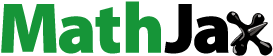
ABSTRACT
The fermented milk samples (n = 25) were examined for the presence of lactic acid bacteria (LAB) with broad antimicrobial potential. Isolates were identified based on biochemical profiling. Antibacterial activity of the LAB was determined against Salmonella typhimurium, Staphylococcus aureus, Escherichia coli and Listeria monocytogenes. L. lactis subsp. lactis showed broad antimicrobial spectrum compared to other isolates and probiotic evaluation showed viability of L. lactis at low pH (3), 3% and 0.3% bile salts. Bacteriocin from the LAB isolate (L. lactis subsp. lactis) was partially purified by precipitation, dialysis and microfiltration followed by molecular weight determination. The partially purified bacteriocin was used for biopreservation of poultry meat against target bacteria (S. aureus and S. typhimurium). The antimicrobial metabolites were also found active against S. typhimurium (11.2 ± 1.72 mm) and E. coli (13.4 ± 1.15 mm). LAB reduced the number of target bacteria by 10-fold in milk after 24 h of incubation. Crude bacteriocin reduced the number of target bacteria in poultry meat, from initial count of 105–106 CFU/g to 101 CFU/g for S. aureus and to 102 CFU/g for S. typhimurium respectively.
RESUMEN
Se examinaron muestras de leche fermentada (n = 25) en busca de bacterias de ácido láctico (LAB) con amplio potencial antimicrobiano. Los aislados se identificaron a partir de perfiles bioquímicos utilizando kits API. Al respecto, se comprobó la actividad antibacteriana de las LAB contra Salmonella typhimurium, Staphylococcus aureus, Escherichia coli y Listeria monocytogenes. El aislado bacteriano de L. lactis subsp. lactis mostró tener un amplio espectro antimicrobiano en comparación con otros aislados. Asimismo, la evaluación de probióticos dio cuenta de la viabilidad de L. lactis a pH bajo (3), concentración de sal (NaCl) al 3% y de sales biliares al 0.3%. Posteriormente, se purificó parcialmente la bacteriocina del aislado de LAB (L. lactis subsp. lactis) empleando precipitación, diálisis y microfiltración, determinándose luego su peso molecular. La bacteriocina parcialmente purificada se utilizó para la conservación biológica de carne de aves de corral contra bacterias diana (S. aureus y S. typhimurium). Además, se constató la actividad de los metabolitos antimicrobianos contra S. typhimurium (11.2 ± 1.72 mm) y E. coli (13.4 ± 1.15 mm). Se comprobó que, después de 24 horas de incubación, las LAB redujeron en 10 veces la cantidad de bacterias diana en la leche. La bacteriocina en bruto redujo el número de bacterias diana en la carne de ave, que del recuento inicial de 105-106 CFU/g pasaron a 101 CFU/g para S. aureus y hasta 102 CFU/g para S. typhimurium.
1. Introduction
Probiotics are nonpathogenic microbes, which induce positive health benefits on the host when ingested in adequate amount (FAO/WHO, Citation2006). The probiotic intake has been found helpful in reducing various ailments ranging from diarrhea to cancer. The probiotics also help in lactose intolerance, lower cholesterol level and enhance the nutrients utilization (Angmo, Kumari, & Bhalla, Citation2016). Lactic acid bacteria (LAB) play an important role in animal and human health by dominating and balancing the gut microflora. LAB exhibit antagonistic activity against pathogenic bacteria and inhibit the pathogens and spoilage microorganisms in food and food products (Ramirez-Chavarin, Wacher, Eslava-Campos, & Perez-Chabela, Citation2013). The strains of Lactococcus, Lactobacillus, and Pediococcus show good viability and lowering of pH in fermented foods, due to which they are predominantly used as a starter culture (Reis, Paula, Casarotti, & Penna, Citation2012; Todorov et al., Citation2017). Organic acids, hydrogen peroxide, bacteriocin and other metabolites produced by LAB, play a key role in their antimicrobial capabilities (Branco et al., Citation2010). The rising concern for human well-being and healthy food urges the scientists to explore novel and safe antibacterial compounds for food preservation. The synthetic and chemical preservatives are associated with health hazards therefore, the natural antimicrobials have always been preferred as food preservatives (Kaškonienė et al., Citation2017; Sadiq, Tarning, Aye Cho, & Anal, Citation2017).
