ABSTRACT
This study investigated the changes of DNA and milk quality during storage at temperatures of 2°C, 10°C and 20°C. Milk quality was assessed by measuring the milk protein, milk fat, lactose, pH and acidity. The quality of DNA extracted from milk was evaluated by monitoring DNA content, purity, and degradation as well as by amplifying target sequences from mitochondrial and nuclear genes. In addition, nonlinear regression models were used to analyze the correlations between DNA content and milk quality. As expected, the deterioration was observed in both DNA and milk quality during storage. Mathematical models showed the high correlations (R2 > 0.9) between DNA content and milk quality. These established mathematical models hold the potential to evaluate the milk quality and may be applied practically in the process design and quality control of dairy products in the future.
RESUMEN
El presente estudio investigó los cambios que se producen en el ADN y la calidad de la leche durante su almacenamiento a temperaturas de 2°C, 10°C y 20°C. Al respecto, la calidad de la leche fue valorada a partir de la medición de la proteína láctea, la grasa láctea, la lactosa, el contenido de ADN, su pureza y su degradación, y de la amplificación de las secuencias objetivo de los genes mitocondriales y nucleares. Asimismo, se utilizaron modelos de regresión no lineales para analizar las correlaciones entre el contenido de ADN y la calidad de la leche. Como se esperaba, durante el almacenamiento se produjo el deterioro tanto del ADN como de la calidad de la leche. Los modelos matemáticos dieron cuenta de elevadas correlaciones (R2>0.9) entre el contenido de ADN y la calidad de la leche. Estos modelos matemáticos establecidos posibilitan evaluar la calidad de la leche y en el futuro podrán ser aplicados de manera práctica a la hora de diseñar su procesamiento y realizar el control de calidad de productos lácteos.
KEYWORDS:
1. Introduction
Milk is a good source of protein, lipids, lactose, minerals and vitamins (Haug, Høstmark, & Harstad, Citation2007; Singh, De, & Belkheir, Citation2013). However, raw milk will easily deteriorate during storage because of enzymatic and microbial activity. Therefore, the economic value of milk can be reduced by the proteolysis and lipolysis, which negatively affected its performance. In particular, the cheese yield can be reduced by the hydrolysis of casein (Barbano, Rasmussen, & Lynch, Citation1991; Klei et al., Citation1998). Lipolysis is the process when the transfer of lipids to free fatty acids (FFA) happens, when an increased FFA in milk can result in undesirable flavors and altered functionality (Deeth, Citation2006; Ma et al., Citation2000). The FFA in milk has been shown to increase during storage (Wiking, Frøst, Larsen, & Nielsen, Citation2002). Muir, Kelly, and Phillips (Citation1978) observed a temperature-dependent increase trend in FFA after the storage of milk. So these quality changes during milk storage should be controlled to reduce economic losses.
Quality control of the raw milk in dairy production has attracted extensive attention worldwide (Auldist et al., Citation1996; Goff & Griffiths, Citation2006). Identification of adverse factors contributing to the spoilage of milk during storage is needed. The refrigerated storage of raw milk is widely applied throughout the dairy industrial chain. The milk quality changes during storage since it undergoes numerous physical, chemical and biological processes (Cardoso et al., Citation2014). Prolonged storage time and improper storage temperature can induce adverse alterations in the milk components (Cousin, Citation1982). Driven by the increasing consumption of milk, monitoring the changes of milk quality will be a crucial step for the industry goal of producing consistent and high-quality milk products.
