ABSTRACT
Plant-based meat products have the potential to alleviate meat shortages, and has a limited shelf life of a few days under refrigeration. The main cause of food spoilage is the microorganism growth. In order to investigate which bacteria caused the spoilage and spoilage of plant-based meat, high-throughput sequencing technology was used to determine the predominant spoilage bacterial flora in untreated vacuum-packed (Placed for 14 days) plant-based meat and vacuum-packed plant-based meat treated with a composite essential oil (Placed for 112 days) or composite preservative (Placed for 70 days). The dominant flora in vacuum-packed plant-based meat was Enterococcus (accounting for 86.2%). The main bacterial families in the compound essential oil treatment group were norank_chloroplast and Lactobacillaceae (accounting for 74.2% and 14.23% of total abundance), and in the compound preservative treatment group were norank_chloroplast, Pseudomonadaceae, and Carnobacteriaceae (accounting for 35.98%, 33.19%, and 17.96% of total abundance). Treatment with compound essential oil or compound preservative not only prolongs the shelf life of plant-based meat but also modifies the spoilage bacterial flora. Afterwards, research needs to be continued on the molds and fungi that cause the spoilage of plant-based meat, filling the gap in this research direction. Due to provide practical support for the development of the plant-based meat preservation industry.
Introduction
Demographers estimate that the global population will reach 9.7 billion by 2050 and will peak at 10.4 billion in 2080.[Citation1–3] Population growth not only puts great pressure on the environment and land resources but also causes problems such as food shortages. With the improving quality of contemporary life, people’s pursuit of meat products has also increased. Global availability of meat products is expected to become saturated in the future, due to limited land and food resources.[Citation4] Plant-based meat has emerged as a solution to alleviate meat shortages.[Citation5] Plant-based meat comprises plant proteins (such as soy, pea, or algae protein) as the main raw material, supplemented with other ingredients, and is produced through specific processing techniques (such as extrusion, cutting, and electrospinning), so that the final food product acquires the color, shape, and flavor of meat products. Modern experts and scholars have conducted extensive research and explorations on the optimal formula and processing technology to generate plant-based meat.[Citation6–13] However, during storage after processing, the quality of plant-based meat decreases rapidly due to microorganism growth, resulting in a great reduction in commercial value. In recent years, researchers have paid considerable attention to methods for the preservation of plant-based meat products. Plant-based meat has a limited shelf life of several d during refrigeration.[Citation14] Under 4°C refrigeration and vacuum conditions, the shelf life of plant-based meat is less than 14 days. Shelf life extended to 56 d under refrigeration for plant-based meats treated with a composite preservation solution comprising 1% chitosan, 2.5% tea polyphenols, and 0.04% nisin, and vacuum packaging.[Citation15] The fundamental cause of food spoilage is the growth and reproduction of microorganisms. Some existing studies on food storage have studied the analysis of microbial communities related to spoilage during storage, such as chicken and lamb. These microbial community analyses have important guiding significance for the storage and preservation of related foods. However, there is little research on the dominant microbial community of plant-based meat spoilage, and there is almost no research on the dominant microbial community of plant-based meat raw material spoilage. Only Geeraerts et al.[Citation16] examines the microbial flora of vegetarian meat products in the Belgian market using the (GTG) 5-PCR fingerprint method and determined that the predominant microflora was Lactobacillus sakei, Enterococcus faecium, and Carnobacterium divergens. Because of the different raw materials, the colony composition of microorganisms differs among various meat alternatives. Geeraerts et al. tested the spoilage microbiota of commercially available vegetarian products and did not test vegetarian ingredients. Wang et al.[Citation17] developed a PLA/PBAT film loaded with cinnamaldehyde that effectively delayed the growth of bacteria and prevented moisture evaporation and the texture properties of plant-based meat.
