Abstract
S. typhimurium can infect host cells not only by the well-established “trigger”-mode of invasion, inducing the formation of prominent membrane ruffles e.g., as during macropinocytosis, but also independently of these processes. Recently, we have found that the novel, ruffling-independent entry mechanism usurps the host cell contraction machinery. This mode of entry involves formation of myosin II-rich stress fiber-like structures at invasion sites, likely through stimulation of a RhoA/Rho-kinase signaling pathway and mostly downstream of the Salmonella virulence factor SopB. Importantly, this pathway operates independently of Arp2/3 complex, a central regulator of the macropinocytic entry mode. Here, we will describe our current thinking of how the contraction-dependent uptake mechanism operates to promote Salmonella invasion, and which additional cellular or bacterial factors might get engaged in the process. Finally, we will speculate on the implications of these findings for invasion by other bacterial pathogens, and discuss their impact on the canonical trigger-vs.-zipper classification of entry mechanisms employed by distinct bacterial pathogens.
Keywords: :
The foodborne pathogen Salmonella enterica serovar Typhimurium is capable of infecting a variety of cell types, including phagocytes as well as non-phagocytic epithelial cells of the gut. For decades, Salmonella alongside with Shigella have been considered prototypic pathogens to enter host cells via the so-called “trigger” mechanism.Citation1,Citation2 This entry mechanism is accompanied by pathogen-induced stimulation of profound membrane ruffling as observed in macropinocytosis.Citation3 The diffuse ruffling surrounding invading bacteria phenotypically distinguishes this entry mechanism from the so called “zipper” mode of bacterial invasion, as used by Listeria, where membrane rearrangements and actin polymerization events are more confined to the bacterial contact and entry site. Thus, during “zipper” invasion, the host membrane extends tightly around the bacterium, which is mediated by virulence proteins on the pathogen surface binding to host cell receptors.Citation3 In contrast, membrane ruffling induced by “triggering” Salmonella is ignited by injection of bacterial virulence factors into the host cytosol employing a type III secretion system (TTSS).
A cocktail of injected Salmonella proteins stimulates activation of a central regulator of actin polymerization, the Arp2/3 complex. Notably, Arp2/3 complex is commonly considered to be essential for lamellipodia formation and membrane ruffling, based e.g., on RNAi studies and sequestration of the complex in the cytosol.Citation4-Citation6 Recently however, we explored Salmonella entry into host cells virtually incapable of membrane ruffling,Citation7 which—to our surprise—provided the first evidence that S. Typhimurium is also able to enter host cells in the absence of lamellipodia and ruffles.Citation8 These observations fitted the finding that Arp2/3 complex was also not essential for invasion, but careful comparison of entry efficiencies into host cells abrogated either for ruffling or Arp2/3 complex function revealed an additional layer of complexity. First, these analyses indicated that although Arp2/3 complex can contribute to ruffling-dependent entry, it has additional, ruffling-independent functions, and second, Salmonella can invade their hosts by yet another Arp2/3-independent entry mechanism. In a subsequent study, we have now characterized this novel Arp2/3 complex-independent invasion pathway, which critically relies on activation of myosin II as well as its upstream regulators RhoA and Rho-kinase.