Fermented foods are consumed worldwide, due to their health benefits and cultural importance. The desired microbes associated with fermented foods are beneficial for health and food preservation. In Asia, a wide variety of traditional fermented foods are consumed and these traditional fermented foods can be explored for the isolation of probiotics with preservation potential (Angmo et al., Citation2016). Lactococcus lactis has been recognized as generally regarded as safe (GRAS) by United States Food and Drug Administration (USFDA) and its antimicrobial metabolites, particularly nisin, are in use for the control of spoilage bacteria and foodborne pathogens in food (Akbar & Anal, Citation2014a). Bacteriocin is an effective substitute of chemical preservatives in the food industry. The bacteriocin-producing probiotics have an advantage in competitive interactions with the pathogenic bacteria from the food matrix (Todorov et al., Citation2017). LAB and their metabolites provide health and nutritional benefits to consumers. Presence of LAB in fermented milk products is not only the source of antimicrobials but also provides flavoring compounds such as acetaldehyde in yoghurt and cheese (Parvez, Malik, Ah Kang, & Kim, Citation2006).
Spoilage bacteria and foodborne pathogens are of great concern for the food industry and consumers (Akbar & Anal, Citation2015, Citation2011; Sadiq, Hanpithakpong, Tarning, & Anal, Citation2015). Approximately, 22.8 million cases of foodborne illnesses are recorded every year in South East Asia with a death toll of 27,600 (Akbar & Anal, Citation2013). Use of LAB for the bio-control of pathogens and food preservation is getting attention due to their additional health benefits apart from preservation potential and safe status (Kumaree, Akbar, & Anal, Citation2015; Reis et al., Citation2012). The use of LAB and its bacteriocin in a combination with other preservatives is commercially applicable in hurdle technology for shelf life extension of food (Jofre et al., Citation2008). The aim of this study was to isolate the bacteriocinogenic L. lactis subsp. lactis from fermented milk products and to evaluate its antimicrobial and probiotic characteristics. The LAB was further evaluated for its preservation potential against foodborne bacteria in poultry meat and cow milk samples.
2. Materials and methods
2.1. Isolation and identification of lactic acid bacteria
The local fermented milk products (n = 25) were collected from the greater Bangkok region of Thailand aseptically in sterile sample bottles and transported to Biotechnology Laboratory of the Asian Institute of Technology Thailand. The local fermented milk products were further characterized into yogurt, lassi, cheese, cultured buttermilk, flavored fermented milk and five samples of each fermented milk product were collected. The isolation and identification of LAB were carried out by using the methods of Kumaree et al. (Citation2015) and Ghatani and Tamang (Citation2017) with slight modifications. Briefly, 10 mL of the sample was mixed aseptically with 90 mL of sterile normal saline (NaCl, 0.85% w/v) and further diluted serially up to 10−7. The diluted sample (0.1 mL) was spread over de Man-Rogosa-Sharpe (MRS) agar (Himedia India) supplemented with bromocresol purple (0.06%, w/v) and incubated anaerobically at 37°C for 48–72 h. The isolates were purified by sub-culturing on MRS agar and identified initially with the help of Gram staining (cell morphology and arrangement) and catalase activity (3% H2O2). The isolates were then finally confirmed by biochemical profiling using API kits (BioMérieux, Marcy l’Étoile, France) (Supplementary material, Table S2-S3).
2.2. Screening of antagonistic activity
Four prominent foodborne pathogens including two Gram-positive (Staphylococcus aureus, Listeria monocytogenes) and two Gram-negative (Salmonella typhimurium and Escherichia coli) bacteria were used to study the inhibitory action of the isolated LAB. Antibacterial activity was determined against the selected foodborne pathogens using the spot on lawn, agar well and mix culture methods following Akbar and Anal (Citation2014a). In the spot on lawn method, 10 µl of fresh LAB culture was spotted on the surface of dry MRS agar and incubated at 37℃ for 24–48 h. Molten nutrient agar (0.7% w/w agar) seeded with target pathogens was spread over the visible colonies of LAB and incubated further at 37°C for 16–20 h.
In agar well method, a lawn of target bacteria over Mueller-Hinton agar (Merck, Germany) with wells of 6 mm bored with sterile borer was used. Cell-free supernatants were obtained by centrifuging 24 h grown MRS broth culture of LAB at 8000 rpm and 4°C for 20 min. The supernatant (50 µl) of the LAB adjusted to pH 5.5 with 1 M NaOH and HCl and sterilized by passing through filter membranes (0.2 µm pore size, Minisart, Sartorius Stedim Biotech Co. Ltd., Germany) was added to the well and incubated at 37°C for 16–20 h.
