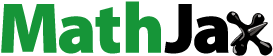
ABSTRACT
This study investigated the effect of different processing conditions on the properties of emulsions and final microcapsules in a pilot scale. A mixed protein system of sodium caseinate and pea protein isolate was used as wall material. The potential correlations amongst properties of emulsions and spray-dried powders, and the stability of nutrients were studied using coefficient correlation analysis. Increasing homogenization pressure or number of passes increased the retention of β-carotene significantly, while increasing the inlet air temperature was effective in preserving Vitamin A. Two-pass homogenization helped to achieve a smaller droplet size of liquid emulsions, which resulted in lower free-fat, higher nutrient retention, and better oxidative stability of powdered microcapsules. Increasing the inlet air temperature led to a higher tendency of lipid oxidation of spray-dried powders. A lower inlet temperature at 127°C increased the water activity and decreased the glass transition temperature of spray-dried powders, which consequently resulted in powder caking.
RESUMEN
El presente estudio investigó el efecto que conlleva utilizar diferentes condiciones de procesamiento en las propiedades de emulsiones y en las microcápsulas finales en una escala piloto. Con este objetivo se utilizó un sistema de proteínas mixtas de caseinato de sodio y aislado de proteína de guisante para el material de la pared. Empleando el análisis de la correlación de coeficientes se investigaron las correlaciones potenciales entre las propiedades de emulsiones y polvos secados por aspersión, así como la estabilidad de los nutrientes. Se constató que si se eleva la presión de homogeneización o el número de pases, aumenta de manera significativa la retención de β-caroteno; en cambio, el incremento de la temperatura del aire entrante resultó ser efectivo para conservar la vitamina A. La homogeneización de dos pases ayudó a producir una gota más pequeña de emulsiones líquidas, lo que resultó en menos grasa libre, una retención de nutrientes más elevada y mayor estabilidad oxidativa de las microcápsulas en polvo. Al elevarse la temperatura del aire entrante se registró una mayor tendencia a que se produjera la oxidación de lípidos de los polvos secados por aspersión. La menor temperatura del aire entrante a 127°C elevó la actividad del agua y disminuyó la temperatura de transición vítrea de los polvos secados por aspersión, lo que dio como resultado la formación de grumos de polvo.
1. Introduction
Microencapsulation is a complex process that involves several energy-intensive steps. The processing conditions during microencapsulation have profound effects on the physicochemical properties of emulsions and final microcapsules, including glass transition temperature (Tg), free-fat content, and the droplet size of emulsion (Bakry et al., Citation2016; Huang et al., Citation2014; Liu, Citation2010). These properties could greatly impact the encapsulation efficacy (EE) and the quality of products during storage (Bajaj, Tang, & Sablani, Citation2015; Botrel, de Barros Fernandes, Borges, & Yoshida, Citation2014; Silva, Azevedo, Cunha, Hubinger, & Meireles, Citation2016). For example, caking is closely related to the Tg and free-fat content of spray-dried powder. Microcapsules with lower Tg are more prone to caking than those with higher Tg (Aberkane, Roudaut, & Saurel, Citation2014). Also, excess free fat on the droplets could accelerate lipid oxidation and degradation of nutrients (Augustin, Sanguansri, & Bode, Citation2006). Therefore, it is important to control those critical physicochemical properties through the optimization of processing parameters to preserve the quality of powders during storage.
Microencapsulation involves two main steps – formation of emulsion and spray drying. An emulsion is prepared using high-pressure homogenization, which is a top-down fluid mechanical process that incorporates shearing and high-pressure treatment (Yu, Wang, Yao, & Liu, Citation2007). The ability of homogenization to produce homogeneous droplets depends on its specially designed valve that allows fluid to pass through under an ultra-high pressure. Therefore, the pressure generated at the valve is a vital processing parameter that can affect both physical and oxidative stability of the emulsion (Hebishy, Buffa, Juan, Blasco-Moreno, & Trujillo, Citation2017). Many studies have reported that a higher homogenization pressure is related to the formation of more stable emulsions with greater zeta-potential and smaller droplets in emulsion (Cano-Ruiz & Richter, Citation1997; Feng & Lee, Citation2016; Mukherjee, Chang, Zhang, & Mukherjee, Citation2017; Tcholakova, Denkov, & Lips, Citation2008). Due to droplet size reduction, the viscosity and firmness of the emulsion would increase with pressure, and the flow behavior will shift from Newtonian to shear thinning, or vice versa, depending on the fluid behavior of emulsion (Hebishy, Zamora, Buffa, Blasco-Moreno, & Trujillo, Citation2017). For oxidative stability, when stabilizers such as whey protein were used, a higher homogenization pressure could produce more stable emulsion due to the increase in protein concentration at the oil-and-water interface (Hebishy, Buffa, Guamis, Blasco-Moreno, & Trujillo, Citation2015). However, using ultra-high-pressure homogenization (UHPH) could also cause high temperature generated at the exit valve and lead to issues such as denaturation of globular proteins and flocculation of droplets (Floury, Desrumaux, & Legrand, Citation2002; Kim, Decker, & McClements, Citation2002; Xu, Mukherjee, & Chang, Citation2018) and accelerated lipid oxidation (Fernandez-Avila & Trujillo, Citation2016). Lastly, UHPH is an extensively energy-consuming process (Feng & Lee, Citation2017; Olenskyj, Feng, & Lee, Citation2017; Zhang & Wang, Citation2012), so it is important to find the optimal homogenization pressure so that emulsions with reasonable droplet sizes can be obtained without an excessive waste of energy.