Role and Activation of Myosin II in Salmonella Invasion
Our findings have unveiled a novel role for myosin II in Salmonella pathogenesis, adding up to the established function in intracellular positioning of the Salmonella-containing vacuole (SCV) following host cell entry.Citation9
But how does myosin II contribute to Salmonella engulfment? Bacterial association causes formation of actin filament- and myosin II-rich structures at sites of bacteria-host cell contact obviously resembling stress fibers.Citation10 Salmonella thus likely stimulates formation and contraction of these stress fiber-like structures below attached bacteria to eventually drive invagination of the host cell membrane and pulling of the bacteria into the host cell cytosol (). This conclusion is supported both by interference with entry upon inhibition of myosin II-based contractility in most cell types, as well as by the stimulation of entry upon induction of contractility.Citation10 Such a pulling mechanism certainly requires tight interaction of bacteria with the host cell membrane. Although this might involve multiple adhesive structures expressed by Salmonella species,Citation11 a recent study established that part of the TTSS, a complex consisting of three translocases (SipB, SipC and SipD) is essential for bacterial association with the host cell surface.Citation12
Figure 1. A novel mechanism of Salmonella invasion. (A) S. typhimurium injects a cocktail of bacterial virulence factors including SopE, SopE2 and SopB that exert different effects on the host cell actin cytoskeleton below the membrane through activation of members of the family of small Rho-GTPases. RhoG activated through SGEF and Rac1 and/or Cdc42 have so far been thought to drive Arp2/3 complex-mediated actin rearrangements mostly leading to bacterial engulfment by membrane ruffling. SopB, however, by an unknown mechanism (?) can also activate RhoA and its downstream effector Rho-kinase. (B) The canonical RhoA→Rho-kinase cascade mediates myosin activation through direct phosphorylation or inactivation of myosin light chain phosphatase, effecting formation of stress fiber-like structures indirectly linking to extracellular bacteria on one side and the cellular contractile machinery and/or focal adhesions on the other side. (C) Myosin II-dependent gliding along antiparallel actin filament arrays eventually drives the bacteria into the host cell cytosol without the need to generate engulfing sheets of ruffling membrane.
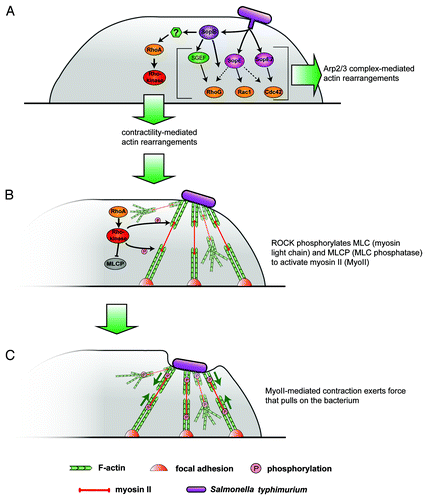
Internalization of S. typhimurium into host cells is mainly initiated then by translocation into the host cell cytosol of the bacterial effectors SopE, SopE2 and SopBCitation1 (). Among these, SopB appears to constitute the predominant activator of myosin II-mediated engulfment,Citation10 again in line with the regulatory role of SopB in myosin II-dependent SCV positioning.Citation9 Importantly, in reference Citation9, it was described that sole expression of SopB in Hela cells is sufficient to induce myosin II phosphorylation, as assessed both globally by protein gel blotting and at the single cell level using fluorescence microscopy. Although these authors concluded myosin not to operate in host cell entry, the data are fully consistent with our observation of SopB-mediated formation of contractile actin filament arrays effecting entry upon SopB delivery.Citation10 The absence of an observed role for myosin II in the engulfment process in this studyCitation9 is likely due to the choice of cell type, as we also failed to observe an effect of myosin II inhibition on entry of wild-type Salmonella in Hela cells. Moderate effects in this cell type were only seen when studying SopB-mediated invasion (infection with ΔsopE/E2—Salmonella). Profound suppression of SopB-mediated invasion by myosin II inhibition was obtained, however, when infecting fibroblasts or epithelial C127i and DU-145 cells. Of note, suppression of myosin II activity even increased entry into MDCK cells, for unknown reasons. Together, these observations indicate that activation of the RhoA/Rho-kinase/myosin II signaling axis by SopB must be indirect and involve host factors presumably expressed in a cell type-specific fashion.
Induction of Contractility: Just a Question of Choosing the Right GTPase
We have concluded that SopB links to myosin II activation through RhoA and its effector Rho-kinase (), but the signaling pathway is far from being completely understood. In particular, the signaling events accompanying RhoA activation by SopB remain to be identified and described. For instance, SopB has an intrinsic phosphatidylinositol phosphatase (PIPase) activity,Citation13 and SopB-generated phosphoinositides are known to induce activation of RhoG through the guanine nucleotide exchange factor (GEF) SGEF. The latter mediates RhoG-induced membrane rufflingCitation14 but likely not contractility, although weak induction of myosin phosphorylation was previously also observed upon ectopic expression of constitutively active RhoG.Citation9 It is tempting to speculate instead that SopB-derived phosphoinositide species may also talk to a GEF specific for RhoA, although the relevance of the PIPase activity of SopB in contractility-mediated invasion remains to be established.
