ABSTRACT
Physicochemical and digestion properties of chitosan modified starch prepared by dry heat treatment were investigated. Starch granule aggregation occurred following the addition of chitosan and this trend was enhanced with increasing chitosan concentration. The size of chitosan modified starch particles was about six times of that of the native starch granules. Interactions between the hydroxyl groups of starch and the amino groups of chitosan was confirmed by Fourier transform infrared spectroscopy analysis. The disappearance of the trough in the Rapid Visco Analyzer profile indicated that the cross-links increased the starch shear resistance. The cross-links between starch and chitosan also reduced starch digestion rate compared to the control (p < 0.001), and this reduction was further enhanced with an increasing chitosan concentration. Additionally, the addition of chitosan altered starch digestion kinetics from one phase to two phases, implying that chitosan modification changed both starch structure and its digestion behaviors.
RESUMEN
Se investigaron las propiedades fisicoquímicas y digestivas del almidón modificado con chitosán preparado mediante tratamiento de calor seco. El agregado de gránulos de almidón ocurrió después de la adición de chitosán y esta tendencia mejoró con el aumento en la concentración de chitosán. El tamaño de las partículas de almidón modificado con chitosán fue alrededor de 6 veces mayor que aquel de los gránulos de almidón nativos. Se confirmaron las interacciones entre los grupos hidroxilo del almidón y los grupos amino del chitosán mediante el análisis espectroscópico de infrarrojos de transformada de Fourier. La desaparición de la canalización en el perfil del analizador de viscosidad (RVA) indicó que la reticulación produjo un aumento en la resistencia al corte del almidón. La reticulación entre el almidón y el chitosán también redujo el ritmo digestivo del almidón en comparación con la muestra control (p < 0,001). Esta reducción fue posteriormente mejorada con un aumento en la concentración de chitosán. Además, la adición de chitosán alteró la cinética digestiva del almidón de una a dos fases, implicando que la modificación con chitosán comportó cambios en la estructura y comportamiento digestivo del almidón.
PALABRAS CLAVE:
1. Introduction
Starch is the most abundant storage glucan composed of two main structural components, amylose and amylopectin (Sajilata, Singhal, & Kulkarni, Citation2006). Enzyme-driven starch hydrolysis is one of the fundamental metabolic processes in human digestion to maintain a proper blood glucose level. Based on the rate of digestion, starch can be classified as rapidly digestible starch (RDS), slowly digestible starch (SDS), and resistant starch (RS) (Englyst, Kingman, & Cummings, Citation1992). RDS and SDS, though differ in their digestion rates, are both digested completely in the small intestine, but cause a different increased glucose in the blood glucose levels (Chung, Shin, & Lim, Citation2008; Zhang & Hamaker, Citation2009). In recent years, the effect of RS on human health has gained increasing interest in that it is not digested in the upper gastrointestinal tract, but is fermented in the large intestine with beneficial effects for the gut environment (Lafiandra, Riccardi, & Shewry, Citation2014). The applications of SDS and RS in foods can moderate glycemic response and maintain a healthy microorganism profile in the human gut.
Previous studies suggest that the granular and molecular structures of starch change after dry heat treatment (Chung, Min, Kim, & Lim, Citation2007). Dry heat treatment at 160ºC for 3.5 h is a normally used method to produce modified starches (Chiu, Schiermeyer, Thomas, & Shah, Citation1998). Compared to other chemical modification methods, dry heating is a simple, safe method and produces little if any by-products. Chiu et al. (Citation1999) reported that thermally treated starches were functionally equivalent to the chemically cross-linked starches and more recent reports have described the properties and potential applications of starch-polysaccharide blends or suspensions (Liu, Adhikari, Guo, & Adhikari, Citation2013; Sasaki & Kohyama, Citation2012). Further modification includes the addition of certain kinds of gums (hydrocolloids) into starch which can effectively modify starch properties such as texture, water mobility, and stability (Rosell, Yokoyama, & Shoemaker, Citation2011; Sikora, Kowalski, & Tomasik, Citation2008; Tischer, Noseda, De Freitas, Sierakowski, & Duarte, Citation2006). The addition of gum also strongly influences the viscosity of the mixtures, and these changes in the viscosity of starch suspensions or paste are considered to be associated with the interactions between starch and gums (Freitas, Gorin, Neves, & Sierakowski, Citation2003). Previous study found that the paste viscosity of waxy maize starch was increased by dry heating with sodium alginate or carboxymethyl-cellulose (CMC), but that of potato starch was reduced (Lim, Han, Lim, & BeMiller, Citation2002). Their further study demonstrated that dry heat treatment with xanthan altered the paste viscosity of the modified starches at the greatest extent, compared to sodium alginate and CMC (Lim, BeMiller, & Lim, Citation2003). When rice starches with different