Spray drying is a technique of dehydration that converts atomized liquid feed into powder (Usha & Pothakamury, Citation1995). Spray drying includes two periods – constant-rate drying and falling-rate drying. During the constant-rate drying period, the surface of droplets is saturated with water vapor. On the contrary, in the falling-rate drying period, the surface is no longer saturated and dry patches start to appear. In order to reduce surface energy, fats would migrate along with water vapor to the surface during the falling-rate period (Park & Drake, Citation2017). This migration of fats will eventually lead to fat residue at the surface of microcapsules, which is commonly termed as ‘surface fat’ or ‘free fat.’ Surface fat is directly exposed to air, so it is prone to lipid oxidation, which will eventually generate oxidative off-flavors. Therefore, it is important to reduce the free fat during spray drying (Sharma, Jana, & Chavan, Citation2012). One way to decrease free-fat content is to reduce the duration of the falling-rate period by lowering the inlet air temperature. It is well-established that higher inlet air temperatures usually increase the free-fat content and lower EE%, and also lead to higher hydroperoxide content of the product during storage (Ixtaina, Julio, Wagner, Nolasco, & Tomás, Citation2015; Ogrodowska, Tańska, & Brandt, Citation2017). However, this phenomenon is not absolute, some studies shown that free-fat content is sometimes reduced by increased inlet air temperature, depending on the wall materials and characteristics of the emulsions (Shamaei, Seiiedlou, Aghbashlo, Tsotsas, & Kharaghani, Citation2017). It is also reported that increased inlet air temperature could help obtain more intact and spherical particles without crack or fissure (Tolve, Condelli, Can, & Tchuenbou-Magaia, Citation2018; Wang, Duke, & Wang, Citation2017). Moreover, the inlet air temperature can impact other physical properties such as moisture content, color, and particle size, which affect the quality of powders (Santhalakshmy, Bosco, Francis, & Sabeena, Citation2015). Hence, it is crucial to find the optimal inlet air temperature to produce powders with desired physical properties.
In previous studies, the effects of processing conditions on the properties of emulsions have been elucidated. However, only limited correlations have been built between the processing conditions and stability of nutrients as well as other physicochemical properties of products during microencapsulation. Thus, this study selected a mixed protein system of sodium caseinate and pea protein isolate as wall material, and vitamin A palmitate, β-carotene, and docosahexaenoic acid (DHA) as core materials because these nutrients are prone to degradation or tend to oxidize during storage. This study aimed to investigate the effect of homogenization condition and spray drying inlet air temperature on the stability of selected nutrients and physicochemical properties of produced powders.
2. Materials and methodology
2.1. Materials
Sodium caseinate (NaCN) was obtained from Fonterra Co. Group. Ltd (New Zealand). Pea protein isolate (PPI, yellow pea) was from Cosucra (Belgium). The protein contents were tested using Kjeldahl method (N x 6.25). The protein contents of NaCN and PPI were 90.2% and 83.3%, respectively. Corn syrup solid was supplied by Roquette (France). Soybean oil was purchased from Fuji (Singapore). Vitamin A palmitate, β-carotene, and DHA were purchased from DSM (Singapore). All other chemical reagents were of analytical grade.
2.2. Experimental design
In this study, NaCN and PPI blends were used as wall materials. The total protein and fat contents of spray-dried powders were kept constant at 20% (w/w) and 50% (w/w) respectively, for all samples. Vitamin A palmitate, -carotene, and DHA were incorporated as core nutrients. The homogenization pressure, number of passes, and the inlet air temperature were varied for each sample. All the experiments were performed in the pilot scale to mimic the industrial manufacturing conditions.
2.3. Preparation of oil-in-water (O/W) emulsions and spray-dried powders
Three liquid streams including the oil blend (soy oil, 2527 IU/100 g Vitamin A palmitate, 300.4 mg/100 g β-carotene and 150 mg/100 g DHA), protein blend (NaCN and PPI), and corn syrup solid solution were compounded at a temperature between 55°C and 60°C using a high shear speed mixer (Essential Engineering, Singapore). Coarse emulsion was prepared by mixing the liquid mixtures for 30 min to ensure that all proteins were fully hydrated and no lumps were observed. Further homogenization was performed using a two-stage homogenizer (APV, Germany, model: G5 – 8, 7 BX) under various pressures from 2000 psig to 4000 psig to form a fine emulsion. All the prepared emulsions were set at total solid contents ranged from 30% to 33% (w/w).
The O/W emulsions were then treated with an ultra-high-temperature-short-time (UHTST) process at 121°C for 5 s. The heat-treated samples were further evaporated to achieve a total solid content of 45% (w/w) using a two-effect falling film evaporator (Model: SPX Anhydro 2FFTE-1TVR, Denmark; conditions: inlet flow at 60 l per hour, constant vacuum of 0.8 bar, steam pressure at 2.5 bar to product an outlet product concentrate of 65°C). After evaporation, the concentrates were spray-dried using a pilot scale spray dryer (SPX, Denmark; conditions: feed temperature at 60°C, feed flow rate at 20 kg per hour, and hot air flow rate at 550 kg per hour) equipped with a two-fluid nozzle atomization system (nozzle diameter at 0.45 mm). The inlet air temperature of the dryer was set for five different levels from 187°C to 127°C, and the outlet temperature was at 80°C. The targeted moisture content of final spray-dried powder was set at 2.5% (i.e. to produce a batch size of 20 kg powder, an estimation of about 23.3 kg water to be removed from a feed concentrate of 45% total solid during spray drying).
2.4. Characterization of the properties of emulsions
2.4.1. Droplet size
The droplet size in emulsions was measured before UHTST and after evaporation. The mean emulsion droplet size in each sample was measured using a laser diffraction particle size analyzer (LS 13320, Beckman Coulter Ltd., Florida, US). The refractive indices of water and oil were set as 1.33 and 1.45, respectively. All measurements were done in triplicated. The particle size was shown as the volume-weighted mean diameter (d4,3), which can be mathematically expressed by Equation (1):
where ni represents the number of droplets with diameter di.
2.5. Characterization of the properties of spray-dried powders
2.5.1. Glass transition temperature (Tg)
The glass transition temperature (Tg) of spray-dried powders were determined using a differential scanning calorimetry (DSC Q2000, TA Instruments, New Castle, USA). An amount of 5 mg of sample was placed in a hermetically sealed pan, against an empty pan as reference. Samples were first equilibrated at −50°C, followed by a heating process with the ramping rate at 2°C/min, until 140°C. Every 80 s, an auto-modulation was conducted at ±1°C. Tg was then analyzed and reported according to the DSC curves. Triplicated readings were taken for each sample.
2.5.2. Free fat and encapsulation efficiency (EE)
Encapsulation efficiency (EE) was determined by extracting un-encapsulated oil (i.e., free fat) present on the surface of microcapsules. The EE is expressed as the fraction of encapsulated oil over the total amount of oil, as shown in Equation (2):
where total oil is the total amount of oil added and surface oil is the amount of non-encapsulated oil presented on the surface of microcapsules.
