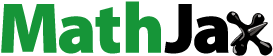
ABSTRACT
In this work, the structural and functional properties of proteins from black and yellow quinoa were assessed as well as the in vitro digestibility. Black quinoa flours contained higher amounts of crude fiber and lower amounts of total starch compared to the yellow counterpart. Structurally, electrophoretic SDS-PAGE bands corresponding to 50 kDa 11S globulin, and 55 kDa protein (under non-reducing conditions), and 31–33 kDa (under reducing conditions) were observed in both isolates. Fat absorption and water solubility indexes were comparatively lower in the black quinoa protein isolate. Both isolates showed a good nitrogen solubility index and emulsifying activity. In vitro protein digestibility was similar for both varieties (>95%). The addition of the protein isolates obtained from quinoa flours could be considered as a good alternative to increase the protein content of different foods taking advantage of key functional properties such as nitrogen solubility index and emulsifying activity.
RESUMEN
En este trabajo, las propiedades funcionales y estructurales de proteínas de quinoa negra y amarilla fueron evaluadas, así como su digestibilidad in vitro. Las harinas de quinoa negra tuvieron porcentajes de fibra cruda más altos y contenido de almidón total bajos comparado con las harinas de quinoa amarilla. Estructuralmente se observaron, en geles de SDS-PAGE, bandas de 50 kDa que corresponden a globulinas 11S, así como una proteína de 55 kDa (en condiciones no reductoras) y 31 a 33 kDa (en condiciones reductoras) para ambos aislados. Análisis de espectroscopía infrarroja con transformada de Fourier, mostraron una proporción relativamente alta de estructuras desordenadas. Los índices de absorción de grasa y solubilidad en agua fueron comparativamente más bajos en aislados proteicos de quinoa negra. Ambos aislados mostraron buenos índices de solubilidad de nitrógeno y actividad emulsificante. La digestibilidad proteica in vitro fue similar para ambas variedades (>95%). La adición de aislados proteicos obtenidos de harina de quinoa, podría ser considerada como una buena alternativa para aumentar el contenido proteico de diferentes alimentos, tomando ventaja de propiedades funcionales clave como índice de solubilidad de nitrógeno y actividad emulsificante.
1. Introduction
Pseudocereals are plants that produce seeds similar in function and composition to cereals (Alvarez-Jubete, Arendt, & Gallagher, Citation2010). Quinoa is a pseudocereal cultivated in Peru and Bolivia since ancient times by the Incas, and it is considered by FAO as one of the foods of the future, due to its capacity for agronomic adaptation (Toapanta, Carpio, Vilcacundo, & Carrillo, Citation2016). There are around 100 varieties of quinoa, among them red, black and yellow/white cultivars, being the last two the most produced in terms of volume (Arzapalo Quinto, Huamán Cóndor, Quispe Solano, & Espinoza Silva, Citation2015). Recently, quinoa has emerged because is a good source of different nutrients for the human diet, it has a high protein content (around 15%) and an excellent amino acid profile, close to the ideal balance recommended by FAO (Elsohaimy, Refaay, & Zaytoun, Citation2015). Quinoa has high amounts of lysine and methionine compared to other cereals and pseudocereals, such as maize and amaranth. Lysine content in quinoa seeds is above the FAO/WHO recommendation (5.5 g/100 g protein) (López, Galante, Robson, Boeris, & Spelzini, Citation2018). The main protein fractions include albumins and globulins (44–77% of total protein) with a low content of prolamines (0.5–0.7%) (Guerreo-Ochoa, Pedreschi, & Chirinos, Citation2015). These grains are prepared in different ways for direct consumption or quinoa based-foods production. Although they were once perceived as less nutritious and an incomplete source of essential amino acids, plant proteins are now viewed as a healthy alternative for meeting protein needs or recommendations for all ages (Richter, Skulas-Ray, Champagne, & Kris-Etherton, Citation2015). Protein isolates can be added as an ingredient to different foods to meet requirements of “functional foods” (Chuck-Hernández & Ozuna, Citation2019). Protein isolation is performed over the flour to obtain a sample containing a high concentration of protein. This procedure is achieved by the treatment of defatted or partially defatted flours with alcohol or diluted acid to solubilize carbohydrates, thus obtaining concentrated or isolated products. The use of this method to obtain protein isolates is commonly applied to soybean seeds, for other foods such as amaranth, quinoa or chia, fewer studies have been reported (Avila Ruiz, Xiao, van Boekel, Minor, & Stieger, Citation2016; Brinegar & Goundan, Citation1993). During the extraction of proteins and their isolation, these compounds may be induced to critical structural changes resulting from treatments with alkaline or acid solutions. Changes in protein structure also modify the functional properties of the extracted proteins. Hence, the study of the isolation conditions and the characterization of the obtained products is relevant to reduce the adverse effects that could occur as affectation in functional properties and digestibility (López et al., Citation2018). Moreover, it has been reported that the structure of proteins is influenced by several physiological properties of food. The study of the effects of specific conformational changes in the secondary structure of proteins and their effect on the functional, chemical and digestibility evaluation needs to be addressed (Vanga et al., Citation2015).
In this sense, yellow quinoa has been studied extensively by many researchers, and there is sufficient information about its characterization, the production of protein isolates, as well as their functional properties (Abugoch James, Citation2009; Avila Ruiz et al., Citation2016; Elsohaimy et al., Citation2015; Guerreo-Ochoa et al., Citation2015; Toapanta et al., Citation2016). Likewise, quinoa proteins can yield bioactive peptides after enzymatic hydrolysis and these short-chain and low molecular weight peptides possess higher potential as antihypertensive agents or as antioxidants (reducing the number of free radicals) (Aluko & Monu, Citation2003). However, to our knowledge there are no studies for black quinoa in terms of protein isolation and the physicochemical, functional and in vitro protein digestibility of the resulting isolates. Proteins extracted from black quinoa represent an opportunity in the food industry to be used as functional ingredients, through the production of isolates with high protein concentration and quality. This work aimed to obtain black quinoa protein isolates in order to further evaluate structural and functional properties and in vitro protein digestibility compared with the performance of yellow quinoa isolates (YQPI).
2. Materials and methods
2.1. Biological materials
Black (BQF) and yellow quinoa (YQF) flours were used as raw materials for protein extraction and were supplied by Campo Verde Company (Peru). These materials were kept in sealed polyethylene bags under refrigeration (5ºC) until their use.
2.2. Reagents
For protein extraction from quinoa flours, two solutions of NaOH (50% w/v) and HCl (15% v/v) were prepared (Desarrollo de Especialidades Químicas, García, N.L., Mexico). Enzymes used for in vitro protein digestibility (porcine pancreatic trypsin, T4799; bovine pancreatic chymotrypsin, C4129 and S. griseous protease type XIV, P5147) were purchased from Sigma-Aldrich.