amylose content were heat-treated in a dry state after being dispersed with low or medium viscosity CMC, the modified waxy starch exerted significant changes in viscosity throughout pasting, indicating that the interactions occurs between the hydroxyl groups of amylopectin of rice starch and carboxylate acid groups of CMC (Li, Shoemaker, Ma, Shen, & Zhong, Citation2008). Among the normally used gums, chitosan has attracted interest in that it is a nontoxic, biodegradable, and biocompatible cationic polysaccharide (Mathew, Brahmakumar, & Abraham, Citation2006). However, the unique properties of chitosan are attributed to its strong mechanical strength and flexibility, biodegradable nature, and antibacterial characteristics (Bangyekan, Aht-Ong, & Srikulkit, Citation2006). Many of the previous studies describing the interactions between starch and chitosan have focused on the development of edible film preparations for packing materials (Liu et al., Citation2013; Muscat, Adhikari, Adhikari, & Chaudhary, Citation2012). Considering the existence of protonated amino groups (NH2) in chitosan molecules, its unique structure may contribute to the development of chitosan modified starch granules through interactions between the two polymers. This cross-linked product may show improved properties in terms of the modified starch but could also be used for delivering chitosan to the human gut. There are limited reports in the literature on the interaction between starch and chitosan under dry conditions and the properties of the chitosan modified starch. To the best of our knowledge, there has been no report describing the effect of the interactions between starch and chitosan on starch digestion properties. Thus, in this current study, waxy maize starch was selected as a model for studying these interactions, and chitosan modified waxy starches were prepared using dry heat treatment, and physicochemical and digestion properties of the modified starch were examined using a number of techniques, such as SEM, RVA, FTIR, and enzyme digestion. This study extends our previous research of the effect of chitosan modification on starch thermal and antibacterial properties (Zhou, Zheng, Wang, Yang, & Chu, Citation2015).
2. Materials and methods
2.1. Materials
Waxy maize starch was purchased from Huanong Co. Ltd (Dezhou, China). Chitosan with a deacetylation degree of 75–85% was obtained from Jinrun Ltd (Henan, China). Artificial saliva α-amylase (A-3176, Type VI-B from porcinepancreas), amyloglucosidase (A7095, from Aspergillusniger), and pepsin (P6887, from porcine gastric mucosa) were purchased from Sigma-Aldrich. Glucose assay kit (GOPOD method) was obtained from Megazyme International Ireland. Other chemicals were reagent grade and used as received.
2.2. Sample preparation
Samples of 1.5, 3.0, or 4.5 g of chitosan powder was mixed with 300 mL 1% acetic acid solution, respectively, and stirred at room temperature until the chitosan was completely dissolved. Samples of 48.5, 47.0, or 45.5 g of waxy starch was then added into the above prepared chitosan solution and stirred for 1 h until complete dispersion of starch granules. The mixture was then neutralized using NaOH to pH 5.0, and then spray dried (Mobile Minor TM, GEA, China) at the conditions of an inlet air temperature of 200ºC and an outlet air temperature of 95ºC to produce starches containing 3%, 6%, and 9% chitosan, respectively. The obtained starch powder was further dehydrated in an oven at 50ºC until the moisture content was less than 3%, and then further heat-treated at 135ºC for 2 h to allow interactions to occur between starch and chitosan molecules (Li et al., Citation2008; Lim et al., Citation2003). A control sample was prepared but without chitosan addition. Meanwhile, the examination of native starch was also included in this study for understanding the effect of dry heat treatment on starch property.
2.3. Scanning electron microscope (SEM)
The morphology of the prepared starch samples was visualized using a SEM (KYKY-EM6000C, Hitachi Limited, Japan). The starch samples were directly deposited on aluminum stubs using a double-sided adhesive tape and then coated using a thin gold layer with gold sputter instrument. An accelerating potential of 12 kV was used for imaging.
2.4. Particle size distribution
The particle size distribution of the prepared starch samples was determined using a laser particle size analyzer (BT-9300S, Bettersize, China). The conditions used for the measurement were a refraction parameter of 1.52, absorbance 0.01, and an obscuration factor from 10% to 20%. The starch samples were transferred to the instrument’s dispersion circulator tank, which contained deionized water and the starch samples were added into the circulator tank until yielding an obscuration factor up to around 12%.
2.5. Fourier transform infrared analysis (FTIR)
The IR spectra of samples were measured using a Fourier transform infrared (FTIR) spectrometer (VECTOR 22, Bruker, German) at a wavenumber range of 4000–600 cm−1. The resolution and signal to noise ratio (SNR) were 0.4 cm−1 and 15,000:1, respectively. One hundred milligrams of sample was used for each test and each sample was vacuum sealed in a water-resistant polyethylene pack and stored in a container containing silica gel desiccant before the IR tests.