The total oil was extracted and quantified using the Soxhlet extraction method. The non-encapsulated oil was determined using the following method. Spray-dried powder (2 g) was placed in a 50 mL centrifuge tube and then 20 mL of petroleum ether was added. The mixture was vortexed for 1 min and filtered. Afterward, 40 mL of petroleum was used to wash the tube, filter paper, and glass funnel twice. The filtrate was then collected and placed in a flat-bottom flask. After evaporating petroleum ether, the flat-bottom flask was placed in a vacuum oven at 60°C and dried to constant weight. The amount of non-encapsulated oil was calculated as the mass difference between the initial clean flask and the flask containing extracted oil residue.
2.5.3. Moisture content (MC)
The MC was measured using a moisture analyzer (HR83 Halogen Moisture Analyzer, Mettler Toledo, Switzerland). About 3 g of sample was spread on the weighing pan. The sample was heated to 100°C then held at that temperature until constant mass was achieved. Triplicated analyses were done for each sample. The equipment was reset and cooled to <50°C before the next analysis.
2.5.4. Water activity (aw)
The aw was measured using an aw meter (4TE AquaLab Water Activity Meter, Decagon Devices, Inc, Pullman, USA). The instrument was first standardized using a standard with aw of 0.500. The sample cup was filled halfway with powder and the aw reading at 20°C was taken.
2.5.5. Morphology
The surface morphology of spray-dried powders was imaged using an environmental scanning electron microscope (ESEM; Quanta 450 FEG, FEI Company, Hillsboro, USA). The blocks were mounted on SEM stubs and coated with 60 nm of gold-palladium by a sputter coater. Images were taken at a magnification of 500 times and an accelerating voltage of 5.0 kV.
2.6. Stability of nutrients during storage
Spray-dried powders were packed in 400 g metal can with nitrogen flush to less than 2% residue oxygen (RO%), and then stored under accelerated temperature of 40°C (RH = 75%) for up to 150 days. Afterward, the content of Vitamin A palmitate, β-carotene, and DHA remained after 30 and 120 days of storage were measured using the following method. The entire lipid fraction was extracted with isooctane. The extracted samples were then centrifuged to separate the isooctane layer from the alcohol/water layer. An aliquot of the upper layer was injected into HPLC. Quantification of Vitamin A, β-carotene, and DHA were performed with a C18 RP-HPLC column (GE Healthcare, Waukesha, WI, USA), with a UV detector at 195 nm. Water with acetic acid (0.12%, v/v) was used as eluent A while acetonitrile was used as eluent B. The concentrations of Vitamin A, β-carotene and DHA were calculated by comparing peak areas of each sample to standards with known concentrations.
2.7. Oxidative stability
The generation of off-flavors was evaluated using the previous method (Rognlien, Duncan, O’keefe, & Eigel, Citation2012) with a few modifications. The panel consisted of six certified sensory panelists who were extensively trained for eight months and had successfully passed the screening test before sensory evaluation. On day 30, 60, 90, 120, and 150, panelists were asked to evaluate the powder by smelling and tasting. A category scale for oxidation ranging from 0 to 5 was used to quantify the off-flavor generated due to oxidation. The description of the scale was as follows: 0 – None; 1 – Very slight oxidation (cardboardy ½, no painty or rancid); 2 – Slight oxidation (cardboardy 1, no painty or rancid); 3 – Moderate oxidation (cardboardy 1½, no painty or rancid); 4 – Much oxidation (threshold to 1½ painty or rancid, may have cardboardy notes); 5 – Extreme oxidation (2–3 painty or rancid, may have cardboardy notes).
2.8. Statistical analysis
Difference amongst the group was analyzed by one-way ANOVA (p < 0.05). Linear correlation coefficients between each treatment and properties were determined, including processing conditions, nutrient retentions, and other powder properties. In the Principle component analysis (PCA), the treatment and measured variables with significant correlations were labeled as X and Y variables, respectively. Cluster analysis was performed with Euclidean distance based on the Y-score variables. Both linear correlation and PCA analysis were conducted following a previous study (Kuo & Lee, Citation2017). All the statistical analysis was performed using OriginPro 2017 (Origin Lab Corporation, Northampton, MA, USA).
3. Results and discussion
3.1. Effect of homogenization pressure on the properties of emulsions and powders
3.1.1. Droplet sizes of liquid emulsions
shows the changes in the droplet size of emulsions prepared using different homogenization conditions before UHTST and after evaporation. For all emulsions, the droplet size increased by almost two-fold after evaporation. It indicates the heat treatments (UHTST and Evaporation) result in a significant increase in droplet sizes and a potential impact on emulsifying properties. This observation was consistent with the study done by Franco, Partal, Ruiz-M Rquez, Conde, and Gallegos (Citation2000), where the average particle size of pea protein-stabilized O/W emulsion increased when treated at a temperature between 60 to 70°C. During evaporation, emulsion was heated to the range of 65 to 70°C, which induced globular pea proteins at the oil/water interface of the droplets to denature. This denaturation increased the protein–protein interactions amongst droplets, which contributed to the flocculation of droplets and the consequent increase in droplet size (Raikos, Citation2010).
Figure 1. Change in the size of droplet in emulsion prepared at different homogenization pressure and number of passes before UHT and after evaporation. Different lower case letters indicate significant difference (p < 0.05) in droplet size before UHT. Different upper case letters indicate significant difference (p < 0.05) in droplet size after evaporation.
Figura 1. Cambio en el tamaño de la gota de una emulsión preparada a distintas presiones de homogeneización y diferente número de pases antes de la uperización UHT y después de la evaporación. Las distintas letras minúsculas indican la existencia de una diferencia significativa (p < 0.05) en el tamaño de la gota antes de la uperización UHT. Las distintas letras mayúsculas muestran la existencia de una diferencia significativa (p < 0.05) en el tamaño de la gota después de la evaporación.
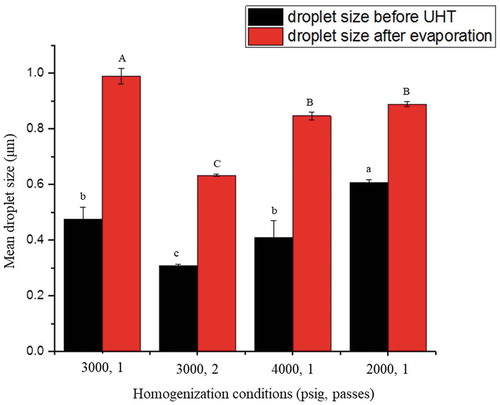
The sizes of droplets decreased when homogenization pressure increased from 2000 to 4000 psig. The fine emulsion prepared using homogenization pressure of 3000 psig for 2 passes had the smallest droplet size before UHT and after evaporation. This finding agreed with previous research that either increasing the homogenization pressure or the number of passes from one to two was effective in reducing droplet size (Yuan, Gao, Zhao, & Mao, Citation2008). The result also suggested that increasing the number of homogenization cycles could be more effective in reducing the size of droplet than increasing the total homogenization pressure. However, repetition of emulsification process or increasing homogenization cycles are also associated with recoalescence, which could lead to a larger droplet size. The size of final droplet is a consequence of the competition between disruption and recoalescence, and the final size increases while the rate of recoalescence is higher than the rate of disruption. Recoalescence could be mitigated by increasing the viscosity and utilizing fast-adsorbing emulsifiers (Mahdi Jafari, He, & Bhandari, Citation2006; Mao et al., Citation2009).