Recent technological advances in chemical and instrumental analysis and in mid-infrared spectroscopy (MIRS) methodologies in particular are being applied to measurement of dairy products quality. Compared to biochemical analysis, traditional sensory, chemical and instrumental methods used to assess milk quality are limited in analyzing large-scale samples (Guy & Fenaille, Citation2006; Korel & Balaban, Citation2002; Larsen, Kidmose, Kristensen, Beaumont, & Mortensen, Citation2013; Mariaca & Bosset, Citation1997; Marsili, Citation2000). In recent years, molecular techniques have been one of the powerful tools in quality inspection of dairy products and in research of molecular nutrition (Liu, Gao, Yang, Ku, & Zan, Citation2014). Whether this method can be utilized to monitor the milk quality remains unknown. The DNA obtained from the milk is mainly extracted from somatic cells, which could also be influenced by heating process and storage. So the DNA content may decline since the milk somatic cells may undergo apoptosis during storage (Sedinova, Urbanova, Skarda, & Jukl, Citation1985; Urbanova, Sedinova, Skarda, Skardová, & Jukl, Citation1985). Therefore, the changes of DNA content may reflect the milk quality during storage. This study aimed to establish mathematical models to analyze the correlations between the milk quality and DNA content, to offer a new way for milk quality evaluation.
2. Materials and methods
2.1. Materials
Authentic samples of pooled raw milk from cow were obtained from the collection tank of local dairy farm in Xi’an, Shaanxi province of China. Samples were transported on ice packs (to maintain an optimum low temperature) to the laboratory. A total of 242 milk samples were prepared and stored at the three temperatures (2°C, 10°C and 20°C) for a specified number of days before analysis.
2.2. Chemicals
Proteinase K, Sodium dodecyl sulfonate (SDS) and Tris-base were purchased from Sigma-Aldrich. In addition, the molecular biology reagents were obtained from Labtech International Co., Ltd. (Uckfield, UK). All other reagents used in the experiment were of analytical grade and purchased from Jingbo Chemical Reagent Co., Ltd. (Xi’an, China).
2.3. Analysis of milk quality
The milk fat, protein and lactose were measured by milk composition analyzer (Lactoscan LA, MCC, Bulgaria) according to the manufacturer’s standard protocols. The pH value of the milk samples was measured using a pH meter (Yidian Co., PHS-3C, China). The titratable acidity was determined by the standard method of the International Dairy Federation (IDF, Citation1981). Milk samples stored at 2°C were submitted for analyzing milk composition, titratable acidity and pH during 15 days of storage. The composition of milk stored at 10°C and 20°C was only analyzed within three and two storage days because the heavy deterioration was observed in milk stored at 10°C and 20°C for over three and two storage days, which suggested that the milk was no longer suitable for composition analysis through milk composition analyzer. The titratable acidity and pH could be measured during 10 and 7 storage days for 10°C and 20°C, respectively.
2.4. DNA extraction and quality evaluation
2.4.1. DNA extraction
The DNA was extracted from 10-mL milk according to the method described in our previous work (Liu et al., Citation2014). The method of DNA extraction could avoid a stress response in the sampled animals affecting milk production compared with traditional extraction methods, which obtained DNA from blood and organ tissues (Buitkamp & Gotz, Citation2004). Milk stored at 2°C, 10°C and 20°C could not be sampled for DNA extraction within 15, 10 and 7 storage days, respectively.
2.4.2. Spectrophotometer measurements
The DNA content (expressed as ng/μL) was calculated using UV spectrophotometer (Bio-Rad, SmartSpec 3000, USA), and the absorbance ratio at 260 and 280 nm (A260/A280) was taken as a purity index.
2.4.3. Agarose gel electrophoresis
The integrity (level of degradation) of total DNA was examined by agarose gel electrophoresis. Specifically, 4 µL of each DNA extract was mixed with 1 µL of 6 × loading dye and analyzed in a 1.0% (w/v) agarose gel electrophoresis at 100 V for 40 min. After electrophoresis, the gel was visualized under UV light (Siemon, BIO-BEST200E, USA) and digitally photographed.
