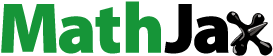
ABSTRACT
Bacterial cellulose films synthesized by Komagataeibacter xylinus were combined with glycerol and chitosan to obtain composite films with improved UV-barrier. In part 2, the interaction among the compounds was analyzed by scanning electron microscopy, UV-Vis and FT-IR spectra, thermogravimetry, and differential scanning calorimetry. The water permeability, water solubility and water retention capacity were analyzed by response surface methodology. The optical properties indicated that glycerol and chitosan improved the UV-barrier properties, obtaining average transmittance values in the UV regions below 5.15%. This fact opens multiple possibilities for applications in foods and products sensitive to sunlight radiation. Hence, these properties would facilitate the application of BC–glycerol–chitosan films on fresh food, with high moisture content and susceptible to oxidation by the sunlight radiation. In addition, the thermal properties of the films showed that they could be applied in heat-treated foods at temperatures until 135°C.
RESUMEN
Las películas de celulosa bacteriana sintetizadas por Komagataeibacter xylinus se combinaron con glicerol y quitosano para obtener películas compuestas con propiedades de barrera contra la radiación UV mejoradas. En la parte 2, la interacción entre los compuestos se analizó mediante microscopía electrónica de barrido, espectros UV-Vis y FT-IR, termogravimetría y calorimetría diferencial de barrido. La permeabilidad al vapor de agua, la solubilidad en agua y la capacidad de retención de agua se analizaron mediante la metodología de superficie de respuesta. Las propiedades ópticas indicaron que el glicerol y el quitosano mejoraron las propiedades de barrera a los rayos UV, obteniendo valores de transmitancia promedio en las regiones UV por debajo de 5,15%. Este hecho abre múltiples posibilidades de aplicación en alimentos y productos sensibles a la radiación solar. Por tanto, estas propiedades facilitarían la aplicación de películas de BC–glicerol–quitosano sobre alimentos frescos, con alto contenido de humedad y susceptibles de oxidación por la radiación solar. Además, las propiedades térmicas de las películas mostraron que podrían aplicarse con alimentos tratados térmicamente a temperaturas de hasta 135°C.
PALABRAS CLAVE:
1. Introduction
Consumers are increasingly concerned about environmental problems derived from high consumption and uncontrolled management of synthetic plastics. The main materials employed for food packaging are high-, low- and very low-density polyethylene, polyethylene terephthalate, polyvinyl chloride, polystyrene and polypropylene. These materials represent approximately 90% of total plastic production (Napper & Thompson, Citation2019).
Innovative biodegradable packaging materials with active functional properties have been developed to be more than a physical barrier to protect food from external factors (Janjarasskul & Suppakul, Citation2018; Khan et al., Citation2020; Wyrwa & Barska, Citation2017). An active packaging is a system where the packaging material, the packed product, and the environment interact to extend the shelf life, keeping its sensory and quality properties (Pang et al., Citation2019; Wyrwa & Barska, Citation2017).
The additives used to enrich the polymer matrix and to obtain a functional package are usually components with the ability to remove or adsorb elements such as gases (oxygen, carbon dioxide, ethylene) or light (UV blocking agents), to release antioxidant, antimicrobial or flavoring components, among other properties. This functional packaging could be useful to reduce foodstuff wastes (Ozdemir & Floros, Citation2004; Vilela et al., Citation2017).
Among the main potential materials to develop new biodegradable materials of natural origin is the bacterial cellulose (BC). It is excreted as exopolysaccharide by aerobic bacteria, such as Achromobacter, Alcaligenes, Aerobacter, Agrobacterium, Azotobacter, Komagataeibacter (formerly Gluconacet-obacter), Pseudomonas, Rhizobium, Sarcina, Dickeya and Rhodobacter (D. Lin et al., Citation2020; Mohammadkazemi et al., Citation2015; Ruka et al., Citation2012; Szymańska-Chargot et al., Citation2017; Yamada et al., Citation2012).
BC is a new material with interesting properties that have driven most of the research studies to develop new BC-based biomaterials focusing on possible applications in the medical field (Stumpf et al., Citation2018; Ullah et al., Citation2016). However, there is a significant lack of studies evaluating the potential of this material to develop active packaging films for application in food (Cazón & Vázquez, Citation2020a).
The high porosity and 3D structure of BC make it easy to modify its properties exsitu after the fermentation bioprocess, by dipping in baths with plasticizers or soluble polymer solutions. Note exsitu modifications are modifications done after the BC films are formed. This post-modification method has been also studied for regenerated cellulose-based films (Cazon et al., Citation2018, Citation2020b). However, the unique structure of BC and its capability for exsitu modification offer a great advantage to reach a higher number of interactions between the components (Cazón & Vázquez, Citation2020c; Reiniati et al., Citation2017).
Glycerol is used as a food additive authorized by the European Food Safety Authority (EFSA) (Mortensen et al., Citation2017). Due to its properties, such as non-volatility, cheap and low toxicity, glycerol is extensively used as a plasticizer in polysaccharide-based films, such as cellulose (Sirviö et al., Citation2018; Sun et al., Citation2018). Adding plasticizers to the polymer matrix is an effective technique to overcome the mechanical limitations of the films.
In addition to the desirable plasticizing effect of glycerol on BC samples, previous research also showed the positive effect of glycerol to improve the UV-light barrier properties of cellulose-based films. The presence of glycerol decreases the transmittance values in the UV-light region, although it also increases the opacity values to increase the transmittance values in the VIS-light region (Cazon et al., Citation2019b, Citation2020).
Chitosan has being considered for several applications in medical, nutrition and food technology fields (Khemir et al., Citation2020). Chitosan is a substance accepted as Generally Recognized As Safe (GRAS) (Montilla et al., Citation2013). Chitosan can be combined with other materials to improved functional properties of composite films. It is biodegradable, biofunctional, non-toxic, biocompatible, and has antimicrobial properties against a wide range of microorganisms. Chitosan is a waste of the shellfish industry (Aider, Citation2010; Cazón & Vázquez, Citation2020b; Morin-Crini et al., Citation2019).
