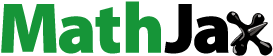
ABSTRACT
Collagen is an abundant structural protein found in many organs of animals, for example skin, bones, and connective tissues. Chicken trachea is a collagen-rich fraction of chicken offal that is converted to a solid waste stream during poultry processing. Collagen hydrolysates and peptides have unique biological properties, which is potentially converted from chicken trachea and can be used as a useful functional ingredient. Fresh chicken trachea was sterilized by autoclaving at 121°C for 30 min and dried using freeze drier to obtain chicken trachea flakes (CTF). CTF had 68.56% protein with the 50% solubility at pH 6–10. The enzymatic hydrolysis of chicken trachea was performed using Alcalase®, Flavourzyme®, Protamex®, and Papain under the optimal condition of each enzyme. An improvement in solubility was observed. Chicken trachea hydrolysates (CTH) with the highest solubility were detected in samples hydrolyzed with Alcalase®, follow by Protamex®, Papain, and Flavourzyme®, respectively. Within an hour of hydrolysis, the CTH obtained from Alcalase® hydrolysis had the highest total amino acid contents. The top three amino acids found in this hydrolyzate powder were Glutamic acid (Glu), Glycine (Gly), and Aspartic acid (Asp). Moreover, this sample exhibited the greatest antioxidant and bioactive properties as shown by the highest antioxidant capacity from DPPH and FRAP assay (4.42 TEAC µMol/mg CTH and 22.48 TEAC µMol/mg CTH, respectively) and the lowest IC50 of ACE I inhibitor (0.41 mg/mL). These results suggested that Alcalase® hydrolysis provided the chicken trachea collagen hydrolyzate with unique properties that could be used in various food systems.
GRAPHICAL ABSTRACT

Introduction
Poultry is one of the top 10 exported agricultural products of Thailand and contributes 11% of the Thai GDP about 110,484 million baths in 2020. Moreover, the increase in investment was reported at 1.5% to 2% every year.[Citation1] Wastes and by products from chicken production line are the most important problems for Thai chicken industries. Chicken slaughterhouses produce several solid and liquid waste streams. Solid wastes were head, bone, feather, or offal, which were filled in land or used as an ingredient of fertilizer, while liquid was blood and cleaning water. It was treated before being sent out to the environment. Poor waste management in chicken slaughterhouse leads to pollution problem and pestilence problems.[Citation2] Over the past decade, research on liquid waste has provided opportunities for manufacturers to valorize such waste, which has resulted in improved economic and environmental sustainability of the process. However, the solid wastes have largely been overlooked. The industries start to embrace the idea of waste management, which is not just for disposal, but requires innovative solutions that are cost-effective, minimize landfill disposal and greenhouse gases, and incorporate recycling and reuse.[Citation3]
Windpipe or trachea is a key organ of most air-breathing animals with lungs. It is a vertical tube made of rings of cartilage connecting the pharynx and larynx to the lungs, allowing the passage of air. Cartilage contains specialized cells called chondrocytes that produce a large amount of collagenous extracellular matrix, abundant ground substance that is rich in proteoglycan and elastin fibers. Chicken trachea is a part of the solid waste from slaughterhouses.[Citation4] One problem with using chicken trachea for the production of functional peptides is that they are highly contaminated with microorganisms. High-temperature-long-time sterilization is the preferred thermal process, as it eliminates all forms of virus, bacteria, yeast, and fungi. Moreover, under intense heat treatment collagen undergoes thermal hydrolysis to yield peptides and amino acids.[Citation5]
Currently, enzymatic hydrolysis with food-grade proteases is preferred to produce protein hydrolyzate because it provides nontoxic by-products and the product composition and quality is easily controlled.[Citation6,Citation7] Proteases can be classified into two groups including endopeptidase and exopeptidase. Endopeptidases are proteolytic peptidases that break peptide bonds of non-terminal amino acids, while exopeptidases break peptide bonds from end-pieces of terminal amino acids. Therefore, endopeptidases cannot break down peptides into monomers, while exopeptidases can break down proteins into monomers. The important factors that should be controlled and optimized are enzyme-to-substrate ratio, hydrolysis temperature, hydrolysis time, and pH value.[Citation8] .[Citation9] Peptide is a chain of amino acids joined by peptide bonds to form a single molecule. There are 20 naturally occurring amino acids and, like letters into words, they can be combined into an immense variety of different molecules. When a molecule consists of 2–50 amino acids it is called a peptide, whereas a larger chain of >50 amino acids generally is referred to as a protein.[Citation10] The function that a peptide carries out is dependent on the types of amino acids involved in the chain and their sequence, as well as the specific shape of the peptide. In the human body, peptides are found in every cell and tissue and perform a wide range of essential functions. Maintenance of appropriate concentration and activity levels of peptides is necessary to achieve homeostasis and maintain health. These substances are essential proteins for human life that provide a wide variety of health promoting benefits consisting of antioxidants, antimicrobials, anticancers, antihypertensives and anti-aging.[Citation11–13] Protein hydrolyzate might be an alternative approach for adding value to waste from poultry industry, which is an attractive ingredient.
This research investigated the appropriate enzymatic hydrolysis condition for producing functional and bioactive hydrolysates from chicken trachea was studied. Chemical, antioxidant, and bioactive properties of chicken trachea hydrolyzate were demonstrated. The molecular weight of the produced hydrolyzate was also revealed using SDS-PAGE.