3.1.2. Physical properties of spray-dried powders
Caking is a deleterious physical change that might occur in spray-dried products, which is undesirable and could lead to a severe quality issue. The susceptibility of powders to caking is related to Tg. shows the physical properties of spray dried powders including MC, aw and Tg, under different processing conditions. It indicated a positive correlation between moisture content and aw, but negatively with Tg.
Table 1. Effect of processing conditions (homogenization pressures and spray drying inlet temperature) on free fat content, moisture content, water activity, and glass transition temperature of spray-dried powders.
Tabla 1. Efecto de las condiciones de procesamiento (presión de homogeneización y temperatura de entrada del secado por aspersión) en el contenido de grasa libre y de humedad, en la actividad del agua y la temperatura de transición vítrea de los polvos secados por aspersión.
Encapsulation efficiency is a critical parameter to assess the quality of emulsion-based spray-dried powders by measuring the residual free-fat content on the surface. Products with high free-fat content are prone to lipid oxidation and usually possess poor dispersibility in water, as well as poor powder flowability (Sharma et al., Citation2012). Therefore, the reduction of free fat is essential for improving the EE%, oxidative stability, and redispersibility (Tang & Li, Citation2013). By comparing different homogenization pressures ()), it was found that the sample with homogenization pressure of 2000 psig was significantly lower in EE% than other samples (p < 0.05). It agreed with the results of emulsion droplet size, where the sample processed with the lowest homogenization pressure at 2000 psig had the largest droplet size. The results implied that low homogenization pressure was insufficient for proteins to form a viscoelastic film at the oil–water interface, which consequently resulted in oil leakage during drying. Increasing homogenization pressure or the number of passes increased the amount of protein at the oil/water interface and the thickness of the viscoelastic film around the dispersed droplets, which helped to improve the stability of emulsion, and reduce the migration of fat to the surface of particles during spray drying (Park & Drake, Citation2017), and thus reduce the free fat content (Vignolles, Jeantet, Lopez, & Schuck, Citation2007).
Figure 2. (a) Effect of homogenization pressure and the number of passes on the encapsulation efficiency (EE%) of spray-dried powders. (b) Effect of inlet air temperature on the encapsulation efficiency (EE%) of spray-dried powders. Different letters mean significant difference at 95% confidence level.
Figura 2. (a) Efecto de la presión de homogeneización y del número de pases en la eficiencia de encapsulación (EE%) de los polvos secados por aspersión. (b) Efecto de la temperatura del aire de entrada en la eficiencia de encapsulación (EE%) de polvos secados por aspersión. Las distintas letras indican diferencias significativas a un nivel de confianza de 95%.
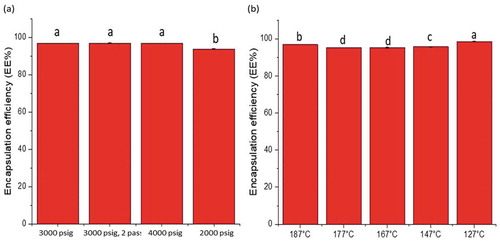
3.1.3. Stability of nutrients during storage
shows the retentions of different nutrients under different homogenization conditions after one month and four months of storage at accelerated temperature 40°C. The retention of DHA and vitamin A palmitate decreased by 24.0–26.7% and 17.0–19.8%, respectively, at the end of the four-month storage. There was no significant difference (p > 0.05) between different homogenization conditions. However, a significant difference was found in the retention of β-carotene. -carotene decreased by 4.40% to 25.2% at the end of 120-day storage. The emulsion that was homogenized twice had the smallest droplet size, and the spray-dried powder had the best retention of
-carotene and highest EE%. It is suggested that homogenizing the sample for more passes assist in the formation of more stable and uniform emulsions, which provides greater protection for encapsulated micro-nutrients. It is also notable that only β-carotene showed a significant difference in retention during storage other than vitamin A and DHA. This observation could be due to the high susceptibility of β-carotene to lipid oxidation compared to the other two (Tan & Nakajima, Citation2005).
Figure 3. Effect of homogenization pressure and number of passes on the retention of (a) DHA, (b) Vitamin A, and (c) β-carotene after 30 days (i.e. 1 M) and 120 days (i.e. 4 M) of storage; effect of spray drying inlet air temperature on the retention of (d) DHA, (e) Vitamin A palmitate, and (f) β-carotene after 30 days (i.e. 1 M) and 120 days (i.e. 4 M) of storage. Different lower case and upper case letters mean significant difference at 95% confidence level after 1 M and 4 M of storage, respectively.
Figura 3. Efecto de la presión de homogeneización y del número de pases en la retención de (a) ácido docosahexaenoico DHA, (b) vitamina A, y (c) β-caroteno después de 30 días (esto es, 1 M) y 120 días (4 M) de almacenamiento; efecto de la temperatura del aire de entrada en el secado por aspersión en la retención de (d) DHA, (e) palmitato de vitamina A y (f) β-caroteno después de 30 días (1 M) y 120 días (4 M) de almacenamiento. Las distintas letras minúsculas y mayúsculas indican diferencias significativas a un nivel de confianza de 95% después de 1 M y de 4 M de almacenamiento, respectivamente.