2.3. Proximate analysis of yellow and black quinoa flours
Quantification of moisture, ash, crude fiber, total starch, and crude protein contents was carried out following the methods described by the AOAC (Citation1992) 925.10; 923.03; 962.09; 996.11; 978.02, respectively. Crude fat was performed using the 30–10.01 Official Method AACCI (Citation2000) with a slight modification due to the use of petroleum ether instead of ethyl ether. Nitrogen free extract (NFE) was calculated using Equation (1). All determinations were done in triplicate.
2.4. Extraction of quinoa proteins and production of isolates
Final extraction yield (PY, %) and solid yield (SY, %) were calculated for both, the BQF and YQF. The pH shifting method was applied for protein extraction (Salinas-Valdés, De la Rosa Millán, Serna-Saldivar, & Chuck-Hernández, Citation2015). Flours from yellow and black quinoa were passed through an 80 U.S. sieve and placed in separate containers. Then, distilled water was added in a 1:10 ratio and the pH of the solution was adjusted to 9.0 with NaOH (50% w/v). The mix was placed in a rotating incubator (RF 1575, VWR, OR, U.S.A.) at 50ºC and 70 rpm for 1 h. After this time, the solution was centrifuged (5804R, Eppendorf, Hamburg, Germany) at 10,000 rpm for 10 min at room temperature. The supernatant was poured into a beaker and a solution of HCl (15% v/v) was used to adjust the pH to 4.5. Thereafter, the suspension was centrifuged during 5 min at 10,000 rpm. The resultant products (black quinoa protein isolate, BQPI, and yellow quinoa protein isolate, YQPI) were adjusted to pH 7.0 using NaOH (50% w/v) and lyophilized (FreeZone series, LABCONCO) at −40°C and 0.200 MPa during 72h.
2.5. Structural characterization of yellow and black quinoa protein isolates (YQPI and BQPI)
2.5.1. Electrophoresis
Characterization of the BQPI and YQPI was performed by SDS-PAGE under reducing and non-reducing conditions. Protein isolates were dissolved in electrophoretic sample buffer [2% (w/v) SDS, 20% (v/v) glycerol, 0.01% (w/v) bromophenol blue, and 62.5 mM Tris-HCl pH 6.8]. Samples were heated in boiling water for 3 min and then loaded (15μg protein) in each well. Electrophoresis (Mini-Protean I cell, Bio-Rad) was conducted at 150 V until the tracking dye reached the bottom of the resolving gel consisting of acrylamide (12.5%, w/v). Protein bands were stained with Coomassie Brilliant Blue R-250 (Bio-Rad).
2.5.2. FTIR analysis
FTIR analysis was made in an ATR-FTIR (Perkin Elmer, Spectrum ONE, Norwalk, VA, USA). Data was collected in absorbance mode with a 4 cm−1 resolution and the spectra analyzed with Spectrum software (v.5.3.0) in the region from 1690 to 1600 cm−1 to evaluate the secondary structure (Vanga et al., Citation2015).
2.6. Functional evaluation of black and yellow quinoa protein isolates (BQPI and YQPI)
Water solubility (WSI), water absorption (WAI) and fat absorption (FAI) indexes were assayed according to Ochoa-Rivas, Nava-Valdez, Serna-Saldívar, and Chuck-Hernández (Citation2017). FAI was carried out using soybean oil (Nutrioli, Proteínas Naturales S.A. de C.V., Monterrey, N.L., Mexico). Analysis of nitrogen solubility index (NSI) was determined with the low-stir conventional method Ba 11–65 (AOCS, Citation2006). Finally, the emulsifying activity (EA) was obtained using the procedure described by Sánchez-Reséndiz et al. (Citation2018).
2.7. In vitro digestibility of yellow and black quinoa proteins isolates (YQPI and BQPI)
This determination was assessed using the following enzymes: porcine pancreatic trypsin type IX-S (T4799, Sigma Aldrich), bovine pancreatic chymotrypsin type II (C4129, Sigma Aldrich), and S. griseus protease type XIV (P5147, Sigma Aldrich) according to the procedure reported by Ochoa-Rivas et al. (Citation2017). Briefly: a 50 ml suspension containing 6.25 mg/ml of protein was prepared and adjusted to pH 8. Separately, a multi-enzymatic solution was prepared by mixing trypsin (1.6 mg/mL), chymotrypsin (3.1 mg/mL), and S. griseus protease (1.3 mg/mL). The protein solution was maintained in agitation, and 5 mL of the multi-enzymatic solution was added. The pH drop was recorded over a 10 min period using a potentiometer previously calibrated with standard pH solutions.
2.8. Statistical analysis
For all data gathered, an analysis of variance (ANOVA) was performed with a 95% level of confidence. Tukey’s tests (α < 0.05) were used to detect differences among treatments. Statistical analyses were performed with the Minitab Statistical Software (version 17, Minitab Ltd., Conventry, U.K.).
3. Results and discussion
3.1. Proximate analysis of yellow (YQF) and black quinoa (BQF) flours
depicts the results obtained from the proximate analysis of the two quinoa flours. In general, the BQF showed significantly higher values (p < 0.05) in four out of the seven parameters evaluated. Moisture content in the BQF was higher (p < 0.05) than YQF, however, the values in the two flours were lower than those reported by García-Salcedo, Torres-Vargas, and Ariza-Calderón (Citation2018) for quinoa seeds (11.64%), but similar to those described by Paucar-Menacho, Dueñas, Peñas, Frias, and Martínez-Villaluenga (Citation2018) (9.54%). These differences are given by the grain cultivars, the environmental conditions on the field, and the grain storage conditions.
Table 1. Proximal composition of black and yellow quinoa flours.
Tabla 1. Composición proximal de harinas de quinoa negra y amarilla.
Crude fiber and fat contents in the BQF were statistically higher compared to YQF and similar to values reported by Elsohaimy et al. (Citation2015) (4% crude fiber; and 6.8% crude fat). Crude fiber contents were considerably higher than values reported by Repo-Carrasco-Valencia and Serna (Citation2011) in four varieties of quinoa seeds (1.92–3.38%) that were extruded into high fiber and phenolic acids items. Crude fat content was significantly lower in the YQF compared to the BQF. Both flours showed considerably lower values in contrast to the value reported by Ogungbenle (Citation2003) of 9.50% for Canadian quinoa flour.