2.6. The pasting properties
RVA characteristics in regards to starch pasting properties were determined using a RVA analyzer (Perten, Sweden) following the standard operating procedures. Briefly, 2.5 g of starch sample was mixed with 25.0 mL of distilled water, incubated at 50ºC for 1 min, then heated from 50ºC to 95ºC at a rate of 15ºC∙min−1, maintained at 95ºC for 2.5 min, and then finally cooled down to 50ºC at a rate of 15ºC∙min−1 before incubating at 50ºC for 2.5 min. The samples were then stirred at 160 rpm. Each sample was measured in triplicate.
2.7. Starch digestion
The starch sample was digested through a simulated human small intestinal digestion system following the method described by Htoon et al. (Citation2009). Briefly, the starch was pretreated using artificial saliva α-amylase in a carbonate buffer at pH 7.0, and then mixed with pepsin after acidification by HCl. The mixture was neutralized with NaOH, and then was digested by pancreatin and amyloglucosidase at physiological pH (6.0) and temperature (37ºC) and incubated for up to 12 h. At different intervals, the supernatant was removed at the end of the chosen incubation time by centrifugation and analyzed for glucose content. After the digestion, the indigestible starch (i.e. resistant starch, RS) was collected, freeze dried, and analyzed for its physicochemical properties. The digestibility for each starch was calculated as the percentage of digested starch in the total starch at the designated incubation intervals. The amount of released glucose from the digested starch was measured using the Megazyme glucose assay kit (GOPOD method), and the amount of digested starch was calculated after the conversion of released glucose into starch by use of factor 0.9.
2.8. Logarithm of slope (LOS) analysis
The Logarithm of Slope (LOS) method was used for investigating the kinetics of the starch digestion process (Poulsen, Ruiter, Visser, & Iversen, Citation2003). The glucose released from the starch granules into the solution at a given time t can be calculated by the following equation:
where Glu is the glucose concentration in the solution at the time of t, and Glu∞ is the glucose concentration in the solution after reaching an equilibrium state. The order parameter t1 is a characteristic quantity related to the glucose releasing rate, and can be viewed as the first passage time of the glucose concentration of . To depict the kinetics more clearly, one can investigate the dependence of the production rates of the free glucose, i.e. the differential of Glu on the timescale. Thus, the equation can be evaluated as followings:
Here, was defined as LOS. From formula (2) one can see that, for a given set of Glu∞ and t1, the dependence of LOS and t showed a linear behavior. Thus, the kinetics of starch digestion can be analyzed using the plots of LOS, i.e. if the linear fitting of LOS and t has an outstanding value of R2, the digestion process should be considered as a one-phase process. On the other hand, if two or more straight lines with an outstanding R2 are introduced for fitting the plots of LOS, the kinetics should be interpreted to fit two or more phase kinetics.
In this study, the curves of the formula (1) were fitted from experimental data based on the least square method. The LOS values were calculated based on formula (1), and the values of different glucose concentration were computed as followings:
2.9. Statistical analysis
The statistical analysis of results was conducted by a pooled t-test and analysis of variance (ANOVA). Samples were analyzed at least three times and the significant difference was defined as p < 0.05.
3. Results and discussion
3.1. Starch morphology by SEM
The morphologies of waxy starch granules before and after dry heat treatment were analyzed using SEM, and the images showed that native waxy maize starch granules had an irregular polyhedral shape with clear edges and corners ((a)). It also demonstrated a smooth surface, consistent with previous work by Sandhu, Kaur, Singh, and Lim (Citation2008). Following the dry heat treatment, the adjacent granules were aggregated to form a larger particle, which demonstrated some irregularities on the surfaces, an effect of dry heat treatment ((b)). With the addition of chitosan, the starch granules seemed to be wrapped by the chitosan molecules through multiple points of the connections, resulting in an increase in aggregated granules ((c–e)). The morphology of chitosan modified starch displayed considerable differences with that of native starch. Moreover, increased chitosan addition influenced starch morphology. For example, there was a lower level of adherence for starch granules when 3% chitosan was used in the system ((c)). However, more aggregated granules were formed and became inseparable when the concentration of chitosan was increased to 6%, which might be related to the increased interactions between chitosan and starch molecules ((d)). Finally, even larger particles were formed when chitosan concentration was up to 9%, suggesting that the linkages between chitosan and starch granules became tighter compared to those with lower chitosan addition ((e)). In summary, the interaction between chitosan and starch granules greatly altered particles morphology.
Figure 1. Morphology of waxy maize starch.(a) before dry heating; (b) after dry heating; (c) starch modified with 3% chitosan; (d) starch modified with 6% chitosan; (e) starch modified with 9% chitosan.
Figura 1. Morfología del almidón de maíz ceroso: (a) antes del tratamiento de calor seco, (b) después del tratamiento de calor seco, (c) almidón modificado con 3% de chitosán; (d) almidón modificado con 6% de chitosán; (e) almidón modificado con 9% de chitosán.
