Abstract
We aimed to evaluate the dextrose equivalent and the dynamic rheological parameters of dextrin obtained by hydrolysis of edible canna starch by using α-amylase enzyme. We found that the dextrose equivalent value and dynamic rheological properties, as presented by storage and loss modulus (Gʹ and G″) values, varied with the incubation time (1, 3, and 5 h) and α-amylase concentration (0.03, 0.04, 0.05, and 0.06% w/w). Increase in the incubation time and α-amylase concentration increased the dextrose equivalent from 9.0 ± 0.2 to 21.3 ± 1.9 and decreased the storage modulus (G′) from 3747.4 to 18.0 Pa and the loss modulus (G″) from 659.4 to 5.5 Pa at 25°C. Meanwhile, G′ decreased from 27781.0 Pa to 11313.1 Pa and G″ decreased from 6647.2 Pa to 1826.4 Pa at 95°C.
INTRODUCTION
Dextrins are obtained from the partial hydrolysis of starch using acids, enzymes, or combinations of both.[Citation1–Citation3] They are used as thickening agents, texturizers, filling agents, and stabilizers in the food and pharmaceutical industries. Moreover, because of their bio-compatibility and degradability, dextrins and other starch-based materials are presently being used to prepare biodegradable hydrogels that have applications in medicine, pharmacy, and biology.[Citation4,Citation5] Furthermore, dextrins are potential excipients for drug-delivery systems.[Citation6,Citation7]
The properties of dextrin are influenced by the type of hydrolysis process and raw starch granules, which affect their molecular distribution and oligosaccharide profiles. Raw starch granules exist as crystalline forms and are resistant to attack by α-amylase enzyme. α-amylase hydrolyzes the α-(1,4)-glycoside linkage of starch by acting at random locations along the starch chain, ultimately yielding maltotriose and maltose from amylose or maltose, glucose, and “limit dextrin” from amylopectin. The extent of starch hydrolysis is generally expressed in terms of the “dextrose equivalent” (DE), a quantity that indicates the number of dextrose molecules released from the hydrolysis of starch. Glucose has a DE value of 100, while starch has a DE of zero.[Citation8] Maltodextrins are partial hydrolysates of starch with DE values below 20.
Dextrins with the same DE could vary in[Citation9] their hygroscopicity, fermentability, viscosity, sweetness, stability, solubility, and bioavailability.[Citation10] Chereji et al.[Citation11] reported that the α-amylase content of 0.10–0.15% assures the best quality for flour dough, which is related to its dextrin properties. Sun et al.[Citation10] reported that the size and viscosity of dextrin decreases with an increase in DE value. Juszczak et al.[Citation12] found that maltodextrins with different DE values exhibited different effects on the rheological properties of potato starch pastes. Maltodextrins with a DE value of 18.2 influenced the pasting characteristics, flow behavior, and viscoelastic properties of starch.
In general, gelatinization of starch is started by swelling and accompanied by leaching of granule constituents, predominantly amylose, and the formation of a three-dimensional networks.[Citation13–Citation16] These changes and the effect of α-amylase action are responsible for the rheological characteristics of dextrins. Rheological behavior of starch is influenced by amylose content, granule size distribution, granule volume fraction, granule shape, granule–granule interaction, and continuous phase viscosity.[Citation17–Citation21] Enzymatic hydrolysis of starch is a critical factor that govern the rheological/pasting behavior of dextrins. In relation to rheological properties of starch, Jiang et al.[Citation22] found that the storage modulus and loss modulus of mung bean starch increased with the increased pressure and the pressurized of mung bean starch gels were pseudoplastic non-Newtonian fluids.
The dynamic storage modulus (Gʹ) is a measure of the energy stored in the material and recovered from it per cycle of sinusoidal deformation, while the loss modulus (Gʺ) is a measure of the energy dissipated or loss per cycle.[Citation23] During the continous heating in dynamic rheometer, the Gʹ of starch progressively increases to a maximum at certain temperature and then drops. The initial increase in Gʹ is attributed to granule swelling.[Citation24] With further increase in temperature, Gʹ decreases, indicating that the gel structure is destroyed[Citation25] that is due to the melting of crystalline region of the granules.[Citation26] The present study aimed to determine the physicochemical properties of edible canna starch, namely, DE, storage modulus (G′), and loss modulus (G″), as a function of α-amylase concentration and incubation time. Edible canna starch was used because of its easy availability and its feasibility as a raw material for dextrin production.