Previous works have evaluated and characterized BC–chitosan films for application as a food packaging, obtaining improved mechanical resistance properties, but showing poor elasticity values (Ul-Islam et al., Citation2011). BC–chitosan–polyvinyl alcohol composite films were evaluated. Results indicated that chitosan and polyvinyl alcohol manifested a reinforcing and plasticizing effect on the BC structure, improving the mechanical, optical and thermal properties of the samples compared with the pure polymer (Cazon et al., Citation2019). However, polyvinyl alcohol produced an increase of the transmittance values of the samples in the UV-light region. Considering the results obtained in previous works and the interest in developing films with antimicrobial and UV radiation barrier properties, the combination of BC–chitosan–glycerol could be a good alternative as biodegradable films for food packaging. Glycerol could manifest a desired plasticizing effect in BC films while improving its UV-light barrier behavior. Chitosan could promote a reinforcing effect of the matrix improving the functionality of the films, thanks to its extensively evaluated antimicrobial properties (Rabea et al., Citation2003).
BC–glycerol–chitosan composites were previously evaluated as artificial dura mater candidates for head trauma (Angtika et al., Citation2018). BC–glycerol–chitosan and BC-glycerol composite films were mainly evaluated for medical application. No studies have evaluated its potential applications as an active food packaging with UV radiation barrier properties.
The present study was focused on analyzing the physicochemical and functional properties of BC–glycerol–chitosan composite films. The effect of the presence of each component and their interactions was studied and compared with those of raw BC films. In part 2, the effect of the ratio of glycerol and chitosan was analyzed, and mathematical models were obtained to assess the effect of the blend composition on water vapor permeability (WVP), water retention or swelling degree (%W) and solubility properties (%S). The structure, microstructure, thermal and optical (UV barrier, transparency, opacity, and color properties) and infrared spectral analyses of the composite samples were assessed by scanning electron microscopy, thermogravimetric, differential scanning calorimetry and UV-VIS and FTIR spectroscopy, respectively.
2. Materials and methods
Raw BC films were elaborated using Komagataeibacter xylinus from the “Colección Española de Cultivos Tipo” (CECT). D(+)-glucose monohydrate (99% extra pure), sodium hydroxide (98%), extra pure anhydrous sodium bromide (99%), chitosan (Mw 100000–300000) and acetic acid (99.5%) were purchased from Acros organics (Geel, Belgium) and yeast extract was provided by Scharlau Microbiology (Barcelona, Spain). Glycerol was purchased from Fisher Scientific (Pittsburgh, PA, US).
2.1. Preparation of films
The elaboration of raw BC films has been described in detail elsewhere (Cazon et al., Citation2019c, Citation2019). Washed BC films were combined with glycerol and chitosan solutions, after removing the excess water from the BC samples using filter paper sheets to obtain the composite films. The combination of the films in the different baths was carried out sequentially. A glycerol solution bath with concentrations ranging between 0% and 5% w/w and another aqueous chitosan solution bath at several concentrations ranging between 0% and 1% w/w with acetic acid 1% (v/v) were prepared. BC–glycerol and BC–chitosan samples were obtained by dipping and soaking for 1 h pure BC pellicles into the glycerol or chitosan solution baths with a specific composition as indicated in the experimental design (). Then, the composite films were placed in a Petri dish and dried at room temperature for 48 h, easing the evaporation of the solvent to obtain the final sample. BC–glycerol–chitosan samples were elaborated from the BC-glycerol samples to facilitate the diffusion of the plasticizer into the BC matrix before blending with chitosan. BC samples were immersed first in a glycerol bath with a specific concentration for 1 h. These samples were dried at room temperature for 24 h to facilitate the partial evaporation of the solvent. Then, the BC–glycerol samples were immersed in a chitosan bath with a specific concentration for 1 h to obtain the BC–glycerol-chitosan films. Finally, BC-glycerol-chitosan samples were dried for 48 h to get samples ready for conditioning and subsequent measurement. The overall elaboration process of BC films combined with glycerol and chitosan is shown in Part 1.
Table 1. Experimental results for water vapor permeability (WVP), water retention capability (%W) and solubility properties.
Tabla 1. Resultados experimentales de permeabilidad al vapor de agua (WVP), capacidad de retención de agua (% W) y propiedades de solubilidad
The composite films were cut into specific sizes depending on the test. The thickness (mm) was measured at five random locations using a thickness meter ET115S (Etari GmbH, Stuttgart, Germany). The samples were stored in desiccators with a saturated sodium bromide solution or silica gel for 5 days, as required for each test.
2.2. Scanning electron microscopy (SEM)
The microstructure and morphology of the BC–glycerol–chitosan composite samples were observed and analyzed by SEM. The dried and gold-coated samples were photographed with a high-vacuum microscope (JEOL JSM-6360LV, Jeol Ltd, Tokyo, Japan) at an accelerating voltage of 20 kV. Samples were attached on slides using conductive double-sided carbon tape.
2.3. Water vapor permeability, film solubility in water and water retention property
Water vapor permeability (WVP) was measured as described elsewhere (Cazon et al., Citation2019), following the ASTM Standard Test Method E96 (https://www.astm.org/Standards/E96.htm). Each test was carried out in triplicate.
Solubility in water (%S) and water retention (%W) were measured gravimetrically as described elsewhere (Cazon et al., Citation2019a), using film portions of 3 × 3 cm2 of each formulation. Each test was carried out in triplicate.
2.4. Optical properties
The percentage of transmittance of the samples was measured in the UV-Vis light regions (190–800 nm), using a spectrophotometer V-670 (Jasco Inc, Japan). The transparency and opacity values of the samples were calculated from the transmittance and absorbance values of the samples at 500 and 600 nm of wavelength, as described elsewhere (Cazon et al., Citation2019a). The test was run in duplicate for each sample.
The color of the samples was studied through the L*, a* and b* parameters. The CIE parameters were determined by the Spectra Manager software (Jasco Inc, Japan) established on the second standard observer with light source D65.
2.5. Fourier-transform infrared spectroscopy (FT-IR)
The presence of specific chemical groups and the crosslinking in the optimized samples were evaluated by an FT-IR (ABB Bomen 102 (ABB Ltd, Zurich Switzerland) spectrometer equipped with a universal attenuated total reflectance (UATR) accessory (SPECAC Golden Gate) with diamond crystal. Prior to the test, the selected samples were conditioned at 65 ± 2% relative humidity and 21 ± 1°C for 48 h. FTIR spectra were recorded at a spectral resolution of 4 cm−1 in the range 400–4000 cm−1.
2.6. Thermal properties of films (TGA/DSC)
A thermogravimetry and a differential scanning calorimetry equipment TGA/DSC 1 (Mettler Toledo, Switzerland) were used to analyze the thermal properties and stability of the BC–glycerol–chitosan samples at several glycerol:chitosan ratios. The samples were placed in hermetic aluminum pans and the test was carried out at a heating rate of 10°C/min from 50°C to 400°C, in atmosphere of N2 (50 mL/min).