In mix culture study the target bacteria and LAB were grown together in LAPTg broth (1.5% peptone, 1% tryptone, 1% glucose, 1% yeast extract, 0.1% tween-80; pH 6.5) with approximate initial inoculum of LAB (105–106CFU/mL) and target bacteria (104–105CFU/mL) and incubated at 37°C for 24–48 h. Growths of and target bacteria were determined by standard plate count using nutrient agar, mannitol salt agar (Himedia, India), xylose lysine deoxycholate agar (Difco, USA) and eosin methylene blue agar (Himedia, India), whereas, the growth of LAB was determined by plate count on MRS agar.
2.3. Probiotics and functional characteristics
The LAB with a broad spectrum of antibacterial activity was selected for further use in biopreservation. Probiotics properties of the selected LAB were examined by following Akbar, Sitara, Ali, Muhammad, and Khan (Citation2014b) and Kaushik et al. (Citation2009).
2.3.1. pH tolerance
Fresh culture (2 µl) of LAB (106–107 CFU/mL) was inoculated in MRS broths with a pre-adjusted pH of 3.0 with 1 M HCl, 7.0 and 10 with 1 M NaOH separately, and incubated at 37°C for 12–24 h. Normal MRS broth with same bacteria was used as control. The resistance to pH was determined by plate count method.
2.3.2. Salt and bile salt tolerance
Fresh culture (2 µl) of LAB was introduced into MRS agar plates and broths supplemented with 3% and 6.5% of common salt (NaCl) and 0.3%, 1.0%, and 2.0% of bile salt (Biomark, India) and incubated at 37°C for 4 h. Normal MRS agar and broth were used as control.
2.3.3. Growth at different temperatures
LAB (106–107 CFU/mL) were inoculated to MRS agar and broth and incubated at different temperatures (10, 25, 35 and 44°C) for 24 h to observe visible growth.
2.3.4. Hydrophobicity
Hydrophobicity of isolated LAB was determined by following Akbar et al. (Citation2014b). Fresh LAB culture was centrifuged for 10 min at 8000 rpm and washed with sterile normal saline. Cell suspension was measured for optical density (OD600) (A0). Toluene (1 mL) was mixed with 3 mL of the suspension and mixed well for 2 min and left standing for 15–20 min. The OD600 of the lower aqueous phase (A) was measured. Cell surface hydrophobicity (%H) was calculated using the following equation.
2.4. Partial purification and molecular mass determination of bacteriocin
LAB (5%) was grown in 1,000 mL MRS broth at 37°C for 24 h. Following the incubation, the broth was centrifuged at 8000 rpm for 15 min. The cell-free supernatant was then subjected to 80% ammonium sulfate precipitation (Maldonado-Barragán, Caballero-Guerrero, Martín, Ruiz-Barba, & Rodríguez, Citation2016). The precipitate was separated by centrifugation at 12,000 rpm for 40 min at 4°C and re-suspended in potassium phosphate buffer (50 mmol/l, pH 7.0) and exhaustively dialyzed overnight through 1000 Da molecular weight-cut-off-dialyzed membrane (Spectra/Por 7 dialysis tubing, 1 K MWCO, 38 mm flat width) against the same buffer. Final solution was filtered through 0.2 µm pore size diameter (Minisart, Sartorius Stedim Biotech Co. Ltd., Germany). Antibacterial activity of partially purified bacteriocin was confirmed by spotting 20 µl on the surface of nutrient agar seeded with target bacteria and incubated at 37°C for 16–24 h.
Sodium dodecyl sulfate polyacrylamide gel electrophoresis (SDS-PAGE) was performed in a 16.5% and 10% gel as described by Schägger and Von (Citation1987). Low molecular-weight peptide markers (BioRad, USA) were used as a standard to compare with the molecular mass of bacteriocin. The gel was stained with Coomassie brilliant blue R-250 (1 g/L) in 50% methanol and 10% acetic acid.
2.5. Protective culture study
The L. lactis subsp. lactis was applied against S. aureus and Salmonella typhimurium in pasteurized milk by following the method of Ołdak, Zielińska, Rzepkowska, and Kołożyn-Krajewska (Citation2017), with slight modifications. Salmonella typhimurium and S. aureus free pasteurized milk was used in this experiment. The fresh culture of L. lactis subsp. lactis (5 mL, 107–108 CFU/mL) in sterile normal saline was added to 45 mL of milk, whereas S. typhimurium and S. aureus (106–107 CFU/mL) were also added to milk samples already inoculated with L. lactic subsp. lactis and mixed well by vortex and incubated at 35 ± 2°C. The milk samples inoculated with S. typhimurium, S. aureus, and L. lactis subsp. lactis separately were used as control using the same incubation conditions. Number of the inoculated bacteria was counted from 0 h to 72 h with the help of standard plate count method using MRS medium for L. lactis subsp. lactis, Xylose Lysine Deoxycholate agar (XLD) (Difco, USA), for S. typhimurium and Mannitol Salt Agar (MSA) (Himedia, India) for S. aureus, whereas pH of the milk samples was also recorded each time using pH meter (Hanna, USA). Milk samples inoculated with each bacterium and without bacteria were used as a control in the study.