2.4.4. PCR amplification
To further investigate the integrity of mitochondrial DNA (mtDNA) and nuclear (nDNA) extracted from milk, two pairs of primers were designed to amplify the bovine Cytb and B2M gene, respectively. The fragment length for Cytb is 271 bp: 5ʹ-GCC ATA TAC TCT CCT TGG TGA CA-3ʹ and 5ʹ-GTA GGC TTG GGA ATA GTA CGA-3ʹ. The fragment length for B2M is 729 bp: 5ʹ-GGC TTT CCC AGC ATC ACT AAC-3ʹ and 5ʹ-TCA CAG CAC CAC CAA ACT TAT CT-3ʹ. The PCR was performed using a PCR system (Thermo Fisher Scientific Inc., Aritik, USA) in a 10 μL reaction mixture containing 1 μL of genomic DNA template, 10 × PCR buffer, dNTP Mixture (2.5 mM), each primer (20 µM) and Taq DNA polymerase (5 U/μL). The PCR protocol of Cytb (B2M) was 95°C for 5 min followed by 94°C denaturation for 30 s, 60°C (62°C) annealing for 30 s, and 30 cycles of 72°C for 30 s, and a final extension at 72°C for 10 min. The PCR products were also examined through 1.0% (w/v) agarose gel electrophoresis and visualized using UV light according to the method as described above.
2.5. Statistical analysis
The analysis of variance (ANOVA) was used to detect differences between data mean values (SPSS Version 14.0, SPSS Inc.). The mathematical models were used for determining the correlation between the milk quality indices and DNA content with nonlinear regression model of SPSS software. A goodness-of-fit test for each model was ascertained considering coefficient of determination (R2).
3. Results
3.1. Changes in milk quality during storage
The milk composition (fat, protein and lactose) and freshness indices (pH and acidity) were investigated during milk storage at three temperatures (2°C, 10°C and 20°C) in the current study. These parameters we measured are commonly used within the dairy industry to predict the suitability of milk for processing.
When milk was stored at 2°C, 10°C and 20°C, the mean milk fat level increased from 4.04% to 4.12% between day 0 and day 15 ()), from 4.04% to 4.65% between day 0 and day 3 ()), and from 4.04% to 4.57% between day 0 and day 2 ()), respectively.
Figure 1. Changes in milk fat (a-c), milk protein (d-f) and lactose (g-i) of milk during storage at 2°C (a, d and g), 10°C (b, e and h) and 20°C (c, f and i), respectively.
Figura 1. Cambios en la grasa láctea (a-c), proteína láctea (d-f) y lactosa (g-i) de la leche durante su almacenamiento a temperaturas de 2°C (a, d y g), 10°C (b, e y h) y 20°C (c, f y i), respectivamente.
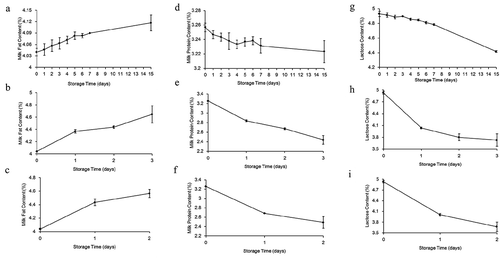
The milk protein level was decreased from 3.26% to 3.22% between day 0 and day 15 ()), from 3.26% to 2.44% between day 0 and day 3 ()), and from 3.26% to 2.49% between day 0 and day 2 ()) when milk was stored at 2°C, 10°C and 20°C, respectively.
The lactose level was also decreased from 4.93% to 4.42% between day 0 and day 15 ()), from 4.93% to 3.73% between day 0 and day 3 ()), and from 4.93% to 3.67% between day 0 and day 2 ()) when milk was stored at 2°C, 10°C and 20°C, respectively.
Because the pH and acidity could be measured even when milk was stored for a long time at three temperatures, the data from three temperatures were compared at the same storage time. Results showed a temperature-dependent decrease in pH and a temperature-dependent increase in acidity of milk during storage, with lower pH and higher acidity linked to milk stored at 20°C and 10°C compared with 2°C ().
3.2. Changes in DNA quality of milk during storage
DNA content and purity were detected by UV spectrophotometry, and results were shown in . DNA content was decreased from 49.57 ng/μL to 21.00 ng/μL between 0 day and 15 day ()), from 49.57 ng/μL to 10.24 ng/μL between 0 day and 7 day ()), and from 49.57 ng/μL to 1.67 ng/μL between 0 day and 7 day ()) when milk was stored at 2°C, 10°C and 20°C, respectively. It is generally accepted that a DNA sample is pure when its A260/A280 ratio reaches 1.8. In this study, all A260/A280 ratios from milk samples stored at 2°C, 10°C and 20°C almost reached 1.8 during storage ().
