass Drosophila barrier defenses. Finally, microbes that do not inflict disease in wild type or even in immunocompromised flies can still be studied if their virulence factors are genetically expressed preferentially orthotopically in fly tissues homologous to those relevant to human pathophysiology.
Much of the knowledge gained from Drosophila studies of human microbes is and will continue to be important for biomedical research because most infection models strive to recapitulate conserved aspects of human disease. Despite the existence of rough guidelines, there is no strict formula of success in modeling human disease in flies. Thus, validation of any new findings conventionally necessitates the use of mammalian models of infectious disease.
Disclosure of Potential Conflicts of Interest
No potential conflicts of interest were disclosed.
Acknowledgments
We thank Chrysoula Pitsouli for critical reading of the manuscript and our funding sources, Marie Curie GIG-Infection Cancer and Fontation Sante YASante2013 to Y.A.
References
- Qin J, Li R, Raes J, Arumugam M, Burgdorf KS, Manichanh C, Nielsen T, Pons N, Levenez F, Yamada T, et al, MetaHIT Consortium. A human gut microbial gene catalogue established by metagenomic sequencing. Nature 2010; 464:59 - 65; http://dx.doi.org/10.1038/nature08821; PMID: 20203603
- Apidianakis Y, Rahme LG. Drosophila melanogaster as a model for human intestinal infection and pathology. Dis Model Mech 2011; 4:21 - 30; http://dx.doi.org/10.1242/dmm.003970; PMID: 21183483
- Bosco-Drayon V, Poidevin M, Boneca IG, Narbonne-Reveau K, Royet J, Charroux B. Peptidoglycan sensing by the receptor PGRP-LE in the Drosophila gut induces immune responses to infectious bacteria and tolerance to microbiota. Cell Host Microbe 2012; 12:153 - 65; http://dx.doi.org/10.1016/j.chom.2012.06.002; PMID: 22901536
- Canny GO, McCormick BA. Bacteria in the intestine, helpful residents or enemies from within?. Infect Immun 2008; 76:3360 - 73; http://dx.doi.org/10.1128/IAI.00187-08; PMID: 18474643
- Gupta GR. Tackling pneumonia and diarrhoea: the deadliest diseases for the world’s poorest children. Lancet 2012; 379:2123 - 4; http://dx.doi.org/10.1016/S0140-6736(12)60907-6; PMID: 22682449
- Mariappan V, Vellasamy KM, Hashim OH, Vadivelu J. Profiling of Burkholderia cepacia secretome at mid-logarithmic and early-stationary phases of growth. PLoS One 2011; 6:e26518; http://dx.doi.org/10.1371/journal.pone.0026518; PMID: 22046299
- Schneider DS, Ayres JS, Brandt SM, Costa A, Dionne MS, Gordon MD, Mabery EM, Moule MG, Pham LN, Shirasu-Hiza MM. Drosophila eiger mutants are sensitive to extracellular pathogens. PLoS Pathog 2007; 3:e41; http://dx.doi.org/10.1371/journal.ppat.0030041; PMID: 17381241
- Ayres JS, Schneider DS. A signaling protease required for melanization in Drosophila affects resistance and tolerance of infections. PLoS Biol 2008; 6:2764 - 73; http://dx.doi.org/10.1371/journal.pbio.0060305; PMID: 19071960
- Pilátová M, Dionne MS. Burkholderia thailandensis is virulent in Drosophila melanogaster. PLoS One 2012; 7:e49745; http://dx.doi.org/10.1371/journal.pone.0049745; PMID: 23209596
- Kocazeybek B. Chronic Chlamydophila pneumoniae infection in lung cancer, a risk factor: a case-control study. J Med Microbiol 2003; 52:721 - 6; http://dx.doi.org/10.1099/jmm.0.04845-0; PMID: 12867569
- Cocchiaro JL, Valdivia RH. New insights into Chlamydia intracellular survival mechanisms. Cell Microbiol 2009; 11:1571 - 8; http://dx.doi.org/10.1111/j.1462-5822.2009.01364.x; PMID: 19673891
- Derré I, Pypaert M, Dautry-Varsat A, Agaisse H. RNAi screen in Drosophila cells reveals the involvement of the Tom complex in Chlamydia infection. PLoS Pathog 2007; 3:1446 - 58; http://dx.doi.org/10.1371/journal.ppat.0030155; PMID: 17967059
- Vanha-Aho LM, Kleino A, Kaustio M, Ulvila J, Wilke B, Hultmark D, Valanne S, Rämet M. Functional characterization of the infection-inducible peptide Edin in Drosophila melanogaster. PLoS One 2012; 7:e37153; http://dx.doi.org/10.1371/journal.pone.0037153; PMID: 22606343
- Buchon N, Broderick NA, Poidevin M, Pradervand S, Lemaitre B. Drosophila intestinal response to bacterial infection: activation of host defense and stem cell proliferation. Cell Host Microbe 2009; 5:200 - 11; http://dx.doi.org/10.1016/j.chom.2009.01.003; PMID: 19218090
- Paredes JC, Welchman DP, Poidevin M, Lemaitre B. Negative regulation by amidase PGRPs shapes the Drosophila antibacterial response and protects the fly from innocuous infection. Immunity 2011; 35:770 - 9; http://dx.doi.org/10.1016/j.immuni.2011.09.018; PMID: 22118526
- Vodovar N, Acosta C, Lemaitre B, Boccard F. Drosophila: a polyvalent model to decipher host-pathogen interactions. Trends Microbiol 2004; 12:235 - 42; http://dx.doi.org/10.1016/j.tim.2004.03.007; PMID: 15120143
- Ahlund MK, Rydén P, Sjöstedt A, Stöven S. Directed screen of Francisella novicida virulence determinants using Drosophila melanogaster. Infect Immun 2010; 78:3118 - 28; http://dx.doi.org/10.1128/IAI.00146-10; PMID: 20479082
- Asare R, Akimana C, Jones S, Abu Kwaik Y. Molecular bases of proliferation of Francisella tularensis in arthropod vectors. Environ Microbiol 2010; 12:2587 - 612; http://dx.doi.org/10.1111/j.1462-2920.2010.02230.x; PMID: 20482589
- Akimana C, Al-Khodor S, Abu Kwaik Y. Host factors required for modulation of phagosome biogenesis and proliferation of Francisella tularensis within the cytosol. PLoS One 2010; 5:e11025; http://dx.doi.org/10.1371/journal.pone.0011025; PMID: 20552012
- Moule MG, Monack DM, Schneider DS. Reciprocal analysis of Francisella novicida infections of a Drosophila melanogaster model reveal host-pathogen conflicts mediated by reactive oxygen and imd-regulated innate immune response. PLoS Pathog 2010; 6:e1001065; http://dx.doi.org/10.1371/journal.ppat.1001065; PMID: 20865166
- Vonkavaara M, Pavel ST, Hölzl K, Nordfelth R, Sjöstedt A, Stöven S. Francisella is sensitive to insect antimicrobial peptides. J Innate Immun 2013; 5:50 - 9; http://dx.doi.org/10.1159/000342468; PMID: 23037919