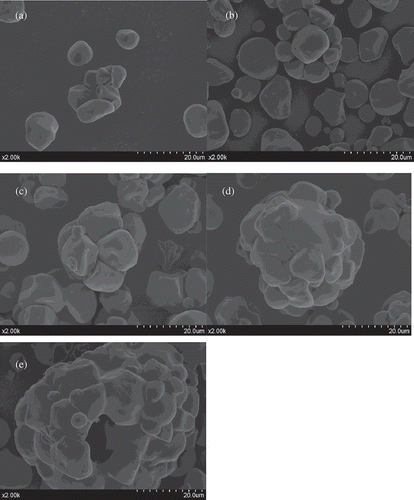
3.2. Particle size distribution
The particle size distribution of each starch sample was measured to study the changes in granule size and the possible aggregation caused by both heat treatment and the addition of chitosan. shows that the peak of the particle size distribution curve was increased following dry heat treatment (without chitosan addition) although this shift was not significant compared to the native starch (p > 0.05). Moreover, dry heat treatment also caused the particle size distribution curve to become broader. The plausible interpretation for these changes caused by dry heat treatment may be due to the formation of aggregated particles which is consistent with the observations from SEM. Alternatively, the expansion of the starch granules caused by the heat treatment might also be another factor contributing to the changes in granule size distribution patterns. The shape of the granule size distribution curves of chitosan modified starches showed a single peak, suggesting that the interactions between starch and chitosan occurred evenly in the reaction system. Meanwhile, the main particle size increased to around 60 μm, which is almost six times larger than the native starch granules. This distribution character may also indicate that the starch granules were aggregated together and wrapped by the chitosan molecules. Consistently, the main peak of the particle size distribution shifted toward a higher value following the increased chitosan addition from 3% to 9% (). Among the three chitosan modified starches, there was a similar granule distribution curve with 3% and 6% chitosan addition, but the addition of chitosan at 9% level led to a significant change in the distribution ().
3.3. Interactions between starch and chitosan by FTIR
The effect of chitosan modification on starch IR spectral characteristics was examined and the results are displayed in . The spectrum of chitosan molecules was characterized by three main absorptions. The first absorption in the spectrum demonstrated a broad band ranging from 3600 ~ 3100 cm−1, which is attributed to N–H and O–H stretching vibrations. The absorbance band at 3423.03 cm−1 is associated with intermolecular hydrogen bonding of chitosan molecules (De Vasconcelos et al., Citation2006). The second, a weak absorption located at 2921.23 cm−1 was associated with C–H stretching (Zivanovic, Li, Davidson, & Kit, Citation2007), and the third was related to the amide-I band located around 1646.91 cm−1 (Wan, Wu, Yu, & Wen, Citation2006). There was also a broad band ranging between 1180 and 953 cm−1, which is typical of saccharide bands (Jiugao, Ning, & Xiaofei, Citation2005). For native starch, the typical absorption band at 3417.24 cm−1 was attributed to the hydrogen-bonded hydroxyl groups associated with free inter- and intramolecular-bonded hydroxyl group; the bands located at 2934.12 cm−1 and 2894.63 cm−1 were attributed to C–H stretching, and the band at 1638.19 cm−1 was assigned to the δ(O–H) bending of water molecules (Mano, Koniarova, & Reis, Citation2003). These typical saccharide bands were identified belonging to starch absorption characteristics.
Figure 3. IR spectrum of waxy maize starch before and after dry heat treatment with or without chitosan.
Figura 3. Espectro IR del almidón de maíz ceroso antes y después del tratamiento de calor seco con o sin chitosán.
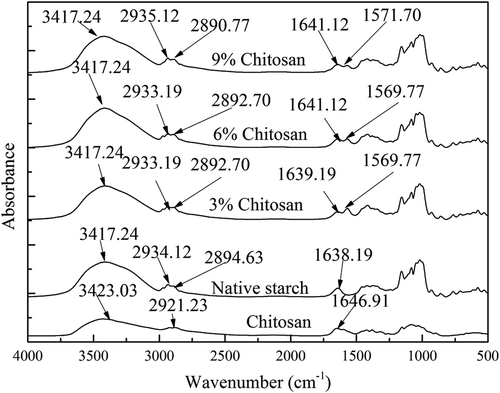
The interactions which occurred between starch and chitosan molecules can be confirmed via the analysis of the changes of their corresponding IR spectra following the treatment. As can be seen from , compared to the IR spectra of pure chitosan and starch, the chitosan modified starch at 6% addition showed a peak located at 1569.77 cm−1, which is attributed to amide–NH2 absorption. This result might suggest that interactions occur between the hydroxyl groups of starch and the amino groups of chitosan. Furthermore, the characteristic peak of the hydrogen bond in the modified starch remained at 3417.24 cm−1, which is different from that of pure chitosan, but is consistent with that of the native starch, indicating that the main ingredient in the modified starch determined the absorption characters. Meanwhile, the C–H stretching peaks of the native starch at 2934.12 cm−1 and 2894.63 cm−1 shifted toward lower wavenumbers of 2933.19 cm−1 and 2890.77 cm−1, respectively, after dry heat treatment (e.g. the control). The absorption located at 2921.23 cm−1 for the pure chitosan was shifted to a higher wavenumber of 2933.19 cm−1 following the dry heat treatment, which might indicate the formation of the inter- and intramolecular hydrogen bonding as well between chitosan and starch during dry heat treatment (Xu, Kim, Hanna, & Nag, Citation2005). This study found that the interactions between two polymers led to the band at 1638.19 cm−1 being split into two peaks, and more importantly, the absorption ratio of the two peaks was highly associated with the chitosan concentrations used in the system, which might further suggest that the interactions between the two polymers occurred during the treatment and were further enhanced following the addition of chitosan from 3% to 9%.