Materials and methods
Materials
Chicken trachea was obtained from a chicken slaughterhouse in Bangkok, Thailand, and frozen for further experiment. The four commercial proteases used in this study were Alcalase®, Flavourzyme®, Protamex®, and Papain. Alcalase®, Flavourzyme®, Protamex® from Novozyme were purchased from local ingredient supplier in Thailand (Brenntag Co. Ltd, Bangkok), while papain from papaya latex were obtained from Sigma-Aldrich (St. Louis, MO). Disodium tetraborate, o-phthaldialdehyde, DL-dithiothreitol, SDS, and serine were purchased from Sigma-Aldrich (St. Louis, MO). Precast Gels (4–15% stain), sample loading buffer, Coomassie Bryant blue stain, and molecular weight standard for SDS-PAGE were purchased from Bio-Rad (USA). The reagent including 2, 2-diphenyl-1-picryl-hydrazyl (DPPH), 2,2′-azino-bis (3-ethylbenzothiazoline-6-sulfonic acid) (ABTS), 2,4,6-Tri(2-pyridyl)-s-triazine (TPTZ), and dipeptidyl carboxypeptidase extracted from rabbit lung were obtained from Sigma-Aldrich (St. Louis, MO).
Raw material preparation
Frozen chicken trachea was thawed and cleaned in cold tap water. Three hundred grams of the sample were mixed with distilled water at a ratio of 1:2 (w/w), sterilized at 121 C° for 30 min by autoclave, and then cooled to room temperature. The blended sample was dried using a freeze dryer (give make and model) to obtain chicken trachea flake (CTF). The CTF was frozen and stored at – 20 °C until required for further analysis of protein and fat content according to the AOAC method[Citation14] and protein solubility using the Hartree-Lowry assay.[Citation15]
Enzymatic hydrolysis
CTF was subjected to hydrolysis using the conditions shown in . Protein hydrolyzate is produced according to the method previously described by[Citation15] with some modifications. The CTF was mixed with distilled water and adjusted to the optimum pH of the enzyme (see ) using 0.1 N NaOH. The reactions were performed in 50 mL tube with controlled temperature using a Shaking water bath (Linear Shaking Water Bath, Grant Instruments). In order to inactivate the protease enzyme, the mixture was heated at 100°C for 15 min in hot air oven. After that, the sample was cooled down at room temperature and centrifuged at 2,010 g at 4°C for 30 min (Avanti Centrifuge, J-26 XP). The supernatant was collected and freeze-dried at −50°C, under vacuum (Edwards, Supermodulyo Freeze Dryer) to obtain chicken trachea hydrolyzate (CTH) from each enzyme, and finally it was stored at −20°C until further analysis.
Table 1. Conditions for the enzymatic hydrolysis of different proteases
Protein content
The nitrogen content of the sample was analyzed using CHN determination (Exeter Analytical, Hygroscopic Model 440). The crude protein content in dried chicken trachea hydrolyzate was calculated using equation (1) from Kjeldahl method.[Citation16]
where, F is conversion factor (i.e., 6.25).
Degree of hydrolysis
The OPA method was used to monitor the degree of hydrolysis (DH) by monitoring the reaction of o-phthaldialdehyde (OPA) with the amino groups produced during enzymatic hydrolysis. Protein hydrolyzate solution (400 µL) was added to 3 mL of OPA reagents and mixed with vortex for 5 sec. The mixture was left at room temperature for 2 min before measuring the OD at 340 nm using a Genesys 10s UV-vis spectrophotometer (Thermo Scientific, UK). The DH was calculated using equation (2) to (4):
DH = h/htot × 100 (2)
h = (serine-NH2 – β)/(α megv)/g protein (3)
serine-NH2 = *
(4)
The value for constants β, α, and htot were 0.40, 1.00, and 7.6, respectively, as suggested by .[Citation17]
Sodium dodecyl sulfate-polyacrylamide gel electrophoresis (SDS-PAGE)
SDS-PAGE was performed to reveal the molecular weight profile of protein hydrolyzate. SDS PAGE precast gel (4–20%, Mini-Protean Gels, Bio-Rad) was used to analyze the protein hydrolyzate from chicken trachea. SDS PAGE was conducted under reduced conditions using a Mini-PROTEAN Tetra cell, Coomassie Brilliant Blue R-250 (Sigma) was used to stain the gels, and Plus2 Prestained (See Blue) was applied as standard marker. The electrophoretic analysis was performed using Mini-PROTEAN Tetra Cell electrophoresis apparatus (Bio-Rad Laboratories Inc., Hercules, CA) at a constant voltage of 120 V for 40 min.[Citation18]
Protein Solubility at different pH
Ten milligrams of samples were dissolved in 10 mL distilled water in plastic tube Falcon tubes. Ten CTH solution samples were adjusted to pH 2, 4, 6, 8, 10 with 0.1 NaOH or 0.1 N HCl. The suspension was centrifuged at 9,660 g for 10 min using a high-speed microcentrifuge (Benchmark, MC-12™) and the supernatant was collected. The protein content of the supernatant was analyzed using the Hartree-Lowry assay. The percentage of protein solubility in CTH was performed using a standard curve of BSA.[Citation19]
Antioxidant properties
For all assays, freeze-dried protein hydrolyzate samples hydrolyzed by AL, FL, PT, and PA were dissolved (10 mg/mL) in phosphate buffer except in the FRAP assay where distilled water was used to dissolve the samples.
DPPH radical-scavenging activity
The scavenging effect of CTH samples or the standard Trolox for DPPH radicals was monitored according to the method described in a previous report.[Citation20] Briefly, 500 µl of test sample was added to 500 µl of 0.1 mM DPPH ethanolic solution. The mixture was vortexed for 1 min and then left to stand at room temperature for 30 min in the dark, and then its absorbance was read at 517 nm using a Genesys 10s UV-vis spectrophotometer (Thermo Scientific, UK). The ability to scavenge the DPPH radical was calculated using the following equation.[Citation5] A standard curve was prepared using Trolox in the range of 5–50 µM. The DPPH activity of CTH was expressed as µmol Trolox equivalents (TE)/mg CTH.
where, Acontrol is the absorbance of DPPH solution without sample, Asample is the absorbance of DPPH solution plus test sample, and Ablank is the absorbance of the phosphate buffer plus ethanol.