MATERIALS AND METHODS
Materials
Edible canna (Canna edulis Kerr.) tubers were purchased from farmers in Malang City, Indonesia.
Isolation of Starch
Starch isolation was initiated by washing the edible canna tubers to remove solid dirt followed by crushing them to form a paste. The paste was mixed with water (1:3), and the mixture was sieved using a fine cotton cloth to separate the fibers. The fibers remained on the cloth, while the dispersed starch and protein passed through it. The crude dispersed starch was washed with water and left to precipitate for 12–24 h, followed by air-drying for at least 1 day. The dried starch was then milled to obtain fine starch flour.
Enzymatic Hydrolysis for Dextrin Production
A slurry containing 30% of starch (w/v) was adjusted to a pH of 5.3 and gelatinized at 85°C for 15 min. The gelatinized starch was then hydrolyzed using various concentrations of α-amylase (0.03, 0.04, 0.05, and 0.06% w/w of starch) at 95°C and incubated at 1, 3, and 5 h. After cooling at room temperature, α-amylase was inactivated by heating the liquid at 125°C for 20 min. After cooling, dextrin was analyzed.
Determination of DE Value of Dextrin
Approximately 1 g of sample was diluted with aquadest to 50 mL and centrifuged; 0.5 mL of the solution was then mixed with 3,5-dinitrosalicylic acid to measure the content of reducing sugar compared with glucose standard. DE value was calculated using the following equation:
Viscoelastic Behaviors
The analysis of storage modulus (G′) a measure of elastic response and loss modulus (G″) a measure of viscous response as a function of temperature was conducted using dynamic oscillatory test performed using a strain/stress controlled rheometer (Discovery Hybrid Rheometer; TA Instruments, New Castle, DE, USA). This rheometer was equipped with 2.5-cm diameter parallel plates, with an inter-plate distance of 1 mm. Preliminary tests were run in frequency sweep mode by varying the strain amplitude from 0.05 to 100% to obtain the selected strain. The selected strain (0.06%) was within the linear viscoelastic region. After reaching equilibrium at the initial temperature of 25°C, the samples were heated continuously at 2.5°C/min to a final temperature of 100°C. During the heating process, the samples were sheared at an angular frequency of 10 rad/s (Hz,) with a strain amplitude of 0.06% and a number of data points. The storage modulus (G′) and loss modulus (G″) were continuously monitored during the dynamic rheological testing.
RESULTS AND DISCUSSION
Rheological Properties
In general, several factors influence the rheological properties or viscosity of dextrin, namely, the molecular weight, the ratio of amylose to amylopectin, the free water content, and the temperature of dextrin. We observed that the amount of dextrose produced during the hydrolysis process (DE value) varies with the incubation time and the concentration of α-amylase enzyme. The time of contact between the enzyme and starch, and the enzyme concentration directly affects the rate of hydrolysis, consequently increasing the DE value of dextrin. Ba et al.[Citation27] reported that hydrolysis of starch by α-amylase led to reduction of the crystalline structure and produced dextrin with a Newtonian behavior.
Dextrin of edible canna starch obtained from various concentrations of α-amylase (0.03, 0.04, 0.05, and 0.06% w/w of starch) during 1 h incubation had relatively low storage modulus (G′) and loss modulus (G″; ); the resulting modulus values (G′ and G″) increased markedly when the thermal scanning temperatures exceeded 60.8, 53.1, 54.1, and 55.9°C, respectively, before achieving stability at a value in the range of 70–100°C. No intersection area was observed between G′ and G″ curves; however, the differences between G′ and G″ were lowest (0.05 Pa) at 63.3, 56.2, 57.2, and 60.3°C.
FIGURE 1 Storage modulus (Gʹ) and loss modulus (Gʺ) of edible canna dextrin obtained from various α-amylase concentration during 1 h hydrolysis.