- Wandler AM, Guillemin K. Transgenic expression of the Helicobacter pylori virulence factor CagA promotes apoptosis or tumorigenesis through JNK activation in Drosophila. PLoS Pathog 2012; 8:e1002939; http://dx.doi.org/10.1371/journal.ppat.1002939; PMID: 23093933
- Botham CM, Wandler AM, Guillemin K. A transgenic Drosophila model demonstrates that the Helicobacter pylori CagA protein functions as a eukaryotic Gab adaptor. PLoS Pathog 2008; 4:e1000064; http://dx.doi.org/10.1371/journal.ppat.1000064; PMID: 18483552
- Muyskens JB, Guillemin K. Helicobacter pylori CagA disrupts epithelial patterning by activating myosin light chain. PLoS One 2011; 6:e17856; http://dx.doi.org/10.1371/journal.pone.0017856; PMID: 21445303
- Kubori T, Shinzawa N, Kanuka H, Nagai H. Legionella metaeffector exploits host proteasome to temporally regulate cognate effector. PLoS Pathog 2010; 6:e1001216; http://dx.doi.org/10.1371/journal.ppat.1001216; PMID: 21151961
- Oh CT, Moon C, Jeong MS, Kwon SH, Jang J. Drosophila melanogaster model for Mycobacterium abscessus infection. Microbes Infect 2013; 15:788 - 95; http://dx.doi.org/10.1016/j.micinf.2013.06.011; PMID: 23831804
- Vuković D, Parezanović V, Savić B, Dakić I, Laban-Nestorović S, Ilić S, Ćirković I, Stepanović S. Mycobacterium fortuitum endocarditis associated with cardiac surgery, Serbia. Emerg Infect Dis 2013; 19:517 - 9; http://dx.doi.org/10.3201/eid1903.120763; PMID: 23750363
- Philips JA, Rubin EJ, Perrimon N. Drosophila RNAi screen reveals CD36 family member required for mycobacterial infection. Science 2005; 309:1251 - 3; http://dx.doi.org/10.1126/science.1116006; PMID: 16020694
- Broutin V, Bañuls AL, Aubry A, Keck N, Choisy M, Bernardet JF, Michel C, Raymond JC, Libert C, Barnaud A, et al. Genetic diversity and population structure of Mycobacterium marinum: new insights into host and environmental specificities. J Clin Microbiol 2012; 50:3627 - 34; http://dx.doi.org/10.1128/JCM.01274-12; PMID: 22952269
- Dionne MS, Ghori N, Schneider DS. Drosophila melanogaster is a genetically tractable model host for Mycobacterium marinum. Infect Immun 2003; 71:3540 - 50; http://dx.doi.org/10.1128/IAI.71.6.3540-3550.2003; PMID: 12761139
- Kim JJ, Lee HM, Shin DM, Kim W, Yuk JM, Jin HS, Lee SH, Cha GH, Kim JM, Lee ZW, et al. Host cell autophagy activated by antibiotics is required for their effective antimycobacterial drug action. Cell Host Microbe 2012; 11:457 - 68; http://dx.doi.org/10.1016/j.chom.2012.03.008; PMID: 22607799
- Manzanillo PS, Ayres JS, Watson RO, Collins AC, Souza G, Rae CS, Schneider DS, Nakamura K, Shiloh MU, Cox JS. The ubiquitin ligase parkin mediates resistance to intracellular pathogens. Nature 2013; 501:512 - 6; http://dx.doi.org/10.1038/nature12566; PMID: 24005326
- Koo IC, Ohol YM, Wu P, Morisaki JH, Cox JS, Brown EJ. Role for lysosomal enzyme beta-hexosaminidase in the control of mycobacteria infection. Proc Natl Acad Sci U S A 2008; 105:710 - 5; http://dx.doi.org/10.1073/pnas.0708110105; PMID: 18180457
- Verma P, Tapadia MG. Immune response and anti-microbial peptides expression in Malpighian tubules of Drosophila melanogaster is under developmental regulation. PLoS One 2012; 7:e40714; http://dx.doi.org/10.1371/annotation/4b02305d-dcb8-40db-8f1f-1f7f0da51544; PMID: 22808242
- Philips JA, Porto MC, Wang H, Rubin EJ, Perrimon N. ESCRT factors restrict mycobacterial growth. Proc Natl Acad Sci U S A 2008; 105:3070 - 5; http://dx.doi.org/10.1073/pnas.0707206105; PMID: 18287038
- Aymeric JL, Givaudan A, Duvic B. Imd pathway is involved in the interaction of Drosophila melanogaster with the entomopathogenic bacteria, Xenorhabdus nematophila and Photorhabdus luminescens. Mol Immunol 2010; 47:2342 - 8; http://dx.doi.org/10.1016/j.molimm.2010.05.012; PMID: 20627393
- Galac MR, Lazzaro BP. Comparative pathology of bacteria in the genus Providencia to a natural host, Drosophila melanogaster. Microbes Infect 2011; 13:673 - 83; http://dx.doi.org/10.1016/j.micinf.2011.02.005; PMID: 21354324
- Apidianakis Y, Mindrinos MN, Xiao W, Lau GW, Baldini RL, Davis RW, Rahme LG. Profiling early infection responses: Pseudomonas aeruginosa eludes host defenses by suppressing antimicrobial peptide gene expression. Proc Natl Acad Sci U S A 2005; 102:2573 - 8; http://dx.doi.org/10.1073/pnas.0409588102; PMID: 15695583
- Apidianakis Y, Pitsouli C, Perrimon N, Rahme L. Synergy between bacterial infection and genetic predisposition in intestinal dysplasia. Proc Natl Acad Sci U S A 2009; 106:20883 - 8; http://dx.doi.org/10.1073/pnas.0911797106; PMID: 19934041
- Mulcahy H, Sibley CD, Surette MG, Lewenza S. Drosophila melanogaster as an animal model for the study of Pseudomonas aeruginosa biofilm infections in vivo. PLoS Pathog 2011; 7:e1002299; http://dx.doi.org/10.1371/journal.ppat.1002299; PMID: 21998591
- Buchon N, Broderick NA, Chakrabarti S, Lemaitre B. Invasive and indigenous microbiota impact intestinal stem cell activity through multiple pathways in Drosophila. Genes Dev 2009; b 23:2333 - 44; http://dx.doi.org/10.1101/gad.1827009; PMID: 19797770
- Vodovar N, Vinals M, Liehl P, Basset A, Degrouard J, Spellman P, Boccard F, Lemaitre B. Drosophila host defense after oral infection by an entomopathogenic Pseudomonas species. Proc Natl Acad Sci U S A 2005; 102:11414 - 9; http://dx.doi.org/10.1073/pnas.0502240102; PMID: 16061818
- Lucas RL, Lee CA. Unravelling the mysteries of virulence gene regulation in Salmonella typhimurium. Mol Microbiol 2000; 36:1024 - 33; http://dx.doi.org/10.1046/j.1365-2958.2000.01961.x; PMID: 10844688
- Jones RM, Wu H, Wentworth C, Luo L, Collier-Hyams L, Neish AS. Salmonella AvrA coordinates suppression of host immune and apoptotic defenses via JNK pathway blockade. Cell Host Microbe 2008; 3:233 - 44; http://dx.doi.org/10.1016/j.chom.2008.02.016; PMID: 18407067
- Mabery EM, Schneider DS. The Drosophila TNF ortholog eiger is required in the fat body for a robust immune response. J Innate Immun 2010; 2:371 - 8; http://dx.doi.org/10.1159/000315050; PMID: 20505310
- Shinzawa N, Nelson B, Aonuma H, Okado K, Fukumoto S, Miura M, Kanuka H. p38 MAPK-dependent phagocytic encapsulation confers infection tolerance in Drosophila. Cell Host Microbe 2009; 6:244 - 52; http://dx.doi.org/10.1016/j.chom.2009.07.010; PMID: 19748466