High-throughput sequencing refers to gene sequencing in a certain region of a sample using specific primers to identify and analyze various types of microbial genomes. Using this technology, the sequencing of microbial genomes can be completed in a short time.[Citation18] Therefore, high-throughput sequencing plays a crucial role in microbial identification in the field of food preservation. Here, the V3-V4 region of plant-based meat samples treated with a combination of natural preservatives and essential oils was measured using high-throughput sequencing technology, and the dominant bacterial community of spoilage was identified. At the same time, the influence and change of compound preservative and compound EO treatment on plant-based meat bacteria were compared. The study provides new ideas for the development of preservatives and a theoretical basis for selecting more targeted preservatives to improve the shelf life of plant-based meat.
Materials and methods
Materials
The following materials were used in this study: soybean protein isolate (SPI) (Shandong Yuwang Ecological Food Industry Co., Ltd., Dezhou, China); pea protein (Yantai Shuangta Food Co., Ltd., Yantai, China); wheat gluten (Fanxian Huanghe Industrial Co., Ltd., Fanxian, China); L-cysteine (Henan Wanbang Industry Co., Ltd., Zhengzhou, China). Sample treatments included cinnamon, clove, and honeysuckle EOs (all food grade; Hangzhou Tianyi Fragrance Fine Co., Ltd., Hangzhou, China); chitosan (deacetylation = 92%, molecular weight = 4.0 × 105), tea polyphenols, and nisin (food grade; Henan Wanbang Industrial Co., Ltd., Zhengzhou, China).
Preparation and treatment of plant-based meat
Preparation: The plant-based meat was prepared according to the method described by Dai et al..[Citation15] The formula – SPI (20%), wheat gluten (35%), pea protein (45%), L-cysteine (accounting for 5% of the total protein content), and rice bran protein (accounting for 0.05% of the total protein content) – obtained from the laboratory was fed to a 36–40 double screw extruder (Hunan Chuangxiang Intelligent Technology Co., Ltd), with the following parameters set at extrusion temperature: 160°C; screw speed: 280 r/min; solid addition: 8.5 kg/h; and water addition: 13.5 kg/h.
Treatment: The packaging bags were autoclaved at 121°C, 15 min before packaging. The extruded samples were cut into meat pieces (3 × 1 × 0.5 cm) in a sterile room, and the products were cooled to room temperature. For subsequent analyses, the generated plant-based products were divided into three groups as follows:
Untreated group (CK group): The products were vacuum sealed and stored at 4°C. The composition of bacterial flora was determined after 14 d. Composite EO group (1% cinnamon, 1% clove, and 10% honeysuckle EO group; referred to as J group): The samples were inserted into the composite preservation liquid, impregnated for 5 min, dried, sealed, put in 4°C refrigerator. Bacterial composition was determined after 112 d. Composite preservative group (1% chitosan, 2.5% tea polyphenols, and 0.04% nisin are referred to as K group): The samples were inserted into the composite preservation liquid, impregnated for 5 min, dried, and sealed in a 4°C refrigerator. Bacterial composition was determined after 70 d.
Sample detection: Shanghai Meiji Biomedical Technology Co., Ltd. Was commissioned to conduct the sequencing. The sequencing process is shown in ; the primer names and experimental materials are presented in , respectively.
Table 1. Primer names and sequences.
Table 2. Materials for PCR amplification[Citation19].
PCR response parameters:
1×(3 min, 95°C);
Circulation number (30 s, 95°C; 30 s, annealing temperature; 45 s, 72°C);
10 min, 72°C, 10°C, until the end.
PCR Amplifier: ABI GeneAmp® 9700.
Biodiversity analysis
The dilution curve represents a random number of sequences extracted from a sample and is used to estimate the Alpha diversity index. The abscissa represents the number of reads extracted from a sample and the ordinate represents the values of the Alpha diversity index; evaluation of whether the amount of sequencing data is sufficient follows, based on whether the drawn curve is gentle.[Citation20,Citation21] The Alpha diversity index includes the Sobs, Shannon, Simpson, and Ace indices.