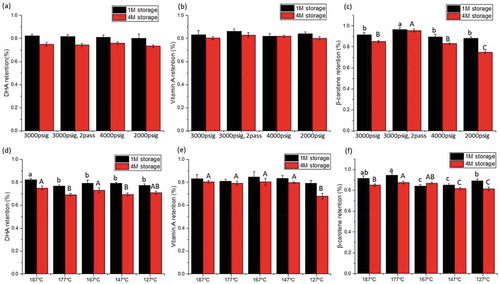
3.2. Effect of the inlet air temperature on the properties of spray-dried powders
3.2.1. Physical properties of spray-dried powder
EE% is associated with the migration of fats during the falling-rate drying period and the extent of interfacial coverage around the dispersed particles in the emulsion. According to ), the highest EE% was found in the sample processed with low inlet air temperature (127°C). In this case, low inlet air temperature induces a mild drying process, in which a limited falling-rate period was involved. Since migration of fats occurs primarily in the falling-rate period (Lukasiewicz, Citation1989), limiting this stage could result in a relatively small amount of surface fat. The EE% decreased when the inlet air temperature increased to 167°C. Similar results were also obtained by Nijdam and Langrish (Citation2006) who found that the EE% was higher for milk powder spray dried at 120°C than at 200°C. At higher temperature, fat becomes more mobile and migrates more readily to the surface of particles, increasing the free-fat content. Interestingly, when the inlet air temperature was further increased to 187°C in this study, a decrease in free fat or increase in EE% was observed. A possible explanation would be at a higher temperature, the rate of evaporation of water was also higher and led to a rapid crust formation before fat could migrate to the surface of spray-dried powder (Nijdam & Langrish, Citation2006), which resulted in lower free-fat content.
summarizes the impact of inlet air temperature on the different physical properties of spray-dried powders. When the inlet air temperature decreased from 187°C to 127°C, the MC of powder increased from 2.33% to 5.54% and the aw increased from 0.188 to 0.635. Accordingly, Tg of final products reduced from 97.12°C to 40.77°C. There were significant linear correlations (p < 0.05) between these parameters. Typically, using a higher inlet air temperature is more likely to achieve a complete drying process, and the products would have lower moisture content (Arya & Thakur, Citation1990). Though a higher EE% was achieved for the sample processed at 127°C, the powder became lumpy after 30 days, and serious caking occurred after 150 days of storage at 40°C. It was attributed to its high aw (0.635) and low Tg (40.77°C), which agreed with previous studies (Huang et al., Citation2014; Liu, Citation2010; Sauvant, Cansell, Sassi, & Atgié, Citation2012).
3.2.2. Stability of nutrients during storage
The effect of inlet air temperatures on the retention of different nutrients after storage is shown in ). -carotene, DHA, and Vitamin A palmitate decreased by 12.4% to 18.6%, 25.0% to 30.7%, and 19.5% to 32.0%, respectively, at the end of 120 days of storage. There was no clear trend between inlet air temperature and the retention of
-carotene and DHA. However, there was a significant positive correlation (p < 0.05; R = 0.815) between the inlet air temperature and the retention of Vitamin A palmitate (see ). Arya & Thakur (Citation1990) reported that Vitamin A palmitate is sensitive to moisture and when aw increased, its degradation also increased significantly. This finding was consistent with current experimental data in , which showed that there were negative linear correlations between the retention of vitamin A palmitate and MC (p < 0.05, R = −0.952) or aw (p < 0.05, R = −0.921).
Table 2. Pearson correlations between processing parameters and properties of spray-dried powders. Positive values indicate positive correlations between two attributes, and negative values indicate negative correlations between two attributes. Values that deviated from 0 indicate strong correlation, and significant correlations are marked with asterisk signs.
Tabla 2. Correlaciones de Pearson entre los parámetros de procesamiento y las propiedades de los polvos secados por aspersión. Los valores positivos indican correlaciones positivas entre dos atributos, mientras que los valores negativos señalan correlaciones negativas entre dos atributos. Los valores que se desvían de 0 muestran una correlación fuerte; las correlaciones significativas están indicadas por un asterisco.
3.3. Pearson’s correlation
Pearson correlation analysis was performed to identify if processing conditions and properties of products are significantly correlated. In , there was a significant positive correlation between the inlet air temperature and the retention of vitamin A palmitate. There was also a good correlation between homogenization pressure and the retention of β-carotene. The results from this study suggested that increasing homogenization pressure or the number of homogenization passes helped to protect β-carotene in emulsions with droplet sizes within micron range. MC was found to have a negative correlation with inlet air temperatures and vitamin A retention. Lastly, a significant negative correlation was also found between aw and Tg, indicating that aw could be a better parameter to predict Tg than MC.
Principal component analysis (PCA) and cluster analysis were performed to differentiate and group processing conditions based on their properties of dry powders and nutrients retention. As shown in , four clusters were identified. The results show that the clusters of 187°C inlet temperature coupled with a higher homogenization pressure (including 3000 psig, 1 or 2 passes, and 4000 psig, 1 pass) share the similarities of higher DHA and β-carotene retention, and higher Tg. Spray drying at 127°C inlet temperature led to a better EE%; however, higher degradations of all the 3 nutrients were observed during storage due to a higher MC and aw. The study demonstrated that the homogenization conditions and spray drying inlet air temperature have significant impacts on the physical properties and nutrients stability of spray-dried powders.
Figure 4. Principle component analysis biplot of Factor 1 and Factor 2 by the correlation matrix of mean properties results with a total of 76.25% variability. Factors were rotated using varimax rotation. Each formulation was mapped on the PCA diagram based on their differences on each property. A cluster analysis was performed and the results were shown as green circles, indicating clustered samples possessing similar properties. Powder microcapsule properties include moisture content, water activity, glass transition temperature (Tg), encapsulation efficiency, and nutrients (Vitamin A, DHA, and β-carotene) retention.
Figura 4. El análisis de los componentes principales biespaciales del Factor 1 y el Factor 2 empleando la matriz de correlación de propiedades medias arroja un total de 76.25% de variabilidad. Los factores fueron rotados usando una rotación varimax. Cada formulación quedó mapeada en el diagrama PCA según sus diferencias en cada propiedad. Se realizó un análisis de clúster y los resultados se muestran como círculos verdes, lo que indica que las muestras en clústeres poseen propiedades similares. Las propiedades de las microcápsulas en polvo incluyen el contenido de humedad, la actividad del agua, la temperatura de transición vítrea (Tg), la eficiencia de encapsulación y la retención de nutrientes (vitamina A, DHA y β-caroteno).