- Polilli E, Parruti G, Fazii P, D’Antonio D, Palmieri D, D’Incecco C, Mangifesta A, Garofalo G, Del Duca L, D’Amario C, et al. Rapidly controlled outbreak of Serratia marcescens infection/colonisations in a neonatal intensive care unit, Pescara General Hospital, Pescara, Italy, April 2011. Euro Surveill 2011; 16:19892; PMID: 21699768
- Nehme NT, Liégeois S, Kele B, Giammarinaro P, Pradel E, Hoffmann JA, Ewbank JJ, Ferrandon D. A model of bacterial intestinal infections in Drosophila melanogaster. PLoS Pathog 2007; 3:e173; http://dx.doi.org/10.1371/journal.ppat.0030173; PMID: 18039029
- Cronin SJ, Nehme NT, Limmer S, Liegeois S, Pospisilik JA, Schramek D, Leibbrandt A, Simoes RdeM, Gruber S, Puc U, et al. Genome-wide RNAi screen identifies genes involved in intestinal pathogenic bacterial infection. Science 2009; 325:340 - 3; http://dx.doi.org/10.1126/science.1173164; PMID: 19520911
- Wong XM, Younger S, Peters CJ, Jan YN, Jan LY. Subdued, a TMEM16 family Ca2+-activated Cl- channel in Drosophila melanogaster with an unexpected role in host defense. Elife 2013; 2:e00862; http://dx.doi.org/10.7554/eLife.00862; PMID: 24192034
- Herren JK, Lemaitre B. Spiroplasma and host immunity: activation of humoral immune responses increases endosymbiont load and susceptibility to certain Gram-negative bacterial pathogens in Drosophila melanogaster. Cell Microbiol 2011; 13:1385 - 96; http://dx.doi.org/10.1111/j.1462-5822.2011.01627.x; PMID: 21740495
- Blow NS, Salomon RN, Garrity K, Reveillaud I, Kopin A, Jackson FR, Watnick PI. Vibrio cholerae infection of Drosophila melanogaster mimics the human disease cholera. PLoS Pathog 2005; 1:e8; http://dx.doi.org/10.1371/journal.ppat.0010008; PMID: 16201020
- Wang Z, Hang S, Purdy AE, Watnick PI. Mutations in the IMD pathway and mustard counter Vibrio cholerae suppression of intestinal stem cell division in Drosophila. MBio 2013; 4:e00337 - 13; http://dx.doi.org/10.1128/mBio.00337-13; PMID: 23781070
- Guichard A, Cruz-Moreno B, Aguilar B, van Sorge NM, Kuang J, Kurkciyan AA, Wang Z, Hang S, Pineton de Chambrun GP, McCole DF, et al. Cholera toxin disrupts barrier function by inhibiting exocyst-mediated trafficking of host proteins to intestinal cell junctions. Cell Host Microbe 2013; 14:294 - 305; http://dx.doi.org/10.1016/j.chom.2013.08.001; PMID: 24034615
- Dlu P, Goriacheva II, Andrianov BV, Reznik NL, Lazebnyĭ OE, Kulikov AM. The endosymbiotic bacterium Wolbachia enhances the nonspecific resistance to insect pathogens and alters behavior of Drosophila melanogaster. Genetika 2007; 43:1277 - 80; PMID: 17990528
- Teixeira L, Ferreira A, Ashburner M. The bacterial symbiont Wolbachia induces resistance to RNA viral infections in Drosophila melanogaster. PLoS Biol 2008; 6:e2; http://dx.doi.org/10.1371/journal.pbio.1000002; PMID: 19222304
- Hedges LM, Yamada R, O’Neill SL, Johnson KN. The small interfering RNA pathway is not essential for Wolbachia-mediated antiviral protection in Drosophila melanogaster. Appl Environ Microbiol 2012; 78:6773 - 6; http://dx.doi.org/10.1128/AEM.01650-12; PMID: 22798369
- Wong ZS, Hedges LM, Brownlie JC, Johnson KN. Wolbachia-mediated antibacterial protection and immune gene regulation in Drosophila. PLoS One 2011; 6:e25430; http://dx.doi.org/10.1371/journal.pone.0025430; PMID: 21980455
- Rottschaefer SM, Lazzaro BP. No effect of Wolbachia on resistance to intracellular infection by pathogenic bacteria in Drosophila melanogaster. PLoS One 2012; 7:e40500; http://dx.doi.org/10.1371/journal.pone.0040500; PMID: 22808174
- Koo JT, Alleyne TM, Schiano CA, Jafari N, Lathem WW. Global discovery of small RNAs in Yersinia pseudotuberculosis identifies Yersinia-specific small, noncoding RNAs required for virulence. Proc Natl Acad Sci U S A 2011; 108:E709 - 17; http://dx.doi.org/10.1073/pnas.1101655108; PMID: 21876162
- An D, Apidianakis Y, Boechat AL, Baldini RL, Goumnerov BC, Rahme LG. The pathogenic properties of a novel and conserved gene product, KerV, in proteobacteria. PLoS One 2009; 4:e7167; http://dx.doi.org/10.1371/journal.pone.0007167; PMID: 19779606
- Guichard A, McGillivray SM, Cruz-Moreno B, van Sorge NM, Nizet V, Bier E. Anthrax toxins cooperatively inhibit endocytic recycling by the Rab11/Sec15 exocyst. Nature 2010; 467:854 - 8; http://dx.doi.org/10.1038/nature09446; PMID: 20944747
- Kotiranta A, Lounatmaa K, Haapasalo M. Epidemiology and pathogenesis of Bacillus cereus infections. Microbes Infect 2000; 2:189 - 98; http://dx.doi.org/10.1016/S1286-4579(00)00269-0; PMID: 10742691
- Ma J, Benson AK, Kachman SD, Hu Z, Harshman LG. Drosophila melanogaster Selection for Survival of Bacillus cereus Infection: Life History Trait Indirect Responses. Int J Evol Biol 2012; 2012:935970; http://dx.doi.org/10.1155/2012/935970; PMID: 23094195
- Gill M, Ellar D. Transgenic Drosophila reveals a functional in vivo receptor for the Bacillus thuringiensis toxin Cry1Ac1. Insect Mol Biol 2002; 11:619 - 25; http://dx.doi.org/10.1046/j.1365-2583.2002.00373.x; PMID: 12421420
- Cox CR, Gilmore MS. Native microbial colonization of Drosophila melanogaster and its use as a model of Enterococcus faecalis pathogenesis. Infect Immun 2007; 75:1565 - 76; http://dx.doi.org/10.1128/IAI.01496-06; PMID: 17220307
- Teixeira N, Varahan S, Gorman MJ, Palmer KL, Zaidman-Remy A, Yokohata R, Nakayama J, Hancock LE, Jacinto A, Gilmore MS, et al. Drosophila host model reveals new enterococcus faecalis quorum-sensing associated virulence factors. PLoS One 2013; 8:e64740; http://dx.doi.org/10.1371/journal.pone.0064740; PMID: 23734216
- Karczewski J, Troost FJ, Konings I, Dekker J, Kleerebezem M, Brummer RJ, Wells JM. Regulation of human epithelial tight junction proteins by Lactobacillus plantarum in vivo and protective effects on the epithelial barrier. Am J Physiol Gastrointest Liver Physiol 2010; 298:G851 - 9; http://dx.doi.org/10.1152/ajpgi.00327.2009; PMID: 20224007
- Storelli G, Defaye A, Erkosar B, Hols P, Royet J, Leulier F. Lactobacillus plantarum promotes Drosophila systemic growth by modulating hormonal signals through TOR-dependent nutrient sensing. Cell Metab 2011; 14:403 - 14; http://dx.doi.org/10.1016/j.cmet.2011.07.012; PMID: 21907145