Figure 2. Changes in acidity (a) and pH (b) of milk during storage at 2°C, 10°C and 20°C, respectively.
Figura 2. Cambios en la acidez (a) y el pH (b) de la leche durante su almacenamiento a temperaturas de 2°C, 10°C y 20°C, respectivamente.
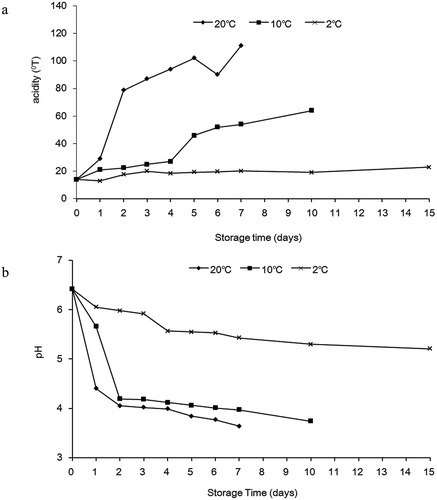
Figure 3. Changes in the DNA content (a-c) and purity (d-f) obtained from milk during storage at 2°C (a and d), 10°C (b and e) and 20°C (c and f), respectively.
Figura 3. Cambios en el contenido de ADN (a-c) y su pureza (d-f) obtenido de la leche durante su almacenamiento a temperaturas de 2°C (a y d), 10°C (b y e) y 20°C (c y f), respectivamente.
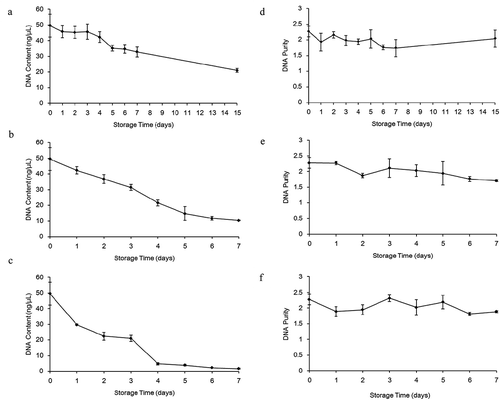
Agarose gel electrophoresis was applied to evaluate the integrity of DNA we obtained, and the results were shown in . All samples yielded the positive results, which suggested that the DNA was successfully extracted from milk. However, significant differences in DNA degradation were observed between different temperatures. In detail, when milk was stored at 2°C, DNA was quite intact between 0 day and 7 day, but it became partially degraded when milk was stored for 15 days ()). When milk was stored at 10°C, all DNA was intact between 0 day and 7 day ()). When milk was stored at 20°C, DNA was sharply degraded even milk was stored for 1 day ()), which indicated a temperature-dependent degradation in DNA integrity.
Figure 4. Changes in the integrity of DNA obtained from milk during storage at 2°C (a), 10°C (b) and 20°C (c), respectively. M represented DL2000 marker, the numbers represented the storage day of milk samples.
Figura 4. Cambios en la integridad del ADN obtenido de la leche durante su almacenamiento a temperaturas de 2°C (a), 10°C (b) y 20°C (c), respectivamente. M representa el marcador DL2000 y los números representan el día del almacenamiento de las muestras de leche.
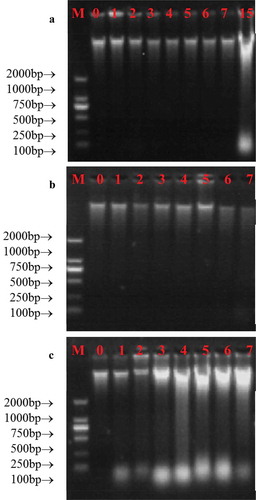
The PCR amplification results for both mtDNA and nDNA were shown in . Results showed that both mtDNA and nDNA fragments could be amplified for all samples, which suggested the quality of DNA extracted from stored milk was acceptable. The storage condition we used here could not influence the integrity of both mtDNA and nDNA. In addition, these results further confirmed that the bovine DNA was successfully extracted from raw milk.