ABTS radical-scavenging activity
ABTS radical scavenging activity of samples or Trolox was carried out using the method described previously.[Citation21] ABTS radical solution was prepared by mixing 10 mL of 7 mM 2,2′-azino-bis(3-ethylbenzothiazoline-6-sulfonic acid) and 5 mL 2.45 mM potassium persulfate and incubating for 15 h at 37°C. ABTS (900 µl) was mixed with 100 µL of sample and held for 6 min. The control was prepared by adding 100 µL of phosphate buffer instead of sample. For the blank, buffer was added instead of ABTS. Absorbance of the reaction mixture was observed at 734 nm using a Genesys 10s UV-vis spectrophotometer (Thermo scientific, UK). Scavenging activity was calculated using the following equation:
A standard curve was prepared using Trolox in the range of 20–200 µM. The activity was expressed as µmol Trolox equivalents (TE)/mg sample.
FRAP radical-scavenging activity
Ferric reducing ability (FRAP) method was applied to measure the ferric ion reducing capacity of chicken trachea hydrolysates.[Citation22] The model is based on the change of absorbance at 595 nm,37°C to detect the formation of the complex tripiridiltriazine (TPTZ)–Fe(II) in the presence of reducing agents. All hydrolysates were dissolved in distilled water. Absorbance was read after incubating for 30 min using a Genesys 10s UV-vis spectrophotometer (Thermo Scientific, UK). A standard curve of FeSO4 .7H2O, which related to the concentration of Trolox (50–200 µM) to the absorbance at 595 nm, was performed. The results were expressed as Trolox equivalents (TE)/mg sample.
Antihypertensive properties using ACE inhibitor
CTH samples possessing the highest antioxidant activity obtained from each enzyme were selected for measurement of ACE inhibitor activity according to the method of[Citation23] with slight modifications. The samples and enzymes were dissolved in 50 mM HEPES–HCl buffer containing 300 mM NaCl (pH 8.3). Thirty microliters of CTH (0.1, 0.25, 0.5, 0.75, 1 mg/mL) were mixed with ACE solution (8 mU/50 mL, 50 µL). When the mixture was pre-incubated at 37°C for 5 min, then the 50 µl of hippuryl-L-histidyl-L-leucine (HHL, 6 mg/mL) solution were added into the mixture to initiate the reaction and incubated at 37°C for 15 min. Three hundred and eighty microliters of 1 M HCl were added to terminate the enzymatic reaction. The hippuric acid formed was extracted with 1.5 mL of ethyl acetate, and the mixture was vigorously mixed for 1 min, followed by centrifugation at 9,660 g for 10 min using a high-speed microcentrifugation (Benchmark, MC-12™). The supernatant (1 mL) was collected and heated in boiling water to evaporate the solvent and the hippuric acid retained was dissolved in 1 mL of distilled water and the absorbance read at 228 nm. The ACE-inhibitory activity (%) was determined using the following equation.
where Acontrol is the absorbance of 50 mM HEPES–HCl buffer containing 300 mM plus NaCl (pH 8.3) without sample, Asample is the absorbance of 50 mM HEPES–HCl buffer containing 300 mM plus NaCl (pH 8.3) with sample, Acontrol blank and Asample blank are where ACE solution was added into the reaction without terminating the reaction with 1 M HCl. The IC50 value was defined as the concentration of inhibitor required to inhibit 50% of the ACE activity.
LC/MS methodology for Determination of Peptide Mass Distribution
The samples were reconstituted at 10 mg/mL in 95% ACN/5% MiliQ water (18.2 Ω) and further diluted down to 1 mg/mL using the same buffer system. The chemical profiling was performed on a Dionex UltiMate 3000 UHPLC system (Thermo, Hemel Hempstead, UK) connected to a Thermo Scientific Q-Exactive Basic Hi-Resolution mass spectrometer system (Thermo, Hemel Hempstead, UK).
The chromatographic separations were achieved using a Waters T3 high strength silica (HSS) C18 UHPLC column (150 mm x 1.8 mm ID, 1.7 µm particle size) (Waters, Elstree, UK) with a flow rate of 400 µL/min operating at 45°C. The LC buffers were a binary solvent system consisting of Buffer A (Ultrapure water 18.2 Ω x ≤ 1 ppb Total organic content (TOC) with 0.1% formic acid) and Buffer B consisted of Optima grade acetonitrile with 0.1% formic acid. The LC profile was as follows: 0 min (5% B) hold for 1 min, the n process with increasing linear gradient to 100% B at 11 mins (curve 5), this was held for further 3 min (wash period) and a further column stabilization time of another 3 mins at the original starting condition (5% B).
For the heated electrospray ionization (HESI) source, the electron transfer capillary temperature was set to 275°C with an applied voltage of 3.5 kV. The Rf frequency of the S-lens was set to 50. The HESI’s Sheath flow rate was set to 50, the auxiliary flow set to 15, and a sweep gas set to 3 (all arbitrary units). Finally, the HESI was operating 425°C throughout the analysis. For the MS1 profiling, the mass spectrometer was operating at 35 K mass resolution of 200 m/z with a scan rate of approximately 7 scan/s−1 and a mass range of 100–1000 m/z. The automatic gain control (AGC) was set to 1e6 with a maximum injection time of 100 ms. The total run time per analytical run was approximately 20 min from injection to injection. 5 µL injection volume and the samples were held at 4°C within the autosampler module prior to and following injections.
Total amino acid content
Total amino acids of CTH were determined using HPLC with a fluorescence detector as described by previous study.[Citation24] The sample was hydrolyzed with 6 N HCl and placed in a heating block at 110°C for 22 h. After acid hydrolysis, an internal standard was added to the hydrolyzate and then this was diluted with deionized water, which had been filtered with a 0.45 µm membrane filter. The filtrate was mixed using a vortex mixer with AccQ-fluor derivatization buffer and AccQ-fluor reagent to derivatize the amino acids. Then, the sample was heated at 55°C for 10 minutes in a heating block, after which it was injected onto a Hypersil Gold column C18 (4.6×150 mm,3um) and analyzed using HPLC. Identification of the amino acids in the sample was carried out by comparing their retention times with the standards.