- Liu D. Identification, subtyping and virulence determination of Listeria monocytogenes, an important foodborne pathogen. J Med Microbiol 2006; 55:645 - 59; http://dx.doi.org/10.1099/jmm.0.46495-0; PMID: 16687581
- Yano T, Mita S, Ohmori H, Oshima Y, Fujimoto Y, Ueda R, Takada H, Goldman WE, Fukase K, Silverman N, et al. Autophagic control of listeria through intracellular innate immune recognition in drosophila. Nat Immunol 2008; 9:908 - 16; http://dx.doi.org/10.1038/ni.1634; PMID: 18604211
- Mansfield BE, Dionne MS, Schneider DS, Freitag NE. Exploration of host-pathogen interactions using Listeria monocytogenes and Drosophila melanogaster. Cell Microbiol 2003; 5:901 - 11; http://dx.doi.org/10.1046/j.1462-5822.2003.00329.x; PMID: 14641175
- Ma ES, Wong CL, Lai KT, Chan EC, Yam WC, Chan AC. Kocuria kristinae infection associated with acute cholecystitis. BMC Infect Dis 2005; 5:60; http://dx.doi.org/10.1186/1471-2334-5-60; PMID: 16029488
- Nehme NT, Quintin J, Cho JH, Lee J, Lafarge MC, Kocks C, Ferrandon D. Relative roles of the cellular and humoral responses in the Drosophila host defense against three gram-positive bacterial infections. PLoS One 2011; 6:e14743; http://dx.doi.org/10.1371/journal.pone.0014743; PMID: 21390224
- Cataldo MA, Taglietti F, Petrosillo N. Methicillin-resistant Staphylococcus aureus: a community health threat. Postgrad Med 2010; 122:16 - 23; http://dx.doi.org/10.3810/pgm.2010.11.2218; PMID: 21084777
- Tabuchi Y, Shiratsuchi A, Kurokawa K, Gong JH, Sekimizu K, Lee BL, Nakanishi Y. Inhibitory role for D-alanylation of wall teichoic acid in activation of insect Toll pathway by peptidoglycan of Staphylococcus aureus. J Immunol 2010; 185:2424 - 31; http://dx.doi.org/10.4049/jimmunol.1000625; PMID: 20639481
- Kurokawa K, Gong JH, Ryu KH, Zheng L, Chae JH, Kim MS, Lee BL. Biochemical characterization of evasion from peptidoglycan recognition by Staphylococcus aureus D-alanylated wall teichoic acid in insect innate immunity. Dev Comp Immunol 2011; 35:835 - 9; http://dx.doi.org/10.1016/j.dci.2011.03.001; PMID: 21453720
- Atilano ML, Yates J, Glittenberg M, Filipe SR, Ligoxygakis P. Wall teichoic acids of Staphylococcus aureus limit recognition by the drosophila peptidoglycan recognition protein-SA to promote pathogenicity. PLoS Pathog 2011; 7:e1002421; http://dx.doi.org/10.1371/journal.ppat.1002421; PMID: 22144903
- Ayyaz A, Giammarinaro P, Liégeois S, Lestradet M, Ferrandon D. A negative role for MyD88 in the resistance to starvation as revealed in an intestinal infection of Drosophila melanogaster with the Gram-positive bacterium Staphylococcus xylosus. Immunobiology 2013; 218:635 - 44; http://dx.doi.org/10.1016/j.imbio.2012.07.027; PMID: 23083631
- Zbinden A, Köhler N, Bloemberg GV. recA-based PCR assay for accurate differentiation of Streptococcus pneumoniae from other viridans streptococci. J Clin Microbiol 2011; 49:523 - 7; http://dx.doi.org/10.1128/JCM.01450-10; PMID: 21147955
- Pham LN, Dionne MS, Shirasu-Hiza M, Schneider DS. A specific primed immune response in Drosophila is dependent on phagocytes. PLoS Pathog 2007; 3:e26; http://dx.doi.org/10.1371/journal.ppat.0030026; PMID: 17352533
- Shirasu-Hiza MM, Dionne MS, Pham LN, Ayres JS, Schneider DS. Interactions between circadian rhythm and immunity in Drosophila melanogaster. Curr Biol 2007; 17:R353 - 5; http://dx.doi.org/10.1016/j.cub.2007.03.049; PMID: 17502084
- Ben-Ami R, Lamaris GA, Lewis RE, Kontoyiannis DP. Interstrain variability in the virulence of Aspergillus fumigatus and Aspergillus terreus in a Toll-deficient Drosophila fly model of invasive aspergillosis. Med Mycol 2010; 48:310 - 7; http://dx.doi.org/10.3109/13693780903148346; PMID: 19642052
- Lionakis MS, Kontoyiannis DP. Fruit flies as a minihost model for studying drug activity and virulence in Aspergillus. Med Mycol 2005; 43:Suppl 1 S111 - 4; http://dx.doi.org/10.1080/13693780400020030; PMID: 16110801
- Matskevich AA, Quintin J, Ferrandon D. The Drosophila PRR GNBP3 assembles effector complexes involved in antifungal defenses independently of its Toll-pathway activation function. Eur J Immunol 2010;; 40:1244 - 54; http://dx.doi.org/10.1002/eji.200940164; PMID: 20201042
- Le Bourg E, Massou I, Gobert V. Cold stress increases resistance to fungal infection throughout life in Drosophila melanogaster. Biogerontology 2009; 10:613 - 25; http://dx.doi.org/10.1007/s10522-008-9206-y; PMID: 19067222
- Khan MS, Ahmad I, Aqil F, Owais M, Shahid M, Musarrat J. Virulence and pathogenicity of fungal pathogens with special reference to Candida albicans. In: Ahmad I, Owais M, Shahid M, Aqil F, editors. Combating fungal infections: problems and remedy. Berlin, Heidelberg, Germany: Springer-Verlag; 2010. p. 21-45
- Quintin J, Asmar J, Matskevich AA, Lafarge MC, Ferrandon D. The Drosophila Toll pathway controls but does not clear Candida glabrata infections. J Immunol 2013; 190:2818 - 27; http://dx.doi.org/10.4049/jimmunol.1201861; PMID: 23401590
- Kocks C, Cho JH, Nehme N, Ulvila J, Pearson AM, Meister M, Strom C, Conto SL, Hetru C, Stuart LM, et al. Eater, a transmembrane protein mediating phagocytosis of bacterial pathogens in Drosophila. Cell 2005; 123:335 - 46; http://dx.doi.org/10.1016/j.cell.2005.08.034; PMID: 16239149
- Qin QM, Luo J, Lin X, Pei J, Li L, Ficht TA, de Figueiredo P. Functional analysis of host factors that mediate the intracellular lifestyle of Cryptococcus neoformans. PLoS Pathog 2011; 7:e1002078; http://dx.doi.org/10.1371/journal.ppat.1002078; PMID: 21698225
- Apidianakis Y, Rahme LG, Heitman J, Ausubel FM, Calderwood SB, Mylonakis E. Challenge of Drosophila melanogaster with Cryptococcus neoformans and role of the innate immune response. Eukaryot Cell 2004; 3:413 - 9; http://dx.doi.org/10.1128/EC.3.2.413-419.2004; PMID: 15075271
- Ha EM, Oh CT, Bae YS, Lee WJ. A direct role for dual oxidase in Drosophila gut immunity. Science 2005; 310:847 - 50; http://dx.doi.org/10.1126/science.1117311; PMID: 16272120
- Pongas GN, Ben-Ami R, Lewis RE, Walsh TJ, Kontoyiannis DP. Culture medium composition affects the lethality of Cunninghamella bertholletiae in a fly model of mucormycosis. Antimicrob Agents Chemother 2009; 53:4569; http://dx.doi.org/10.1128/AAC.00994-09; PMID: 19635953