2.7. Statistical analysis
The experimental design denoted the independent variables glycerol and chitosan content as A and B, respectively. The effect of the glycerol and chitosan content on the dependent variables (WVP, %W and %S) was calculated and evaluated following a complete factorial design. Cook’s distance was used to detect outliers (Cook, Citation1977) and the Box-Cox data transformation technique was used to reduce anomalies (Sakia, Citation1992). The statistical analysis was accomplished using Design Expert® 10.0.6 software (Stat-Ease, Inc., Minneapolis, MN, USA).
3. Results and discussion
BC–glycerol–chitosan composite samples were obtained by dipping in baths with several concentrations of glycerol (0–5%) and chitosan (0–1%). The average thickness of the samples ranged between 2.10 × 10−2 and 2.98 × 10−2 mm. The thickness of the samples increased when the chitosan and glycerol content increased.
3.1. Scanning electron microscopy (SEM)
SEM was used to analyze the microstructure of the composite films and the modification at the structural level due to the incorporation of glycerol and chitosan. SEM images and their analysis provide an insight into the structure of the polymer that helps to understand and explain the behavior of the composite film in subsequent analyses, such permeability properties. The successful incorporation of glycerol and chitosan can be observed in the SEM images of bottom and top sides () and in the SEM images of the cross section ().
Figure 1. Scanning electron microscopy images of the top and bottom sides of the bacterial cellulose–glycerol–chitosan composite films.
Figura 1. Imágenes de microscopía electrónica de barrido de los lados superior e inferior de las películas compuestas de celulosa bacteriana–glicerol–quitosano
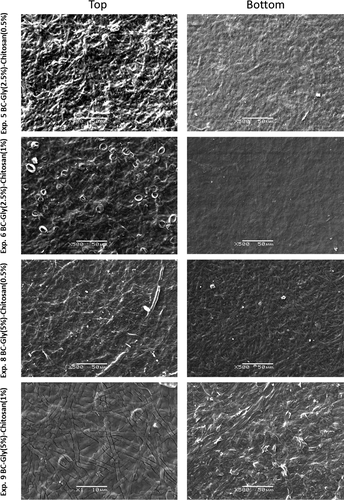
Figure 2. Scanning electron microscopy images of the cross section of the developed bacterial cellulose (BC) – glycerol (Gly) - chitosan composite films.
Figura 2. Imágenes de microscopía electrónica de barrido de la sección transversal de las películas compuestas de celulosa bacteriana (BC) - glicerol (Gly))- quitosano
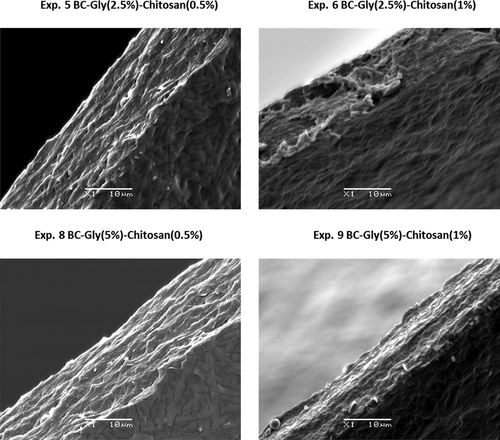
Previous reports indicated that chitosan and glycerol can diffuse through the pores of the BC matrix and interact with the microfibrils, promoting changes in the physicochemical properties (Cazon et al., Citation2019a, Citation2020a; Kim et al., Citation2011; Sun et al., Citation2018; Ul-Islam et al., Citation2011; Lin et al., Citation2013). In our work, the SEM images showed the effect of both components and their interactions on the BC microstructure. The increase of glycerol content enhanced the diffusion and retention of chitosan in the polymeric matrix, promoting a smooth and homogeneous surface (). Glycerol covered the BC fibers, increasing the accessible active groups to interact with chitosan, resulting in a higher interaction glycerol-chitosan among BC fibers.
The cross-section images showed how the increase of chitosan content promoted a more compact structure (). The increase of glycerol content increased the thickness and allowed obtaining a less compact structure () than BC samples with a higher chitosan content.
Accordingly, the SEM images of and suggested that the diffusion of chitosan and glycerol through BC microfibers was possible following the elaboration process detailed in the scheme of Part 1, promoting the interaction among the components resulting in a denser network with a lower porosity. After immersion of the BC film in glycerol solution bath, the high porosity of the 3D structure of BC eased the homogeneous spread of glycerol among the BC fibers. As a result, glycerol coated the BC fibers as shown in the SEM images. A similar behavior also shown in previous studies (Cazon et al., Citation2020a; Sun et al., Citation2018).
3.2. Evaluation of the water vapor permeability properties
Each food product possesses a specific moisture requirement that determines its shelf-life and organoleptic properties. Hence, WVP properties play a crucial role in food packaging as it determines the amount of moisture that spreads through the packaging film. The moisture barrier property prevents to gain or loss of moisture that can deteriorate the food quality of dry or fresh products (Priyadarshi et al., Citation2018).
BC composite samples combined with glycerol and chitosan showed WVP values ranging between 2.38 × 10−11 and 1.21 × 10−10 g/m·s·Pa (). Data fitted well to a quadratic equation. The Box-Cox plot recommended transforming the data to inverse of WVP to get a better fit of the equation. The model was significant with an F-value of 60.78 and a p-value of 0.0033 (). In the WVP model, the linear and quadratic effects of glycerol concentration terms were significant. The chitosan concentration (p-value of 0.6247) and the interaction between glycerol and chitosan (p-value of 0.1284) were no significant. The linear effect of the glycerol (F-value of 260.60) had a higher effect than the quadratic effect of the glycerol (F-value of 29.95) on the WVP response. The value of r2 was 0.99 and the predicted r2 value (0.88) was in reasonable agreement with adjusted r2 (0.97). The adequate precision value (19.71) implying an adequate signal ().
Table 2. Analysis of variance (ANOVA) for water vapor permeability (WVP), water retention (%W) and water solubility (%S) properties.
Tabla 2. Análisis de varianza (ANOVA) para las propiedades de permeabilidad al vapor de agua (WVP), retención de agua (% W) y solubilidad en agua (% S)
Table 3. Fit statistics of the obtained model for each dependent variable: moisture content, tensile properties (water vapor permeability (WVP), water retention capacity (%W) and solubility properties (%S).