Results and discussion
Characteristics of chicken trachea flake
The protein and fat content of CT and CTF were not significantly different on a dry basis () (p > .05). The total protein content of CT and CTF was 69.71% and 68.56%, and the fat content was 16.53% and 16.95%, respectively.
Table 2. Protein and fat contents (% dried basis) of fresh chicken trachea (CT) and chicken trachea flake (CTF)
These results are comparable to the protein content in duck, chicken, and ostrich trachea of 66.19, 79.19, and 84.38, respectively.[Citation25] It is clear that heat, freezing, and drying does not affect the protein and fat content of CTF because temperature fluctuations change only the protein structure but not nitrogen content[Citation26] Hence, the detected protein content in the sample remained high.
Protein is a major component of CTF, and the isoelectric point (pI) of the protein constituents is an important factor determining its solubility prior to enzymatic hydrolysis. CTF had a good solubility of 45–50% over the pH range 6–10, while it was lower than 45% at pH 2. The protein is insoluble at pH 4 represented the isoelectric point where its net charge is zero.[Citation25] The results showed that the pI of CTF was close to pH 4, and for this reason, the suitable hydrolysis condition of CTF was in the range of pH at 6–10.
Characteristics of chicken trachea hydrolyzate (CTH)
presents the soluble protein released and DH of CTH obtained from the various enzyme treatments over a period of 6 hours. For all enzymes, the initial rate of soluble protein release and the increase in DH was extremely high during the first hydrolysis hour. For Protamex® and Flavourzyme®, the soluble protein content reached the highest point after 2–3 hours and then changed slightly. For Alcalase® and papain protein release, it continually remained at a constant rate between 1 and 6 hours. After 6 hours, the CTH hydrolyzed by Alcalase® had highest soluble protein content, followed by papain, Protamex®, and Flavourzyme® (). These results indicate the effectiveness of protein hydrolysis using commercial proteases that are suitable for commercial applications.
Figure 1. Protein content and DH of CTH hydrolyzed with 4 protease(AL = Alcalase®, FL = Flavourzyme®, PT = Protamex® and PA = Papain) at different hydrolysis time (0, 1, 2, 3, 4, 5, 6 hours)
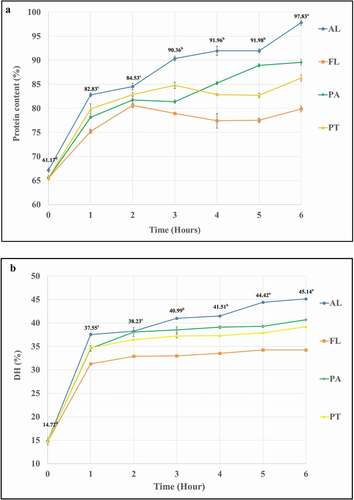
The DH of the CTH hydrolysates produced by these enzymes had similar trends to the soluble protein released. Initially, the DH increased sharply in the first hour, and then slowed down from 1 to 6 hours. After 6 hours of hydrolysis, the final DH of all samples was followed in the same order as for the protein released, i.e., the highest DH was found with Alcalase®, followed by papain, Protamex®, and finally Flavourzyme®. This might be because of the highest enzyme activity of Alcalase®. This enzyme gave the aggressive hydrolysis with the activity of 2.4–4.0 AU-A/g. This commercial protease was versatile endo-protease suited for animal protein extraction and provided very extensive hydrolysis. With this property, Alcalase® yielded the hydrolyzate with the highest DH.
Regarding the fat content in CTF, the effect of fat from raw material on enzymatic hydrolysis was not detected. The solid-to-liquid ratio was controlled at 4%w/v. Only 4 g of dried chicken trachea flake was added into 100 mL water, hence the lipid in the sample during hydrolysis was then diluted 25 times. For each hydrolysis flask (8 g to 200 mL), it contained only 1.356 g of fat in 200 mL of water. At this concentration, the effect on enzyme activity was not detected. Moreover, there was a report of squid head protein hydrolysis that also uses commercial protease hydrolysis without fat reduction.[Citation27]
SDS-PAGE () was also used to monitor the progress of proteolysis. The CTH obtained from Alcalase® and Protamex® hydrolysis showed almost complete hydrolysis after the first hour of hydrolysis, with all peptides formed having molecular weight smaller than 6 kDa. On the contrary, larger peptides were observed when Flavourzyme® or Papain enzyme was used and these persisted in protein bands for longer hydrolysis times. CTH with papain hydrolysis shows pronounced bands on the SDS PAGE gel over a wide range of molecular weights.
Figure 2. SDS-PAGE analysis of CTH hydrolyzed with Alcalase® (A), Flavourzyme® (B), Protamex® (C) and Papain (D). Lane contents are as follows: M1 is Marker; M2 is Small molecule Marker; flow-through; Lane 3 is Protease; 0 H – 6 H are CTH that hydrolyzed and inactivate at 0, 1, 2, 3, 4, 5 and 6 hours
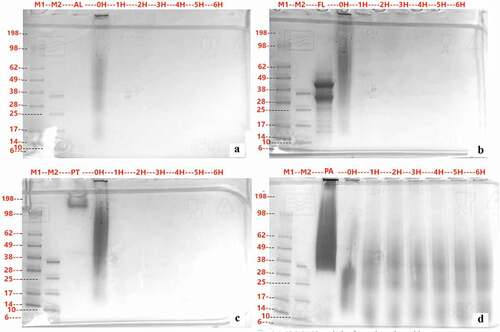
The CTH hydrolysis results can be compared to other protein systems hydrolyzed with the same enzymes. Hydrolysates have been produced from low-ash poultry meal using Alcalase®, Protamex®, and Flavourzyme®, giving a DH (%) at 7 h of 9.41, 10.63, and 2.54, respectively.[Citation28] Goat milk hydrolyzed with Alcalase®, Flavourzyme®, Papain, proteinase K, and trypsin gave a significantly higher DH with proteinase K, Alcalase®, and trypsin than for Papain and Flavourzyme®.[Citation29] Rice bran protein concentrate and soybean protein hydrolyzed with Alcalase® and Papain showed a higher DH with Alcalase®.[Citation6] Various authors report that, when compared to other commercial protease, Alcalase® results in higher protein recovery. Shrimp waste hydrolyzed with Alcalase® showed mean protein recovery in the range of 59–60%, which was significantly (p ≤ 0.05) higher than Neutrase® and also higher than Protamex® and Flavourzyme® by 5.31% and 8.20%, respectively.[Citation7] This result might be due to the combination of enzyme in Alcalase® which was endopeptidases. Peptides and protein hydrolysates received from this enzyme hydrolysis were generated fast and aggressive at the optimal pH and temperature used in this study. As a result, protein hydrolyzate with high DH and low molecular weight was observed.