Statistical analysis
Data processing and mapping analysis were performed using the Meiji Biocloud platform (vip.majorbio.com). Significant differences were determined using Tukey’s test (at p < .05), using Origin 2019b (IBM, Chicago, IL, USA). Three parallel experiments were performed for each experimental group.
Results
High-throughput sequencing results
High-throughput sequencing of the 16-S rRNA V3–V4 region in the three samples produced a total of 253,505 effective sequences, with an average length of 419.5, a base number of 17,763,164, a Chao index of 64, and 99.97% coverage. These findings indicate that all bacteria in the plant-based meat were detected and confirm the reliability of the obtained data. For the bioinformatic statistical analysis of operational taxonomic units (OTUs) at 97% similarity, the microorganisms in the plant-based meat were classified into 1 bound, 11 phyla, 16 classes, 41 orders, 51 families, 68 genera, and 197 species, and 116 OTUs were obtained.
Assessment of spoilage bacterial diversity in plant-based meat
Species-taxonomic annotation of OTUs was performed, and the abundance information corresponding to each OTU annotation result per sample was evaluated. The adequacy of sample size can be assessed based on whether the Pan/core species curve flattens.[Citation22] The core species curve in flattened out, indicating a sufficient sample size. As illustrated in , the Shannon dilution curve flattened out, indicating that the sequencing data were sufficient. Rank – abundance curves can be used to explain two aspects of diversity, namely, species richness and community uniformity. In the horizontal direction, species richness is reflected by the width of the curve: the larger the curve on the horizontal axis, the higher the species richness. The shape of the curve (flattening degree) reflects the uniformity of the community in the sample: the flatter the curve, the more uniform the species distribution.[Citation23,Citation24] As shown in , the rapid and steep decline of the curve for the CK group indicates a high proportion of dominant flora and low diversity in the sample. Bacterial abundance was greater in the J and K groups than in the CK group. In conclusion, high-throughput sequencing can cover the diversity of plant-based meat bacteria.
Alpha diversity index analysis
Microbial diversity can reflect the richness and diversity of microbial communities based on the analysis of single-sample diversity (Alpha diversity)[Citation25] (.) The Sobs index represents the actual observations of richness,[Citation26] with larger values indicating greater community richness. One of the indices used by Simpson to estimate microbial diversity in a sample is commonly used in ecology to quantitatively describe the biodiversity of a region.[Citation27,Citation28] Larger values of the Simpson index indicate lower community diversity. The Shannon index, which is used to estimate microbial diversity in a sample, is similar to the Simpson diversity index and is often used to reflect community alpha diversity. Larger Shannon values indicate higher community diversity. The Chao index that estimates the number of OTUs contained in a sample using the Chao1 algorithm is commonly used in ecology to estimate the total number of species.[Citation29] The Sobs, Shannon, and Chao indices of the CK group were significantly lower than those of the J and K groups, whereas the Simpson index of the CK group was higher than that of the other groups. This indicates that the CK group species abundance was reduced compared to that of the other two treatment groups. Statistically significant differences observed between the CK and the J groups. In contrast, the K group did not differ statistically from the other two groups.
Principal component analysis (PCA) of the plant-based artificial meat spoilage microflora
PCA uses variance decomposition to reflect the differences of multiple groups of data in a two-dimensional coordinate map. The coordinate axis takes two eigenvalues that reflect the differences between samples.[Citation30,Citation31] The closer the distance between samples in the PCA plot, the more similar the sample species composition is. Conversely, samples with different species composition tend to be further apart.[Citation32] As shown in , the cumulative contribution rate of the first (PC1) and the second (PC2) principal components for the three different treatments is 99.93%, confirming the main characteristics of the three samples. The three samples were distributed in different quadrants and were not clustered. This clearly indicates the differences in the bacterial flora composition after the three treatments.