2.6. Biopreservation of poultry meat by bacteriocin
A challenge study for the biopreservation of fresh poultry meat was conducted using S. typhimurium and S. aureus as target bacteria by following the method of Akbar and Anal (Citation2014a) with slight modifications. Meat pieces with a surface area of 4 cm2, approximately equal to 10 g were contaminated with target bacteria (105–106 CFU/g). Partially purified bacteriocin (10 μg/g of meat) was spread equally on the surface of the contaminated meat pieces with the help of hand operated sterile spray bottles and kept at 6 ± 2°C for four days. The number of target bacteria was counted with the help of standard plate count method using XLD and MSA. Bacteriocin free samples incubated at the same condition were used as control.
2.7. Statistical analysis
Data were analyzed by one-way analysis of variance (ANOVA) and Tukey test to determine significant group differences (p < 0.05) between samples by using SPSS statistical software package (SPSS, version 23.0, USA).
3. Results and discussion
3.1. Isolation and antagonistic activity of lactic acid bacteria
Six different species were found in all 25 fermented milk samples analyzed for the presence of potential bacteriocinogenic LAB (Table S1). Based on the morphological and microscopic characteristics bacterial isolates were found to be bacilli (rods), and predominantly cocci (round) in short and long chain. The bacteria were biochemically identified with the help of API kits, and the antimicrobial spectrum against foodborne pathogens was determined. On the bases of prominent antimicrobial spectrum L. lactis subsp. lactis (Table S1) was further analyzed for its probiotic characteristic, bacteriocin extraction and for biopreservation. Lactococcus lactis subsp. lactis were found active against wide range of foodborne pathogens, used as target bacteria in this study. Rodrıguez et al. (Citation2012) reported that only 52 out of all 169 LAB isolates from infant feces showed inhibition zone higher than 10 mm against Escherichia coli and Listeria innocua. Malini and Savitha (Citation2012) reported 135 isolates of LAB from food sources including cheese, paneer, and sausages, belonged to the groups Lactobacillus, Lactococcus, Pediococcus and Bifidobacterium, and reported that only 75% of the isolates showed antibacterial activity.
The highest antibacterial activity of L. lactis subsp. lactis was found against Listeria monocytogenes (27.3 ± 1.4 mm) and Staphylococcus aureus (21.2 ± 1.2 mm). The antimicrobial activity against Salmonella typhimurium presented an inhibition zone of 11.2 ± 1.72 mm, whereas for Escherichia coli 13.4 ± 1.15 mm (.). The L. lactis subsp. lactis were found effective against the target bacteria in mix culture study in LAPTg broth and all the target bacterial growth were significantly (p < 0.05) reduced to undetectable (zero CFU/mL) from initial inocula 105–106 CFU/mL after 24–48 h incubation confirmed by standard plate count. Guessas, Hadadji, Saidi, and Kihal (Citation2007) reported the antagonistic effect of L. lactis subsp. lactis in agar plates and liquid milk. In this study L. lactis subsp. lactis isolated from fermented milk products showed antimicrobial potential against different tested pathogens. LAB previously isolated from fermented Indian products and cocoa fermentation were found effective against wide range of pathogenic bacteria; moreover, the antimicrobial effects of LAB were similar to the L. lactis subsp. lactis isolated in the current study (Angmo et al., Citation2016; Santos et al., Citation2016). Moreover, Ojha, Kerry, Alvarez, Walsh, and Tiwari (Citation2016) reported that cell-free extracts of Lactobacillus sakei obtained after fermentation of ultrasound treated samples showed remarkable antimicrobial activity against Staphylococcus aureus, Listeria monocytogenes, Escherichia coli and Salmonella typhimurium at lower concentrations compared to control.
Figure 1. Zone of inhibition of LAB and its metabolites against target bacteria (a) Zone of inhibition (ZI) of CFS (cell-free supernatant) of L. lactis by agar well diffusion and (b) Zone of inhibition (ZI) of L. lactis by spot on lawn method.