When inspecting the literature, it is worthwhile to consider yet another myosin II activation pathway alongside the well-studied RhoA/Rho-kinase pathway.Citation15 This alternative pathway involves Cdc42-mediated activation of MRC kinase (MRCK), which in turn can also phosphorylate and thus activate myosin II.Citation16,Citation17 Again, ectopic Cdc42 expression was also able to partially activate myosin II phosphorylation or to restore SCV positioning in the absence of SopB.Citation9 Cdc42 is a known target of SopE/E2Citation18 but also thought to be activated during SopB-dependent invasion by unknown signaling events.Citation14 Interestingly, SopB has recently been shown to interact directly with Cdc42 to regulate intracellular Salmonella replication.Citation19 In spite of all these data, however, a significant contribution of Cdc42 to SopB-mediated activation of contractility and invasion remains unlikely, due to the following reasons: First, effects of RhoA or Rho-kinase inhibition were quantitatively comparable to suppression of myosin II activity,Citation10 indicating that a potential Cdc42/MRCK signaling pathway cannot efficiently substitute for the stimulation of myosin II activity upon interference with the RhoA/Rho-kinase pathway. Second, fibroblast cells genetically deficient for Cdc42Citation20 were equally efficient in SopB-mediated invasion as their Cdc42-expressing controls (unpublished data), precluding a predominant role for Cdc42 in SopB-induced myosin II activation and invasion, at least in the cell types and experimental settings used in our studies. Together, we conclude that SopB drives contractility in invasion mostly through RhoA.
Mechanisms of Stress Fiber Generation at Invasion Sites
How is the contractile machinery established underneath invading bacteria and how does it promote bacterial entry? Are actin filaments generated by de novo nucleation or are preformed filaments recruited to the entry site perhaps?
De novo generation of actin filaments at sites of invasion would per definition require the activity of actin filament nucleators, either of host cell or bacterial origin. Interestingly, the Salmonella virulence factor SipC was previously described to be capable of catalyzing actin filament nucleation.Citation21 However, SipC was unable to drive entry and to induce such structures in the absence of either Sop (isogenic mutant lacking Sops E, E2 and B), thus it seems unlikely that SipC alone can efficiently nucleate actin filaments that are subsequently bundled into functional stress fiber-like structures for invasion.Citation10 In addition, ectopic expression of SopB was sufficient to induce stress fiber formation in Hela cells in the absence of any other bacterial factor, thus potential accessory factors should derive from the host cell.Citation9 We can likely exclude Arp2/3 complex as potential actin filament nucleator of contractile entry structures, since this pathway also operated upon Arp2/3 RNAi.Citation10 Potential host cell nucleators involved instead certainly include members of the formin family. For instance, the RhoA effector and Diaphanous-related formin mDia1 has early been implicated in the formation of cellular stress fibers in cooperation with Rho-kinase-mediated myosin II phosphorylation.Citation22 However, mDia1 is not essential for stress fiber formation,Citation23 and the formin family in mammals comprises 15 members, including additional RhoA effectors.Citation24 Furthermore, recent years of research have unveiled an entire new class of actin nucleators, comprising a growing list of members such as Spire or Cordon-Bleu that assemble actin filaments using clusters of actin monomer binding modules.Citation25 Although involvement of some family members, e.g., the muscle protein Leiomodin,Citation26 seems unlikely due to tissue specificity, future efforts addressing this issue could first aim at narrowing down whether these actin filaments are generated by formin-dependent or -independent mechanisms, e.g., by employing general formin inhibitors.Citation27
Formation of robust stress fiber-like structures at entry sites at the membrane may also involve bundling proteins such as α-actinin,Citation22 but no data are available at present to determine whether the molecular inventory of bacteria-induced stress fibers at the membrane are by any means different from those anchoring the actin cytoskeleton in substrate adhesions. Future work will also have to address this question.