Figure 5. Changes in the integrity of mtDNA (a-c) and nDNA (d-f) obtained from milk during storage at 2°C (a and d), 10°C (b and e) and 20°C (c and f). M represented DL2000 marker, and the numbers represented the storage day of milk samples.
Figura 5. Cambios en la integridad del ADNmt (ADN mitocondrial) (a-c) y el ADNn (ADN nuclear) (d-f) obtenidos de la leche durante su almacenamiento a temperaturas de 2°C (a y d), 10°C (b y e) y 20°C (c y f). M representa el marcador DL2000 y los números representan el día del almacenamiento de las muestras de leche.
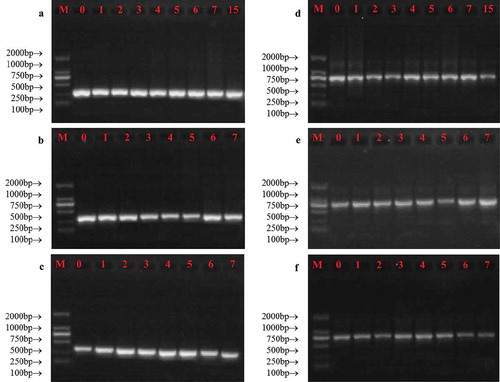
3.3. The correlation between DNA content and milk quality
Because the storage temperature for raw milk usually ranged from 2°C to 6°C, the correlations between DNA content and milk quality indices were only analyzed at 2°C, and the results were shown in . These models were proposed to analyze the correlation between DNA content and above milk quality indices at 2°C by polynomial fitting. The models had good fit with high correlation coefficients for milk fat (R2 = 0.9921), milk protein (R2 = 0.9635), lactose (R2 = 0.9813), pH (R2 = 0.9541) and acidity (R2 = 0.9065) with DNA content. In addition, DNA content was negatively correlated with both milk fat content and acidity, whereas it was positively correlated with milk protein content, lactose content, and pH value.
4. Discussion
This study demonstrated that the storage time affected both physicochemical properties and DNA quality of milk. As we all know that physicochemical changes occur in milk during the storage as the result of the growth of bacteria and the interactions among these macromolecular substances (Frederiksen et al., Citation2011; Ikonen, Morri, Tyrisevä, Ruottinen, & Ojala, Citation2004; Ku et al., Citation2016). The decreased protein and lactose level could be attributed to proteolysis and glycolysis, which were caused by microbes and enzymes in milk. Milk soured during storage because the fermentation of lactose, proteolysis and lipolysis increased the lactic acid, amino acid and fatty acid. Although the lipolysis can transfer lipids into free fatty acids, which may result in a decrease in fat content of milk, the results showed an increasing trend in fat content in the current study. This difference may be explained by the fact that milk fat globule membrane (MFGM) permeability was also influenced during storage, which could produce a larger free fat content.
The various mechanisms that contribute to DNA degradation have attracted considerable attention in recent years (Ballari & Martin, Citation2013). Food processing and storage could cause DNA degradation. In the present study, the DNA presented in milk was also degraded after storage under all conditions, which reflected in the decrease of DNA content and the electrophoretic results. These observations are in agreement with Spaniolas, Bazakos, Bihmidine, Georgousakis, and Kalaitzis (Citation2008) who found that the DNA in olive oil degraded after storage. This result was similar with Hara, Nakanishi, Yoneyama, Saito, and Takada (Citation2016), who suggested that bloodstain and blood should be stored at a relative low temperature to prevent DNA degradation during long-term storage. These studies described above have proved that DNA presented in food gradually degraded during storage, but the relations between DNA content and storage time have not been studied. The newly presented exponential functions were able to explain the process of DNA degradation.