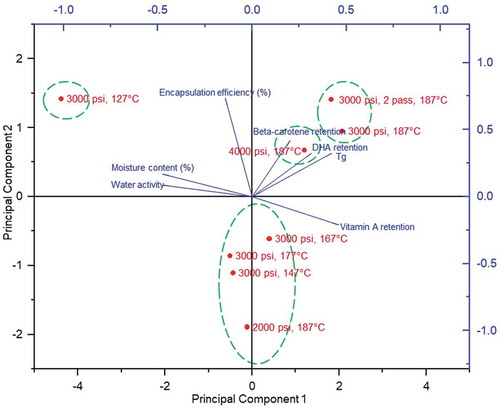
3.4. Morphology
shows the SEM images of microcapsules obtained at a magnification of 500 times. All the particles in dry powders show spherical shape, which is a typical presentation for the morphology of protein-rich spray-dried powder. All the samples exhibit intact and smooth surfaces without major crack, leaking or breakage. However, the particles were shriveled, and the shrinkage could be induced by water loss during spray drying (Koga, Andrade, Ferruzzi, & Lee, Citation2016). A similar presentation was observed on spray-dried microcapsules prepared by Bajaj et al. (Citation2017) using pea protein as wall material. The distribution of particle sizes varied among samples and was ranged broadly from 5 to 100 μm. The heterogeneous particle size distribution was also reported in other studies as a consequence of the top-down process (Koga et al., Citation2016; Qv, Zeng, & Jiang, Citation2011).
Figure 5. SEM images of microcapsules as powder samples that were processed with different homogenization conditions and spray drying inlet air temperatures, R1 (3000 psig, 1 pass, 187°C), R2 (3000 psig, 2 pass, 187°C), R3 (4000 psig, 1 pass, 187°C), R4 (2000 psig, 1 pass, 187°C), R5 (3000 psig, 1 pass, 177°C), R6 (3000 psig, 1 pass, 167°C), R7 (3000 psig, 1 pass, 147°C), and R8 (3000 psig, 1 pass, 127°C). The images were taken at a magnification of 500 times. (Length of dotted line = 100 m).
Figura 5. Imágenes SEM de las microcápsulas como muestras en polvo procesadas con distintas condiciones de homogeneización y de temperaturas de aire de entrada para el secado por aspersión, R1 (3000 psi, 1 pase, 187°C), R2 (3000 psig, 2 pases, 187°C), R3 (4000 psig, 1 pase, 187°C), R4 (2000 psig, 1 pase, 187°C), R5 (3000 psig, 1 pase, 177°C), R6 (3000 psig, 1 pase, 167°C), R7 (3000 psig, 1 pase, 147°C) y R8 (3000 psig, 1 pase, 127°C). Las imágenes fueron ampliadas 500 veces. (Extensión de la línea punteada = 100 m)
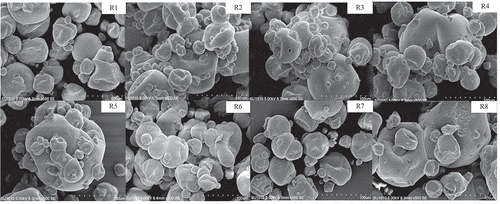
3.5. Sensory evaluation and oxidative stability
shows the perceived extent of oxidation evaluated by the sensory panel after 30, 60, 90, 120, and 150 days of storage at 40°C. Amongst samples spray-dried at different inlet air temperatures, lower inlet air temperature (i.e. 127°C and 147°C) tended to have a better oxidation score. A low inlet air temperature led to a small amount of peroxide formed (Aghbashlo, Mobli, Madadlou, & Rafiee, Citation2013). Since lipid oxidation is a free-radical chain reaction (Sánchez-Paz et al., Citation2008), spray drying at high inlet air temperatures can promote free-radical generation, which eventually caused extensive lipid oxidation during storage. When spray drying at a higher inlet air temperature (i.e. 187°C), the oxidation stability can be improved significantly by adjusting homogenization conditions. Among samples processed with different homogenization conditions, sample R2 (3000 psig, 2 passes, 187°C inlet air temperature) had the least perceived extent of oxidation after storage, followed by Sample R3 (4000 psig). This observation implied that increasing the homogenization pressure and the number of passes reduced the perceived extent of oxidation of products stored under accelerated conditions. Let, Jacobsen, Sørensen, and Meyer (Citation2007) found that droplet size was directly correlated to lipid oxidation. By homogenizing the sample for more passes or increasing the homogenization pressure, smaller droplet sizes of emulsions can be formed. Here, smaller droplet sizes were helpful in retaining sensory properties and minimizing the generation of oxidative off-flavors compared to large droplet sizes.
Figure 6. Perceived extent of oxidation of spray-dried powders prepared using (a) different homogenization conditions and (b) different inlet air temperatures. Samples were evaluated by panelists after 30, 60, 90, 120, and 150 days of storage under accelerated storage condition (40°C). Results were the average of ratings by 6 trained sensory panelists.
Figura 6. Grado de oxidación percibido en los polvos secados por aspersión preparados utilizando (a) distintas condiciones de homogeneización y (b) distintas temperaturas de aire de entrada. Las muestras fueron evaluadas por panelistas después de 30, 60, 90, 120 y 150 días de almacenamiento bajo condiciones aceleradas de almacenaje (40°C). Los resultados reflejan el promedio de las calificaciones emitidas por seis panelistas sensoriales capacitados.
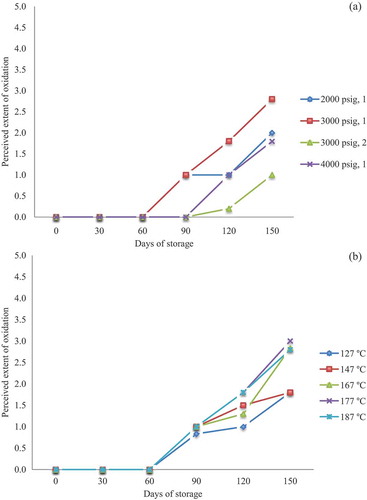
4. Conclusions
Homogenization and spray drying are important processes that require optimization to obtain emulsions and spray-dried powders with desired properties. In this study of mixed NaCN and PPI system, increasing homogenization pressure or the number of passes was found to increase the retention of β-carotene significantly, while increasing the inlet air temperature was effective in preserving vitamin A. Increasing the inlet air temperature from 127°C to 187°C led to a higher tendency of lipid oxidation of spray-dried powders. The oxidation stability can be improved significantly by adjusting homogenization conditions when spray drying at higher inlet air temperature (187°C). Two-pass homogenization helped to achieve a smaller droplet size of liquid emulsion, which resulted in lower free-fat content and higher β-carotene retention, along with better oxidative stability of powdered microcapsules. The results also indicated a potential inherent correlation between properties of liquid emulsion and powdered microcapsules. On the other hand, a lower spray drying inlet temperature at 127°C increased the moisture content and water activity and decrease the glass transition temperature of spray-dried powders, which consequently resulted in powder caking and nutrients degradation. These findings would be useful for determining the optimal processing conditions to produce spray-dried powders with desirable physicochemical properties and good retention of nutrients. Future studies should investigate a wider range of these factors coupled with the optimized method to discover the best combination of these processing parameters.