3.4. Pasting properties by RVA
The pasting characteristics of waxy maize starch and the chitosan modified waxy maize starches were measured and are shown in (RVA profile of pure chitosan was not present in due to its insolubility during the pasting measurement). The control had an increased peak viscosity (PV) compared to the native starch, although this difference was not significant (p > 0.05). This indicated that the sample preparation procedure changed starch structure, leading to the starch granules being more readily hydrated than the native form. Meanwhile, a significant reduction in the breakdown (BD) value as a result of poor shear resistance was also noticed in the control, which further confirmed the changes in the starch granule structure due to heat treatment. Moreover, a higher final viscosity (FV) measured in the control suggested that more starch molecules leached out of the starch granules during gelatinization and were involved in the retrogradation process, which subsequently contributed to a greater FV than the native starch. This study suggests that dry heat treatment could break intra- or intermolecular hydrogen bonds of starches, which would facilitate the swelling of starch granules and result in disruption of starch granules. However, other studies have reported that dry heat treatment without gum scan significantly reduce the peak and final viscosities of the waxy rice starch (Li et al., Citation2013). It has been proposed that, in addition to the disruption of hydrogen bonds by dry heating, the excessive heat could cleave the glycosidic linkages, contributing to a reduction in viscosity. These varying results may be due to the different sample preparation conditions used in the current and previous studies.
Figure 4. Changes in pasting properties of starch after chitosan modification.
Figura 4. Cambios en las propiedades pastosas del almidón después de la modificación con chitosán.
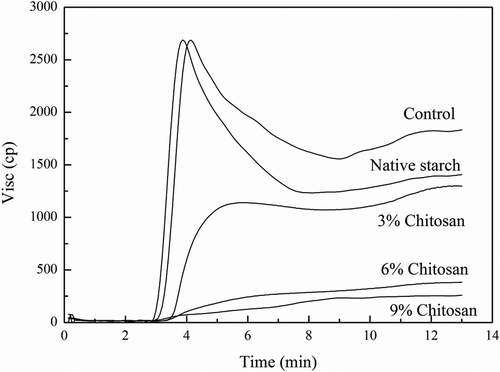
This is not surprising given the complexity of changes seen with the addition of chitosan in pasting curves associated with structural modifications in the sample. The most striking feature of the RVA graphic data was the rapid decrease in paste PV when the ionic gum interacted with starch granules (). The RVA curves of all the chitosan modified starches were characterized by the disappearance of the trough and significant reduced viscosity at all points. Thus, the swelling of the starch granules were restricted and the disruption of the granules was highly limited even at a temperature of 95°C. Furthermore, the present data also indicates that the PV (and hydration power) of the modified starch initially decreased following the addition of chitosan from 3% to 9%. The shear-stabilization of the starch granules was also enhanced by the dry heat treatment with chitosan, which was similar to the modified starches obtained through chemical cross-linkages (Mathew et al., Citation2006). Previous studies have also suggested that ester bonds can be formed when the mixtures of starch and ionic gums were dry heated (Lim et al., Citation2003, Citation2002), and that starch structure becomes progressively organized following these interactions.
3.5. Starch digestion properties
The digestion property of each starch sample can be characterized by the release of glucose at different digestion intervals and their corresponding digestion curves are shown in . The digestion process of each starch sample was divided into two stages: a rapid hydrolysis and a slower but more stable glucose releasing process, which was consistent with previous studies (Singh, Dartois, & Kaur, Citation2010). The plausible interpretation for this phenomenon is a fast removal of less organized areas in starch, which is then followed by a slow erosion of highly structured regions of the starch granules. The control showed the highest digestion rate among all the samples, indicating that the sample preparation procedure influenced starch structure, making it more susceptible to the enzyme hydrolysis. The enhanced digestibility of the control was highly consistent with its physical properties as revealed by its pasting characteristics in this study.
Figure 5. The digestion curve of starch before and after chitosan modification.