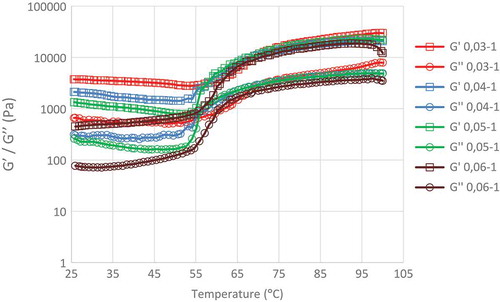
shows the storage modulus (G′) and loss modulus (G″) of the edible canna dextrin obtained from various concentrations of α-amylase during 3 h incubation. Both G′ and G″ increased markedly when the thermal scanning temperature exceeded 58.2°C, before reaching a stable high value in the range of 63°C–100°C. Xue and Ngadi[Citation28] reported that the increase in G′ from 58.0 to 68.0°C indicates an increase in the elastic properties of food batter (a complex systems comprised of water, flour or starch, and seasonings into which food products are dipped prior to cooking). This was attributed to the gelatinization of starch (dextrin), resulting in the onset of structure formation in which the fluid-like batter transforms into a solid-like coating.
FIGURE 2 Storage modulus (Gʹ) and loss modulus (Gʺ) of edible canna dextrin obtained from arious α-amylase concentration during 3 h of hydrolysis.
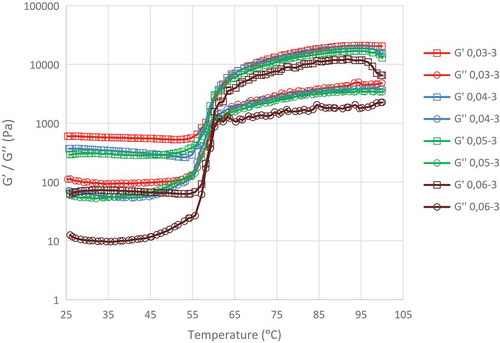
No intersection area was observed between G′ and G″ curves; however, a minimum difference between G′ and G″ (0.05 Pa) was observed at 59.2 and 60.2°C. The increase in α-amylase concentration from 0.04 to 0.06% considerably increased the G′ and G″ values at 56.2, 57.2, and 60.3°C. However, this increase was not observed when the α-amylase concentration was 0.03%.
shows the augmentation of storage modulus (G′) and loss modulus (G″) of the edible canna dextrin as a function of enzyme concentration (0.03, 0.04, and 0.05% w/w of starch) during 5 h-incubation. When the thermal scanning temperature exceeded 53.1, 58.2, and 61.2°C, respectively, G′ and G″ values markedly increased before attaining a stable high value in the range of 64–100°C. Dextrin obtained from 0.03 and 0.04% w/w of α-amylase showed no intersection area between the curves of G′ and G″. Minimum difference between G′ and G″ was observed at 54.9 and 60.2°C. Meanwhile, for dextrin hydrolyzed with 0.05% w/w of α-amylase, an intersection between G′ and G″ curves (0.05 Pa) was observed at temperatures from 60.2 to 62.3°C. The increase in G′ and G″values indicated that dextrin changed its phase from liquid to solid. However, the absence of intersection point between G′ and G″ showed that the true solid phase was not achieved due to the hydrolysis of starch molecules by α-amylase. This hydrolysis generated dextrin with a molecular weight lower than that of starch. Clark and Ross-Murphy[Citation29] defined the point of intersection between G′ and G″ curves as the gelation temperature.
FIGURE 3 Storage modulus (Gʹ) and loss modulus (Gʺ) of edible canna dextrin obtained from various α-amylase concentration during 5 h hydrolysis.