Disclosure statement
No potential conflict of interest was reported by the authors.
Additional information
Funding
References
- Aberkane, L., Roudaut, G., & Saurel, R. (2014). Encapsulation and oxidative stability of PUFA-Rich oil microencapsulated by spray drying using pea protein and pectin. Food and Bioprocess Technology, 7(5), 1505–1517.
- Aghbashlo, M., Mobli, H., Madadlou, A., & Rafiee, S. (2013). Influence of wall material and inlet drying air temperature on the microencapsulation of fish oil by spray drying. Food and Bioprocess Technology, 6(6), 1561–1569.
- Arya, S., & Thakur, B. (1990). Effect of water activity on vitamin A degradation in wheat flour (Atta). Journal of Food Processing and Preservation, 14(2), 123–134.
- Augustin, M. A., Sanguansri, L., & Bode, O. (2006). Maillard reaction products as encapsulants for fish oil powders. Journal of Food Science, 71(2). doi:10.1111/j.1365-2621.2006.tb08893.x
- Bajaj, P. R., Bhunia, K., Kleiner, L., Joyner, H. S., Smith, D., Ganjyal, G., & Sablani, S. S. (2017). Improving functional properties of pea protein isolate for microencapsulation of flaxseed oil. Journal of Microencapsulation, 34(2), 218–230.
- Bajaj, P. R., Tang, J., & Sablani, S. S. (2015). Pea protein isolates: Novel wall materials for microencapsulating flaxseed oil. Food and Bioprocess Technology, 8(12), 2418–2428.
- Bakry, A. M., Abbas, S., Ali, B., Majeed, H., Abouelwafa, M. Y., Mousa, A., & Liang, L. (2016). Microencapsulation of oils: A comprehensive review of benefits, techniques, and applications. Comprehensive Reviews in Food Science and Food Safety, 15(1), 143–182.
- Botrel, D. A., de Barros Fernandes, R. V., Borges, S. V., & Yoshida, M. I. (2014). Influence of wall matrix systems on the properties of spray-dried microparticles containing fish oil. Food Research International, 62, 344–352.
- Cano-Ruiz, M., & Richter, R. (1997). Effect of homogenization pressure on the milk fat globule membrane proteins. Journal of Dairy Science, 80(11), 2732–2739.
- Feng, Y., & Lee, Y. (2016). Surface modification of zein colloidal particles with sodium caseinate to stabilize oil-in-water pickering emulsion. Food Hydrocolloids, 56, 292–302.
- Feng, Y., & Lee, Y. (2017). Microfluidic fabrication of hollow protein microcapsules for rate-controlled release. RSC Advances, 7(78), 49455–49462.
- Fernandez-Avila, C., & Trujillo, A. (2016). Ultra-high pressure homogenization improves oxidative stability and interfacial properties of soy protein isolate-stabilized emulsions. Food Chemistry, 209, 104–113.
- Floury, J., Desrumaux, A., & Legrand, J. (2002). Effect of ultra‐high‐pressure homogenization on structure and on rheological properties of soy protein‐stabilized emulsions. Journal of Food Science, 67(9), 3388–3395.
- Franco, J. M., Partal, P., Ruiz-M Rquez, D., Conde, B., & Gallegos, C. (2000). Influence of pH and protein thermal treatment on the rheology of pea protein-stabilized oil-in-water emulsions. Journal of the American Oil Chemists’ Society, 77(9), 975–984.
- Hebishy, E., Buffa, M., Guamis, B., Blasco-Moreno, A., & Trujillo, A.-J. (2015). Physical and oxidative stability of whey protein oil-in-water emulsions produced by conventional and ultra high-pressure homogenization: Effects of pressure and protein concentration on emulsion characteristics. Innovative Food Science & Emerging Technologies, 32, 79–90.
- Hebishy, E., Buffa, M., Juan, B., Blasco-Moreno, A., & Trujillo, A.-J. (2017). Ultra high-pressure homogenized emulsions stabilized by sodium caseinate: Effects of protein concentration and pressure on emulsions structure and stability. LWT-Food Science and Technology, 76, 57–66.
- Hebishy, E., Zamora, A., Buffa, M., Blasco-Moreno, A., & Trujillo, A.-J. (2017). Characterization of whey protein oil-in-water emulsions with different oil concentrations stabilized by ultra-high pressure homogenization. Processes, 5(1), 6.
- Huang, H., Hao, S., Li, L., Yang, X., Cen, J., Lin, W., & Wei, Y. (2014). Influence of emulsion composition and spray-drying conditions on microencapsulation of tilapia oil. Journal of Food Science and Technology, 51(9), 2148–2154.
- Ixtaina, V. Y., Julio, L. M., Wagner, J. R., Nolasco, S. M., & Tomás, M. C. (2015). Physicochemical characterization and stability of chia oil microencapsulated with sodium caseinate and lactose by spray-drying. Powder Technology, 271, 26–34.
- Kim, H., Decker, E., & McClements, D. (2002). Impact of protein surface denaturation on droplet flocculation in hexadecane oil-in-water emulsions stabilized by β-lactoglobulin. Journal of Agricultural and Food Chemistry, 50(24), 7131–7137.
- Koga, C. C., Andrade, J. E., Ferruzzi, M. G., & Lee, Y. (2016). Stability of trans‐resveratrol encapsulated in a protein matrix produced using spray drying to UV light stress and simulated gastro‐intestinal digestion. Journal of Food Science, 81(2). doi:10.1111/1750-3841.13176
- Kuo, W. Y., & Lee, Y. (2017). Correlating structural properties to sodium release of model solid lipoproteic colloids. Journal of Food Engineering, 203, 16–24.
- Let, M. B., Jacobsen, C., Sørensen, A.-D. M., & Meyer, A. S. (2007). Homogenization conditions affect the oxidative stability of fish oil enriched milk emulsions: Lipid oxidation. Journal of Agricultural and Food Chemistry, 55(5), 1773–1780.