As expected, in the composition analysis of quinoa flours highlights the protein contents, where the YQF showed a higher value (p < 0.05) compared with the BQF. Importantly, both flours studied herein contained relatively higher amounts of protein than those reported by other authors for other varieties of quinoa flours, which ranged from 13.40% to 16.5% (Di Renzo, Reale, Boscaino, & Messia, Citation2018; Paucar-Menacho et al., Citation2018; Repo-Carrasco-Valencia & Serna, Citation2011). In general, the adequate protein content of the quinoa flours made them suitable raw materials for the extraction and manufacture of protein isolates with good nutritional quality.
Regarding total starch contents and NFE, both quinoa flours contained significantly lower quantities of these compounds when compared with other studies; however, the YQF had a statistically higher content of the two parameters evaluated. In quinoa grains, starch is the most important carbohydrate, and according to Vega-Gálvez et al. (Citation2010) total starch in this pseudocereal ranges from 58.1 to 64.2%, where 11% is comprised by linear amylose chains. In this sense, the BQF showed lower values, whereas the YQF contained similar amounts. Quinoa starch has excellent functional properties because of its resistance to retrogradation (Repo-Carrasco, Espinoza, & Jacobsen, Citation2003). Repo-Carrasco-Valencia and Serna (Citation2011) reported carbohydrates or NFE values between 75.76 and 78.86% in four quinoa varieties (“La Molina 89”, “Kcancolla”, “Blanca de Juli”, and “Sajama”) cultivated in Lima and Pruno, Peru. In a study conducted by Ogungbenle (Citation2003), nutritional evaluation was performed in Canadian quinoa seed. This author reported 53.3% of carbohydrates, similar to the values obtained in both the YQF and BQF. Besides, our results agree with Nascimento et al. (Citation2014), who found values between 51 and 61% for this type of pseudocereal.
Statistically, BQF showed a higher value of ash content than YQF. However, when compared with other studies, BQF and YQF contained twice the values of Canadian quinoa (1.2%) (Ogungbenle, Citation2003) and similar values (2.43%) to those obtained in saponin-free quinoa (Chenopodium quinoa var. Pasankalla) cultivated in Peru (Paucar-Menacho et al., Citation2018). This means that BQF and YQF could be considered as good sources of minerals rich in potassium, iron, calcium, and magnesium.
3.2. Extraction of proteins from BQF and YQF
shows the results of the protein extraction yield of BQF and YQF. There were no statistical differences in the final protein extraction yield (PY%) between flours; but for the case of solid yield (SY%), the YQF showed a higher value, indicating that some other compounds (non-protein based) were coextracted during the protein extraction step.
Table 2. Analysis of protein extraction from yellow and black quinoa flours.
Tabla 2. Análisis de extracción proteica de harinas de quinoa amarilla y negra.
Regarding PY%, our results are similar to the reported by Brinegar and Goundan (Citation1993), with 47% of final protein extraction, but lower in comparison with the 74.3% obtained by Avila Ruiz et al. (Citation2016) and the 76.3% reached by Guerreo-Ochoa et al. (Citation2015) at pH 11 using NaCl (0.1 N). In the production of protein isolates, several parameters such as protein content of the raw material, thermal treatment of the flour, chemical composition, as well as the conditions used during the extraction process can significantly influence production yields. Toapanta et al. (Citation2016) evaluated the effect of pH during the production of quinoa protein isolates. They evaluated five pH levels (2, 3, 4, 5, and 6) and found that at pH 2 and 3, the protein extraction increased. Thus, it is important to select the best raw materials and optimize the extraction conditions, so that the highest possible isolate yield is obtained.
As described by Föste, Elgeti, Brunner, Jekle, and Becker (Citation2015), alkaline extraction conditions alter the conformation of proteins exposing more hydrophobic groups by increasing interaction with water, thus promoting the solubility. According to these authors, extraction of proteins from quinoa at pH 10 is adequate, since higher pH levels negatively affect lysine bioavailability due to the generation of lysinoalanine, which reduces protein digestibility and biological value.
Protein extraction of BQF and YQF was carried out at pH 9, the PY% values of our samples were different from those described in a study where protein isolates were obtained from a Bolivian Organic Royal Quinoa (Chenopodium quinoa) by milling fractionation and solvent extraction (Föste et al., Citation2015). They reported protein extraction yield up to 71% on the white quinoa fraction, while in the bran fraction the yield was lower, ranging between 28.18 and 35.97%. However, the highest values of protein purity were quantified in the bran fractions.
Another factor affecting protein extraction of the quinoa flours was the lipid content, since a deffating step was not performed in the samples. Lipids are known to diminish protein extraction due to the formation of protein-lipid emulsions, even when lipids are present in low contents. In a study conducted by Ruiz et al. (Citation2016), protein isolates were obtained from deffated quinoa of the sweet cultivar Atlas harvested in Santiago, Chile. They described protein extraction yields from 24 to 37% when the procedure was conducted at pH 8 and 11, respectively. Furthermore, the protein purity values were high (90-93%) at these pH levels. Nevertheless, changes in the conformational structure need to be revised, since digestibility can be compromised by extreme alkaline conditions. Although we employed pH 9 and 4.5 during the key protein extraction steps, the PY% obtained from BQF and YQF were higher than the reported in deffated quinoa flours. A different trend of higher extraction yield of quinoa proteins was reported by Chauhan, Cui, and Eskin (Citation1999) employing seeds of cultivar D-407 Colorado Semi-Dwarf, grown in Rossburn, Manitoba. Extraction yields of proteins to obtain concentrates were in the range of 52% and 72.1% desaponized proteins. These values were higher than those found herein for the BQF and YQF.
3.3. Structural characterization of black (BQPI) and yellow (YQPI) quinoa protein isolates
3.3.1. Electrophoretic profile
In quinoa, albumins and globulins are the major protein fractions (Jancurová, Minarovicová, & Dandár, Citation2009). It is well-known that extraction methods change the chemical characteristics of proteins. To characterize the proteins profile in non-reducing and reducing conditions, SDS-PAGE electrophoresis was performed for BQPI and YQPI (). In the gel, bands with different molecular weights are depicted and agree with electrophoretic profiles previously published by different authors for other quinoa varieties (Alonso-Miravalles & O’Mahony, Citation2018). According to Avila Ruiz et al. (Citation2016), one of the protein fractions present in quinoa is 11S globulin, with a molecular weight of 50 kDa. On the other hand, Abugoch, Romero, Tapia, Silva, and Rivera (Citation2008) reported the presence of a 31–33 kDa chenoprotein and a 55 kDa protein, which correspond to globulins. Elsohaimy et al. (Citation2015) reported that the protein bands smaller than 20 kDa belong to albumin components. These bands were slightly more intense in the BQPI than in YQPI obtained under reducing conditions.