Solubility of chicken trachea hydrolyzate
All freeze-dried powders made from the hydrolyzed CTF using any of the proteases had greater protein solubility than for the non-hydrolyzed CTF, with the solubility increasing with hydrolysis time as shown in . Alcalase® (AL) and Protamex® (PT) created CTH, which had high solubility at pH 4 after hydrolysis for only 1 h. However, Flavouryme® (FL) and Papain (PA) showed a more gradual increase in solubility with hydrolysis time. Enzymatic hydrolysis reduced the average molecular mass of proteins/peptides and liberates ionizable groups. Both factors improve protein solubility.[Citation30] Collagen has a distinct composition compared to other proteins, as it is rich in glycine and proline, and does not contain cysteine and tryptophan.[Citation5] As a result of prolonged heating in water, collagen undergoes partial hydrolysis, which occurs with structural changes, due to the breaking of inter-chain bonds, transforming into collagen hydrolyzate.[Citation31] Results indicated that cleavage of peptide bonds was higher in enzymatic hydrolysis and led to the formation of smaller peptides and free amino acids.[Citation20]
Bioactive properties of chicken trachea hydrolyzate
The antioxidant properties of CTH produced by the different proteases and at different hydrolysis times were measured using DPPH, ABTS, and FRAP assays. These methods were measured the ability of a molecule to donate hydrogen atoms to free radicals. The data of these three assays are shown in presented as Trolox Equivalent Antioxidant Capacity (TEAC) in μM/mL. For all samples, and for all assays, there was an initial rapid increase in antioxidant capacity within the first hour of hydrolysis, with the curves leveling off for longer hydrolysis times. In general, Alcalase® was the best protease for producing antioxidant protein hydrolyzate from CTF (DPPH = 4.02–4.27 TEAC μM/mL, ABTS = 134.29–148.84 TEAC μM/mL; FRAP = 16.67–22.48 TEAC μM/mL) whilst Papain gave CTH with the lowest antioxidant properties (DPPH = 0.43–3.71 TEAC μM/mL, ABTS = 67.99–89.87 TEAC μM/mL; FRAP = 11.57–12.51 TEAC μM/mL) (). For Alcalase®, the optimum antioxidant capacity found in the FRAP assay after 1 hour and then started to decrease again after 3 hours. Protamex® and Flavourzyme® gave CTH with antioxidant capacity intermediate between Alcalase® and Papain, but the three assays did not give a consistent view of which of these two gave CTH with higher antioxidant capacity. It should be noted that although the three assays do, in general, give consistent trends in antioxidant capacity of the hydrolysates from the four enzymes, the absolute TEAC units for the three assays differ markedly. This is because these assays used the different theoretical basis of the chromogen formation reaction. Chemical reagents that form different chromogen could be detected with spectrophotometer at different wavelengths.[Citation32] Hence, the different numbers of antioxidant capacity from these three assays resulted.
Figure 4. Antioxidant ability of hydrolyzate from chicken trachea hydrolyzed with 4 protease (AL = Alcalase®, FL = Flavourzyme®, PT = Protamex® and PA = Papain) at different hydrolysis time (0, 1, 2, 3, 4, 5, 6 H.)
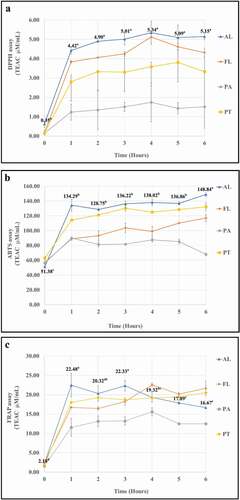
Proteins are biopolymers that consist of amino acids linked by peptide bonds that are formed between the primary carboxyl and amino groups. The other parts of the amino acids constitute the side groups that contribute to the structural and functional properties of proteins.[Citation33] The interactions between the R residues of amino acids in the polypeptide chains, which can establish different types of intramolecular bonds such as: hydrogen bonds, ionic bonds, covalent bonds, van der Waals forces, dipole–dipole interactions. The reason is that peptides chains have the potential to donate hydrogen atoms that can quench free radicals; these groups could possess excess electrons that can also be used to neutralize free radicals.[Citation34]
The ACE inhibitory capacity of CTH hydrolyzed by the four proteases for 1 h was measured and is presented in by 50% as IC50 i.e. the concentration at which 50% inhibition occurs. CTH itself has ACE inhibitory activity with an IC50 of around 1.2 mg/mL. This was improved significantly by hydrolysis of the CTH for 1 h, with a reduction in IC50 of around 50% or greater. Alcalase is the best enzyme for producing antihypertensive CTH hydrolyzate with an IC50 of 0.422 mg/mL.