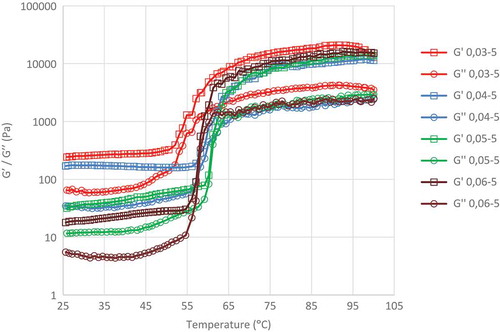
It was reported that in thermo-reversible materials, the solution viscosity decreases with increasing temperature until the gelling temperature (thermal gel point) is achieved, after which the viscosity rises sharply.[Citation30–Citation33] and show the data from rheological measurements at 25°C, while and show the data from rheological measurements at 95°C. The hydrolyses of edible canna starches by α-amylase at 0.03, 0.04, 0.05, and 0.06% w/w concentrations increased the DE values of its dextrins when the incubation time was increased from 1 to 5 h. This explains the variations observed in the rheological properties of dextrin, as shown by the significant reduction in G′ and G″ values, when measured using a rheometer at 25°C (see and ). The G′ and G″ values at 25°C significantly differed from those at 95°C. The G′ value at 25°C did not exceed 3747.4 Pa, while those at 95°C were between 11313.1 and 27781.0 Pa. The G″ value at 25°C did not exceed 659.4 Pa, while those at >95°C were between 1826.4 Pa and 6647.2 Pa.
TABLE 1 Storage modulus (Gʹ) and dextrose equivalent (DE) values of dextrin obtained from various α-amylase concentration and incubation time at 25°C rheological measurements
TABLE 2 Loss modulus (Gʺ) and dextrose equivalent (DE) values of dextrin obtained from various α-amylase concentration and incubation time at 25°C rheological measurements
TABLE 3 Storage modulus (Gʹ) and dextrose equivalent (DE) values of dextrin obtained from various α-amylase concentration and incubation time at 95°C rheological measurements
TABLE 4 Loss modulus (Gʺ) and dextrose equivalent (DE) values of dextrin obtained from various α-amylase concentration and incubation time at 95°C rheological measurements
and 4 show the data from rheological measurements at 95°C, where the hydrolysis of edible canna starches by α-amylase at 0.03, 0.04, 0.05, 0.06% w/w concentrations increased the DE values of its dextrins when the incubation time was increased from 1 to 5 h. This explains the variations observed in the rheological properties of dextrin, as shown by the significant decreased in G′ and G″ values. The decrease in G′ was correlated with a decrease in the viscosity of dextrin because of an increase in the DE along with the increase in hydrolysis activity. The increase in α-amylase concentration from 0.03 to 0.06% considerably decreased G′ from 27781.0 to 18275.0 Pa after hydrolysis for 1 h, and from 20764.7 to 11762.4 Pa after hydrolysis for 3 h. These data suggest that the storage modulus G′ decreases with a decrease in the molecular weight of starch/dextrin.
The rheological properties of dextrin at 95°C showed that the G″ value decreased along with a decrease in the molecular weight of dextrin due to an increase in α-amylase concentration and incubation time. During 1 h hydrolysis, the G″ value considerably decreased from 6647.2 to 3791.4 Pa, when α-amylase concentration was increased from 0.03 to 0.06%. The G″ value decreased from 4506.5 to 1826.4 Pa when α-amylase concentration was increased from 0.03 to 0.06% during 3 h hydrolysis, and decreased from 3998.4 to 2428.1 Pa during 5 h hydrolysis. G″ decreased markedly when the molecular weight of dextrin decreased due to enzyme activity. G′ and G″ values considerably increased when the temperature was increased to 95°C. We observed that G′ attained the highest value when the temperature exceeded 95°C, and decreased with a decrease in enzyme concentration and incubation time. This finding showed that a decrease in the molecular weight of dextrin led to a decrease in G′ at a temperature ≥95°C. G″ exhibited similar results; however, its value was considerably lower than that of G′. The extension of incubation time (from 1 to 5 h) and increase in the α-amylase concentration (from 0.03 to 0.06%) raised the DE from 9.0% ± 0.2 to 21.3% ± 1.9%.