Figure 1. Electrophoresis (SDS-PAGE, 12.5%, Coomassie blue stained) of black and yellow quinoa protein under non-reducing (YQ and BQ) and reducing conditions (YQ-R and BQ-R). In reducing conditions for both isolates, bands with different molecular weights are appreciated, that show the existence of globulins in the range 20–23 and 51 kDa, chenoproteins (30–35 kDa) and albumins (10 kDa). STD. Molecular weight standard.
Figura 1. Gel de electroforesis (SDS-PAGE, 12.5%, teñido con azul de Coomassie) de proteína de quinoa negra y amarilla bajo condiciones no reductoras (YQ y BQ) y reductoras (YQ-R y BQ-R). En condiciones reductoras, para ambos aislados se observan bandas con diferente peso molecular que muestran la presencia de globulinas en el rango de 20 a 23 y 51 kDa, de chenoproteinas (30 a 35 kDa), y albúminas (10 kDa). STD es el estándar de peso molecular.
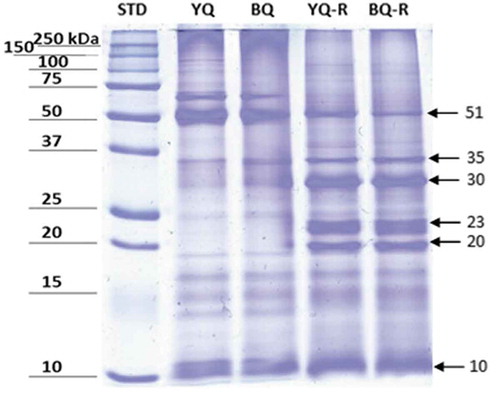
In this study, it was observed that BQPI and YQPI exhibited different protein patterns in non-reducing and reducing conditions, as previously described by other authors (Mäkinen, Zannini, Koehler, & Arendt, Citation2016; Valenzuela, Abugoch, Tapia, & Gamboa, Citation2013). It is evident that there is a lot of information about the study of quinoa proteins of different varieties; however, both in black and yellow varieties there are scarce data on their electrophoretic profile, so it is advisable to characterize these proteins in more detail with the aim of seeking the promotion of their use as functional ingredients.
Toapanta et al. (Citation2016), obtained protein isolates from quinoa first using alkaline pH followed by precipitation at different pH levels and analyzed resulting protein isolates with electrophoresis. They reported bands of molecular weights of 33–36 kDa, and 20–22 kDa corresponding to 11S AS and 11S AB globulins, respectively; similar to the profiles obtained herein for the BQPI and YQPI samples. According to Mäkinen et al. (Citation2016), chenopodin (an 11S type globulin) is the main protein contained in quinoa seeds. This protein with ~49 and 57 kDa subunits (AB-11S) is associated with a hexamer by non-covalent interactions. Each subunit consists of an acidic A-(Mr ≈28 and 34 kDa) and a basic B-(Mr ≈17 and 19 kDa) chain, that is linked by a disulfide bond, which agreed with our SDS-PAGE results.
It is important to highlight that one band at ≈70 kDa was detected in both protein isolates separated under non-reducing conditions. However, in the BQPI and YQPI obtained by reducing conditions this band was not observed likely to the composition and degree of the unfolding of protein isolates, which were regulated by specific or selected different combinations of extraction and pH precipitation.
Berti et al. (Citation2004) assessed the content of gliadin-like proteins in quinoa flours by immunochemical approaches to investigate if this pseudocereal was suitable to produce gluten-free foods. These authors evaluated protein fractions of Bolivian quinoa (Chenopodium quinoa Willd) and also fractionated proteins by sequential flour extraction. A SDS-PAGE under reducing conditions and fixed porosity (12% gel) along with immunoblotting was performed. Results indicated that there were non-covalently bound protein aggregates present in quinoa flours. Bands corresponding to 50 kDa 11S globulin, 31–33 kDa (chenoprotein) and a 55 kDa protein (under non-reducing conditions) were reported and were similar to the ones obtained in either BQPI and YQPI samples under reducing conditions. With these results, the authors concluded that quinoa flours and protein isolates could be a safe choice for the production of gluten-free products, at least from an immunological approach, since their immune reactivity was very low.
3.3.2. Analysis of secondary structures of quinoa protein isolates by FTIR
FTIR is used to characterize functional groups of molecules and, in the case of proteins, widely employed to determine their secondary structure. The repeating units in the protein usually produce nine characteristic IR absorption bands which are called A, B and I–VI, with the Amide I band (1700–1600 cm−1) being the more sensitive and used. This Amide I band is highly associated with the secondary structure of the proteins accounting for about 80% of the peptide linkages, i.e. C = O stretch (Vanga et al., Citation2015). Only a few reports are known to describe the secondary structure of quinoa proteins but apparently is very similar to amaranth counterpart, since both pseudo-cereals belong to the Caryophyllales order (Drzewiecki et al., Citation2003). shows the proportion of secondary structures present in BQPI and YQPI, obtained from the Amide I region. In general, results showed that α-helix and β-sheets (antiparallel) were the major structures in the two isolates, accounting for approximately 54-58%. The highest proportions in both isolates were associated with α-helix structures, indicating that there were no differences in the conformation of this particular structure. According to D’Amico, Schoenlechner, Tömösköszi, and Langó (Citation2017), there is a predominance of α-helix and low share of β-sheets due to low intensities of Amide III bands.
Table 3. Proportion of secondary structures on the Amide I region present in black (BQPI) and yellow (YQPI) quinoa protein isolates.
Tabla 3. Porcentaje de estructuras secundarias en la región de Amida I presentes en aislados proteicos de quinoa negra (BQPI) y amarilla (YQPI).
The β-sheets (antiparallel) structures give information about the backbone conformation independent of the amino acid sequence, its hydrophilic or hydrophobic properties, and charge of the quinoa protein isolates (Krimm & Bandekar, Citation1986). Apparently, the presence of parallel β-sheet structures is not usual in synthetic polypeptides and it was identified in both BQPI and YQPI (). Unfortunately, there is no current information to compare our results with other investigations. Therefore, further analyses should be performed.
Contrary to our results, D’Amico et al. (Citation2017), found by using circular dichroism spectroscopy, β-sheets as prevalent structures, while the α-helix were in low percentages. The proportion of β-sheet structures was similar in the BQPI and YQPI, and according to Vanga et al. (Citation2015), a high proportion of β-sheets enhances protein digestibility, which agrees with data reported in , where protein digestibility values in both quinoa isolates were similar and high.