Figure 5. ACE inhibitor (IC50) of hydrolyzate from chicken trachea hydrolyzed with Alcalase® (AL), Flavourzyme® (FL), Protamex® (PT) and Papain (PA)
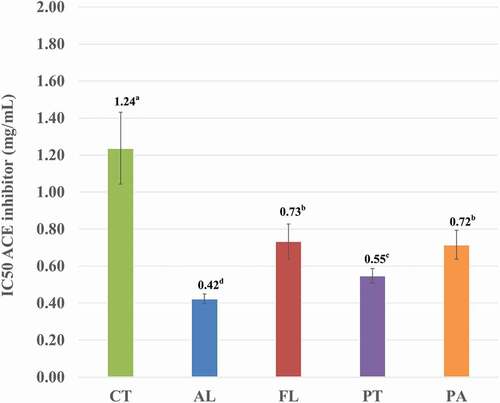
A variety of antioxidant and antihypertensive peptides have been isolated from hydrolysates of foods, plants, food by-products, and from various meat proteins.[Citation35] A report in 2017[Citation36] investigated the production of bioactive peptide from bovine lungs using Papain, Pepsin, and Alcalase®. The researchers reported that Alcalase® hydrolysis produced hydrolyzate with the lowest IC50 for the FRAP assay.[Citation36] A report also revealed that ACE inhibitory peptide was isolated and purified from chicken bone extract by enzymatic digestion.[Citation37] These results were supported by[Citation13]who found antihypertensive activity in four peptides in myosin heavy chain from boneless chicken legs.[Citation13] In addition, the report from[Citation23] revealed and identified 10 peptides, consisting of 5–10 amino acid residues, in chicken leg bone protein hydrolyzed with Alcalase®.
Molecular weight and amino acid of chicken trachea hydrolyzate
The average molecular weight (MW) of CTH hydrolyzed by Alcalase® (AL), Flavourzyme® (FL), Protamex® (PT), and Papain (PA) for 1 h. is shown in . FL gave the lowest average MW at 252.65 Da followed by Papain (283.05 Da), Protamex (411.48 Da), and Alcalase® the highest (487.43). This supports the results of SDS PAGE, which indicated that all samples contained peptides of molecular weight lower than 6 kDa after hydrolysis. Flavourzyme® and Protamex® endo and exo – peptidase activity, cleaving the protein at both terminal positions and in the middle of the protein/peptide chain, thus increasing the proportion of low molecular weight peptides faster, when compare with Alcalase®, which is endopeptidase activity.[Citation38–40]
Table 3. Average molecular weight (Da) of hydrolyzate from chicken trachea hydrolyzed with Alcalase (AL), Flavourzyme (FL), Protamex (PT), and Papain (PA). Average molecular weight was calculated from HPLC/MS data for hydrolysates from four enzymes after 1 hour of hydrolysis
Total amino acid composition of CTF and CTH after hydrolysis for 1 h by the four enzymes showed release of 15 amino acids in the protein hydrolyzate (). The most abundant amino acids in both CTF and all CTH were Glu, Gly, Ala, and Pro. Alcalase® hydrolyzate had the highest total amino acid content of 62.71 mg/100 mg, consistent with its endo-peptidase activity.
Table 4. Total amino acid of hydrolyzate from chicken trachea hydrolyzed with Alcalase® (AL), Flavourzyme® (FL), Protamex® (PT) and Papain (PA)
Hydrolysis enhances hydrophilic amino acids in the terminal chain of peptide and as a result improves the solubility of protein hydrolyzate. This was confirmed by an increase in hydrophilic amino acids such as serine (Ser) and threonine (Thr) when CTF was hydrolyzed by Alcalase® and Protamex®. Moreover, Glu – a charged hydrophilic amino acid and Gly – a polar amino acid was increased by enzymatic hydrolysis. The hydrophobic amino acids in the protein hydrolyzate have been reported to contribute to their antioxidant and antihypertensive properties.[Citation41] According to the research of Leon-Lopez, et al. (2019), the enzymatic treatment of collagen showed significant increases in aspartic acid and glutamic acid due to enzymatic cleavage of the polypeptide chains of collagen fibers.[Citation31] Furthermore, the protein hydrolyzate of chicken meat hydrolyzed by Alcalase® contained glutamic acid and tryptophan at 14.00% and 12.37%, respectively.[Citation42]
Conclusion
This study indicated that chicken trachea can be a source of protein for producing protein hydrolyzate. Alcalase®, Flavourzyme®, Protamex®, and Papain were effectively hydrolyzed chicken trachea to produce unique collagen hydrolysates. The Alcalase® hydrolysis for 1 hour provided collagen hydrolyzate with the best antioxidant activity. The obtained hydrolyzate had molecular weight lower than 6 kDa and had good solubility along the studied pH range. The solubility of the hydrolyzate showed an improvement over the non-hydrolyzed CTF, particularly at pH 4 (the isoelectric point of collagen). The antioxidant and antihypertensive properties showed a correlation with hydrophobic amino acid content. In conclusion, chicken tracheas appear to be a suitable alternative source of collagen hydrolyzate compared to typical sources like bone skin and offal.
Acknowledgments
The authors gratefully acknowledge Research and Researchers for Industries – RRI (PHD59I0014) for funding this research, CPF Food Research and Development Center for financial and ingredient supports, Department of Product Development, Faculty of Agro-Industry, Kasetsart University, Thailand, and School of Engineering and Physical Sciences, Heriot-Watt University for instrumental support of this work. The authors declare that there is no conflict of interest.
Disclosure statement
No potential conflict of interest was reported by the author(s).
Additional information
Funding
References
- Chuasuwan, C. Frozen and Processed Chicken Industry of Thailand. Krungsri Res. 2020, 1–6.
- Lasekan, A.; Abu Bakar, F.; Hashim, D. Potential of Chicken by-products as Sources of Useful Biological Resources. Waste Manag. 2013, 33(3), 552–565. DOI: https://doi.org/10.1016/j.wasman.2012.08.001.
- Brandelli, A.; Sala, L.; Kalil, S. J. Microbial Enzymes for Bioconversion of Poultry Waste into Added-Value Products. Food Res. Int. 2015, 73, 3/12. DOI: https://doi.org/10.1016/j.foodres.2015.01.015.