Figura 5. Curva digestiva del almidón antes y después de la modificación con chitosán.
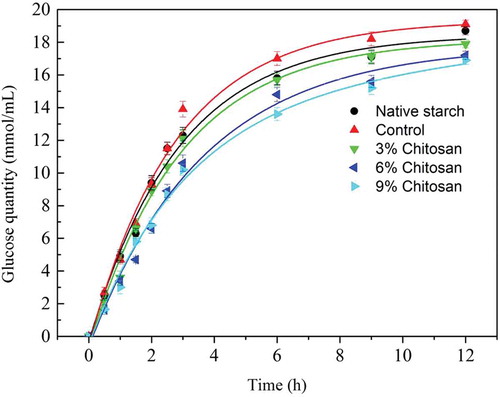
Although there was a slight reduction in the digestibility of the modified starch with 3% chitosan compared to the control, a further reduction of starch digestibility was achieved when the chitosan addition was increased to 6% (p < 0.001) (). This reduction might be attributed to a more tightly structured starch due to the interactions with chitosan molecules, which was confirmed by the IR results. The molecular interactions may also strengthen the starch granule structure, leading to a limited granule swelling and less starch molecules leaching during gelatinization compared to the control. Although it has been reported that the addition of some gums (e.g. xanthan) can limit starch gelatinization, to the best of our knowledge, this is the first demonstration of the effect of ionic gum modification on starch digestion behavior. Further understanding of the interaction mechanisms between starch and other biomacromolecules will contribute to further development of value-added products.
The digestion characteristics of each starch sample were further investigated using a LOS analysis method. It is interesting to note that the digestion of control and native starch granules showed one-phase kinetics (). From the digestion curve, the control had a higher value of the slope of the LOS plots (0.18132) than its native form (0.17391), indicating the control had a greater digestion rate compared to the native at all the digestion points. The difference in the digestion characteristics between the two starch samples seems to be highly related to their physicochemical properties. In contrast, all of the chitosan modified starches demonstrated two-phase digestion kinetics, indicating the modification significantly changed starch digestion behavior. The intersection between the two linear phases of the LOS plot indicated that a slower phase became the predominant reaction (Edwards, Warren, Milligan, Butterworth, & Ellis, Citation2014), following 5–7 h amylolysis (). More importantly, the total extent of amylolysis (Glu∞, the sum of y-intercepts) (Edwards et al., Citation2014) was found to be reduced by increasing chitosan addition, implying a positive effect of chitosan addition on suppressing starch BD.
Figure 6. The LOS analysis of the starch samples following the digestion process.
Figura 6. Análisis LOS de las muestras de almidón después del proceso de digestión.
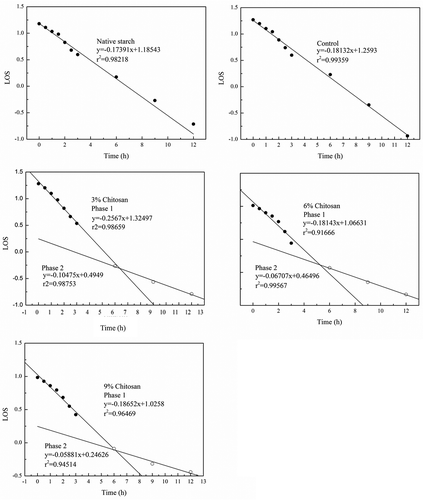
Among the three modified starches, the absolute value of the slope of the LOS plots decreased following increased chitosan addition from 3% to 6%, and this trend further supported the potential relationship between starch structure and its digestion behavior. Moreover, for starch digestion, the value of the slope at phase 2 was reduced with increased chitosan addition. Whilst further studies on the effect of the aforementioned processes on enzyme access to facilitate starch digestion will be needed, the LOS analysis is a useful method for evaluating starch digestion kinetics, and the results from this study suggest that chitosan modification shows potential for increasing the amount of SDS and RS in the starch via molecular modification.
4. Conclusion
In this study, the effect of chitosan addition on the physicochemical and digestion properties of starch was investigated. As shown by the changes in the FTIR spectrum following the dry heat treatment, the interaction between starch and chitosan molecules occurred primarily through cross-links. The interactions between the two ingredients enlarged the particle size to more than six times that of the control. Pasting properties were shown to be another sensitive parameter for evaluating the interactions between starch and chitosan and the effect of these interactions on starch gelatinization properties. Following these interactions, starch granule swelling was greatly inhibited as demonstrated by the RVA study. The chitosan modified starches showed a significant reduction in pasting viscosity at all points, which may be due to starch granules being greatly strengthened through the potential interactions. More importantly, these interactions also showed a significant effect on starch digestion properties in that the digestion rate and the final glucose concentrations of the amylase-driven hydrolysis reactions were reduced with increased chitosan addition. Meanwhile, the digestion kinetics also changed from one phase to two phases following chitosan addition. These changes in the digestion pattern may also imply that the cross-linking areas on the surface of starch granules prohibit digestive enzyme access. Finally, this study suggests that chitosan modification provides an effective method for developing novel products based on glucose-moderated release.