The presence of loops (310-helix) was not found in BQPI nor YQPI. This structure differs from the α-helix in that the internal hydrogen bonding occurs between residues i and i + 3 instead of i and i + 4 in α-helices. It has been reported that quinoa polypeptides are rather randomly organized, and this agrees with the relatively high proportion of unordered structures in the BQPI and YQPI. For the aggregate structures, apparently there is a two-fold proportion in the BQPI, but this difference must be studied deeper before conclusions are made.
There are no data reported in the literature related to the profile of the secondary structure and digestibility of quinoa protein isolates. It could be assumed that the use of the BQPI and YQPI as ingredients in some foods may have a positive nutritional impact because isolates showed higher protein digestibility rates compared with other alternatives.
3.4. Functional properties of black and yellow quinoa protein isolates (BQPI and YQPI)
The functional properties of food biopolymers are important in product formulation and manufacture (Abugoch et al., Citation2008). Among the functional properties related to hydration or protein-water interaction, the WSI, WAI, and NSI were evaluated in BQPI and YQPI.
WSI is the number of solids that are recovered from the supernatant by putting the evaluated material in contact with a certain amount of water. This index is expressed as a percentage concerning the initial weight of the sample. The WSI of BQPI was statistically lower than YQPI (). Our results were lower compared with those reported for quinoa isolates obtained at pH 4 (25.59%) (Elsohaimy et al., Citation2015). It is likely that the observed difference is due to the fact that the quinoa used was not previously washed, thus, the saponins and lipids contained in the BQF and YQF affected this parameter.
Table 4. Functional evaluation and in-vitro digestibility of quinoa protein isolates.
Tabla 4. Evaluación funcional y digestibilidad in-vitro de aislados proteicos de quinoa.
According to Chauhan et al. (Citation1999), saponins affect the surface properties of quinoa proteins, thereby decreasing solubility. The pH as well as the thermal treatment used during extraction have important effects on solubility of quinoa proteins. Ogungbenle (Citation2003) reported solubility values between 15 and 52% on protein isolates obtained at pH 6 and pH 8, respectively, suggesting that proteins isolated at moderately acidic pH regions may be useful in the formulation of carbonated beverages; however, the values obtained in the BQPI and YQPI were comparatively lower and other potential applications should be studied. Similar low solubility values (around 5% at pH 4–5) were reported for quinoa protein concentrates obtained by an alkaline extraction method (Aluko & Monu, Citation2003).
WAI is an important parameter related to the content and type of proteins in foods, as well as to various factors such as particle size and the presence of fiber (Moure, Sineiro, Domínguez, & Parajó, Citation2006). WAI values did not show significant differences between BQPI and YQPI (). Similar values (3.0 g/g) were reported for quinoa isolates but were lower than desaponized protein isolates (5.7 g/g) (Chauhan et al., Citation1999).
Thus, BQPI and YQPI showed a water absorption behavior similar to the observed in quinoa and amaranth flours with values of 2.85 and 2.63 g/g, respectively. These low values are similar to chickpeas, lentils, soy, canola, and bean flours. WAI and water retention capacity in these materials (whole flours) depend on the content and distribution of macromolecules in the matrix, mainly starch, that traps water and gelatinizes, as well as water absorption by the fiber and dissociation of proteins. In protein isolates, this gives way to higher exposure of the polar groups in the peptide chains, thus, increasing the retention of the water molecules (García-Salcedo et al., Citation2018).
NSI is related to the hygroscopic property of proteins when dispersed in a NaCl solution and is used to provide a more concrete approach to the performance of proteins when added to foods. In , the NSI of BQPI and YQPI indicate that there were no statistical differences between the two protein isolates and the data was higher compared to values reported by Chauhan et al. (Citation1999) (10%) for proteins at pH 4.5. Another study conducted by Soria-Hernández, Serna-Saldívar, and Chuck-Hernández (Citation2015) reported similar NSI values of 27.61% for a soy-maize protein concentrate, but when the protein concentrate was hydrolyzed, the NSI values significantly increased. In this case, if BQPI and YQPI are required to have more solubility in a specific food matrix, a higher degree of hydrolysis in the isolates can be achieved.
It has been reported that vegetal proteins generally exhibit this pattern of low values of NSI at pH<4, and when pH is raised, it enhances solubility reaching the highest value at pH 10. This is due to conformational changes. Our results were similar with those reported in defatted chia (Salvia hispanica L.) rich protein fractions, where at pH 8, NSI values averaged 32.78% (Vázquez-Ovando, Betancur-Ancona, & Chel-Guerrero, Citation2013).
The functional hydration properties of BQPI and YQPI by extraction conditions and thermal treatments produced the aggregation of quinoa proteins and changed their surface hydrophobicity (López et al., Citation2018). These changes improved their functional properties and possible applications in different food products.
Fat absorption index (FAI) of a protein is expressed as the milliliters of oil that are retained for each gram of tested isolate. shows that the YQPI presented a higher FAI value. Both isolates were higher than 1.88 mL/g reported for protein isolates from four quinoa varieties by Elsohaimy et al. (Citation2015), and similar to Nasir Adnan, Pasha, Sadiq Butt, and Nawaz (Citation2015). These authors assayed FAI between 2.86 and 3.03 and attributed the differences mainly to the unique globular structure and particle size in the quinoa varieties and protein isolates, which was also related to the foaming capacity.
In soybean proteins, it has been reported that the fat binding capacity is enhanced by protein denaturation due to the exposure of non-polar amino acids. In protein isolates of Tepary beans, albumin has a FAI of 4.07 mL/g higher than the globulin (1.26 mL/g) counterpart. This finding is because the albumin fraction was structurally more lipophilic due to the presence of a large number of non-polar side chains and thus adsorbed more oil (Zayas, Citation1997). However, globulins and albumins constitute almost 75% of the overall protein associated to the quinoa isolates, which resulted in relatively low FAI values (Avila Ruiz et al., Citation2016).
This makes us conclude that FAI depends on the protein source and their fractions, so further analyses must be carried out in the BQPI and YQPI fractions to better understand their technological applications.
The emulsifying activity (EA) is the ability of a protein to bind two immiscible liquids, in which one of them is dispersed in the other to form and stabilize an emulsion (Lam & Nickerson, Citation2013; Soria-Hernández et al., Citation2015). The EA of BQPI and YQPI did not show significant differences (), indicating that these materials had acceptable stability 24 h after the mixture of oil, water, and protein was done.
According to L’hocine, Boye, and Arcand (Citation2006), higher EA values indicate the presence of more native proteins in the isolates. They evaluated the effect of different solvents and extraction processes for the extraction of proteins obtained from whole and defatted soybean flours. They also concluded that the modification of extraction processes (heat precipitation, acidic pretreatment and re-solubilization at pH 5–6) have a more significant effect on the EA compared to the different solvents used.