- Jaroenviriyapap, T.; Vittayanont, M. Type and Content of Chondroitin Sulphate and Collagen in Poultry Tracheas. Asian J. Food Agro. Indus. 2009, 2(4), 974–980.
- Cleland, T. P.; Vashishth, D. Bone Protein Extraction without Demineralization Using Principles from Hydroxyapatite Chromatography. Anal. Biochem. 2015, 472, 62–66. DOI: https://doi.org/10.1016/j.ab.2014.12.006.
- Ahmadifard, N. Comparison the Effect of Three Commercial Enzymes for Enzymatic Hydrolysis of Two Substrates (Rice Bran Protein Concentrate and Soybean Protein) with SDS-PAGE. J. Food Sci. Technol. 2016, 53(2), 1279–1284.
- Dey, S. S.; Dora, K. C. Optimization of the Production of Shrimp Waste Protein Hydrolysate Using Microbial Proteases Adopting Response Surface Methodology. J. Food Sci. Technol. 2014, 51(1), 16–24. DOI: https://doi.org/10.1007/s13197-011-0455-4.
- Aluko, R. E. Food Protein-Derived Peptides: Production, Isolation, and Purification. In Proteins in Food Processing. Rickey Y. Yada, University of Guelph, Canada, Woodhead Publishing, UK. 2018, 389-412.
- Yan, Q. J. Isolation, Identification and Synthesis of Four Novel Antioxidant Peptides from Rice Residue Protein Hydrolyzed by Multiple Proteases. Food Chem. 2015, 179, 290–295. DOI: https://doi.org/10.1016/j.foodchem.2015.01.137.
- Banerjee, P.; Shanthi, C. Isolation of Novel Bioactive Regions from Bovine Achilles Tendon Collagen Having Angiotensin I-converting Enzyme-inhibitory Properties. Process Biochem. 2012, 47(12), 2335–2346. DOI: https://doi.org/10.1016/j.procbio.2012.09.012.
- Mora, L.; Reig, M.; Toldrá, F. Bioactive Peptides Generated from Meat Industry By-products. Food Res. Int. 2014, 65, 344–349. DOI: https://doi.org/10.1016/j.foodres.2014.09.014.
- Muguruma, M.; Ahhmed, A.; Katayama, K.; Kawahara, S.; Maruyama, M.; Nakamura, T. Identification of Pro-drug Type ACE Inhibitory Peptide Sourced from Porcine Myosin B: Evaluation of Its Antihypertensive Effects in Vivo. Food Chem. 2009, 114(2), 516–522.
- Terashima, M.; Baba, T.; Ikemoto, N.; Katayama, M.; Morimoto, T.; Matsumura, S. Novel Angiotensin-converting Enzyme (ACE) Inhibitory Peptides Derived from Boneless Chicken Leg Meat. J. Agric. Food Chem. 2010, 58(12), 7432–7436.
- A.O.A.C. Association of Official Analytical Chemists, 18 ed.; AOAC International: Maryland, USA, 2005.
- Jain, S.; Anal, A. K. Optimization of Extraction of Functional Protein Hydrolysates from Chicken Egg Shell Membrane (ESM) by Ultrasonic Assisted Extraction (UAE) and Enzymatic Hydrolysis. LWT - Food Sci. Technol. 2016, 69, 295–302. DOI: https://doi.org/10.1016/j.lwt.2016.01.057.
- Noman, A.; Xu, Y.; AL-Bukhaiti, W. Q.; Abed, S. M.; Ali, A. H.; Ramadhan, A. H.; Xia, W. Influence Of Enzymatic Hydrolysis Conditions On The Degree Of Hydrolysis And Functional Properties Of Protein Hydrolysate Obtained From Chinese Sturgeon (Acipenser sinensis) by using papain enzyme. Process Biochem. 2018, 67, 19–28. DOI: https://doi.org/10.1016/j.procbio.2018.01.009.
- Nielsen, P. M.; Petersen, D.; Dambmann, C. Improved Method for Determining Food Protein Degree of Hydrolysis. J. Food Sci. 2001, 66(5), 642–646. DOI: https://doi.org/10.1111/j.1365-2621.2001.tb04614.x.
- Abeyrathne, E. D.; Lee, H. Y.; Jo, C.; Suh, J. W.; Ahn, D. U. Enzymatic Hydrolysis of Ovomucin and the Functional and Structural Characteristics of Peptides in the Hydrolysates. Food Chem. 2016, 192, 107–113. DOI: https://doi.org/10.1016/j.foodchem.2015.06.055.
- Lovrien, R.; Matulis, D. Assays for Total Protein. In Commonly Used Techniques; John Wiley & Sons, Inc, 2005, 0(1), A.3A.1-A.3A.14.
- Chakka, A. K. In-vitro Antioxidant and Antibacterial Properties of Fermentatively and Enzymatically Prepared Chicken Liver Protein Hydrolysates. J. Food Sci. Technol. 2015, 52(12), 8059–8067.
- Binsan, W.; Benjakul, S.; Visessanguan, W.; Roytrakul, S.; Tanaka, M.; Kishimura, H. Antioxidative Activity of Mungoong, an Extract Paste, from the Cephalothorax of White Shrimp (Litopenaeus Vannamei). Food Chem. 2008, 106(1), 185–193.
- Giménez, B.; Alemán, A.; Montero, P.; Gómez-Guillén, M. C. Antioxidant and Functional Properties of Gelatin Hydrolysates Obtained from Skin of Sole and Squid. Food Chem. 2009, 114(3), 976–983.
- Cheng, F.-Y.; Wan, T.-C.; LIU, Y.-T.; Chen, C.-M.; Lin, L.-C.; SAKATA, R. Determination of angiotensin-I Converting Enzyme Inhibitory Peptides in Chicken Leg Bone Protein Hydrolysate with Alcalase. Anim. Sci. J. 2009, 80(1), 91–97.