Abundance of the plant-based meat spoilage microflora at the family-level
The Venn diagram of shows 21 bacteria in the CK group, 44 in the J group, and 41 in the K group. Of these, 17 bacteria were present in all three treatment groups. As shown in , the CK group comprised 86.2% of Enterococcaceae, 7.81% of Staphylococcaceae, 3.41% of Moraxellaceae, and 1.49% of other families. The J group comprised 74.2% of norank_chloroplast, 14.23% of Lactobacillaceae, 3.69% of Streptococcaceae, 2.47% of Comamonadaceae, 1.31% of Micrococcaceae, 1.06% of Enterococcaceae, and 3.04% of other bacteria. The compound preservative treatment group was composed of 35.98% of norank_chloroplast, 33.19% of Pseudomonadaceae, 17.96% of Carnobacteriaceae, 6.27% of Lactobacillaceae, 2.36% of Comamonadaceae, 1.90% of Streptococcaceae, 0.94% of Enterococcaceae, and 1.40% of other flora. In conclusion, vacuum-packed plant-based meat was dominated by Enterococcaceae. The bacterial composition of plant-based meat treated with compound EO or preservative was substantially different from that of the untreated vacuum-packed product.
Figure 8. Bar graphs of the bacterial abundance in the microbiota of the different groups. Relative abundance is indicated at the family level.
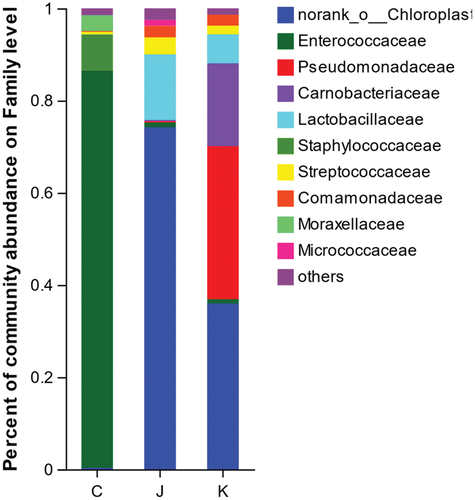
Discussion and conclusion
High-throughput sequencing was used to identify the major spoilage bacterial flora in plant-based meat in untreated (14 d), composite-EO-treated (1% cinnamon, 1% clove, and 10% honeysuckle EOs) (112 d), and compound-preservative-treated (1% chitosan, 2.5% tea polyphenols, and 0.04% nisin) (70 d) vacuum-packed meat. The dominant bacterial flora in the untreated plant-based meat was Enterococcaceae. The bacterial composition of the plant-based meat treated with compound EOs and compound preservatives was considerably different. The main bacterial families in the compound-EO treatment group were norank_chloroplast and Lactobacillaceae, accounting for 74.2% and 14.23% of total abundance, respectively. The major bacterial families in the compound-preservative treatment group were norank_chloroplast, Pseudomonadaceae, and Carnobacteriaceae, accounting for 35.98%, 33.19%, and 17.96% of total abundance, respectively.
Using high-throughput sequencing technology, the V3-V4 regions of three sets of 16S samples were sequenced, and the dominant bacterial communities of spoilage in the three sets of samples were obtained. Due to the antimicrobial substances in biopreservative s and plant essential oils, makes treatment with compound preservatives or compound EO not only prolongs the shelf life of plant-based meat but also alters the composition of plant-based meat spoilage bacteria. This study provides a theoretical basis for the future selection of more targeted plant-based meat preservatives and provides a practical support for the development of the plant-based meat preservation industry. However, there are also certain limitations. This study did not investigate the effect of the bacterial flora under three different treatments for the same days. There is a lack of research on the dominant spoilage microbiota of plant-based meat, 16S (third-generation) mold and fungi. As well as the biotoxicity of biopreservative and plant essential oil and the harm to human body will be studied, so that consumers can get more secure and safe plant-based meat.