- Chamilos G, Lewis RE, Hu J, Xiao L, Zal T, Gilliet M, Halder G, Kontoyiannis DP. Drosophila melanogaster as a model host to dissect the immunopathogenesis of zygomycosis. Proc Natl Acad Sci U S A 2008; 105:9367 - 72; http://dx.doi.org/10.1073/pnas.0709578105; PMID: 18583479
- Lamaris GA, Chamilos G, Lewis RE, Kontoyiannis DP. Virulence studies of Scedosporium and Fusarium species in Drosophila melanogaster. J Infect Dis 2007; 196:1860 - 4; http://dx.doi.org/10.1086/523765; PMID: 18190268
- Pal S, St Leger RJ, Wu LP. Fungal peptide Destruxin A plays a specific role in suppressing the innate immune response in Drosophila melanogaster. J Biol Chem 2007; 282:8969 - 77; http://dx.doi.org/10.1074/jbc.M605927200; PMID: 17227774
- Gottar M, Gobert V, Matskevich AA, Reichhart JM, Wang C, Butt TM, Belvin M, Hoffmann JA, Ferrandon D. Dual detection of fungal infections in Drosophila via recognition of glucans and sensing of virulence factors. Cell 2006; 127:1425 - 37; http://dx.doi.org/10.1016/j.cell.2006.10.046; PMID: 17190605
- Evans SE, Leventakos K, Ben-Ami R, You D, Thakkar SG, Lewis RE, Kontoyiannis DP. Toll-deficient Drosophila are resistant to infection by Pneumocystis spp.: additional evidence of specificity to mammalian hosts. Virulence 2010; 1:523 - 5; http://dx.doi.org/10.4161/viru.1.6.13903; PMID: 21178507
- Lewis RE, Ben-Ami R, Best L, Albert N, Walsh TJ, Kontoyiannis DP. Tacrolimus enhances the potency of posaconazole against Rhizopus oryzae in vitro and in an experimental model of mucormycosis. J Infect Dis 2013; 207:834 - 41; http://dx.doi.org/10.1093/infdis/jis767; PMID: 23242544
- Garrey JL, Lee YY, Au HH, Bushell M, Jan E. Host and viral translational mechanisms during cricket paralysis virus infection. J Virol 2010; 84:1124 - 38; http://dx.doi.org/10.1128/JVI.02006-09; PMID: 19889774
- Mukherjee S, Hanley KA. RNA interference modulates replication of dengue virus in Drosophila melanogaster cells. BMC Microbiol 2010; 10:127; http://dx.doi.org/10.1186/1471-2180-10-127; PMID: 20420715
- Sessions OM, Barrows NJ, Souza-Neto JA, Robinson TJ, Hershey CL, Rodgers MA, Ramirez JL, Dimopoulos G, Yang PL, Pearson JL, et al. Discovery of insect and human dengue virus host factors. Nature 2009; 458:1047 - 50; http://dx.doi.org/10.1038/nature07967; PMID: 19396146
- Cherry S, Kunte A, Wang H, Coyne C, Rawson RB, Perrimon N. COPI activity coupled with fatty acid biosynthesis is required for viral replication. PLoS Pathog 2006; 2:e102; http://dx.doi.org/10.1371/journal.ppat.0020102; PMID: 17040126
- Tsai CW, McGraw EA, Ammar ED, Dietzgen RG, Hogenhout SA. Drosophila melanogaster mounts a unique immune response to the Rhabdovirus sigma virus. Appl Environ Microbiol 2008; 74:3251 - 6; http://dx.doi.org/10.1128/AEM.02248-07; PMID: 18378641
- Adamson AL, Wright N, LaJeunesse DR. Modeling early Epstein-Barr virus infection in Drosophila melanogaster: the BZLF1 protein. Genetics 2005; 171:1125 - 35; http://dx.doi.org/10.1534/genetics.105.042572; PMID: 16079238
- Adamson A, LaJeunesse D. A study of Epstein-Barr virus BRLF1 activity in a Drosophila model system. ScientificWorldJournal 2012; 2012:347597; http://dx.doi.org/10.1100/2012/347597; PMID: 22629134
- Lossius A, Johansen JN, Torkildsen Ø, Vartdal F, Holmøy T. Epstein-Barr virus in systemic lupus erythematosus, rheumatoid arthritis and multiple sclerosis—association and causation. Viruses 2012; 4:3701 - 30; http://dx.doi.org/10.3390/v4123701; PMID: 23342374
- Settles EW, Friesen PD. Flock house virus induces apoptosis by depletion of Drosophila inhibitor-of-apoptosis protein DIAP1. J Virol 2008; 82:1378 - 88; http://dx.doi.org/10.1128/JVI.01941-07; PMID: 17989181
- Jovel J, Schneemann A. Molecular characterization of Drosophila cells persistently infected with Flock House virus. Virology 2011; 419:43 - 53; http://dx.doi.org/10.1016/j.virol.2011.08.002; PMID: 21872290
- Jorge SA, Santos AS, Spina A, Pereira CA. Expression of the hepatitis B virus surface antigen in Drosophila S2 cells. Cytotechnology 2008; 57:51 - 9; http://dx.doi.org/10.1007/s10616-008-9154-z; PMID: 19003172
- Steinberg R, Shemer-Avni Y, Adler N, Neuman-Silberberg S. Human cytomegalovirus immediate-early-gene expression disrupts embryogenesis in transgenic Drosophila. Transgenic Res 2008; 17:105 - 19; http://dx.doi.org/10.1007/s11248-007-9136-5; PMID: 17912601
- Leulier F, Marchal C, Miletich I, Limbourg-Bouchon B, Benarous R, Lemaitre B. Directed expression of the HIV-1 accessory protein Vpu in Drosophila fat-body cells inhibits Toll-dependent immune responses. EMBO Rep 2003; 4:976 - 81; http://dx.doi.org/10.1038/sj.embor.embor936; PMID: 12973300
- Marchal C, Vinatier G, Sanial M, Plessis A, Pret AM, Limbourg-Bouchon B, Théodore L, Netter S. The HIV-1 Vpu protein induces apoptosis in Drosophila via activation of JNK signaling. PLoS One 2012; 7:e34310; http://dx.doi.org/10.1371/journal.pone.0034310; PMID: 22479597
- Hao L, Sakurai A, Watanabe T, Sorensen E, Nidom CA, Newton MA, Ahlquist P, Kawaoka Y. Drosophila RNAi screen identifies host genes important for influenza virus replication. Nature 2008; 454:890 - 3; http://dx.doi.org/10.1038/nature07151; PMID: 18615016
- Lam VK, Tokusumi T, Cerabona D, Schulz RA. Specific cell ablation in Drosophila using the toxic viral protein M2(H37A). Fly (Austin) 2010; 4:338 - 43; http://dx.doi.org/10.4161/fly.4.4.13114; PMID: 20798602
- Adamson AL, Chohan K, Swenson J, LaJeunesse D. A Drosophila model for genetic analysis of influenza viral/host interactions. Genetics 2011; 189:495 - 506; http://dx.doi.org/10.1534/genetics.111.132290; PMID: 21775472
- Habayeb MS, Cantera R, Casanova G, Ekström JO, Albright S, Hultmark D. The Drosophila Nora virus is an enteric virus, transmitted via feces. J Invertebr Pathol 2009; 101:29 - 33; http://dx.doi.org/10.1016/j.jip.2009.02.003; PMID: 19236875
- Habayeb MS, Ekström JO, Hultmark D. Nora virus persistent infections are not affected by the RNAi machinery. PLoS One 2009; 4:e5731; http://dx.doi.org/10.1371/journal.pone.0005731; PMID: 19478998