The rheological properties of C. edulis dextrin at 25°C varied with the incubation time and α-amylase concentration. The increase in incubation time and α-amylase concentration decreased the storage modulus (G′) from 3747.4 to 18.0 Pa and the loss modulus (G″), from 659.4 to 5.5 Pa. Meanwhile, when the rheological properties were measured at 95°C, we observed that G′ decreased from 27781.0 to 11313.1 Pa, while G″ decreased from 6647.2 to 1826.4 Pa. Storage modulus (G′) and loss modulus (G″) significantly increased when the thermal scanning temperature exceeded the range of 53.1–61.2°C, before attaining a stable high value within the range of 63–100°C. The increase in G′ and G″ values indicated that dextrin was converted from liquid phase to solid phase. Moreover, the absence of intersection point between G′ and G″ indicated that the true solid phase was not achieved due to the degradation of edible canna starch by α-amylase that produced small dextrins.
CONCLUSION
The hydrolyses of edible canna starches by α-amylase produce a mixture of α-limit dextrin, maltose, and oligosaccharide. The DE and the rheological properties of C. edulis dextrin varied with incubation time and α-amylase concentration, as evident in the variation exhibited by the storage and loss modulus (G′ and G″) values.
FUNDING
The authors acknowledge the financial support of Minister of Education and Culture Republic of Indonesia (MP3EI Project).
Additional information
Funding
REFERENCES
- White, D.R.J.; Hudson, P.; Adamson, J.T. Dextrin Characterization by High-Performance Anion-Exchange Chromatography Pulsed Amperometric Detection and Size-Exclusion Chromatography-Multi-Angle Light Scattering-Refractive Index Detection. J. Chromatogr A. 2003, 997(1–2), 79–85.
- Chronakis, I.S.; Kasapis, S.; Richardson, R.K. Small Deformation Rheological Properties of Maltodextrin Milk-Protein Systems. Carbohydrate Polymers 1996, 29, 137–148.
- Carvalho, J.; Goncalves, C.; Gil, A.M.; Gama, F.M. Production and Characterization of a New Dextrin Based Hydrogel. European Polymer Journal 2007, 43, 3050–3059.
- Wong, W.H.; Mooney, D.J. Synthesis and Properties of Biodegradable Polymers Used as Synthetic Matrices for Tissue Engineering. In Synthetic Biodegradable Polymer Scafolds; Atala, A.; Mooney, D.; Vaganti, J.P.; Langer, R.; Eds.; Springer, Birkhauser: Boston, 1997.
- Marques, A.P.; Reis, R.L.; Hunt, J.A. The Biocompatibility of Novel Starch-Based Polymers and Composites: In Vitro Studies. Biomaterials 2002, 23(6), 1471–1478.
- Qi, X.; Tester, R.F. Bioadhesive Properties of β-Limit Dextrin. J Pharm Pharmaceut Sci (www.csps Canada.org) 2011, 14(1), 60–66.
- Hirst, D.H.; Chicco, D.; German, L.; Duncan, R. Dextrins as Potential Carriers for Drug Targeting: Tailored Rates of Dextrin Degradation by Introduction of Pendant Groups. International Journal of Pharmaceutics 2001, 230, 57–66.
- Dokik, P.; Jakovljevic, J.; Dokic-Baucal, L. Molecular Characteristics of Maltodextrins and Rheological Behaviour of Diluted and Concentrated Solutions. Colloids Surfaces A 1998, 141, 435–440.
- Marchal, L.M.; Beeftink, H.H.; Tramper, J. Towards a Rational Design of Commercial Maltodextrins. Trends Food Sci. Technol 1999, 10, 345–355.
- Sun, J.; Zhao, R.; Zeng, J.; Li, G.; Li, X. Characterization of Destrins with Different Dextrose Equivalents. Molecules 2010, 15, 5162–5173. DOI:10.3390 /molecule, 15085162
- Chereji, R.; Dogaru, D.; Bordei, D.; Mateescu, C. Scientifical Researches. Agroalimentary Processes and Technologies 2005, XI(1) 101–108.
- Juszczak, L.; Galkowska, D.; Witczak, T.; Fortuna, T. Effect of Maltodextrins on the Rheological Properties of Potato Starch Pastes and Gels. International Journal of Food Science 2013, 2013, http://dx.doi.org/10.1155/2013/869362
- Elianson, A.C. Retrogradation of Starch as Measured by Differential Scanning Calorimetry. In New Approaches to Research on Cereal Carbohydrates, Hill, R.D.; Munck, L.; Eds.; Elsevier Science Publishers: Amsterdam, Netherlands, 1985; 93–98.