Author contributions
Conceptualization, F.L. and Z.X.; methodology, Z.D., L.H., M.G., Y.Z., and T.X.; validation, F.L.; formal analysis, Z.D. and L.H.; investigation, Z.D., L.H., M.G., and Y.Z.; data curation, Z.D.; writing – original draft preparation, Z.D.; writing – review and editing, F.L. and T.X.; visualization, Z.D. and T.X.; supervision, F.L. and Z.X.; project administration, F.L.; funding acquisition, F.L. and Z.X. Z.D. and Z.X. contributed equally to this work. All authors have read and agreed to the published version of the manuscript.
Data availability statement
The datasets generated for this study are available upon request from the corresponding author.’
Disclosure statement
No potential conflict of interest was reported by the author(s).
Additional information
Funding
References
- Ahmad, M.; Qureshi, S.; Akbar, M. H.; Siddiqui, S. A.; Gani, A.; Mushtaq, M.; Hassan, I.; Dhull, S. B. Plant-Based Meat Alternatives: Compositional Analysis, Current Development and Challenges. Appl. Food Res. 2022, 2(2), 100154. DOI: 10.1016/J.AFRES.2022.100154.
- Bohrer, B. M. An Investigation of the Formulation and Nutritional Composition of Modern Meat Analogue Products. Food Sci. Hum. Wellness. 2019, 8(4), 320–329. DOI: 10.1016/j.fshw.2019.11.006.
- Bryant, C. J. We Can’t Keep Meating Like This: Attitudes Towards Vegetarian and Vegan Diets in the United Kingdom. Sustainability-Basel. 2019, 11(23), 6844. DOI: 10.3390/su11236844.
- Hartmann, C.; Siegrist, M. Our Daily Meat: Justification, Moral Evaluation and Willingness to Substitute. Food Qual. Prefer. 2020, 80, 103799. DOI: 10.1016/j.foodqual.2019.103799.
- Graca, J.; Godinho, C. A.; Truninger, M. Reducing Meat Consumption and Following Plant-Based Diets: Current Evidence and Future Directions to Inform Integrated Transitions. Trends Food Sci. Tech. 2019, 91, 380–390. DOI: 10.1016/j.tifs.2019.07.046.
- Kaleda, A.; Talvistu, K.; Vaikma, H.; Tammik, M. L.; Rosenvald, S.; Vilu, R. Physicochemical, Textural, and Sensorial Properties of Fibrous Meat Analogs from Oat-Pea Protein Blends Extruded at Different Moistures, Temperatures, and Screw Speeds. Fut. Foods. 2021, 4, 100092. DOI: 10.1016/J.FUFO.2021.100092.
- Carmo, C. S. D.; Knutsen, S. H.; Malizia, G.; Dessev, T.; Geny, A.; Zobel, H.; Myhrer, K. S.; Varela, P.; Sahlstrøm, S. Meat Analogues from a Faba Bean Concentrate Can Be Generated by High Moisture Extrusion. Fut. Foods. 2021, 3, 100014. DOI: 10.1016/J.FUFO.2021.100014.
- Schreuders, F. K. G.; Dekkers, B. L.; Bodnar, I.; Erni, P.; Boom, R. M.; van der Goot, A. J. Comparing Structuring Potential of Pea and Soy Protein with Gluten for Meat Analogue Preparation. J. Food Eng. 2019, 261, 32–39. DOI: 10.1016/j.jfoodeng.2019.04.022.
- Immonen, M.; Chandrekusuma, A.; Sibakov, J.; Poikelispaa, M.; Tuula, S. S. Texturization of a Blend of Pea and Destarched Oat Protein Using High-Moisture Extrusion. Foods. 2021, 10(7), 1517. DOI: 10.3390/FOODS10071517.