DNA is more stable in slight alkali environment, and so the degradation of DNA mainly attributed to the acidity continued to rise during storage of milk, as a consequence of the fermentation of lactose to lactic acid. This result was consistent with some previous studies. For example, Bauer, Weller, Hammes, and Hertel (Citation2003) concluded that the pH was the major effective parameter to cause DNA degradation in food. Weiss, Ros-Chumillas, Peña, and Egea-Cortines (Citation2007) observed that the plasmid DNA degraded in the acidic orange juice matrix, and this result further confirmed that the pH could significantly influence the DNA in food matrix. Interestingly, our data did not show significant differences in PCR recovery of mtDNA and nDNA between fresh and stored milk. The recovery of mtDNA and nDNA is fundamental in an authentication process by PCR-based methods. The extra finding proved the storage conditions we adopted here would not influence the species identification for milk.
Although many methods (traditional sensory, chemical and instrumental method) have been used to assess milk quality (Guy & Fenaille, Citation2006; Korel & Balaban, Citation2002; Larsen et al., Citation2013; Mariaca & Bosset, Citation1997; Marsili, Citation2000), the DNA-based method has been not explored. This study introduced a DNA-based method to assess the milk quality, and the established polynomial models showed the great potential in monitoring milk quality changes by DNA-based method.
5. Conclusions
Few researchers have examined the effects of storage conditions on DNA quality in milk. In this study, significant changes in DNA integrity and content were observed during the storage of milk. Therefore, the deterioration of milk quality could be directly reflected by the DNA degradation. In addition, the established mathematical models with R2 > 0.9 provided the basis for evaluating milk quality during storage based on the content of DNA.
Disclosure statement
No potential conflict of interest was reported by the authors.
Additional information
Funding
References
- Auldist, M. J., Coats, S., Sutherland, B. J., Mayes, J. J., McDowell, G. H., & Rogers, G. L. (1996). Effects of somatic cell count and stage of lactation on raw milk composition and the yield and quality of Cheddar cheese. Journal of Dairy Reserach, 63, 269–280.
- Ballari, R. V., & Martin, A. (2013). Assessment of DNA degradation induced by thermal and UV radiation processing: Implications for quantification of genetically modified organisms. Food Chemistry, 141, 2130–2136.
- Barbano, D. M., Rasmussen, R. R., & Lynch, J. M. (1991). Influence of milk somatic cell count and milk age on cheese yield. Journal of Dairy Science, 74, 369–388.
- Bauer, T., Weller, P., Hammes, W. P., & Hertel, C. (2003). The effect of processing parameters on DNA degradation in food. European Food Research and Technology, 217, 338–343.
- Buitkamp, J., & Gotz, K. U. (2004). Use of milk samples from a milk evaluation program for the genotyping of cows. Archiv Fur Tierzucht, 47, 15–26.
- Cardoso, V. L., Lima, M. L. P., Nogueira, J. R., Carneiro, R. L. R. D., Sesana, R. C., Oliveira, E. J., & El Faro, L. (2014). Economic values for milk production and quality traits in south and southeast regions of Brazil. Revista Brasileira De Zootecnia, 43, 636–642.
- Cousin, M. A. (1982). Presence and activity of psychrotrophic microorganisms in milk and dairy products: A review. Journal of Food Protection, 45, 172–207.
- Deeth, H. C. (2006). Lipoprotein lipase and lipolysis in milk. International Dairy Journal, 16, 555–562.
- Frederiksen, P. D., Hammershøj, M., Bakman, M., Andersen, P. N., Andersen, J. B., Qvist, K. B., & Larsen, L. B. (2011). Variations in coagulation properties of cheese milk from three Danish dairy breeds as determined by a new free oscillation rheometry-based method. Dairy Science and Technology, 91, 309–321.
- Goff, H. D., & Griffiths, M. W. (2006). Major advances in fresh milk and milk products: Fluid milk products and frozen desserts. Journal of Dairy Science, 89, 1163–1173.
- Guy, P. A., & Fenaille, F. (2006). Contribution of mass spectrometry to assess quality of milk‐based products. Mass Spectrometry Reviews, 25, 290–326.
- Hara, M., Nakanishi, H., Yoneyama, K., Saito, K., & Takada, A. (2016). Effects of storage conditions on forensic examinations of blood samples and bloodstains stored for 20years. Legal Medicine, 18, 81–84.