- Cordes EJ, Licking-Murray KD, Carlson KA. Differential gene expression related to Nora virus infection of Drosophila melanogaster. Virus Res 2013; 175:95 - 100; http://dx.doi.org/10.1016/j.virusres.2013.03.021; PMID: 23603562
- Wong SL, Chen Y, Chan CM, Chan CS, Chan PK, Chui YL, Fung KP, Waye MM, Tsui SK, Chan HY. In vivo functional characterization of the SARS-Coronavirus 3a protein in Drosophila. Biochem Biophys Res Commun 2005; 337:720 - 9; http://dx.doi.org/10.1016/j.bbrc.2005.09.098; PMID: 16212942
- Chan CM, Ma CW, Chan WY, Chan HY. The SARS-Coronavirus Membrane protein induces apoptosis through modulating the Akt survival pathway. Arch Biochem Biophys 2007; 459:197 - 207; http://dx.doi.org/10.1016/j.abb.2007.01.012; PMID: 17306213
- Chan E, Tsui S, Chan CM, Chan CS, Chen YW, Law P, Wong A. Molecular and genetic characterisation of the SARS coronavirus auxiliary protein X1 in Drosophila. Hong Kong Med J 2008; 14:Suppl 4 14 - 6; PMID: 18708668
- Kotadia S, Kao LR, Comerford SA, Jones RT, Hammer RE, Megraw TL. PP2A-dependent disruption of centrosome replication and cytoskeleton organization in Drosophila by SV40 small tumor antigen. Oncogene 2008; 27:6334 - 46; http://dx.doi.org/10.1038/onc.2008.254; PMID: 18663356
- Rose PP, Hanna SL, Spiridigliozzi A, Wannissorn N, Beiting DP, Ross SR, Hardy RW, Bambina SA, Heise MT, Cherry S. Natural resistance-associated macrophage protein is a cellular receptor for sindbis virus in both insect and mammalian hosts. Cell Host Microbe 2011; 10:97 - 104; http://dx.doi.org/10.1016/j.chom.2011.06.009; PMID: 21843867
- Xu J, Hopkins K, Sabin L, Yasunaga A, Subramanian H, Lamborn I, Gordesky-Gold B, Cherry S. ERK signaling couples nutrient status to antiviral defense in the insect gut. Proc Natl Acad Sci U S A 2013; 110:15025 - 30; http://dx.doi.org/10.1073/pnas.1303193110; PMID: 23980175
- Liu Q, Huang W, Nie J, Zhu R, Gao D, Song A, Meng S, Xu X, Wang Y. A novel high-throughput vaccinia virus neutralization assay and preexisting immunity in populations from different geographic regions in China. PLoS One 2012; 7:e33392; http://dx.doi.org/10.1371/journal.pone.0033392; PMID: 22438922
- Moser TS, Sabin LR, Cherry S. RNAi screening for host factors involved in Vaccinia virus infection using Drosophila cells. J Vis Exp 2010; 42:2137; PMID: 20834214
- Moussavi M, Tearle H, Fazli L, Bell JC, Jia W, Rennie PS. Targeting and killing of metastatic cells in the transgenic adenocarcinoma of mouse prostate model with vesicular stomatitis virus. Mol Ther 2013; 21:842 - 8; http://dx.doi.org/10.1038/mt.2012.285; PMID: 23337981
- Nakamoto M, Moy RH, Xu J, Bambina S, Yasunaga A, Shelly SS, Gold B, Cherry S. Virus recognition by Toll-7 activates antiviral autophagy in Drosophila. Immunity 2012; 36:658 - 67; http://dx.doi.org/10.1016/j.immuni.2012.03.003; PMID: 22464169
- Delgado MA, Elmaoued RA, Davis AS, Kyei G, Deretic V. Toll-like receptors control autophagy. EMBO J 2008; 27:1110 - 21; http://dx.doi.org/10.1038/emboj.2008.31; PMID: 18337753
- Schnettler E, Sterken MG, Leung JY, Metz SW, Geertsema C, Goldbach RW, Vlak JM, Kohl A, Khromykh AA, Pijlman GP. Noncoding flavivirus RNA displays RNA interference suppressor activity in insect and Mammalian cells. J Virol 2012; 86:13486 - 500; http://dx.doi.org/10.1128/JVI.01104-12; PMID: 23035235
- Munoz-Erazo L, Natoli R, Provis JM, Madigan MC, King NJ. Microarray analysis of gene expression in West Nile virus-infected human retinal pigment epithelium. Mol Vis 2012; 18:730 - 43; PMID: 22509103
- Chotkowski HL, Ciota AT, Jia Y, Puig-Basagoiti F, Kramer LD, Shi PY, Glaser RL. West Nile virus infection of Drosophila melanogaster induces a protective RNAi response. Virology 2008; 377:197 - 206; http://dx.doi.org/10.1016/j.virol.2008.04.021; PMID: 18501400
- Tzelepis I, Kapsetaki SE, Panayidou S, Apidianakis Y. Drosophila melanogaster: a first step and a stepping-stone to anti-infectives. Curr Opin Pharmacol 2013; 13:763 - 8; http://dx.doi.org/10.1016/j.coph.2013.08.003; PMID: 23992884
- Lemaitre B, Hoffmann J. The host defense of Drosophila melanogaster. Annu Rev Immunol 2007; 25:697 - 743; http://dx.doi.org/10.1146/annurev.immunol.25.022106.141615; PMID: 17201680
- Ferrandon D. The complementary facets of epithelial host defenses in the genetic model organism Drosophila melanogaster: from resistance to resilience. Curr Opin Immunol 2013; 25:59 - 70; http://dx.doi.org/10.1016/j.coi.2012.11.008; PMID: 23228366
- Apidianakis Y, Mindrinos MN, Xiao W, Tegos GP, Papisov MI, Hamblin MR, Davis RW, Tompkins RG, Rahme LG. Involvement of skeletal muscle gene regulatory network in susceptibility to wound infection following trauma. PLoS One 2007; 2:e1356; http://dx.doi.org/10.1371/journal.pone.0001356; PMID: 18159239
- Hamilos G, Samonis G, Kontoyiannis DP. Recent advances in the use of drosophila melanogaster as a model to study immunopathogenesis of medically important filamentous fungi. Int J Microbiol 2012; 2012:583792; http://dx.doi.org/10.1155/2012/583792; PMID: 22518146
- Wang JH, Valanne S, Rämet M. Drosophila as a model for antiviral immunity. World J Biol Chem 2010; 1:151 - 9; http://dx.doi.org/10.4331/wjbc.v1.i5.151; PMID: 21541000
- Schneider DS, Ayres JS. Two ways to survive infection: what resistance and tolerance can teach us about treating infectious diseases. Nat Rev Immunol 2008; 8:889 - 95; http://dx.doi.org/10.1038/nri2432; PMID: 18927577
- Davis MM, Engström Y. Immune response in the barrier epithelia: lessons from the fruit fly Drosophila melanogaster. J Innate Immun 2012; 4:273 - 83; http://dx.doi.org/10.1159/000332947; PMID: 22237424
- Kounatidis I, Ligoxygakis P. Drosophila as a model system to unravel the layers of innate immunity to infection. Open Biol 2012; 2:120075; http://dx.doi.org/10.1098/rsob.120075; PMID: 22724070
- Alarco AM, Marcil A, Chen J, Suter B, Thomas D, Whiteway M. Immune-deficient Drosophila melanogaster: a model for the innate immune response to human fungal pathogens. J Immunol 2004; 172:5622 - 8; PMID: 15100306