- Chiang, J. H.; Tay, W.; Ong, D. S. M.; Liebl, D.; Ng, C. P.; Henry, C. J. Physicochemical, Textural and Structural Characteristics of Wheat Gluten-Soy Protein Composited Meat Analogues Prepared with the Mechanical Elongation Method. Food Struct. 2021, 28, 100183. DOI: 10.1016/J.FOOSTR.2021.100183.
- Dekkers, B. L.; Boom, R. M.; Goot, A. J. V. D. Structuring Processes for Meat Analogues. Trends Food Sci. Tech. 2018, 81, 25–36. DOI: 10.1016/j.tifs.2018.08.011.
- Dekkers, B. L.; Nikiforidis, C. V.; Goot, A. J. V. D. Shear-Induced Fibrous Structure Formation from a Pectin/SPI Blend. Innov. Food Sci. Emerg. 2016, 36, 193–200. DOI: 10.1016/j.ifset.2016.07.003.
- Zhang, J. C.; Liu, L.; Liu, H. Z.; Yoon, A.; Rizvi, S. S. H.; Wang, Q. Changes in Conformation and Quality of Vegetable Protein During Texturization Process by Extrusion. Crit. Rev. Food Sci. 2019, 59(20), 3267–3280. DOI: 10.1080/10408398.2018.1487383.
- Sun, C. X.; Ge, J.; He, J.; Gan, R. Y.; Fang, Y. P. Processing, Quality, Safety, and Acceptance of Meat Analogue Products. Engineering. 2021, 7(5), 674–678. DOI: 10.1016/j.eng.2020.10.011.
- Dai, Z. H.; Han, L. N.; Li, Z.; Gu, M. Q.; Xiao, Z. G.; Lu, F. Combination of Chitosan, Tea Polyphenols, and Nisin on the Bacterial Inhibition and Quality Maintenance of Plant-Based Meat. Foods. 2022, 1524, 11. DOI: 10.3390/FOODS11101524.
- Geeraerts, W.; Vuyst, L. D.; Leroy, F. Ready-To-Eat Meat Alternatives, a Study of Their Associated Bacterial Communities. Food Biosci. 2020, 37, 100681. DOI: 10.1016/j.fbio.2020.100681.
- Wang, L.; Xu, J. G.; Zhang, M. M.; Zheng, H.; Li, L. Preservation of Soy Protein-Based Meat Analogues by Using PLA/PBAT Antimicrobial Packaging Film. Food Chem. 2022, 380, 132022. DOI: 10.1016/J.FOODCHEM.2021.132022.
- Zhao, W. H.; Liang, Z.; Qian, M.; Li, X. L.; Dong, H.; Bai, W. D.; Wei, Y. L.; He, S. G. Evolution of Microbial Communities During Fermentation of Chi-Flavor Type Baijiu as Determined by High-Throughput Sequencing. LWT-Food Sci. Technol. 2022, 170, 114102. DOI: 10.1016/j.lwt.2022.114102.
- Wang, G.; Xu, X.; Zhou, L.; Wang, C.; Yang, F. A Pilot-Scale Study on the Start-Up of Partial Nitrification-Anammox Process for Anaerobic Sludge Digester Liquor Treatment. Bioresource. Technol. 2017, 241, 181–189. DOI: 10.1016/j.biortech.2017.02.125.
- Zhang, Q. Q.; Li, D.; Zhang, W.; Jiang, M.; Chen, X. H.; Dong, M. S. Comparative Analysis of the Bacterial Diversity of Chinese Fermented Sausages Using High-Throughput Sequencing. LWT-Food Sci. Technol. 2021, 150, 111975. DOI: 10.1016/J.LWT.2021.111975.
- Blanco, C.; Verbanic, S.; Seelig, B.; Chen, I. A. Easy Diver: A Pipeline for Assembling and Counting High-Throughput Sequencing Data from in vitro Evolution of Nucleic Acids or Peptides. J. Mol. Evol. 2020, 88(6), 477–481. DOI: 10.1007/s00239-020-09954-0.