Figura 1. Zona de inhibición de LAB y sus metabolitos contra bacterias diana (a) Zona de inhibición (ZI) de CFS (sobrenadante libre de células) de L. lactis por difusión en agar y (b) Zona de inhibición (ZI) de L. lactis por el método de mancha en el césped “spot on lawn method.”
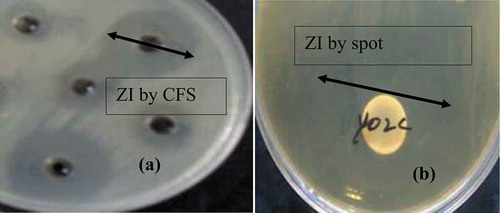
3.2. Probiotics characteristic
Probiotic characteristics of L. lactis subsp. lactis were determined by exposing to different ranges of pH and temperature, different concentrations of salt and bile salt and its percentage of hydrophobicity (). L. lactis subsp. lactis growth in acidic (pH 3) MRS broth decreased by 70% after 12 h incubation, whereas normal growth was observed in neutral (pH 7), and no media growth was observed in alkaline (pH 10) conditions. The bacterium was found tolerant to 3% salt (NaCl) concentration, whereas no growth was observed in 6.5% salt. L. lactis subsp. lactis were able to grow at temperatures 10, 25 and 35°C and no growth was observed at 44°C. The growth was found persistent in bile salt concentration 0.3%, while decrease in initial bacterial count was observed with increasing concentration of bile salt in the media. The percentage of hydrophobicity for L. lactis subsp. lactis was 61%. Pan and Zhang (Citation2008) reported good growth of L. lactis subsp. lactis in 0.1% bile salt and decrease in growth with the increasing bile salt concentration. They also reported the growth stability of L. lactis subsp. lactis at low pH (2.5 and 4.5) and the growth was maintained above 108 CFU/mL for 6 h. Resistance of LAB to bile salt concentration of 0.3% is considered as a good probiotics characteristic (Rodrıguez et al., Citation2012).
Table 1. Probiotic and functional characteristics of L. lactis subsp. lactis isolated from fermented milk.
Tabla 1. Características probióticas y funcionales de L. lactis subsp. lactis aislada de leche fermentada.
3.3. Protective culture study
The putative probiotic bacteria L. lactis subsp. lactis used against S. typhimurium and S. aureus in milk as a protective culture were found active against both of the target bacteria. It was observed that the pathogenic bacterial growth was significantly (p < 0.05) reduced with the passage of time ( & ). Target bacterial growth was initially not hindered by the growth of L. lactis subsp. lactis in a mix culture but subsequent reduction was noted after 24 h of incubation. Such condition might be due to the adjustment of L. lactis subsp. lactis to the environment initially and possibly due to the production of antibacterial compounds after the limitation of nutrients in the milk. The pH of test milk samples also dropped to 5.2 from initial pH 6.8, after 24 h incubation time. Akbar and Anal (Citation2014a) reported the tenfold reduction of S. aureus in a similar study conducted for the preservation of ready to eat poultry meat.
Figure 2. Protective culture study in milk using S. aureus and L. lactis subsp. lactis at 35 ± 2°C storage, Staphylococcus aureus (●), Lactococcus lactis subsp. lactis (■) Lactococcus lactis subsp. lactis control (x), Staphylococcus aureus control (▲).
Figura 2. Estudio de cultivo protegido en leche utilizando S. aureus y L. lactis subsp. lactis almacenado a 35 ± 2°C, Staphylococcus aureus (●), Lactococcus lactis subsp. lactis (■) Lactococcus lactis subsp. lactis control (x), Staphylococcus aureus control (▲).
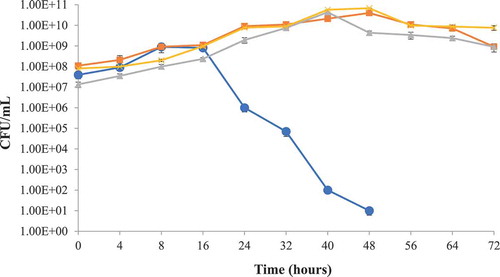
Figure 3. Protective culture study in milk using Salmonella typhimurium and L. lactis subsp. lactis at 35 ± 2°C storage, Salmonella typhimurium (●), Lactococcus lactis subsp. lactis (■) Lactococcus lactis subsp. lactis control (x), Salmonella typhimurium control (▲).