- Glittenberg MT, Kounatidis I, Christensen D, Kostov M, Kimber S, Roberts I, Ligoxygakis P. Pathogen and host factors are needed to provoke a systemic host response to gastrointestinal infection of Drosophila larvae by Candida albicans. Dis Model Mech 2011; 4:515 - 25; http://dx.doi.org/10.1242/dmm.006627; PMID: 21540243
- Apidianakis Y, Rahme LG. Drosophila melanogaster as a model host for studying Pseudomonas aeruginosa infection. Nat Protoc 2009; 4:1285 - 94; http://dx.doi.org/10.1038/nprot.2009.124; PMID: 19680242
- Fauvarque MO, Bergeret E, Chabert J, Dacheux D, Satre M, Attree I. Role and activation of type III secretion system genes in Pseudomonas aeruginosa-induced Drosophila killing. Microb Pathog 2002; 32:287 - 95; http://dx.doi.org/10.1006/mpat.2002.0504; PMID: 12137756
- Panayidou S, Apidianakis Y. Regenerative inflammation: Lessons from drosophila intestinal epithelium in health and disease. Pathogens 2013; 2:209 - 31; http://dx.doi.org/10.3390/pathogens2020209
- Hughes TT, Allen AL, Bardin JE, Christian MN, Daimon K, Dozier KD, Hansen CL, Holcomb LM, Ahlander J. Drosophila as a genetic model for studying pathogenic human viruses. Virology 2012; 423:1 - 5
- Castonguay-Vanier J, Vial L, Tremblay J, Déziel E. Drosophila melanogaster as a model host for the Burkholderia cepacia complex. PLoS One 2010; 5:e11467; http://dx.doi.org/10.1371/journal.pone.0011467; PMID: 20635002
- Akimana C, Kwaik YA. Francisella-arthropod vector interaction and its role in patho-adaptation to infect mammals. Front Microbiol 2011; 2:34; http://dx.doi.org/10.3389/fmicb.2011.00034; PMID: 21687425
- Vonkavaara M, Telepnev MV, Rydén P, Sjöstedt A, Stöven S. Drosophila melanogaster as a model for elucidating the pathogenicity of Francisella tularensis. Cell Microbiol 2008; 10:1327 - 38; http://dx.doi.org/10.1111/j.1462-5822.2008.01129.x; PMID: 18248629
- Reid DW, Muyskens JB, Neal JT, Gaddini GW, Cho LY, Wandler AM, Botham CM, Guillemin K. Identification of genetic modifiers of CagA-induced epithelial disruption in Drosophila. Front Cell Infect Microbiol 2012; 2:24; http://dx.doi.org/10.3389/fcimb.2012.00024; PMID: 22919616
- Dorer MS, Kirton D, Bader JS, Isberg RR. RNA interference analysis of Legionella in Drosophila cells: exploitation of early secretory apparatus dynamics. PLoS Pathog 2006; 2:e34; http://dx.doi.org/10.1371/journal.ppat.0020034; PMID: 16652170
- O’Connor TJ, Boyd D, Dorer MS, Isberg RR. Aggravating genetic interactions allow a solution to redundancy in a bacterial pathogen. Science 2012; 338:1440 - 4; http://dx.doi.org/10.1126/science.1229556; PMID: 23239729
- Dionne MS, Pham LN, Shirasu-Hiza M, Schneider DS. Akt and FOXO dysregulation contribute to infection-induced wasting in Drosophila. Curr Biol 2006; 16:1977 - 85; http://dx.doi.org/10.1016/j.cub.2006.08.052; PMID: 17055976
- Lau GW, Goumnerov BC, Walendziewicz CL, Hewitson J, Xiao W, Mahajan-Miklos S, Tompkins RG, Perkins LA, Rahme LG. The Drosophila melanogaster toll pathway participates in resistance to infection by the gram-negative human pathogen Pseudomonas aeruginosa. Infect Immun 2003; 71:4059 - 66; http://dx.doi.org/10.1128/IAI.71.7.4059-4066.2003; PMID: 12819096
- Limmer S, Haller S, Drenkard E, Lee J, Yu S, Kocks C, Ausubel FM, Ferrandon D. Pseudomonas aeruginosa RhlR is required to neutralize the cellular immune response in a Drosophila melanogaster oral infection model. Proc Natl Acad Sci U S A 2011; 108:17378 - 83; http://dx.doi.org/10.1073/pnas.1114907108; PMID: 21987808
- Stoltz DA, Ozer EA, Taft PJ, Barry M, Liu L, Kiss PJ, Moninger TO, Parsek MR, Zabner J. Drosophila are protected from Pseudomonas aeruginosa lethality by transgenic expression of paraoxonase-1. J Clin Invest 2008; 118:3123 - 31; http://dx.doi.org/10.1172/JCI35147; PMID: 18704198
- Sibley CD, Duan K, Fischer C, Parkins MD, Storey DG, Rabin HR, Surette MG. Discerning the complexity of community interactions using a Drosophila model of polymicrobial infections. PLoS Pathog 2008; 4:e1000184; http://dx.doi.org/10.1371/journal.ppat.1000184; PMID: 18949036
- Korgaonkar A, Trivedi U, Rumbaugh KP, Whiteley M. Community surveillance enhances Pseudomonas aeruginosa virulence during polymicrobial infection. Proc Natl Acad Sci U S A 2013; 110:1059 - 64; http://dx.doi.org/10.1073/pnas.1214550110; PMID: 23277552
- Bangi E, Pitsouli C, Rahme LG, Cagan R, Apidianakis Y. Immune response to bacteria induces dissemination of Ras-activated Drosophila hindgut cells. EMBO Rep 2012; 13:569 - 76; http://dx.doi.org/10.1038/embor.2012.44; PMID: 22498775
- Christofi T, Apidianakis Y. Ras-oncogenic Drosophila hindgut but not midgut cells use an inflammation-like program to disseminate to distant sites. Gut Microbes 2013; 4:54 - 9; http://dx.doi.org/10.4161/gmic.22429; PMID: 23060054
- Apidianakis Y, Que YA, Xu W, Tegos GP, Zimniak P, Hamblin MR, Tompkins RG, Xiao W, Rahme LG. Down-regulation of glutatione S-transferase α 4 (hGSTA4) in the muscle of thermally injured patients is indicative of susceptibility to bacterial infection. FASEB J 2012; 26:730 - 7; http://dx.doi.org/10.1096/fj.11-192484; PMID: 22038048
- Corby-Harris V, Habel KE, Ali FG, Promislow DE. Alternative measures of response to Pseudomonas aeruginosa infection in Drosophila melanogaster. J Evol Biol 2007; 20:526 - 33; http://dx.doi.org/10.1111/j.1420-9101.2006.01267.x; PMID: 17305818
- Ye YH, Chenoweth SF, McGraw EA. Effective but costly, evolved mechanisms of defense against a virulent opportunistic pathogen in Drosophila melanogaster. PLoS Pathog 2009; 5:e1000385; http://dx.doi.org/10.1371/journal.ppat.1000385; PMID: 19381251
- Liu X, Lu R, Xia Y, Wu S, Sun J. Eukaryotic signaling pathways targeted by Salmonella effector protein AvrA in intestinal infection in vivo. BMC Microbiol 2010; 10:326; http://dx.doi.org/10.1186/1471-2180-10-326; PMID: 21182782
- Brandt SM, Dionne MS, Khush RS, Pham LN, Vigdal TJ, Schneider DS. Secreted bacterial effectors and host-produced Eiger/TNF drive death in aSalmonella-infected fruit fly. PLoS Biol 2004; 2:e418; http://dx.doi.org/10.1371/journal.pbio.0020418; PMID: 15562316
- Ayres JS, Schneider DS. The role of anorexia in resistance and tolerance to infections in Drosophila. PLoS Biol 2009; 7:e1000150; http://dx.doi.org/10.1371/journal.pbio.1000150; PMID: 19597539