SopB-Induced Contraction vs. Membrane Ruffling
Importantly, SopB was originally described to induce local membrane ruffling instead of contraction.Citation14,Citation28 We don’t understand at present how SopB can target multiple signaling pathways simultaneously with such distinct cellular outcomes, and what precisely defines the nature of the “output response”—ruffling vs. contraction. One assumption would be that variable availability of distinct cellular SopB targets is at the ground of distinct behaviors observed in different cell lines, but no experimental evidence is available to date to directly prove this simple hypothesis. However, according to our data, a particular cell line may also be accessible to invasion via both, SopB-induced ruffling or contraction, as found e.g., for VA-13 fibroblasts,Citation10 although the formation of either ruffles or stress fibers at entry sites was mechanistically separable. Co-existence at entry sites does not necessarily imply co-regulation.
Thinking beyond the single cell level, the final outcome of SopB signaling to the actin cytoskeleton might also depend on local availability of downstream effectors at sites of bacterial attachment. In vivo, this may be of particular importance on different surfaces with differing molecular properties e.g., in polarized epithelial cells. Interestingly, previous findings already indicated that invasion of either the apical or the basolateral side of polarized epithelial cells may require separable molecular machineries. Rac1 for instance was proposed to be particularly important for entry at the apical but not basolateral side.Citation29 It is thinkable that the versatility of entry effectors and in particular of SopB has evolved due to an advantage derived from the capability to find multiple routes into cells of different tissues, physiological situations or even from different ‘angles.’
Myosin II-Dependent Processes Induced by Other Bacterial Pathogens
The diversity of invasion strategies observed for Salmonella raises the question whether entry mechanisms established for other pathogens might turn out only part of the story. Shigella flexneri, just like S. typhimurium, represents a model pathogen to enter host cells through the trigger mechanism of invasion. Significant homologies of Shigella virulence effectors, e.g., to those of Salmonella SopB, SipC or SipACitation30 raise the possibility that Shigella might also be able in principle to infect cells by means of ruffling-independent processes. Indeed, recent infection experiments performed in our lab indicated that suppression of membrane ruffling using WAVE complex knockdown does not abolish Shigella flexneri entry into fibroblasts (data not shown), as previously seen with S. typhimurium.Citation8 Furthermore, the Shigella effector IpgD shows sequence similarity to Salmonella SopBCitation19 and also generates phosphoinositide messengers to promote invasion,Citation31 indicating that Shigella IpgD might share functions with SopB. Finally, IpgD is thought to support membrane ruffling indirectly by decreasing the coupling strength of the actin network to the membrane,Citation32 but it might also serve additional, so far unrecognized functions.
One more direct hint toward exploitation of contraction-based mechanisms for entry arises from the activity of the effector protein IpgB2. It does not display obvious sequence homology to any Salmonella effector,Citation30 but shares overall structure with SopE and other bacterial GEFs.Citation33-Citation35 Strikingly, IpgB2 was reported to induce formation of stress fiber-like structures in host cells, presumably through activation of RhoA.Citation34-Citation36 Recently, IpgB2 was assigned a role in the invasion process, although only detectable in the absence of the related effector and Rac-GEF IpgB1.Citation34,Citation37 A potential relevance for entry utilizing contraction and/or stress fiber formation downstream of IpgB2 or other Shigella effectors thus remains to be elucidated.
Other bacterial pathogens that might well exploit myosin II-mediated contraction to infect host cells include Neisseria meningitides, already observed for instance to induce stress fibers during host cell invasion.Citation38 Finally, enteropathogenic E. coli (EPEC) have also been described to stimulate the formation of these structures underneath their attachment sites in a RhoA-dependent fashion,Citation39 employing yet another bacterial GEF for RhoA, EspM.Citation33 However, instead of entering the host, these bacteria usually surf on actin filament-filled cell surface pedestals,Citation40 so the relevance of contraction in this context remains unclear. We propose that the induction of contractile stress fibers accompanying early phases of bacterial infection will become more of a timely issue than previously anticipated.