- Pichler, M.; Coskun, Ö. K.; Ortega-Arbulú, A. S.; Conci, N.; Wörheide, G.; Vargas, S.; Orsi, W. D. A 16S rRNA Gene Sequencing and Analysis Protocol for the Illumina MiniSeq Platform. Microbiol. Open. 2018, 7(6), e00611. DOI: 10.1002/mbo3.611.
- Gulin, V.; Vlaičević, B.; Sertić, P. M.; Rebrina, F.; Matoničkin, K. R. Taxonomic and Functional Metrics of Ciliates and Amoeboid Protists in Response to Stream Revitalization. Front. Microbiol. 2022, 13, 842395. DOI: 10.3389/FMICB.2022.842395.
- Bates, S. T.; Clemente, J. C.; Flores, G. E.; Walters, W. A.; Parfrey, L. W.; Knight, R.; Fierer, N. Global Biogeography of Highly Diverse Protistan Communities in Soil. Isme J. 2013, 7(3), 652–659. DOI: 10.1038/ismej.2012.147.
- Liu, W. J.; Li, W. C.; Zheng, H. J.; Kwok, L. Y.; Sun, Z. H. Genomics Divergence of Lactococcus lactis Subsp. Lactis Isolated from Naturally Fermented Dairy Products. Food. Res. Int. 2022, 155, 111108. DOI: 10.1016/J.FOODRES.2022.111108.
- Donohue, M. E.; Rowe, A. K.; Kowalewski, E.; Hert, Z. L.; Karrick, C. E.; Randriamanandaza, L. J.; Zakamanana, F.; Nomenjanahary, S.; Andriamalala, R. Y.; Everson, K. M., et al. Significant Effects of Host Dietary Guild and Phylogeny in Wild Lemur Gut Microbiomes. ISME Commun. 2022, 2(1), 33. DOI: 10.1038/s43705-022-00115-6.
- Forster, S. C.; Clare, S.; Beresfordjones, B. S.; Harcourt, K.; Notley, G.; Stares, M. D.; Kumar, N.; Soderholm, A. T.; Adoum, A.; Wong, H., et al. Identification of Gut Microbial Species Linked with Disease Variability in a Widely Used Mouse Model of Colitis. Nat. Microbiol. 2022, 7(4), 590–599. DOI: 10.1038/s41564-022-01094-z.
- Behairi, S.; Baha, N.; Barakat, M.; Ortet, P.; Achouak, W.; Heulin, T.; Kaci, Y. Bacterial Diversity and Community Structure in the Rhizosphere of the Halophyte Halocnemum Strobilaceum in an Algerian Arid Saline Soil. Extremophiles. 2022, 26(2), 18. DOI: 10.1007/S00792-022-01268-X.
- Ma, J. M.; Ma, K.; Liu, J. L.; Chen, N. N. Rhizosphere Soil Microbial Community Under Ice in a High-Latitude Wetland: Different Community Assembly Processes Shape Patterns of Rare and Abundant Microbes. Front. Microbiol. 2022, 13, 783371. DOI: 10.3389/FMICB.2022.783371.
- Mori, A. S.; Isbell, F.; Seidl, R. β-Diversity, Community Assembly, and Ecosystem Functioning. Trends Ecol. Evol. 2018, 33(7), 549–564. DOI: 10.1016/j.tree.2018.04.012.
- Chetcuti, J.; Kunin, W. E.; Bullock, J. M. Habitat Fragmentation Increases Overall Richness, but Not of Habitat-Dependent Species. Front Ecol. Evol. 2020, 8, 607619. DOI: 10.3389/FEVO.2020.607619.
- Borges, S. L. C.; Pavao, D. C.; Elias, R. B.; Moura, M.; Ventura, M. A.; Silva, L. Taxonomic, Structural Diversity and Carbon Stocks in a Gradient of Island Forests. Sci. Rep. 2022, 12(1), 1038. DOI: 10.1038/s41598-022-05045-w.