- Purdy AE, Watnick PI. Spatially selective colonization of the arthropod intestine through activation of Vibrio cholerae biofilm formation. Proc Natl Acad Sci U S A 2011; 108:19737 - 42; http://dx.doi.org/10.1073/pnas.1111530108; PMID: 22106284
- Berkey CD, Blow N, Watnick PI. Genetic analysis of Drosophila melanogaster susceptibility to intestinal Vibrio cholerae infection. Cell Microbiol 2009; 11:461 - 74; http://dx.doi.org/10.1111/j.1462-5822.2008.01267.x; PMID: 19046341
- Cocklin S, Jost M, Robertson NM, Weeks SD, Weber HW, Young E, Seal S, Zhang C, Mosser E, Loll PJ, et al. Real-time monitoring of the membrane-binding and insertion properties of the cholesterol-dependent cytolysin anthrolysin O from Bacillus anthracis. J Mol Recognit 2006; 19:354 - 62; http://dx.doi.org/10.1002/jmr.784; PMID: 16775845
- Bosco-Drayon V, Poidevin M, Boneca IG, Narbonne-Reveau K, Royet J, Charroux B. Peptidoglycan sensing by the receptor PGRP-LE in the Drosophila gut induces immune responses to infectious bacteria and tolerance to microbiota. Cell Host Microbe 2012; 12:153 - 65; http://dx.doi.org/10.1016/j.chom.2012.06.002; PMID: 22901536
- Blum JE, Fischer CN, Miles J, Handelsman J. Frequent replenishment sustains the beneficial microbiome of Drosophila melanogaster. MBio 2013; 4:e00860 - 13; http://dx.doi.org/10.1128/mBio.00860-13; PMID: 24194543
- Pezzulo AA, Hornick EE, Rector MV, Estin M, Reisetter AC, Taft PJ, Butcher SC, Carter AB, Manak JR, Stoltz DA, et al. Expression of human paraoxonase 1 decreases superoxide levels and alters bacterial colonization in the gut of Drosophila melanogaster. PLoS One 2012; 7:e43777; http://dx.doi.org/10.1371/journal.pone.0043777; PMID: 22952763
- Jones RM, Luo L, Ardita CS, Richardson AN, Kwon YM, Mercante JW, Alam A, Gates CL, Wu H, Swanson PA, et al. Symbiotic lactobacilli stimulate gut epithelial proliferation via Nox-mediated generation of reactive oxygen species. EMBO J 2013; 32:3017 - 28; http://dx.doi.org/10.1038/emboj.2013.224; PMID: 24141879
- Agaisse H, Burrack LS, Philips JA, Rubin EJ, Perrimon N, Higgins DE. Genome-wide RNAi screen for host factors required for intracellular bacterial infection. Science 2005; 309:1248 - 51; http://dx.doi.org/10.1126/science.1116008; PMID: 16020693
- Chambers MC, Song KH, Schneider DS. Listeria monocytogenes infection causes metabolic shifts in Drosophila melanogaster. PLoS One 2012; 7:e50679; http://dx.doi.org/10.1371/journal.pone.0050679; PMID: 23272066
- Needham AJ, Kibart M, Crossley H, Ingham PW, Foster SJ. Drosophila melanogaster as a model host for Staphylococcus aureus infection. Microbiology 2004; 150:2347 - 55; http://dx.doi.org/10.1099/mic.0.27116-0; PMID: 15256576
- Wu K, Conly J, Surette M, Sibley C, Elsayed S, Zhang K. Assessment of virulence diversity of methicillin-resistant Staphylococcus aureus strains with a Drosophila melanogaster infection model. BMC Microbiol 2012; 12:274; http://dx.doi.org/10.1186/1471-2180-12-274; PMID: 23176146
- Ben-Ami R, Watson CC, Lewis RE, Albert ND, Arias CA, Raad II, Kontoyiannis DP. Drosophila melanogaster as a model to explore the effects of methicillin-resistant Staphylococcus aureus strain type on virulence and response to linezolid treatment. Microb Pathog 2013; 55:16 - 20; http://dx.doi.org/10.1016/j.micpath.2012.11.012; PMID: 23232438
- Leone P, Bischoff V, Kellenberger C, Hetru C, Royet J, Roussel A. Crystal structure of Drosophila PGRP-SD suggests binding to DAP-type but not lysine-type peptidoglycan. Mol Immunol 2008; 45:2521 - 30; http://dx.doi.org/10.1016/j.molimm.2008.01.015; PMID: 18304640
- Christofi T, Apidianakis Y. Drosophila immune priming against Pseudomonas aeruginosa is short-lasting and depends on cellular and humoral immunity. F1000Res 2013; 2:76; PMID: 24358857
- Chambers MC, Lightfield KL, Schneider DS. How the fly balances its ability to combat different pathogens. PLoS Pathog 2012; 8:e1002970; http://dx.doi.org/10.1371/journal.ppat.1002970; PMID: 23271964
- Ben-Ami R, Lewis RE, Kontoyiannis DP. Enemy of the (immunosuppressed) state: an update on the pathogenesis of Aspergillus fumigatus infection. Br J Haematol 2010; 150:406 - 17; PMID: 20618330
- Lemaitre B, Nicolas E, Michaut L, Reichhart JM, Hoffmann JA. The dorsoventral regulatory gene cassette spätzle/Toll/cactus controls the potent antifungal response in Drosophila adults. Cell 1996; 86:973 - 83; http://dx.doi.org/10.1016/S0092-8674(00)80172-5; PMID: 8808632
- Berthier E, Lim FY, Deng Q, Guo CJ, Kontoyiannis DP, Wang CC, Rindy J, Beebe DJ, Huttenlocher A, Keller NP. Low-volume toolbox for the discovery of immunosuppressive fungal secondary metabolites. PLoS Pathog 2013; 9:e1003289; http://dx.doi.org/10.1371/journal.ppat.1003289; PMID: 23592999
- Moudgal V, Sobel J. Antifungals to treat Candida albicans. Expert Opin Pharmacother 2010; 11:2037 - 48; http://dx.doi.org/10.1517/14656566.2010.493875; PMID: 20536294
- Fidel PL Jr., Vazquez JA, Sobel JD. Candida glabrata: review of epidemiology, pathogenesis, and clinical disease with comparison to C. albicans. Clin Microbiol Rev 1999; 12:80 - 96; PMID: 9880475
- Mueller S, Gausson V, Vodovar N, Deddouche S, Troxler L, Perot J, Pfeffer S, Hoffmann JA, Saleh MC, Imler JL. RNAi-mediated immunity provides strong protection against the negative-strand RNA vesicular stomatitis virus in Drosophila. Proc Natl Acad Sci U S A 2010; 107:19390 - 5; http://dx.doi.org/10.1073/pnas.1014378107; PMID: 20978209
- Shelly S, Lukinova N, Bambina S, Berman A, Cherry S. Autophagy is an essential component of Drosophila immunity against vesicular stomatitis virus. Immunity 2009; 30:588 - 98; http://dx.doi.org/10.1016/j.immuni.2009.02.009; PMID: 19362021
- Razzell W, Wood W, Martin P. Swatting flies: modelling wound healing and inflammation in Drosophila. Dis Model Mech 2011; 4:569 - 74; http://dx.doi.org/10.1242/dmm.006825; PMID: 21810906
- Jensen RL, Pedersen KS, Loeschcke V, Ingmer H, Leisner JJ. Limitations in the use of Drosophila melanogaster as a model host for gram-positive bacterial infection. Lett Appl Microbiol 2007; 44:218 - 23; http://dx.doi.org/10.1111/j.1472-765X.2006.02040.x; PMID: 17257264