Trigger vs. Zipper: Still a Valid Concept?
For many years, Salmonella have been considered to solely enter host cells via a macropinocytic uptake mode, the “trigger mechanism” of invasion.Citation3 However, evidence for a ruffling-independent, but Arp2/3 complex-dependent entry mode of S. Typhimurium, potentially involving the novel Arp2/3 activator WASH,Citation8,Citation41 as well as the newly described contraction-dependent entry pathwayCitation10 unravel a more complex situation. When looking at further Salmonella serovars, entry strategies in this genus turn out to be even more diversified. A recent interesting publication also describes a ruffling-independent invasion pathway, in this case for the Salmonella enterica serovar Enteritidis.Citation42 This entry pathway is again clearly separable from all Salmonella entry modes mentioned above, as it is unique in not being ignited through the injection of Salmonella effectors via the TTSS. Instead, it apparently operates independently of this apparatus and exploits the Salmonella surface protein Rck. This factor induces internalization with smallish membrane sheets wrapping around the bacterium, possibly through binding to a host cell surface receptor. Of note, this TTSS-independent invasion pathway was also found to be highly cell line-dependent,Citation43 indicating once again that multiple host cell types and/or conditions may be susceptible to Salmonella invasion due to various entry strategies. The Rck gene is also present in S. typhimurium,Citation42 but its potential relevance in invasion by this serovar has remained unknown.
Receptor engagement and discrete wrapping of the host cell membrane around the pathogen are usually considered to represent characteristics of the so called “zipper” mechanism of entry, employed for instance by Listeria monocytogenes. The zipper mode is usually regarded as the counterpart to the ruffling-dependent, trigger-type of invasion traditionally ascribed to Salmonella and Shigella.Citation3 Given the discovery of ruffling-independent invasion modes for different Salmonella strains, capable of operating both in parallel and independent from Rac-mediated membrane ruffling call for revision of the general concept of categorizing these different bacterial genera into “zippering” and “triggering” invaders.
Acknowledgments
This work was supported in part by institutional funds from the Helmholtz Society and grants from the Deutsche Forschungsgemeinschaft (to K.R. and T.E.B.S.). We are grateful to Julia Russ for sharing unpublished information.
References
- McGhie EJ, Brawn LC, Hume PJ, Humphreys D, Koronakis V. Salmonella takes control: effector-driven manipulation of the host. Curr Opin Microbiol 2009; 12:117 - 24; http://dx.doi.org/10.1016/j.mib.2008.12.001; PMID: 19157959
- Patel JC, Galan JE. Manipulation of the host actin cytoskeleton by Salmonella–all in the name of entry. Curr Opin Microbiol 2005; 8:10 - 5; http://dx.doi.org/10.1016/j.mib.2004.09.001; PMID: 15694851
- Cossart P, Sansonetti PJ. Bacterial invasion: the paradigms of enteroinvasive pathogens. Science 2004; 304:242 - 8; http://dx.doi.org/10.1126/science.1090124; PMID: 15073367