- Hennig, V.H.J.; Lechert, H.; Goemann, W. Examination of Swelling Mechanism of Starch By Pulsed NMR Method. Starch 1976, 28, 10–17.
- Steeneken, P.A.M. Rheological Properties of Aqueous Suspensions of Swollen Starch Granules. Carbohydrate Polymers 1989, 11, 23–42.
- Tester, R.F.; Morrison, W.R. Swelling and Gelatinization of Cereal Starches. Cereal Chemistry 1990, 67, 558–563.
- Kaur, L.; Singh, N.; Singh J. Factors Influencing The Properties Of Hydroxypropylated Potato Starches. Carbohydrate Polymers, 2004, 55, 211–223.
- Morikawa, K.; Nishinari, K. 2002. Effects of Granule Size and Size Distribution on Rheological Behaviour of Chemically Modified Potato Starch. Journal of Food Science 2002, 67, 1388–1392.
- Okechkwu, P.E.; Rao, M.A. Influence of Granule Size on Viscosity of Corn Starch Suspension. Journal of Texture Sudies 1995, 26, 501–516.
- Singh, N.; Kaur, L. Morphological, Thermal, and Rheological Properties of Potato Starch Fractions Varying in Granule Size. Journal of the Science of Food and Agriculture 2004, 84, 1241–1252.
- Sing, N.; Singh, J.; Kaur, L.; Sodhi, N.S.; Gill, B.S. Morphological, Thermal, and Rheological Properties of Starches from Different Botanical Sources: A Review. Food Chemistry 2003, 81, 219–231.
- Jiang, B.; Li, W.; Hu, X.; Wu, J.; Shen, Q. Rheology of Mung Bean Starch Treated by High Hydrostatic Pressure. International Journal of Food Properties 2015, 18(1), 81–92.
- Ferry, J.D. Viscoelastic Properties of Polymers, 3rd Ed; Wiley P.: New York, NY: 1980; 641.
- Singh, J.; Kaur, L.; McCarthy, O.J. Factors Influencing the Physico-Chemical, Morphological, Thermal and Rheological Properties of Some Chemically Modified Starches for Food Applications: A Review. Food Hydrocolloid 2007, 21, 1–22.
- Tsai, M.L.; Li, C.F.; Lii, C.Y. Effects of Granular Structure on the Pasting Behavior of Starches. Cereal Chemistry 1997, 74, 750–757.
- Elliasson, A.C. Viscoelastic Behaviour During the Gelatinization Of Starch. I. Comparaison of Wheat, Maize, Potato, and Waxy-Barley Starches. Journal of Texture Studies 1986, 17, 253–265.
- Ba, K.; Blecker, C.; Danthine, S.; Tine, E.; Destain, J.; Thonart, P. Physicochemical Characterization of Dextrins Prepared with Amylases from Sorghum Malt. Starch 2013, 65(11–12), 962–968.
- Xue, J.; Ngadi, M. Rheological Properties of Batter Systems Formulated Using Different Flour Combinations. Journal of Food Engineering 2006, 77(2), 334–341.
- Clark, A.H.; Ross-Murphy, S.B. Structural and Mechanical Properties of Biopolymer Gels. Advance in Polymer Science 1987, 83, 60.
- Zecher, D.; Van Coillie, R.; Cellulose Derivatives. In Thickening and Gelling; Imeson, A., Ed.; Blackie A&P: Glasgow, 1992; 40–65.
- Nussinovitch, A. Hydrocolloid Applications; Blackie A&P: Glasgow, 1997; 105–124.
- Lopes da Silva, J.A.; Rao, M.A. Rheological Behavior of Food Gel System. In: Rheology of Fluid and Semisolid Foods; Rao, M.A.; Ed; Aspen Publication, Inc.: Gaithersburg, Maryland, 1999; 219–318.
- Chen, H.H.; Kang, H.Y.; Chen, D.D., 2008. The Effects of Ingredients and Water Content on the Rheological Properties of Batters and Physical Properties of Crusts in Fried Foods. Journal of Food Engineering, 2008, 88, 45–54.