Disclosure statement
No potential conflict of interest was reported by the authors.
Acknowledgements
This work was financially supported by Agricultural Science and Technology Achievements Transformation Fund (2014GB2A100527), Tianjin Research Program of Application Foundation and Advanced Technology (15JCZDJC34300), the China-European research collaboration program (SQ2013ZOA100001), and the Nature Science Foundation of China (31471701, U1501214).
Additional information
Funding
References
- Bangyekan, C., Aht-Ong, D., & Srikulkit, K. (2006). Preparation and properties evaluation of chitosan-coated cassava starch films. Carbohydrate Polymers, 63(1), 61–71. doi:10.1016/j.carbpol.2005.07.032
- Chiu, C.W., Schiermeyer, E., Thomas, D.J., & Shah, M.B. (1998). U.S. Patent No. 5,725,676. Washington, DC: U.S. Patent and Trademark Office.
- Chiu, C.W., Schiermeyer, E., Thomas, D.J., Shah, M.B., Hanchett, D.J., & Jeffcoat, R. (1999). U.S. Patent No. 5,932,017. Washington, DC: U.S. Patent and Trademark Office.
- Chung, H.J., Min, D., Kim, J.Y., & Lim, S.T. (2007). Effect of minor addition of xanthan on cross‐linking of rice starches by dry heating with phosphate salts. Journal of Applied Polymer Science, 105(4), 2280–2286. doi:10.1002/app.26237
- Chung, H.-J., Shin, D.-H., & Lim, S.-T. (2008). In vitro starch digestibility and estimated glycemic index of chemically modified corn starches. Food Research International, 41(6), 579–585. doi:10.1016/j.foodres.2008.04.006
- De Vasconcelos, C.L., Bezerril, P.M., Dos Santos, D.E.S., Dantas, T.N.C., Pereira, M.R., & Fonseca, J.L.C. (2006). Effect of molecular weight and ionic strength on the formation of polyelectrolyte complexes based on poly (methacrylic acid) and chitosan. Biomacromolecules, 7(4), 1245–1252. doi:10.1021/bm050963w
- Edwards, C.H., Warren, F.J., Milligan, P.J., Butterworth, P.J., & Ellis, P.R. (2014). A novel method for classifying starch digestion by modelling the amylolysis of plant foods using first-order enzyme kinetic principles. Food & Function, 5, 2751–2758. doi:10.1039/C4FO00115J
- Englyst, H.N., Kingman, S.M., & Cummings, J.H. (1992). Classification and measurement of nutritionally important starch fractions. European Journal of Clinical Nutrition, 46, S33–50.
- Freitas, R.A., Gorin, P.A.J., Neves, J., & Sierakowski, M.-R. (2003). A rheological description of mixtures of a galactoxyloglucan with high amylose and waxy corn starches. Carbohydrate Polymers, 51(1), 25–32. doi:10.1016/S0144-8617(02)00095-4
- Htoon, A., Shrestha, A.K., Flanagan, B.M., Lopez-Rubio, A., Bird, A.R., Gilbert, E.P., & Gidley, M.J. (2009). Effects of processing high amylose maize starches under controlled conditions on structural organisation and amylase digestibility. Carbohydrate Polymers, 75(2), 236–245. doi:10.1016/j.carbpol.2008.06.016
- Jiugao, Y., Ning, W., & Xiaofei, M. (2005). The effects of citric acid on the properties of thermoplastic starch plasticized by glycerol. Starch-Stärke, 57(10), 494–504. doi:10.1002/(ISSN)1521-379X
- Lafiandra, D., Riccardi, G., & Shewry, P.R. (2014). Improving cereal grain carbohydrates for diet and health. Journal of Cereal Science, 59(3), 312–326. doi:10.1016/j.jcs.2014.01.001
- Li, Y., Shoemaker, C.F., Ma, J., Shen, X., & Zhong, F. (2008). Paste viscosity of rice starches of different amylose content and carboxymethylcellulose formed by dry heating and the physical properties of their films. Food Chemistry, 109(3), 616–623. doi:10.1016/j.foodchem.2008.01.023
- Li, Y., Zhang, H., Shoemaker, C.F., Xu, Z., Zhu, S., & Zhong, F. (2013). Effect of dry heat treatment with xanthan on waxy rice starch. Carbohydrate Polymers, 92(2), 1647–1652. doi:10.1016/j.carbpol.2012.11.002
- Lim, H.S., BeMiller, J.N., & Lim, S.-T. (2003). Effect of dry heating with ionic gums at controlled pH on starch paste viscosity. Cereal Chemistry, 80(2), 198. doi:10.1094/CCHEM.2003.80.2.198
- Lim, S.-T., Han, J.-A., Lim, H.S., & BeMiller, J.N. (2002). Modification of starch by dry heating with ionic gums. Cereal Chemistry, 79(5), 601–606. doi:10.1094/CCHEM.2002.79.5.601
- Liu, H., Adhikari, R., Guo, Q., & Adhikari, B. (2013). Preparation and characterization of glycerol plasticized (high-amylose) starch-chitosan films. Journal of Food Engineering, 116(2), 588–597. doi:10.1016/j.jfoodeng.2012.12.037
- Mano, J.F., Koniarova, D., & Reis, R.L. (2003). Thermal properties of thermoplastic starch/synthetic polymer blends with potential biomedical applicability. Journal of Materials Science: Materials in Medicine, 14(2), 127–135.