- Machesky LM, Insall RH. Scar1 and the related Wiskott-Aldrich syndrome protein, WASP, regulate the actin cytoskeleton through the Arp2/3 complex. Curr Biol 1998; 8:1347 - 56; http://dx.doi.org/10.1016/S0960-9822(98)00015-3; PMID: 9889097
- Nicholson-Dykstra SM, Higgs HN. Arp2 depletion inhibits sheet-like protrusions but not linear protrusions of fibroblasts and lymphocytes. Cell Motil Cytoskeleton 2008; 65:904 - 22; http://dx.doi.org/10.1002/cm.20312; PMID: 18720401
- Steffen A, Faix J, Resch GP, Linkner J, Wehland J, Small JV, et al. Filopodia formation in the absence of functional WAVE- and Arp2/3-complexes. Mol Biol Cell 2006; 17:2581 - 91; http://dx.doi.org/10.1091/mbc.E05-11-1088; PMID: 16597702
- Steffen A, Rottner K, Ehinger J, Innocenti M, Scita G, Wehland J, et al. Sra-1 and Nap1 link Rac to actin assembly driving lamellipodia formation. EMBO J 2004; 23:749 - 59; http://dx.doi.org/10.1038/sj.emboj.7600084; PMID: 14765121
- Hänisch J, Ehinger J, Ladwein M, Rohde M, Derivery E, Bosse T, et al. Molecular dissection of Salmonella-induced membrane ruffling versus invasion. Cell Microbiol 2010; 12:84 - 98; http://dx.doi.org/10.1111/j.1462-5822.2009.01380.x; PMID: 19732055
- Wasylnka JA, Bakowski MA, Szeto J, Ohlson MB, Trimble WS, Miller SI, et al. Role for myosin II in regulating positioning of Salmonella-containing vacuoles and intracellular replication. Infect Immun 2008; 76:2722 - 35; http://dx.doi.org/10.1128/IAI.00152-08; PMID: 18411289
- Hänisch J, Kölm R, Wozniczka M, Bumann D, Rottner K, Stradal TE. Activation of a RhoA/Myosin II-Dependent but Arp2/3 Complex-Independent Pathway Facilitates Salmonella Invasion. Cell Host Microbe 2011; 9:273 - 85; http://dx.doi.org/10.1016/j.chom.2011.03.009; PMID: 21501827
- Wagner C, Hensel M. Adhesive mechanisms of Salmonella enterica. Adv Exp Med Biol 2011; 715:17 - 34; http://dx.doi.org/10.1007/978-94-007-0940-9_2; PMID: 21557055
- Lara-Tejero M, Galan JE. Salmonella enterica serovar typhimurium pathogenicity island 1-encoded type III secretion system translocases mediate intimate attachment to nonphagocytic cells. Infect Immun 2009; 77:2635 - 42; http://dx.doi.org/10.1128/IAI.00077-09; PMID: 19364837
- Norris FA, Wilson MP, Wallis TS, Galyov EE, Majerus PW. SopB, a protein required for virulence of Salmonella dublin, is an inositol phosphate phosphatase. Proc Natl Acad Sci USA 1998; 95:14057 - 9; http://dx.doi.org/10.1073/pnas.95.24.14057; PMID: 9826652
- Patel JC, Galan JE. Differential activation and function of Rho GTPases during Salmonella-host cell interactions. J Cell Biol 2006; 175:453 - 63; http://dx.doi.org/10.1083/jcb.200605144; PMID: 17074883
- Rottner K, Stradal TE. Actin dynamics and turnover in cell motility. Curr Opin Cell Biol 2011; 23:569 - 78; http://dx.doi.org/10.1016/j.ceb.2011.07.003; PMID: 21807492
- Wilkinson S, Paterson HF, Marshall CJ. Cdc42-MRCK and Rho-ROCK signalling cooperate in myosin phosphorylation and cell invasion. Nat Cell Biol 2005; 7:255 - 61; http://dx.doi.org/10.1038/ncb1230; PMID: 15723050
- Leung T, Chen XQ, Tan I, Manser E, Lim L. Myotonic dystrophy kinase-related Cdc42-binding kinase acts as a Cdc42 effector in promoting cytoskeletal reorganization. Mol Cell Biol 1998; 18:130 - 40; PMID: 9418861