Tabla 3. Estadísticos de ajuste del modelo obtenido para cada variable dependiente: contenido de humedad, propiedades de tracción (permeabilidad al vapor de agua (WVP), capacidad de retención de agua (% W) y propiedades de solubilidad (% S)
EquationEquation 1(1)
(1) forecasts the response of WVP as a function of the glycerol and chitosan concentration of the BC composite samples.
The response surface plot illustrates the variation in the WVP response based on the film composition (). WVP data signaled that chitosan and glycerol increased the permeability values, being higher due to the presence of glycerol and the interaction glycerol-chitosan. The presence of chitosan resulted in a slightly increase of the permeability from 2.38 × 10−11 to 2.79 × 10−11 g/m·s·Pa. As mentioned earlier, chitosan, as other hydrophilic polymer, generally increases the WVP values. Chitosan on BC films produced a slight increase in the water molecules diffusion through the polymer matrix. In this case, the reduced porosity of the matrix probably had a greater impact on the WVP than the presence of chitosan. The WVP value of pure BC samples suggested that the porosity matrix eased the water vapor diffusion through the polymeric structure. Chitosan filled the empty voids in the BC matrix, diminishing the porosity and hindering the water molecules diffusion. In addition, the physical interactions between BC and chitosan decreased the available active groups to interact with water (Lin et al., Citation2013).
Figure 3. Response surface graph of the predicted effect of glycerol and chitosan concentration on (a) water vapor permeability (WVP), (b) solubility (%S) and (c) water retention capacity properties of bacterial cellulose films.
Figura 3. Superficie de respuesta del efecto predicho de la concentración de glicerol y quitosano sobre (a) permeabilidad al vapor de agua (WVP), (b) solubilidad (% S) y (c) propiedades de capacidad de retención de agua de películas de celulosa bacteriana
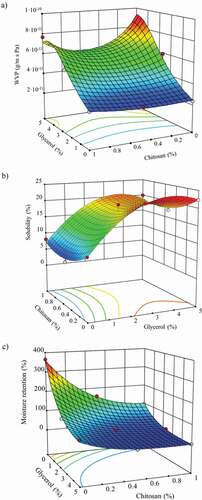
On the other hand, glycerol manifested a significant effect on WVP, promoting a sharp increase of the permeability values from 2.38 × 10−11 to 8.95 × 10−11 g/m·s·Pa. The observed WVP modifications were mainly due to two issues. First, the hygroscopic nature of glycerol with a high amount of free hydroxyl groups increased the moisture adsorption capability, leading to enhanced WVP. Glycerol also interacted with the BC polymer chains and BC–chitosan chains, forming new interactions. The polymers-glycerol interactions increased the intramolecular distance in the structure, making it less dense. The reorganization and increased free volume of the polymer matrix eased the water molecules diffusion. Permeability properties of chitosan-glycerol films and BC films with polyvinyl alcohol, glycerol or polyethylene glycol revealed similar behavior (Cazon et al., Citation2020c; Priyadarshi et al., Citation2018; Sun et al., Citation2018).
3.3. Evaluation of the water solubility and water retention properties
The %S values of the film components after immersing in water for 24 h ranged from 4.53% to 22.35% (). Data were fitted using a quadratic mathematical model. The Box-Cox plot recommended transforming the %S data to a square root of the %S values to better fit. The F-value was 19.20 implying that the model was significant. There was only a 1.74% (p-value was 0.0174) chance that an F-value this large could occur due to noise. The p-values of the %S model terms indicated that only the linear and quadratic effects of glycerol were significant terms (). The linear effect of glycerol content had a higher impact (F-value was 66.08) on the %S response than the quadratic effect of the glycerol content (F-value was 22.39).
The r2 value was 0.97 and the predicted r2 (0.73) was in reasonable agreement with the adjusted r2 (0.92). The adequate precision ratio indicated an adequate signal, being higher than 4 (10.98) as shown in .
EquationEquation 2(2)
(2) forecasts the %S value of the developed films as a function of the glycerol and chitosan content.
shows the response surface plot obtained. During the dissolution process, the water molecules are first adsorbed by the polymer matrix, interacting with the polymer chains, swelling the structure and increasing the free volume. This phenomenon promotes the releasing of the components of the matrix after prolonged exposure and initiating the solubilization of the film (Priyadarshi et al., Citation2018). Due to the strong affinity and water-soluble properties of glycerol, a higher percentage of solubilization was observed in BC–glycerol films, reaching the maximum value of 22.35%. No significant variation was observed as a function of glycerol concentration, reaching similar values of %S.
BC–chitosan samples showed lower %S values because chitosan requires a slightly acid medium to be dissolved. Chitosan was retained into the BC matrix thanks to the stronger BC-chitosan interactions.
The presence of chitosan on BC–glycerol samples diminished the %S values. Probably, the increase of the interactions among the components given by the cross-linking between the amine and hydroxyl groups limited the interactions with the solvent. Results were in agreement with those obtained in previous work (Cazon et al., Citation2019a).
%W properties were evaluated to determine their potential applications such as adsorbent pads or to predict their behavior when in contact with fresh food with high water content or with a dehydrated product. %W data after immersion in water for 24 h ranged from 7.79% to 364.78% (). %W data were fitted using a quadratic model. The Box-Cox plot recommended using the square root of %W values to obtain a better fit. The obtained model was significant with an F-value of 135.83 and p-value of 0.0010. In this model, the linear and quadratic effects of glycerol concentration and chitosan concentration were significant terms (p < .05). According to the F-values, the linear effect of glycerol and chitosan concentration showed higher impact on the response than the quadratic effect of the concentration of the components (). The r2 value was 0.99 and the predicted r2 (0.95) was in reasonable agreement with the adjusted r2 (0.99) because the difference was less than 0.2. The ratio of 34.78 (adequate precision) indicated an adequate signal ().
EquationEquation 3(3)
(3) was used to estimate the response value of %W as function of the glycerol and chitosan content on the BC composite films.
illustrates the response surface plot of the %W of BC–glycerol–chitosan films. Pure BC films had a great %W derived from its 3D porosity structure with high surface area to interact with water molecules. The porosity reduction of the matrix due to the presence of chitosan and the interactions polymer–polymer diminished drastically the available free active sites for interacting with water molecules, resulting in a reduction of the swelling degree values.