The EA of the BQPI and YQPI was higher in comparison to the 79% reported with similar materials (Chauhan et al., Citation1999). However, they showed low water solubility (4.26 and 4.94%, respectively) that limited their emulsifying capacity. Notwithstanding, EA in the isolates studied herein were quite high when compared with chickpea protein isolates (72.9–63.7%), soybean isolates (50.3%) and wheat proteins (40.8–43.8%), but similar to rice proteins isolates (74-85%) (Zayas, Citation1997).
3.5. In vitro protein digestibility of yellow and black quinoa isolates
Protein digestibility is defined as the ability of the organism to hydrolyze, absorb, and use amino acids that are linked by peptide bonds (de Jongh & Broersen, Citation2012). The digestibility of quinoa proteins isolates could be the limiting factor in protein utilization as a food ingredient. For BQPI and YQPI, the digestibilities were 95.71 and 95.80% (), respectively. These values were higher than the 78.37% reported for the same material (Elsohaimy et al., Citation2015), and higher than other grains such as wheat and rice. Similar results were found by Ruales and Nair (Citation1994) in proteins extracted from quinoa variety 40057, grown in Quito, Ecuador. In vitro digestibility rates of 77.7 and 83.3% were reported for raw and washed quinoa, respectively, but protein digestibility values up to 86.1% were reached for quinoa extruded at 120-150°C.
Lipids or fats contained in quinoa affect protein extraction and protein digestibility. Even when the flours used in this work were not defatted, the in vitro digestibility values in BQPI and YQPI were 20-22% higher than those obtained from protein samples of whole quinoa, and 27-21% higher compared to protein samples of defatted quinoa. Some authors attribute the low digestibility of vegetal proteins to antinutritional factors, such as trypsin inhibitors and phytic acid (Avilés‐Gaxiola, Chuck‐Hernández, & Serna Saldívar, Citation2018; Sant’Ana, Mendes, Pires, & Oliveira, Citation2011).
In vitro gastric protein digestibility was evaluated in protein isolates obtained from quinoa at different heat-treatments and various pH levels (8, 9, 10, and 11). The digestibility decreased with increasing pH extraction, which could be ascribed to protein aggregation/protein crosslinking. Protein digestibility of the quinoa protein isolates was higher compared to wholemeal quinoa flour. This indicates how the nutritional value of quinoa protein isolates could be influenced by heat processing, protein extraction conditions, and other grain components, such as lipids and carbohydrates (Ruiz et al., Citation2016).
Similar findings were reported by Vilcacundo et al. (Citation2017) for in vitro gastrointestinal digestion of quinoa protein concentrates. They indicated that at pH levels of 4.5 and 5.5, pepsin decreased significantly its activity; and after subsequent incubation with pancreatic enzymes, quinoa proteins were totally hydrolyzed. This indicates the high digestibility of these isolates under conditions simulating physiological digestion. The high protein digestibility obtained under reducing conditions of both BQPI and YQ makes these sources an excellent option to improve human health and be used as novel ingredients for the food industry.
The effect of saponins content on the in vitro digestibility of quinoa proteins (variety 40057, Quito, Ecuador) was evaluated by Ruales and Nair (Citation1994). Saponins could have negative effects during the digestibility of quinoa seeds. They reported digestibility rates of 83.3% for washed quinoa aimed to remove saponins and 77.7% for raw quinoa. These values were lower when compared with those for BQPI and YQPI samples. Therefore, it is assumed that leftover saponins present in the isolates did not interfere with the protein digestibility.
In this context, the quinoa cultivars used herein had higher in vitro digestibility rates than protein isolates extracted from four genotypes of grains (V7, V2, V9, and V1) grown in Faisalabad, Pakistan and analyzed by Nasir Adnan et al. (Citation2015). These cultivars had protein digestibilities between 75.95 and 78.11%. The extraction of proteins to produce isolates removed fiber components and inhibitors that negatively interfered with digestive enzymes. Therefore, the high in vitro protein digestibility rates of BQPI and YQPI are similar or even better than animal proteins like meat, casein, eggs and fish.
4. Conclusions
The black and yellow quinoa flours showed a high protein percentage when compared with other pseudocereals. There is no sufficient information about structural characterization by FTIR on quinoa protein isolates, and further analysis must be considered. Functional properties of the BQPI and YQPI isolates are considered adequate as additives for the food industry (i.e. production of gluten-free foods) and to improve their nutritional quality. Both isolates presented an excellent in vitro digestibility (>95%). Black quinoa protein isolates are a promising source of functional proteins and the evaluation of their nutritional quality as amino acid profile, saponin concentration and in vivo digestibility is desirable for the following stages of research.
Acknowledgments
Authors acknowledge the Research Center for Protein Development (CIDPRO) at Tecnológico de Monterrey for their support with the facilities and equipment.
Disclosure statement
The authors declare that they have no known competing financial interests or personal relationships that could have appeared to influence the work reported in this paper.
References
- AACCI. (2000). Approved methods of the AACC (10th ed.). A. A. Of & C. Chemists Eds., St. Paul, MN: American Association of Cereal Chemists International.
- Abugoch James, L. E. (2009). Chapter 1. Quinoa (Chenopodium quinoa Willd.): Composition, chemistry, nutritional, and functional properties. Advances in Food and Nutrition Research, 58, 1–31. doi:10.1016/S1043-4526(09)58001-1
- Abugoch, L. E., Romero, N., Tapia, C. A., Silva, J., & Rivera, M. (2008). Study of some physicochemical and functional properties of quinoa (Chenopodium quinoa Willd) protein isolates. Journal of Agricultural and Food Chemistry, 56(12), 4745–4750. doi:10.1021/jf703689u
- Alonso-Miravalles, L., & O’Mahony, J. A. (2018). Composition, protein profile and rheological properties of pseudocereal-based protein-rich ingredients. Foods (Basel, Switzerland), 7(5), 73. doi:10.3390/foods7050073
- Aluko, R. E., & Monu, E. (2003). Functional and bioactive properties of quinoa seed protein hydrolysates. Journal of Food Science, 68(4), 1254–1258. doi:10.1111/j.1365-2621.2003.tb09635.x
- Alvarez-Jubete, L., Arendt, E. K., & Gallagher, E. (2010). Nutritive value of pseudocereals and their increasing use as functional gluten-free ingredients. Trends in Food Science & Technology, 21, 106–113. doi:10.1016/j.tifs.2009.10.014
- AOAC. (1992). Association of official analytical chemists. Official methods 962.09, 978.02, 925.10, 923.03. Crude fiber determination, total nitrogen content, moisture content, ash content. Washington, DC: Association of Official Analytical Chemists.