Figura 3. Estudio de cultivo protegido en leche utilizando Salmonella typhimurium y L. lactis subsp. lactis almacenado a 35 ± 2°C, Salmonella typhimurium (●), Lactococcus lactis subsp. lactis (■) Lactococcus lactis subsp. lactis control (x), Salmonella typhimurium control (▲).
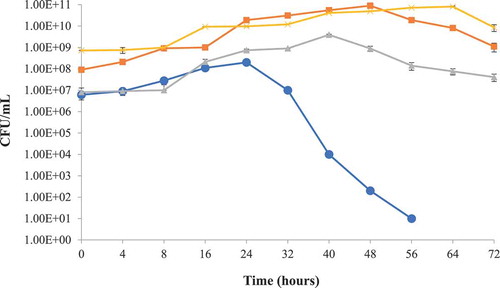
3.4. Biopreservation of poultry meat
The molecular mass of partially purified bacteriocin from L. lactis and standard low molecular weight protein marker is presented in . The diffused bands were observed for partially purified bacteriocin in the range of 14,437–6512 Dalton. This might be due to the crude nature of metabolites and other interfering molecules. The partially purified bacteriocin was found effective against test foodborne pathogens (S. typhimurium and S. aureus) used in the study. The partially purified bacteriocin was found active against the target bacteria in poultry meat, and the target bacterial count 105–106 CFU/g was significantly (p < 0.05) reduced to 101 CFU/g in case of S. aureus and 102 CFU/g in case of S. typhimurium after three days of incubation at low temperature (6 ± 2°C) (.).
Figure 4. Tricine SDS-PAGE for molecular mass analysis of partially purified bacteriocin from Lactococcus lactis subsp. Lactis. Diffused bands in the range of 14437–6512 Daltons.
Figura 4. SDS-PAGE de tricina para el análisis de la masa molecular de la bacteriocina parcialmente purificada de Lactococcus lactis subsp. lactis. Bandas difusas en el rango de 14437–6512 Daltons.
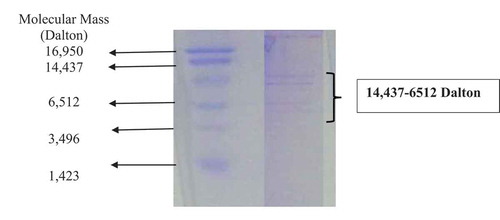
Figure 5. Growth of Staphylococcus aureus and Salmonella typhimurium in fresh poultry meat in the presence of crude bacteriocin at 6 ± 2°C storage, Salmonella typhimurium (x), Staphylococcus aureus (■) Salmonella typhimurium control (♦), Staphylococcus aureus control (▲).
Figura 5. Crecimiento de Staphylococcus aureus y Salmonella typhimurium en carne de ave fresca en presencia de bacteriocina en bruto almacenada a 6 ± 2°C, Salmonella typhimurium (x), Staphylococcus aureus (■) Salmonella typhimurium control (♦), Staphylococcus aureus control (▲).
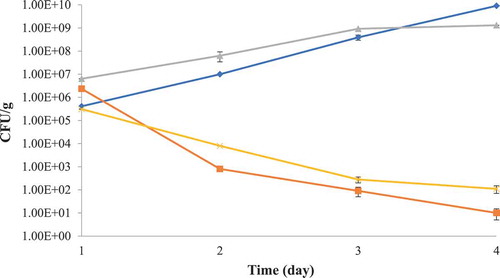
The use of LAB and its antimicrobial metabolites such as organic acid, hydrogen peroxide, and bacteriocin, is a promising ongoing development in food preservation with environmental friendly measures (Jofré et al., Citation2008). Todorov et al. (Citation2017) reported that bacteriocin isolated from LAB was found effective against L. monocytogenes and various other pathogens indicating the applications of bacteriocinogenic LAB in biopreservation of food products.
Antibacterial activity of meat-borne LAB has been reported in biopreservation of cooked meat products (Vermeiren, Devlieghere, & Debevere, Citation2004). Twomey et al. (Citation2000) reported broad antibacterial activity of L. lactis. Jofre et al. (Citation2008) reported the inactivation of Salmonella and L. monocytogenes by bacteriocin in combination with high hydrostatic pressure in ready-to-eat meat products. The antibacterial activity of the L. lactis subsp. lactis against other bacteria is due to its ability to produce a variety of antibacterial compounds including organic acids, hydrogen peroxides and bacteriocin (Rodgers, Citation2003).