Regarding glycerol, the strong reduction effect on the water retention capacity was motivated by two key factors. First, interactions between glycerol and BC reduced the number of free hydroxyl groups that could interact with water molecules. Second, and probably more important, it was the strong hygroscopic character of glycerol. The strong interactions between glycerol and the surrounding humidity led to high equilibrium moisture content values, as discussed in Part 1. During the conditioning stage in silica gel desiccators, due to the strong water–glycerol interactions, it was not possible to remove the moisture from the samples. The high moisture content of the samples and in the reduced free groups resulted in low water adsorption values. The results obtained were in agreement with behaviors previously observed in polysaccharide-based films with glycerol (Cielecka et al., Citation2019; Priyadarshi et al., Citation2018; Thakhiew et al., Citation2010; Lin et al., Citation2013).
3.4. Evaluation of the optical properties
The optical properties of the developed films were evaluated by UV-barrier, transparency, opacity, and color properties. These optical parameters were calculated from the transmittance values of the samples in the UV-Vis light range.
shows the transmittance spectrum of the BC–chitosan–glycerol samples from experiments 5, 6, 8, and 9 obtained in the UV range between 190 and 400 nm. shows the transmittance ranges of the indicated samples. The lower transmittance values ranged from 1.65% to 5.15% in the UV-A region, from 0.61% to 1.17% in the UV-B region and from 0.17% to 0.35% in the UV-C region. BC–glycerol (2.5%)–chitosan (5.0%) showed the lowest transmittance value in the UV region. The increase of the glycerol content slightly increased the transmittance values, probably promoted by the plasticizing effect and the associated structure modifications (Bertuzzi et al., Citation2007; Litjens et al., Citation1999; Quickenden & Irvin, Citation1980).
Table 4. Optical properties of the bacterial cellulose-glycerol-chitosan films. The average percentage of transmittance (%T) in the region UV-A (315–400 nm), UV-B (280–315 nm) and UV-C (200–280 nm), transparency and opacity calculated values of the selected experiments, and thickness values.
Tabla 4. Propiedades ópticas de las películas bacterianas de celulosa-glicerol-quitosana. El porcentaje medio de transmitancia (% T) en la región UV-A (315–400 nm), UV-B (280–315 nm) y UV-C (200–280 nm), valores calculados de transparencia y opacidad de los experimentos seleccionados, y valores de espesor
Figure 4. UV-VIS spectra profile and FT-IR spectra profile of the selected bacterial cellulose–glycerol–chitosan samples.
Figura 4. Perfil de espectros UV-VIS y perfil de espectros FT-IR de las muestras bacterianas de celulosa–glicerol–quitosano seleccionadas
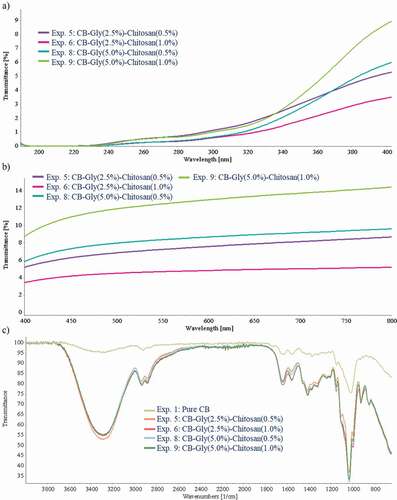
In a previous study, the transmittance values of pure BC in the UV-light region were 6.05%, 3.30% and 1.00% in the UV-A, UV-B and UV-C regions, respectively (Cazon et al., Citation2019a). The low transmittance values in the UV-light region shown by pure BC samples were further lowered by adding glycerol and chitosan. In this way, it was possible to obtain cellulose-based films with improved UV-light barrier properties, with average values below 5.5%. This fact opens multiple possibilities for applications in foods and products sensitive to sunlight radiation.
Transparency and opacity properties are also important parameters determining the viability of the biopolymer as a food packaging material. It is desirable to have high transparency and low opacity values to increase the chances of consumer acceptance of a new biomaterial. shows transparency values ranging between 26.49 and 37.50, and the opacity values ranged from 30.92 to 52.28. These values were calculated from the transmittance data in the Vis range (). The increase of glycerol and chitosan content increased the transparency and decreased the opacity. The BC–glycerol–chitosan interactions allowed improving the visual appearance of the composite films. The values obtained were slightly lower than the values of pure chitosan (Rao et al., Citation2010), but BC–glycerol–chitosan showed better transparency and opacity values than other biopolymer-based samples, such as chitosan with essential oils, chitosan-gelatin or chitosan-guar gum films, among other examples (Ahmed & Ikram, Citation2016; Rao et al., Citation2010).
The color parameters (L*, a* and b*) are shown in . Following a similar trend than the transparency and opacity values, when the chitosan and glycerol were added, the lightness (L*) and the yellowish (b*) increased meanwhile the greenish (a*) decreased. The color changed due to the nature of the polymers and probably due to the polymers-plasticizing interactions.
Table 5. Color properties of the selected experiments of the blend bacterial cellulose-glycerol-chitosan films.
Tabla 5. Propiedades de color de los experimentos seleccionados de la mezcla de películas de celulosa bacteriana-glicerol-quitosano
3.5. Fourier transform infrared spectroscopy (FT-IR)
FT-IR spectra obtained from pure BC and BC–glycerol–chitosan at several concentrations are shown in . FT-IR spectra showed a broad characteristic peak in the 3000–3600 cm−1 range, assigned to the -OH stretching vibrations. Comparing the pure BC spectrum with the spectra of the composite samples, a displacement of the peak was observed at slightly lower wavelengths accompanied by a significant increase in energy adsorption (decreasing the %T values). The sharper peaks and the increased adsorbed energy is caused by the increase in –OH groups due to the presence of chitosan and glycerol, the formation of new hydrogen bonds and the –NH group stretching vibration in this region (Priyadarshi et al., Citation2018; Ul-Islam et al., Citation2011). The adsorption peak at 1650 cm−1 is assigned to the –CO group and the peak at 1420 and 1370 cm−1 are assigned to the –CH asymmetric deformation and –CH bending vibration, respectively, for samples with chitosan (Ul-Islam et al., Citation2011). Another intense peak at 1050 cm−1 was assigned to C–O–C pyranose ring stretching vibration. Note that the presence of glycerol and chitosan caused a shift in wavelength and an increase in energy adsorption, indicating polymeric association by physical interactions (Lin et al., Citation2013).
3.6. Thermal properties (TGA-DSC)
Thermal properties are important to determine the viability of a certain biopolymer blend in the food industry. Their assessment allows evaluating the suitability of the developed biomaterial to be applied on food that may be subjected to intensive heat treatments (Moradi & Kalanpour, Citation2019).