- AOCS. (2006). Official and tentative methods of the American Oil Chemists Society. American Oil Chemist’s Society (AOCS).Urbana, IL, EU.
- Arzapalo Quinto, D., Huamán Cóndor, K., Quispe Solano, M., & Espinoza Silva, C. (2015). Extracción y caracterización del almidón de tres variedades de quinua (Chenopodium quinoa Willd) negra collana, pasankalla roja y blanca junín. Revista De La Sociedad Química Del Perú, 81(1), 44–54.
- Avila Ruiz, G., Xiao, W., van Boekel, M., Minor, M., & Stieger, M. (2016). Effect of extraction pH on heat-induced aggregation, gelation and microstructure of protein isolate from quinoa (Chenopodium quinoa Willd). Food Chemistry, 209, 203–210. doi:10.1016/j.foodchem.2016.04.052
- Avilés‐Gaxiola, S., Chuck‐Hernández, C., & Serna Saldívar, S. O. (2018). Inactivation methods of trypsin inhibitor in legumes: A review. Journal of Food Science, 83(1), 17–29. doi:10.1111/1750-3841.13985
- Berti, C., Ballabio, C., Restani, P., Porrini, M., Bonomi, F., & Iametti, S. (2004). Immunochemical and molecular properties of proteins in Chenopodium quinoa. Cereal Chemistry, 81(2), 275–277. doi:10.1094/CCHEM.2004.81.2.275
- Brinegar, C., & Goundan, S. (1993). Isolation and characterization of chenopodin, the 11S seed storage protein of quinoa (Chenopodium quinoa). Journal of Agricultural and Food Chemistry, 41, 182–185. doi:10.1021/jf00026a006
- Chauhan, G. S., Cui, W., & Eskin, N. A. M. (1999). Effect of saponin on the surface properties of quinoa proteins. International Journal of Food Properties, 2(1), 13–22. doi:10.1080/10942919909524586
- Chuck-Hernández, C., & Ozuna, C. (2019). Protein isolates from meat processing by-products. In C. Galanakis (Ed.), Proteins: Sustainable sources, processing and applications (1st ed., pp. 131–162). United Kingdom: Academic Press. doi: 10.1016/B978-0-12-816695-6.00005-2.
- D’Amico, S., Schoenlechner, R., Tömösköszi, S., & Langó, B. (2017). Proteins and amino acids of kernels. In C. M. Haros & R. Schoenlechner (Eds.), Pseudocereals: Chemistry and Technology (1st ed., pp. 94–116). Oxford, UK: John Wiley & Sons, Ltd.
- de Jongh, H. H. J., & Broersen, K. (2012). Application potential of food protein modification. In Z. Nawaz & S. Naveed (Eds.), Advances in chemical engineering (pp. 584). London, UK: Intech Open.
- Di Renzo, T., Reale, A., Boscaino, F., & Messia, M. C. (2018). Flavoring production in kamut®, quinoa and wheat doughs fermented by Lactobacillus paracasei, Lactobacillus plantarum, and Lactobacillus brevis: A SPME-GC/MS study. Frontiers in Microbiology, 9, 429. doi: 10.3389/fmicb.2018.00429.
- Drzewiecki, J., Delgado-Licon, E., Haruenkit, R., Pawelzik, E., Martin-Belloso, O., Park, Y.-S., … Gorinstein, S. (2003). Identification and differences of total proteins and their soluble fractions in some pseudocereals based on electrophoretic patterns. Journal of Agricultural and Food Chemistry, 51(26), 7798–7804. doi:10.1021/jf030322x
- Elsohaimy, S. A., Refaay, T. M., & Zaytoun, M. A. M. (2015). Physicochemical and functional properties of quinoa protein isolate. Annals of Agricultural Science, 60(2), 297–305. doi:10.1016/j.aoas.2015.10.007
- Föste, M., Elgeti, D., Brunner, A.-K., Jekle, M., & Becker, T. (2015). Isolation of quinoa protein by milling fractionation and solvent extraction. Food and Bioproducts Processing, 96, 20–26. doi:10.1016/J.FBP.2015.06.003
- García-Salcedo, Á. J., Torres-Vargas, O. L., & Ariza-Calderón, H. (2018). Physical-chemical characterization of quinoa (Chenopodium quinoa Willd.), amaranth (Amaranthus caudatus L.), and chia (Salvia hispanica L.) flours and seeds. Acta Agronómica, 67, 215–222. doi:10.15446/acag.v67n2.63666
- Guerreo-Ochoa, M. R., Pedreschi, R., & Chirinos, R. (2015). Optimised methodology for the extraction of protein from quinoa (Chenopodium quinoa Willd.). International Journal of Food Science and Technology, 50, 1815–1822. doi:10.1111/ijfs.12834
- Jancurová, M., Minarovicová, L., & Dandár, A. (2009). Quinoa- A review. Czech Journal of Food Science, 27(2), 71–79. doi:10.17221/32/2008-CJFS
- Krimm, S., & Bandekar, J. (1986). Vibrational spectroscopy and conformation of peptides, polypeptides, and proteins. Advances in Protein Chemistry, 38, 181–364. doi:10.1016/S0065-3233(08)60528-8
- L’hocine, L., Boye, J. I., & Arcand, Y. (2006). Composition and functional properties of soy protein isolates prepared using alternative defatting and extraction procedures. Journal of Food Science, 71(3), C137–C145. doi:10.1111/j.1365-2621.2006.tb15609.x
- Lam, R. S. H., & Nickerson, M. T. (2013). Food proteins: A review on their emulsifying properties using a structure-function approach. Food Chemistry, 141(2), 975–984. doi:10.1016/j.foodchem.2013.04.038
- López, D. N., Galante, M., Robson, M., Boeris, V., & Spelzini, D. (2018). Amaranth, quinoa and chia protein isolates: Physicochemical and structural properties. International Journal of Biological Macromolecules, 109, 152–159. doi:10.1016/J.IJBIOMAC.2017.12.080
- Mäkinen, O. E., Zannini, E., Koehler, P., & Arendt, E. K. (2016). Heat-denaturation and aggregation of quinoa (Chenopodium quinoa) globulins as affected by the pH value. Food Chemistry, 196, 17–24. doi:10.1016/J.FOODCHEM.2015.08.069
- Moure, A., Sineiro, J., Domínguez, H., & Parajó, J. C. (2006). Functionality of oilseed protein products: A review. Food Research International, 39, 945–963. doi:10.1016/j.foodres.2006.07.002
- Nascimento, A. C., Mota, C., Coelho, I., Gueifão, S., Santos, M., Matos, A. S., … Castanheira, I. (2014). Characterisation of nutrient profile of quinoa (Chenopodium quinoa), amaranth (Amaranthus caudatus), and purple corn (Zea mays L.) consumed in the North of Argentina: Proximates, minerals and trace elements. Food Chemistry, 148, 420–426. doi:10.1016/j.foodchem.2013.09.155
- Nasir Adnan, M., Pasha, I., Sadiq Butt, M., & Nawaz, H. (2015). Biochemical characterization of quinoa with special reference to its protein quality. Pakistan Journal of Agricuturural Sciences, 52(3), 731–737.