- Friebel A, Ilchmann H, Aepfelbacher M, Ehrbar K, Machleidt W, Hardt WD. SopE and SopE2 from Salmonella typhimurium activate different sets of RhoGTPases of the host cell. J Biol Chem 2001; 276:34035 - 40; http://dx.doi.org/10.1074/jbc.M100609200; PMID: 11440999
- Rodríguez-Escudero I, Ferrer NL, Rotger R, Cid VJ, Molina M. Interaction of the Salmonella Typhimurium effector protein SopB with host cell Cdc42 is involved in intracellular replication. Mol Microbiol 2011; 80:1220 - 40; http://dx.doi.org/10.1111/j.1365-2958.2011.07639.x; PMID: 21435037
- Czuchra A, Wu X, Meyer H, van Hengel J, Schroeder T, Geffers R, et al. Cdc42 is not essential for filopodium formation, directed migration, cell polarization, and mitosis in fibroblastoid cells. Mol Biol Cell 2005; 16:4473 - 84; http://dx.doi.org/10.1091/mbc.E05-01-0061; PMID: 16014609
- Hayward RD, Koronakis V. Direct modulation of the host cell cytoskeleton by Salmonella actin-binding proteins. Trends Cell Biol 2002; 12:15 - 20; http://dx.doi.org/10.1016/S0962-8924(01)02183-3; PMID: 11854005
- Pellegrin S, Mellor H. Actin stress fibres. J Cell Sci 2007; 120:3491 - 9; http://dx.doi.org/10.1242/jcs.018473; PMID: 17928305
- Peng J, Wallar BJ, Flanders A, Swiatek PJ, Alberts AS. Disruption of the Diaphanous-related formin Drf1 gene encoding mDia1 reveals a role for Drf3 as an effector for Cdc42. Curr Biol 2003; 13:534 - 45; http://dx.doi.org/10.1016/S0960-9822(03)00170-2; PMID: 12676083
- Schönichen A, Geyer M. Fifteen formins for an actin filament: a molecular view on the regulation of human formins. Biochim Biophys Acta 2010; 1803:152 - 63; http://dx.doi.org/10.1016/j.bbamcr.2010.01.014; PMID: 20102729
- Carlier MF, Husson C, Renault L, Didry D. Control of actin assembly by the WH2 domains and their multifunctional tandem repeats in Spire and Cordon-Bleu. Int Rev Cell Mol Biol 2011; 290:55 - 85; http://dx.doi.org/10.1016/B978-0-12-386037-8.00005-3; PMID: 21875562
- Chereau D, Boczkowska M, Skwarek-Maruszewska A, Fujiwara I, Hayes DB, Rebowski G, et al. Leiomodin is an actin filament nucleator in muscle cells. Science 2008; 320:239 - 43; http://dx.doi.org/10.1126/science.1155313; PMID: 18403713
- Rizvi SA, Neidt EM, Cui J, Feiger Z, Skau CT, Gardel ML, et al. Identification and characterization of a small molecule inhibitor of formin-mediated actin assembly. Chem Biol 2009; 16:1158 - 68; http://dx.doi.org/10.1016/j.chembiol.2009.10.006; PMID: 19942139
- Zhou D, Chen LM, Hernandez L, Shears SB, Galan JE. A Salmonella inositol polyphosphatase acts in conjunction with other bacterial effectors to promote host cell actin cytoskeleton rearrangements and bacterial internalization. Mol Microbiol 2001; 39:248 - 60; http://dx.doi.org/10.1046/j.1365-2958.2001.02230.x; PMID: 11136447
- Criss AK, Ahlgren DM, Jou TS, McCormick BA, Casanova JE. The GTPase Rac1 selectively regulates Salmonella invasion at the apical plasma membrane of polarized epithelial cells. J Cell Sci 2001; 114:1331 - 41; PMID: 11256999
- Parsot C. Shigella type III secretion effectors: how, where, when, for what purposes?. Curr Opin Microbiol 2009; 12:110 - 6; http://dx.doi.org/10.1016/j.mib.2008.12.002; PMID: 19157960
- Sansonetti P. Host-pathogen interactions: the seduction of molecular cross talk. Gut 2002; 50:Suppl 3 III2 - 8; http://dx.doi.org/10.1136/gut.50.suppl_3.iii2; PMID: 11953325