- Liu, M. (2010). Understanding the mechanical strength of microcapsules and their adhesion on fabric surfaces (Doctoral dissertation). University of Birmingham, Birmingham, UK. Retrieved from http://etheses.bham.ac.uk/673/
- Lukasiewicz, S. J. (1989). Spray-drying ceramic powders. Journal of the American Ceramic Society, 72(4), 617–624.
- Mahdi Jafari, S., He, Y., & Bhandari, B. (2006). Nano-emulsion production by sonication and microfluidization – A comparison. International Journal of Food Properties, 9(3), 475–485.
- Mao, L., Xu, D., Yang, J., Yuan, F., Gao, Y., & Zhao, J. (2009). Effects of small and large molecule emulsifiers on the characteristics of β-carotene nanoemulsions prepared by high pressure homogenization. Food Technology and Biotechnology, 47(3), 336–342.
- Mukherjee, D., Chang, S. K., Zhang, Y., & Mukherjee, S. (2017). Effects of ultra-high pressure homogenization and hydrocolloids on physicochemical and storage properties of soymilk. Journal of Food Science, 82(10), 2313–2320.
- Nijdam, J., & Langrish, T. (2006). The effect of surface composition on the functional properties of milk powders. Journal of Food Engineering, 77(4), 919–925.
- Ogrodowska, D., Tańska, M., & Brandt, W. (2017). The influence of drying process conditions on the physical properties, bioactive compounds and stability of encapsulated pumpkin seed oil. Food and Bioprocess Technology, 10(7), 1265–1280.
- Olenskyj, A., Feng, Y., & Lee, Y. (2017). Continuous microfluidic production of zein nanoparticles and correlation of particle size with physical parameters determined using CFD simulation. Journal of Food Engineering, 211, 50–59.
- Park, C. W., & Drake, M. (2017). The effect of homogenization pressure on the flavor and flavor stability of whole milk powder. Journal of Dairy Science, 100(7), 5195–5205.
- Qv, X. Y., Zeng, Z. P., & Jiang, J. G. (2011). Preparation of lutein microencapsulation by complex coacervation method and its physicochemical properties and stability. Food Hydrocolloids, 25(6), 1596–1603.
- Raikos, V. (2010). Effect of heat treatment on milk protein functionality at emulsion interfaces. A review. Food Hydrocolloids, 24(4), 259–265.
- Rognlien, M., Duncan, S., O’keefe, S., & Eigel, W. (2012). Consumer perception and sensory effect of oxidation in savory-flavored yogurt enriched with n-3 lipids. Journal of Dairy Science, 95(4), 1690–1698.
- Sánchez-Paz, V., Pastoriza-Gallego, M. J., Losada-Barreiro, S., Bravo-Díaz, C., Gunaseelan, K., & Romsted, L. S. (2008). Quantitative determination of α-tocopherol distribution in a tributyrin/Brij 30/water model food emulsion. Journal of Colloid and Interface Science, 320(1), 1–8.
- Santhalakshmy, S., Bosco, S. J. D., Francis, S., & Sabeena, M. (2015). Effect of inlet temperature on physicochemical properties of spray-dried jamun fruit juice powder. Powder Technology, 274, 37–43.
- Sauvant, P., Cansell, M., Sassi, A. H., & Atgié, C. (2012). Vitamin A enrichment: Caution with encapsulation strategies used for food applications. Food Research International, 46(2), 469–479.
- Shamaei, S., Seiiedlou, S. S., Aghbashlo, M., Tsotsas, E., & Kharaghani, A. (2017). Microencapsulation of walnut oil by spray drying: Effects of wall material and drying conditions on physicochemical properties of microcapsules. Innovative Food Science & Emerging Technologies, 39, 101–112.
- Sharma, A., Jana, A. H., & Chavan, R. S. (2012). Functionality of milk powders and milk‐based powders for end use applications—A review. Comprehensive Reviews in Food Science and Food Safety, 11(5), 518–528.
- Silva, E. K., Azevedo, V. M., Cunha, R. L., Hubinger, M. D., & Meireles, M. A. A. (2016). Ultrasound-assisted encapsulation of annatto seed oil: Whey protein isolate versus modified starch. Food Hydrocolloids, 56, 71–83.
- Tan, C. P., & Nakajima, M. (2005). β-Carotene nanodispersions: Preparation, characterization and stability evaluation. Food Chemistry, 92(4), 661–671.
- Tang, C. H., & Li, X. R. (2013). Microencapsulation properties of soy protein isolate and storage stability of the correspondingly spray-dried emulsions. Food Research International, 52(1), 419–428.
- Tcholakova, S., Denkov, N., & Lips, A. (2008). Comparison of solid particles, globular proteins and surfactants as emulsifiers. Physical Chemistry Chemical Physics, 10(12), 1608–1627.
- Tolve, R., Condelli, N., Can, A., & Tchuenbou-Magaia, F. L. (2018). Development and characterization of phytosterol-enriched oil microcapsules for foodstuff application. Food and Bioprocess Technology, 11(1), 152–163.
- Usha, R., & Pothakamury, U. (1995). Fundamental aspects of controlled release in foods. Trends in. Food Sciences and Technological, 6(12), 397–406.
- Vignolles, M. L., Jeantet, R., Lopez, C., & Schuck, P. (2007). Free fat, surface fat and dairy powders: Interactions between process and product. A review. Le Lait, 87(3), 187–236.
- Wang, B., Duke, S. R., & Wang, Y. (2017). Microencapsulation of lipid materials by spray drying and properties of products. Journal of Food Process Engineering, 40(3). doi:10.1111/jfpe.12477
- Xu, J., Mukherjee, D., & Chang, S. K. (2018). Physicochemical properties and storage stability of soybean protein nanoemulsions prepared by ultra-high pressure homogenization. Food Chemistry, 240, 1005–1013.
- Yu, C., Wang, W., Yao, H., & Liu, H. (2007). Preparation of phospholipid microcapsule by spray drying. Drying Technology, 25(4), 695–702.
- Yuan, Y., Gao, Y., Zhao, J., & Mao, L. (2008). Characterization and stability evaluation of β-carotene nanoemulsions prepared by high pressure homogenization under various emulsifying conditions. Food Research International, 41(1), 61–68.
- Zhang, B., & Wang, Q. (2012). Development of highly ordered nanofillers in zein nanocomposites for improved tensile and barrier properties. Journal of Agricultural and Food Chemistry, 60(16), 4162–4169.