4. Conclusion
It was concluded that LAB with antimicrobial activity has promising capabilities for its use against spoilage and pathogenic microorganisms in food. L. lactis subsp. lactis has the potential to control the number of unwanted bacteria in poultry meat and milk products. The use of L. lactis subsp. lactis as a starter culture or protective culture and its bacteriocin as antimicrobial agents in meat and milk products can prevent the growth of foodborne pathogens in the respective food products. However, further purification and identification of antimicrobial metabolites of probiotics, isolated from traditionally fermented foods can be a new source for novel and natural preservatives. Isolation of novel bacteriocin and other antimicrobials from environmentally friendly sources for biopreservation purpose is the demand for food industries and society for sustainability.
Acknowledgments
This study was conducted in the projects funded by AIP-PARC-2017 USAID and NRPU-HEC 6470.
Disclosure statement
No potential conflict of interest was reported by the authors.
Additional information
Funding
References
- Akbar, A., & Anal, A. K. (2011). Food safety concerns and food-borne pathogens, Salmonella, Escherichia coli and Campylobacter. FUUAST Journal of Biology, 1(1), 5.
- Akbar, A., & Anal, A. K. (2013). Prevalence and antibiogram study of Salmonella and Staphylococcus aureus in poultry meat. Asian Pacific Journal of Tropical Biomedicine, 3(2), 163.
- Akbar, A., & Anal, A. K. (2014a). Occurrence of Staphylococcus aureus and evaluation of anti-staphylococcal activity of Lactococcus lactis subsp. lactis in ready-to-eat poultry meat. Annals of Microbiology, 64(1), 131–138.
- Akbar, A., & Anal, A. K. (2015). Isolation of Salmonella from ready-to-eat poultry meat and evaluation of its survival at low temperature, microwaving and simulated gastric fluids. Journal of Food Science and Technology, 52(5), 3051–3057.
- Akbar, A., Sitara, U., Ali, I., Muhammad, N., & Khan, S. A. (2014b). Isolation and characterization of biotechnologically potent Micrococcus luteus strain from environment. Pakistan Journal of Zoology, 46(4), 967–973.
- Angmo, K., Kumari, A., & Bhalla, T. C. (2016). Probiotic characterization of lactic acid bacteria isolated from fermented foods and beverage of Ladakh. LWT-food Science and Technology, 66, 428–435.
- Branco, K. M. G. R., Nardi, R. M. D., Moreira, J. L. S., Nunes, A. C., Farias, L. M., Nicoli, J. R., & Carvalho, M. A. R. (2010). Identification and in vitro production of Lactobacillus antagonists from women with or without bacterial vaginosis. Brazilian Journal of Medical and Biological Research, 43(4), 338–344.
- FAO/WHO. (2006). Probiotics in food, health and nutritional properties and guidelines for evaluation. FAO Food and Nutritional Paper. No. 85. Rome: WHO/FAO.
- Ghatani, K., & Tamang, B. (2017). Assessment of probiotic characteristics of lactic acid bacteria isolated from fermented yak milk products of Sikkim, India: Chhurpi, Shyow, and Khachu. Food Biotechnology, 31(3), 210–232.
- Guessas, B., Hadadji, M., Saidi, N., & Kihal, M. (2007, October 27–31). Inhibition of Staphylococcus aureus growth by lactic acid bacteria in milk. African Crop Science Conference Proceedings (Vol.8, pp. 1159–1163). Egypt.
- Jofré, A., Aymerich, T., Monfort, J. M., & Garriga, M. (2008). Application of enterocins A and B, sakacin K and nisin to extend the safe shelf-life of pressurized ready-to-eat meat products. European Food Research and Technology, 228(1), 159.
- Kaškonienė, V., Stankevičius, M., Bimbiraitė-Survilienė, K., Naujokaitytė, G., Šernienė, L., Mulkytė, K., & Maruška, A. (2017). Current state of purification, isolation and analysis of bacteriocins produced by lactic acid bacteria. Applied Microbiology and Biotechnology, 101(4), 1323–1335.
- Kaushik, J. K., Kumar, A., Duary, R. K., Mohanty, A. K., Grover, S., & Batish, V. K. (2009). Functional and probiotic attributes of an indigenous isolate of Lactobacillus plantarum. PLoS One, 4(12), e8099.
- Kumaree, K. K., Akbar, A., & Anal, A. K. (2015). Bioencapsulation and application of Lactobacillus plantarum isolated from catfish gut as an antimicrobial agent and additive in fish feed pellets. Annals of Microbiology, 65(3), 1439–1445.