- Niebuhr K, Jouihri N, Allaoui A, Gounon P, Sansonetti PJ, Parsot C. IpgD, a protein secreted by the type III secretion machinery of Shigella flexneri, is chaperoned by IpgE and implicated in entry focus formation. Mol Microbiol 2000; 38:8 - 19; http://dx.doi.org/10.1046/j.1365-2958.2000.02041.x; PMID: 11029686
- Bulgin R, Raymond B, Garnett JA, Frankel G, Crepin VF, Berger CN, et al. Bacterial guanine nucleotide exchange factors SopE-like and WxxxE effectors. Infect Immun 2010; 78:1417 - 25; http://dx.doi.org/10.1128/IAI.01250-09; PMID: 20123714
- Huang Z, Sutton SE, Wallenfang AJ, Orchard RC, Wu X, Feng Y, et al. Structural insights into host GTPase isoform selection by a family of bacterial GEF mimics. Nat Struct Mol Biol 2009; 16:853 - 60; http://dx.doi.org/10.1038/nsmb.1647; PMID: 19620963
- Klink BU, Barden S, Heidler TV, Borchers C, Ladwein M, Stradal TE, et al. Structure of Shigella IpgB2 in complex with human RhoA: implications for the mechanism of bacterial guanine nucleotide exchange factor mimicry. J Biol Chem 2010; 285:17197 - 208; http://dx.doi.org/10.1074/jbc.M110.107953; PMID: 20363740
- Alto NM, Shao F, Lazar CS, Brost RL, Chua G, Mattoo S, et al. Identification of a bacterial type III effector family with G protein mimicry functions. Cell 2006; 124:133 - 45; http://dx.doi.org/10.1016/j.cell.2005.10.031; PMID: 16413487
- Hachani A, Biskri L, Rossi G, Marty A, Menard R, Sansonetti P, et al. IpgB1 and IpgB2, two homologous effectors secreted via the Mxi-Spa type III secretion apparatus, cooperate to mediate polarized cell invasion and inflammatory potential of Shigella flexenri. Microbes Infect 2008; 10:260 - 8; http://dx.doi.org/10.1016/j.micinf.2007.11.011; PMID: 18316224
- Slanina H, Konig A, Hebling S, Hauck CR, Frosch M, Schubert-Unkmeir A. Entry of Neisseria meningitidis into mammalian cells requires the Src family protein tyrosine kinases. Infect Immun 2010; 78:1905 - 14; http://dx.doi.org/10.1128/IAI.01267-09; PMID: 20176789
- Matsuzawa T, Kuwae A, Yoshida S, Sasakawa C, Abe A. Enteropathogenic Escherichia coli activates the RhoA signaling pathway via the stimulation of GEF-H1. EMBO J 2004; 23:3570 - 82; http://dx.doi.org/10.1038/sj.emboj.7600359; PMID: 15318166
- Campellone KG. Cytoskeleton-modulating effectors of enteropathogenic and enterohaemorrhagic Escherichia coli: Tir, EspFU and actin pedestal assembly. FEBS J 2010; 277:2390 - 402; http://dx.doi.org/10.1111/j.1742-4658.2010.07653.x; PMID: 20477869
- Rottner K, Hänisch J, Campellone KG. WASH, WHAMM and JMY: regulation of Arp2/3 complex and beyond. Trends Cell Biol 2010; 20:650 - 61; http://dx.doi.org/10.1016/j.tcb.2010.08.014; PMID: 20888769
- Rosselin M, Virlogeux-Payant I, Roy C, Bottreau E, Sizaret PY, Mijouin L, et al. Rck of Salmonella enterica, subspecies enterica serovar enteritidis, mediates zipper-like internalization. Cell Res 2010; 20:647 - 64; http://dx.doi.org/10.1038/cr.2010.45; PMID: 20368731
- Rosselin M, Abed N, Virlogeux-Payant I, Bottreau E, Sizaret PY, Velge P, et al. Heterogeneity of type III secretion system (T3SS)-1-independent entry mechanisms used by Salmonella Enteritidis to invade different cell types. Microbiology 2011; 157:839 - 47; http://dx.doi.org/10.1099/mic.0.044941-0; PMID: 21109565