- Mathew, S., Brahmakumar, M., & Abraham, T.E. (2006). Microstructural imaging and characterization of the mechanical, chemical, thermal, and swelling properties of starch–chitosan blend films. Biopolymers, 82(2), 176–187. doi:10.1002/(ISSN)1097-0282
- Muscat, D., Adhikari, B., Adhikari, R., & Chaudhary, D.S. (2012). Comparative study of film forming behaviour of low and high amylose starches using glycerol and xylitol as plasticizers. Journal of Food Engineering, 109(2), 189–201. doi:10.1016/j.jfoodeng.2011.10.019
- Poulsen, B.R., Ruiter, G., Visser, J., & Iversen, J.J.L. (2003). Determination of first order rate constants by natural logarithm of the slope plot exemplified by analysis of Aspergillus niger in batch culture. Biotechnology Letters, 25(7), 565–571. doi:10.1023/A:1022836815439
- Rosell, C.M., Yokoyama, W., & Shoemaker, C. (2011). Rheology of different hydrocolloids–rice starch blends. Effect of successive heating–cooling cycles. Carbohydrate Polymers, 84(1), 373–382. doi:10.1016/j.carbpol.2010.11.047
- Sajilata, M.G., Singhal, R.S., & Kulkarni, P.R. (2006). Resistant starch – A review. Comprehensive Reviews in Food Science and Food Safety, 5(1), 1–17. doi:10.1111/crfs.2006.5.issue-1
- Sandhu, K.S., Kaur, M., Singh, N., & Lim, S.-T. (2008). A comparison of native and oxidized normal and waxy corn starches: Physicochemical, thermal, morphological and pasting properties. LWT-Food Science and Technology, 41(6), 1000–1010. doi:10.1016/j.lwt.2007.07.012
- Sasaki, T., & Kohyama, K. (2012). Influence of non-starch polysaccharides on the in vitro digestibility and viscosity of starch suspensions. Food Chemistry, 133(4), 1420–1426. doi:10.1016/j.foodchem.2012.02.029
- Sikora, M., Kowalski, S., & Tomasik, P. (2008). Binary hydrocolloids from starches and xanthan gum. Food Hydrocolloids, 22(5), 943–952. doi:10.1016/j.foodhyd.2007.05.007
- Singh, J., Dartois, A., & Kaur, L. (2010). Starch digestibility in food matrix: A review. Trends in Food Science & Technology, 21(4), 168–180. doi:10.1016/j.tifs.2009.12.001
- Tischer, P.C.S.F., Noseda, M.D., De Freitas, R.A., Sierakowski, M.R., & Duarte, M.E.R. (2006). Effects of iota-carrageenan on the rheological properties of starches. Carbohydrate Polymers, 65(1), 49–57. doi:10.1016/j.carbpol.2005.12.027
- Wan, Y., Wu, H., Yu, A., & Wen, D. (2006). Biodegradable polylactide/chitosan blend membranes. Biomacromolecules, 7(4), 1362–1372. doi:10.1021/bm0600825
- Xu, Y.X., Kim, K.M., Hanna, M.A., & Nag, D. (2005). Chitosan–starch composite film: Preparation and characterization. Industrial Crops and Products, 21(2), 185–192. doi:10.1016/j.indcrop.2004.03.002
- Zhang, G., & Hamaker, B.R. (2009). Slowly digestible starch: Concept, mechanism, and proposed extended glycemic index. Critical Reviews in Food Science and Nutrition, 49(10), 852–867. doi:10.1080/10408390903372466
- Zhou, Z., Zheng, P., Wang, Z., Yang, R., & Chu, Y. (2015). Enhanced thermal and antibacterial properties of cross‐linked waxy maize starch granules by chitosan via dry heat treatment. International Journal of Food Science & Technology, 50(4), 899–905. doi:10.1111/ijfs.2015.50.issue-4
- Zivanovic, S., Li, J., Davidson, P.M., & Kit, K. (2007). Physical, mechanical, and antibacterial properties of chitosan/PEO blend films. Biomacromolecules, 8(5), 1505–1510. doi:10.1021/bm061140p