- Bosch, L.; Alegría, A.; Farré, R. Application of the 6-aminoquinolyl-N-hydroxysccinimidyl Carbamate (AQC) Reagent to the RP-HPLC Determination of Amino Acids in Infant Foods. J. Chromatogr. B. 2006, 831(1–2), 176–183. DOI: https://doi.org/10.1016/j.jchromb.2005.12.002.
- Vittayanont, M.; Jaroenviriyapap, T. Production of Crude Chondroitin Sulfate from Duck Trachea. Int. Food Res. J. 2014, 21(2), 791.
- Khiari, Z.; Pietrasik, Z.; Gaudette, N. J.; Betti, M. Poultry Protein Isolate Prepared Using an Acid Solubilization/precipitation Extraction Influences the Microstructure, the Functionality and the Consumer Acceptability of a Processed Meat Product. Food Struct. 2014, 2(1–2), 49–60.
- Sukkhown, P.; Jangchud, K.; Lorjaroenphon, Y.; Pirak, T. Flavored-functional Protein Hydrolysates from Enzymatic Hydrolysis of Dried Squid By-products: Effect of Drying Method. Food Hydrocolloids. 2018, 76, 103–112. DOI: https://doi.org/10.1016/j.foodhyd.2017.01.026.
- Nchienzia, H. A.; Morawicki, R. O.; Gadang, V. P. Enzymatic Hydrolysis of Poultry Meal with Endo- and Exopeptidases. Poult. Sci. 2010, 89(10), 2273–2280. DOI: https://doi.org/10.3382/ps.2008-00558.
- Shu, G. Effect of Five Proteases Including Alcalase, Flavourzyme, Papain, Proteinase K and Trypsin on Antioxidative Activities of Casein Hydrolysate from Goat Milk. Acta Univ. Cibiniensis Ser. E Food Tech. 2015, 19(2), 66–74.
- Wouters, A. G. B.; Rombouts, I.; Fierens, E.; Brijs, K.; Delcour, J. A. Relevance of the Functional Properties of Enzymatic Plant Protein Hydrolysates in Food Systems. Compr. Rev. Food Sci. Food Saf. 2016, 15(4), 786–800.
- Leon-Lopez, A.; Fuentes-Jiménez, L.; Hernández-Fuentes, A. D.; Campos-Montiel, R. G.; Aguirre-Álvarez, G. Hydrolysed Collagen from Sheepskins as a Source of Functional Peptides with Antioxidant Activity. Int. J. Mol. Sci. 2019, 20(16), 3931.
- Pokorná, J.; Venskutonis, P. R.; Kraujalyte, V.; Kraujalis, P.; Dvořák, P.; Tremlová, B.; Kopřiva, V.; Ošťádalová, M. Comparison of Different Methods of Antioxidant Activity Evaluation of Green and roast C. Arabica and C. Robusta Coffee Beans. Acta Aliment. 2015, 44(3), 454–460.
- Matsui, R.; Honda, R.; Kanome, M.; Hagiwara, A.; Matsuda, Y.; Togitani, T.; Ikemoto, N.; Terashima, M. Designing Antioxidant Peptides Based on the Antioxidant Properties of the Amino Acid Side-chains. Food Chem. 2018, 245, 750–755. DOI: https://doi.org/10.1016/j.foodchem.2017.11.119.
- Aluko, R. E. Amino Acids, Peptides, and Proteins as Antioxidants for Food Preservation. In Handbook of Antioxidants for Food Preservation, 2015; pp 105–140.
- Lafarga, T.; O’Connor, P.; Hayes, M. Identification of Novel Dipeptidyl peptidase-IV and angiotensin-I-converting Enzyme Inhibitory Peptides from Meat Proteins Using in Silico Analysis. Peptides. 2014, 59, 53–62. DOI: https://doi.org/10.1016/j.peptides.2014.07.005.
- O’Sullivan, S. M. Bioactivity of Bovine Lung Hydrolysates Prepared Using Papain, Pepsin, and Alcalase. J. Food Biochem. 2017, 41(6): e12406.
- Nakade, K.; KAMISHIMA, R.; INOUE, Y.; AHHMED, A.; KAWAHARA, S.; NAKAYAMA, T.; MARUYAMA, M.; NUMATA, M.; OHTA, K.; Aoki, T.;; et al. Identification of an Antihypertensive Peptide Derived from Chicken Bone Extract. Anim. Sci. J. 2008, 79(6), 710–715.
- Je, J.-Y.; Lee, K.-H.; Lee, M. H.; Ahn, C.-B. Antioxidant and Antihypertensive Protein Hydrolysates Produced from Tuna Liver by Enzymatic Hydrolysis. Food Res. Int. 2009, 42(9), 1266–1272.
- Chiang, J. H.; Loveday, S. M.; Hardacre, A. K.; Parker, M. E. Effects of Enzymatic Hydrolysis Treatments on the Physicochemical Properties of Beef Bone Extract Using Endo- and Exoproteases. Int. J. Food Sci. Technol. 2019, 54(1), 111–120.
- Nguyen, H. T. M.; Sylla, K. S. B.; Randriamahatody, Z. Enzymatic Hydrolysis of Yellowfin Tuna (Thunnus Albacares) By-Products Using Protamex Protease. Food Technol. Biotechnol. 2011, 49(1), 48–55.
- Onuh, J. O.; Girgih, A. T.; Aluko, R. E.; Aliani, M. In Vitro Antioxidant Properties of Chicken Skin Enzymatic Protein Hydrolysates and Membrane Fractions. Food Chem. 2014, 150, 366–373. DOI: https://doi.org/10.1016/j.foodchem.2013.10.107.
- Kurozawa, L. E.; Park, K. J.; Hubinger, M. D. Optimization of the Enzymatic Hydrolysis of Chicken Meat Using Response Surface Methodology. J. Food Sci. 2008, 73(5), C405–12. DOI: https://doi.org/10.1111/j.1750-3841.2008.00765.x.