The thermal stability of the BC–glycerol–chitosan composite films was assessed by simultaneous TGA-DSC. shows the thermogram of experiments 5, 6, 8, and 9 that allowed analyzing the effect of the interactions BC–glycerol–chitosan on the thermal stability of the developed films.
Figure 5. Thermogravimetry and differential scanning calorimetry of the selected bacterial cellulose–glycerol–chitosan samples.
Figura 5. Termogravimetría y calorimetría diferencial de barrido de las muestras bacterianas de celulosa–glicerol–quitosano seleccionadas
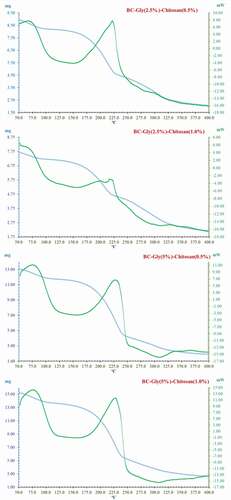
The endothermic peak, common to the four thermograms in the 50–110°C range, is associated with the loss of adsorbed water (Priyadarshi et al., Citation2018; Sun et al., Citation2018). The volatilization of water resulted in a weight loss of 8.66%, 6.74%, 9.93%, and 10.2% for samples from experiments 5, 6, 8, and 9, respectively. The loss of weight until 110°C increased by the increase of the glycerol content, due to its hydrophilic nature. However, these values were far from the values obtained in the analysis of moisture content. The difference between the values could be motivated by several factors. During the conditioning step at 30°C, the samples with glycerol had water molecules condensed on the surface, due to the hygroscopicity character of the glycerol that strongly retained the adsorbed moisture at standard RH conditions (Cazon et al., Citation2020c). Part of the water could have been evaporated before carrying out the FT-IR analysis and until the analysis starting temperature was reached (50°C). Besides, part of the water could volatilize at higher temperatures induced by the strong water–glycerol interactions.
The second endothermic peak was found at 170–230°C, corresponding to the degradation onset temperature of glycerol (Cazon et al., Citation2020a; Sun et al., Citation2018). At 230°C, the total weight losses of the samples were 48.36%, 40.13%, 52.77%, and 53.21% for experiments 5, 6, 8, and 9, respectively. Results indicated that the increase of chitosan content improved the thermal stability properties of the samples, diminishing the weight loss of the samples at lower glycerol content. The chitosan–glycerol and BC–chitosan interactions caused a shift of the polymer degradation temperature to slightly higher temperature values, as shown in .
The third region ranged between 230°C and 350°C. The weight losses were associated with the degradation of chitosan and cellulose. Previous work indicated that the onset temperature of the thermal degradation of BC is 250°C (Yan et al., Citation2017). At 350°C, the calculated weight loss values were 73.68%, 67,88%, 82.99%, and 80.12% for experiments 5, 6, 8 and 9, respectively. In agreement with that behavior, the increase of chitosan content improved the thermal stability of the composite film thanks to the increase of the physical interactions among the components. Probably, the higher weight losses observed implied the simultaneous degradation of the polymers and possible plasticizing remains.
The TGA-DSC results are consistent with the results observed in literature (Cazon et al., Citation2019a; Fernandes et al., Citation2010; Xiao et al., Citation2003). The thermal stability results indicated that the developed BC composite films are stable at a temperature below 135°C. Hence, it would be possible to apply this biodegradable blend material in food products that undergo pasteurization or sterilization processes (Robertson, Citation2012).
4. Conclusions
Chitosan and glycerol penetrated and diffused into the cellulose matrix by immersion in baths as shown by SEM images and FT-IR spectra. Glycerol coated the BC fibers resulting in a plasticizing effect, as indicated by the WVP results. Chitosan promoted the formation of a connected internal network resulting in a reinforcing effect of the film, decreasing its porosity which allowed obtaining films with slightly lower WVP values. The addition of glycerol and chitosan allowed obtaining films with excellent UV-light barrier properties and lower water retention capacity. Hence, these properties would facilitate the application of BC–glycerol–chitosan films on fresh food, with high moisture content and susceptible to oxidation by the sunlight radiation. In addition, the thermal properties of the films analyzed by TGA-DSC demonstrated the viability of these films to be applied in heat-treated foods at temperatures until 135°C.
Acknowledgments
A grant to author Patricia Cazón from CONACYT (Mexico) is gratefully acknowledged. We acknowledge the use of RIAIDT-USC analytical facilities.
Disclosure statement
The authors declare that they have no conflicts of interest.