- Haug, A., Høstmark, A. T., & Harstad, O. M. (2007). Bovine milk in human nutrition–A review. Lipids in Health and Disease, 6, 25.
- IDF 81. (1981). Dried milk-determination of titratable acidity (Routine method). Brussels, Belgium: International Standard.
- Ikonen, T., Morri, S., Tyrisevä, A. M., Ruottinen, O., & Ojala, M. (2004). Genetic and phenotypic correlations between milk coagulation properties, milk production traits, somatic cell count, casein content, and pH of milk. Journal of Dairy Science, 87, 458–467.
- Klei, L., Yun, J., Sapru, A., Lynch, J., Barbano, D., Sears, P., & Galton, D. (1998). Effects of milk somatic cell count on cottage cheese yield and quality. Journal of Dairy Science, 81, 1205–1213.
- Korel, F., & Balaban, M. Ö. (2002). Microbial and sensory assessment of milk with an electronic nose. Journal of Food Science, 67, 758–764.
- Ku, T., Liu, Y. F., Gao, T. L., & Zhao, L. H. (2016). Relationships between viscosity and the contents of macromolecular substances from milk with different storage styles. Food Science and Technology, 4, 49–56.
- Larsen, M. K., Kidmose, U., Kristensen, T., Beaumont, P., & Mortensen, G. (2013). Chemical composition and sensory quality of bovine milk as affected by type of forage and proportion of concentrate in the feed ration. Journal of the Science of Food and Agriculture, 93, 93–99.
- Liu, Y. F., Gao, J. L., Yang, Y. F., Ku, T., & Zan, L. S. (2014). Novel extraction method of genomic DNA suitable for long-fragment amplification from small amounts of milk. Journal of Dairy Science, 97, 6804–6809.
- Ma, Y., Ryan, C., Barbano, D. M., Galton, D. M., Rudan, M. A., & Boor, K. J. (2000). Effects of somatic cell count on quality and shelf-life of pasteurized fluid milk. Journal of Dairy Science, 83, 264–274.
- Mariaca, R., & Bosset, J. O. (1997). Instrumental analysis of volatile (flavour) compounds in milk and dairy products. Le Lait, 77, 13–40.
- Marsili, R. T. (2000). Shelf-life prediction of processed milk by solid-phase microextraction, mass spectrometry, and multivariate analysis. Journal of Agricultural and Food Chemistry, 48, 3470–3475.
- Muir, D. D., Kelly, M. E., & Phillips, J. D. (1978). The effect of storage temperature on bacterial growth and lipolysis in raw milk. International Journal of Dairy Technology, 31, 203–208.
- Sedinova, V., Urbanova, E., Skarda, J., & Jukl, A. (1985). Determination of the number of somatic cells in milk using the rapid diphenylamine DNA filter method. Veterinarni Medicina, 30, 467–475.
- Singh, R., De, S., & Belkheir, A. (2013). Avena sativa (Oat), a potential neutraceutical and therapeutic agent: An overview. Critical Reviews in Food Science and Nutrition, 53, 126–144.
- Spaniolas, S., Bazakos, C., Bihmidine, S., Georgousakis, A., & Kalaitzis, P. (2008). Use of lambda DNA as a marker to assess DNA stability in olive oil during storage. European Food Research and Technology, 227, 175–179.
- Urbanova, E., Sedinova, V., Skarda, J., Skardová, O., & Jukl, A. (1985). Use of the Synpor membrane filter for the separation and determination of the number of somatic cells in the milk of dairy cows using the indole DNA filtration method. Veterinarni Medicina, 30, 409–418.
- Weiss, J., Ros-Chumillas, M., Peña, L., & Egea-Cortines, M. (2007). Effect of storage and processing on plasmid, yeast and plant genomic DNA stability in juice from genetically modified oranges. Journal of Biotechnology, 128, 194–203.
- Wiking, L., Frøst, M. B., Larsen, L. B., & Nielsen, J. H. (2002). Effects of storage conditions on lipolysis, proteolysis and sensoryattributes in high quality raw milk. Milchwissenschaft, 57, 190–194.