- Maldonado-Barragán, A., Caballero-Guerrero, B., Martín, V., Ruiz-Barba, J. L., & Rodríguez, J. M. (2016). Purification and genetic characterization of gassericin E, a novel co-culture inducible bacteriocin from Lactobacillus gasseri EV1461 isolated from the vagina of a healthy woman. BMC Microbiology, 16(1), 37.
- Malini, M., & Savitha, J. (2012). Heat stable bacteriocin from Lactobacillus paracasei subsp. tolerans isolated from locally available cheese: An in vitro study. E3 Journal of Biotechnology and Pharmaceutical Research, 3(2), 28–41.
- Ojha, K. S., Kerry, J. P., Alvarez, C., Walsh, D., & Tiwari, B. K. (2016). Effect of high intensity ultrasound on the fermentation profile of Lactobacillus sakei in a meat model system. Ultrasonics Sonochemistry, 31, 539–545.
- Ołdak, A., Zielińska, D., Rzepkowska, A., & Kołożyn-Krajewska, D. (2017). Comparison of antibacterial activity of Lactobacillus plantarum strains isolated from two different kinds of regional cheeses from Poland: Oscypek and Korycinski Cheese. BioMed Research International, 2017, 1–10.
- Pan, D., & Zhang, D. (2008). Function and safety assessment of Lactococus lactis subsp. lactis LB12 as potential probiotic strain. African Journal of Biotechnology, 7(22), 4037–4044.
- Parvez, S., Malik, K. A., Ah Kang, S., & Kim, H. Y. (2006). Probiotics and their fermented food products are beneficial for health. Journal of Applied Microbiology, 100(6), 1171–1185.
- Ramirez-Chavarin, M. L., Wacher, C., Eslava-Campos, C. A., & Perez-Chabela, M. L. (2013). Probiotic potential of thermotolerant lactic acid bacteria strains isolated from cooked meat products. International Food Research Journal, 20(2), 991.
- Reis, J. A., Paula, A. T., Casarotti, S. N., & Penna, A. L. B. (2012). Lactic acid bacteria antimicrobial compounds: Characteristics and applications. Food Engineering Reviews, 4(2), 124–140.
- Rodgers, S. (2003). Potential applications of protective cultures in cook-chill catering. Food Control, 14(1), 35–42.
- Rodríguez, E., Arqués, J. L., Rodríguez, R., Peirotén, Á., Landete, J. M., & Medina, M. (2012). Antimicrobial properties of probiotic strains isolated from breast-fed infants. Journal of Functional Foods, 4(2), 542–551.
- Sadiq, M. B., Hanpithakpong, W., Tarning, J., & Anal, A. K. (2015). Screening of phytochemicals and in vitro evaluation of antibacterial and antioxidant activities of leaves, pods and bark extracts of Acacia nilotica (L.) Del. Industrial Crops and Products, 77, 873–882.
- Sadiq, M. B., Tarning, J., Aye Cho, T. Z., & Anal, A. K. (2017). Antibacterial activities and possible modes of action of Acacia nilotica (L.) Del. against multidrug-resistant Escherichia coli and Salmonella. Molecules, 22(1), 47.
- Santos, T. T., Ornellas, R. M. S., Arcucio, L. B., Oliveira, M. M., Nicoli, J. R., Dias, C. V., & Vinderola, G. (2016). Characterization of lactobacilli strains derived from cocoa fermentation in the south of Bahia for the development of probiotic cultures. LWT-Food Science and Technology, 73, 259–266.
- Schägger, H., & Von Jagow, G. (1987). Tricine-sodium dodecyl sulfate-polyacrylamide gel electrophoresis for the separation of proteins in the range from 1 to 100 kDa. Analytical Biochemistry, 166(2), 368–379.
- Todorov, S. D., Stojanovski, S., Iliev, I., Moncheva, P., Nero, L. A., & Ivanova, I. V. (2017). Technology and safety assessment for lactic acid bacteria isolated from traditional Bulgarian fermented meat product “lukanka”. Brazilian Journal of Microbiology, 48(3), 576–586.
- Twomey, D. P., Wheelock, A. I., Flynn, J., Meaney, W. J., Hill, C., & Ross, R. P. (2000). Protection against Staphylococcus aureus mastitis in dairy cows using a bismuth-based teat seal containing the bacteriocin, lacticin 3147. Journal of Dairy Science, 83(9), 1981–1988.
- Vermeiren, L., Devlieghere, F., & Debevere, J. (2004). Evaluation of meat born lactic acid bacteria as protective cultures for the biopreservation of cooked meat products. International Journal of Food Microbiology, 96(2), 149–164.