Additional information
Funding
References
- Ahmed, S., & Ikram, S. (2016). Chitosan and gelatin based biodegradable packaging films with UV-light protection. Journal of Photochemistry and Photobiology B: Biology, 163, 115–124. https://doi.org/https://doi.org/10.1016/j.jphotobiol.2016.08.023
- Aider, M. (2010). Chitosan application for active bio-based films production and potential in the food industry: Review. LWT - Food Science and Technology, 43(6), 837–842. https://doi.org/https://doi.org/10.1016/j.lwt.2010.01.021
- Angtika, R. S., Widiyanti, P., & Aminatun. (2018). Bacterial cellulose-chitosan-glycerol biocomposite as artificial dura mater candidates for head trauma. Journal of Biomimetics, Biomaterials and Biomedical Engineering, 36, 7–16. https://doi.org/https://doi.org/10.4028/www.scientific.net/JBBBE.36.7
- Bertuzzi, M. A., Castro Vidaurre, E. F., Armada, M., & Gottifredi, J. C. (2007). Water vapor permeability of edible starch based films. Journal of Food Engineering, 80(3), 972–978. https://doi.org/https://doi.org/10.1016/j.jfoodeng.2006.07.016
- Cazón, P., & Vázquez, M. (2020a). Bacterial cellulose as a biodegradable food packaging material: A review. Food Hydrocolloids, 113, 106530. https://doi.org/https://doi.org/10.1016/j.foodhyd.2020.106530
- Cazón, P., & Vázquez, M. (2020b). Mechanical and barrier properties of chitosan combined with other components as food packaging film. Environmental Chemistry Letters, 8(2), 257–267. Springer Verlag https://doi.org/https://doi.org/10.1007/s10311-019-00936-3
- Cazón, P., & Vázquez, M. (2020c). Improving bacterial cellulose films by ex-situ and in-situ modifications: A review. Food Hydrocolloids, 113, 106514. https://doi.org/https://doi.org/10.1016/j.foodhyd.2020.106514
- Cazon, P., Vazquez, M., & Velazquez, G. (2018). Novel composite films based on cellulose reinforced with chitosan and polyvinyl alcohol: Effect on mechanical properties and water vapour permeability. Polymer Testing, 69(June), 536–544. https://doi.org/https://doi.org/10.1016/j.polymertesting.2018.06.016
- Cazon, P., Vázquez, M., & Velazquez, G. (2019a). Composite films with UV-Barrier properties based on bacterial cellulose combined with Chitosan and Poly(vinyl alcohol): Study of puncture and water interaction properties. Biomacromolecules, 20(5), 2084–2095. https://doi.org/https://doi.org/10.1021/acs.biomac.9b00317
- Cazon, P., Vázquez, M., & Velazquez, G. (2019b). Composite films with UV-barrier properties of bacterial cellulose with glycerol and poly(vinyl alcohol): Puncture properties, solubility, and swelling degree. Biomacromolecules, 20(8), 3115–3125. https://doi.org/https://doi.org/10.1021/acs.biomac.9b00704
- Cazon, P., Vázquez, M., & Velazquez, G. (2019c). Environmentally friendly films combining bacterial cellulose, chitosan, and polyvinyl alcohol: Effect of water activity on barrier, mechanical, and optical properties. Biomacromolecules, 21(2), 753–760. https://doi.org/https://doi.org/10.1021/acs.biomac.9b01457
- Cazon, P., Vázquez, M., & Velázquez, G. (2020). Regenerated cellulose films with chitosan and polyvinyl alcohol: Effect of the moisture content on the barrier, mechanical and optical properties. Carbohydrate Polymers, 236, 116031. https://doi.org/https://doi.org/10.1016/j.carbpol.2020.116031
- Cazon, P., Velázquez, G., & Vázquez, M. (2019). Characterization of bacterial cellulose films combined with chitosan and polyvinyl alcohol: Evaluation of mechanical and barrier properties. Carbohydrate Polymers, 216, 72–85. https://doi.org/https://doi.org/10.1016/j.carbpol.2019.03.093
- Cazon, P., Velázquez, G., & Vázquez, M. (2020a). Characterization of mechanical and barrier properties of bacterial cellulose, glycerol and polyvinyl alcohol (PVOH) composite films with eco-friendly UV-protective properties. Food Hydrocolloids, 99, 105323. https://doi.org/https://doi.org/10.1016/j.foodhyd.2019.105323
- Cazon, P., Velázquez, G., & Vázquez, M. (2020b). Regenerated cellulose films combined with glycerol and polyvinyl alcohol: Effect of moisture content on the physical properties. Food Hydrocolloids, 103, 105657. https://doi.org/https://doi.org/10.1016/j.foodhyd.2020.105657
- Cazon, P., Velázquez, G., & Vázquez, M. (2020c). UV-protecting films based on bacterial cellulose, glycerol and polyvinyl alcohol: Effect of water activity on barrier, mechanical and optical properties. Cellulose, 27(14), 8199–8213. https://doi.org/https://doi.org/10.1007/s10570-020-03346-9
- Cielecka, I., Szustak, M., Kalinowska, H., Gendaszewska-Darmach, E., Ryngajłło, M., Maniukiewicz, W., & Bielecki, S. (2019). Glycerol-plasticized bacterial nanocellulose-based composites with enhanced flexibility and liquid sorption capacity. Cellulose, 26(9), 5409–5426. https://doi.org/https://doi.org/10.1007/s10570-019-02501-1
- Cook, R. D. (1977). Detection of influential observations in linear regression. Technometrics, 19(1), 15–18. https://doi.org/https://doi.org/10.2307/1268249
- Fernandes, S. C. M., Freire, C. S. R., Silvestre, A. J. D., Pascoal Neto, C., Gandini, A., Berglund, L. A., & Salmén, L. (2010). Transparent chitosan films reinforced with a high content of nanofibrillated cellulose. Carbohydrate Polymers, 81(2), 394–401. https://doi.org/https://doi.org/10.1016/j.carbpol.2010.02.037
- Janjarasskul, T., & Suppakul, P. (2018). Active and intelligent packaging: The indication of quality and safety. Critical Reviews in Food Science and Nutrition, 58(5), 808–831. https://doi.org/https://doi.org/10.1080/10408398.2016.1225278
- Khan, M. R., Sadiq, M. B., & Mehmood, Z. (2020). Development of edible gelatin composite films enriched with polyphenol loaded nanoemulsions as chicken meat packaging material. CYTA - Journal of Food, 18(1), 137–146. https://doi.org/https://doi.org/10.1080/19476337.2020.1720826
- Khemir, M., Besbes, N., Khemis, I. B., Di Bella, C., Lo Monaco, D., & Sadok, S. (2020). Determination of shelf-life of vacuum-packed sea bream (Sparus aurata) fillets using chitosan-microparticles-coating. CYTA - Journal of Food, 18(1), 51–60. https://doi.org/https://doi.org/10.1080/19476337.2019.1696893
- Kim, J., Cai, Z., Lee, H. S., Choi, G. S., Lee, D. H., & Jo, C. (2011). Preparation and characterization of a Bacterial cellulose/Chitosan composite for potential biomedical application. Journal of Polymer Research, 18(4), 739–744. https://doi.org/https://doi.org/10.1007/s10965-010-9470-9