- Ochoa-Rivas, A., Nava-Valdez, Y., Serna-Saldívar, S. O., & Chuck-Hernández, C. (2017). Microwave and ultrasound to enhance protein extraction from peanut flour under alkaline conditions: Effects in yield and functional properties of protein isolates. Food and Bioprocess Technology, 10(3), 543–555. doi:10.1007/s11947-016-1838-3
- Ogungbenle, H. N. (2003). Nutritional evaluation and functional properties of quinoa (Chenopodium quinoa) flour. International Journal of Food Sciences and Nutrition, 54(2), 153–158. doi:10.1080/0963748031000084106
- Paucar-Menacho, L. M., Dueñas, M., Peñas, E., Frias, J., & Martínez-Villaluenga, C. (2018). Effect of dry heat puffing on nutritional composition, fatty acid, amino acid and phenolic profiles of pseudocereals grains. Polish Journal of Food and Nutrition Sciences, 68(4), 289–297. doi:10.1515/pjfns-2018-0005
- Repo-Carrasco, R., Espinoza, C., & Jacobsen, S. E. (2003). Nutritional value and use of the Andean crops quinoa (Chenopodium quinoa) and kañiwa (Chenopodium pallidicaule). Food Reviews International, 19(1–2), 179–189. doi:10.1081/FRI-120018884
- Repo-Carrasco-Valencia, R. A.-M., & Serna, L. A. (2011). Quinoa (Chenopodium quinoa Willd.) as a source of dietary fiber and other functional components. Ciência Y Tecnologia Alimentaria Campinas, 31(1), 225–230. doi:10.1590/S0101-20612011000100035
- Richter, C. K., Skulas-Ray, A. C., Champagne, C. M., & Kris-Etherton, P. M. (2015). Plant protein and animal proteins: Do they differentially affect cardiovascular disease risk? Advances in Nutrition (bethesda, Md.), 6(6), 712–728. doi:10.3945/an.115.009654
- Ruales, J., & Nair, B. M. (1994). Effect of processing on in vitro digestibility of protein and starch in quinoa seeds. International Journal of Food Science & Technology, 29(4), 449–456. doi:10.1111/j.1365-2621.1994.tb02086.x
- Ruiz, G. A., Opazo-Navarrete, M., Meurs, M., Minor, M., Sala, G., van Boekel, M., … Janssen, A. E. M. (2016). Denaturation and in vitro gastric digestion of heat-treated quinoa protein isolates obtained at various extraction pH. Food Biophysics, 11(2), 184–197. doi:10.1007/s11483-016-9429-4
- Salinas-Valdés, A., De la Rosa Millán, J., Serna-Saldivar, S. O., & Chuck-Hernández, C. (2015). Yield and textural characteristics of Panela cheeses produced with dairy-vegetable protein (soybean or peanut) blends supplemented with transglutaminase. Journal of Food Science, 80(12), S2950–S2956. doi:10.1111/1750-3841.13126
- Sánchez-Reséndiz, A., Rodríguez-Barrientos, S., Rodríguez-Rodríguez, J., Barba-Dávila, B., Serna-Saldívar, S. O., & Chuck-Hernández, C. (2018). Phosphoesterification of soybean and peanut proteins with sodium trimetaphosphate (STMP): Changes in structure to improve functionality for food applications. Food Chemistry, 260. doi:10.1016/j.foodchem.2018.04.009
- Sant’Ana, R. D. C. O., Mendes, F. Q., Pires, C. V., & Oliveira, M. G. D. A. (2011). Influence of lipid extraction from different protein sources on in vitro digestibility. Ciência E Agrotecnologia, 35(4), 758–764. doi:10.1590/S1413-70542011000400015
- Soria-Hernández, C., Serna-Saldívar, S., & Chuck-Hernández, C. (2015). Physicochemical and functional properties of vegetable and cereal proteins as potential sources of novel food ingredients. Food Technology & Biotechnology, 53(3), 269–277. doi:10.17113/ftb
- Toapanta, A., Carpio, C., Vilcacundo, R., & Carrillo, W. (2016). Analysis of protein isolate from quinoa (Chenopodium quinoa willd). Asian Journal of Pharmaceutical and Clinical Research, 9(2), 332–334.
- Valenzuela, C., Abugoch, L., Tapia, C., & Gamboa, A. (2013). Effect of alkaline extraction on the structure of the protein of quinoa (Chenopodium quinoa Willd.) and its influence on film formation. International Journal of Food Science & Technology, 48(4), 843–849. doi:10.1111/ijfs.12035
- Vanga, S. K., Singh, A., Kalkan, F., Gariepy, Y., Orsat, V., & Raghavan, V. (2015). Effect of thermal and high electric fields on secondary structure of peanut protein. International Journal of Food Properties, 19, 1259–1271. doi:10.1080/10942912.2015.1071841
- Vázquez-Ovando, A., Betancur-Ancona, D., & Chel-Guerrero, L. (2013). Physicochemical and functional properties of a protein-rich fraction produced by dry fractionation of chia seeds (Salvia hispanica L.). CyTA - Journal of Food, 11(1), 75–80. doi:10.1080/19476337.2012.692123
- Vega-Gálvez, A., Miranda, M., Vergara, J., Uribe, E., Puente, L., & Martínez, E. A. (2010). Nutrition facts and functional potential of quinoa (Chenopodium quinoa willd.), an ancient Andean grain: A review. Journal of the Science of Food and Agriculture, 90(15), 2541–2547. doi:10.1002/jsfa.4158
- Vilcacundo, R., Barrio, D., Carpio, C., García-Ruiz, A., Rúales, J., Hernández-Ledesma, B., & Carrillo, W. (2017). Digestibility of quinoa (Chenopodium quinoa Willd.) protein concentrate and its potential to inhibit lipid peroxidation in the zebrafish larvae model. Plant Foods for Human Nutrition, 72(3), 294–300. doi:10.1007/s11130-017-0626-1
- Zayas, J. F. (Ed.). (1997). Oil and fat binding properties of proteins. In Functionality of proteins in food (pp. 228–259). New York, NY: Springer-Verlag Berlin Heidelberg. doi:10.1007/978-3-642-59116-7_5