- Lin, D., Liu, Z., Shen, R., Chen, S., & Yang, X. (2020). Bacterial cellulose in food industry: Current research and future prospects. International Journal of Biological Macromolecules, 158, 1007–1019. https://doi.org/https://doi.org/10.1016/j.ijbiomac.2020.04.230
- Lin, W.-C., Lien, -C.-C., Yeh, H.-J., Yu, C.-M., & Hsu, S. (2013). Bacterial cellulose and bacterial cellulose–chitosan membranes for wound dressing applications. Carbohydrate Polymers, 94(1), 603–611. https://doi.org/https://doi.org/10.1016/j.carbpol.2013.01.076
- Litjens, R. A. J., Quickenden, T. I., & Freeman, C. G. (1999). Visible and near-ultraviolet absorption spectrum of liquid water. Applied Optics, 38(7), 1216–1223. https://doi.org/https://doi.org/10.1364/AO.38.001216
- Mohammadkazemi, F., Azin, M., & Ashori, A. (2015). Production of bacterial cellulose using different carbon sources and culture media. Carbohydrate Polymers, 117, 518–523. https://doi.org/https://doi.org/10.1016/j.carbpol.2014.10.008
- Montilla, A., Ruiz-Matute, A. I., & Corzo, N. (2013). Biological effects and extraction processes used to obtain marine chitosan. In Blanca Hernández‐Ledesma & Miguel Herrero (Eds.), Bioactive compounds from marine foods (pp. 193–217). John Wiley & Sons, Ltd.https://doi.org/https://doi.org/10.1002/9781118412893.ch10
- Moradi, Z., & Kalanpour, N. (2019). Kefiran, a branched polysaccharide: Preparation, properties and applications: A review. Carbohydrate Polymers, 223(March), 115100. https://doi.org/https://doi.org/10.1016/j.carbpol.2019.115100
- Morin-Crini, N., Lichtfouse, E., Torri, G., & Crini, G. (2019). Applications of chitosan in food, pharmaceuticals, medicine, cosmetics, agriculture, textiles, pulp and paper, biotechnology, and environmental chemistry. Environmental Chemistry Letters, 17, 1667–1692. https://doi.org/https://doi.org/10.1007/s10311-019-00904-x
- Mortensen, A., Aguilar, F., Crebelli, R., Di Domenico, A., Dusemund, B., Frutos, M. J., Galtier, P., Gott, D., Gundert-Remy, U., Leblanc, J.-C., Lindtner, O., Moldeus, P., Mosesso, P., Parent-Massin, D., Oskarsson, A., Stankovic, I., Waalkens-Berendsen, I., Woutersen, R. A., & Lambré, C., & (ANS), E. P. on F. A. and N. S. added to F.. (2017). Re-evaluation of glycerol (E 422) as a food additive. EFSA Journal, 15(3), e04720. https://doi.org/https://doi.org/10.2903/j.efsa.2017.4720
- Napper, I. E., & Thompson, R. C. (2019). Chapter 22 - Marine Plastic Pollution: Other Than Microplastic. In T. M. Letcher & D. A. Vallero (Eds.), Waste (2nd ed., pp. 425–442). Academic Press. https://doi.org/https://doi.org/10.1016/B978-0-12-815060-3.00022-0
- Ozdemir, M., & Floros, J. D. (2004). Active food packaging technologies. Critical Reviews in Food Science and Nutrition, 44(3), 185–193. https://doi.org/https://doi.org/10.1080/10408690490441578
- Pang, M., Cao, L., Cao, L., Sheb, Y., & Wang, H. (2019). Properties of nisin incorporated ZrO2/poly (vinyl alcohol) - wheat gluten antimicrobial barrier films. CyTA - Journal of Food, 17(1), 400–407. https://doi.org/https://doi.org/10.1080/19476337.2019.1587517
- Priyadarshi, R., Sauraj, Kumar, B., & Negi, Y. S. (2018). Chitosan films incorporated with citric acid and glycerol as an active packaging material for extension of green chilli shelf life. Carbohydrate Polymers, 195(December 2017), 329–338. https://doi.org/https://doi.org/10.1016/j.carbpol.2018.04.089
- Quickenden, T. I., & Irvin, J. A. (1980). The ultraviolet absorption spectrum of liquid water. The Journal of Chemical Physics, 72(8), 4416–4428. https://doi.org/https://doi.org/10.1063/1.439733
- Rabea, E. I., Badawy, M. E. T., Stevens, C. V., Smagghe, G., & Steurbaut, W. (2003). Chitosan as antimicrobial agent: Applications and mode of action. Biomacromolecules, 4(6), 1457–1465. https://doi.org/https://doi.org/10.1021/bm034130m
- Rao, M. S., Kanatt, S. R., Chawla, S. P., & Sharma, A. (2010). Chitosan and guar gum composite films: Preparation, physical, mechanical and antimicrobial properties. Carbohydrate Polymers, 82(4), 1243–1247. https://doi.org/https://doi.org/10.1016/j.carbpol.2010.06.058
- Reiniati, I., Hrymak, A. N., & Margaritis, A. (2017). Recent developments in the production and applications of bacterial cellulose fibers and nanocrystals. Critical Reviews in Biotechnology, 37(4), 510–524. https://doi.org/https://doi.org/10.1080/07388551.2016.1189871
- Robertson, G. L. (2012). Food Packaging. Principles and practice (3rd ed.). CRC Press. https://doi.org/https://doi.org/10.1201/b21347
- Ruka, D. R., Simon, G. P., & Dean, K. M. (2012). Altering the growth conditions of Gluconacetobacter xylinus to maximize the yield of bacterial cellulose. Carbohydrate Polymers, 89(2), 613–622. https://doi.org/https://doi.org/10.1016/j.carbpol.2012.03.059
- Sakia, R. M. (1992). The Box-Cox Transformation Technique: A Review. The Statistician, 41(2), 169–178. https://doi.org/https://doi.org/10.2307/2348250
- Sirviö, J. A., Visanko, M., Ukkola, J., & Liimatainen, H. (2018). Effect of plasticizers on the mechanical and thermomechanical properties of cellulose-based biocomposite films. Industrial Crops and Products, 122, 513–521. https://doi.org/https://doi.org/10.1016/j.indcrop.2018.06.039
- Stumpf, T. R., Yang, X., Zhang, J., & Cao, X. (2018). In situ and ex situ modifications of bacterial cellulose for applications in tissue engineering. Materials Science and Engineering: C, 82, 372–383. https://doi.org/https://doi.org/10.1016/j.msec.2016.11.121
- Sun, Y., Meng, C., Zheng, Y., Xie, Y., He, W., Wang, Y., Qiao, K., & Yue, L. (2018). The effects of two biocompatible plasticizers on the performance of dry bacterial cellulose membrane: A comparative study. Cellulose, 25(10), 5893–5908. https://doi.org/https://doi.org/10.1007/s10570-018-1968-z
- Szymańska-Chargot, M., Chylińska, M., Cybulska, J., Kozioł, A., Pieczywek, P. M., & Zdunek, A. (2017). Simultaneous influence of pectin and xyloglucan on structure and mechanical properties of bacterial cellulose composites. Carbohydrate Polymers, 174, 970–979. https://doi.org/https://doi.org/10.1016/j.carbpol.2017.07.004
- Thakhiew, W., Devahastin, S., & Soponronnarit, S. (2010). Effects of drying methods and plasticizer concentration on some physical and mechanical properties of edible chitosan films. Journal of Food Engineering, 99(2), 216–224. https://doi.org/https://doi.org/10.1016/j.jfoodeng.2010.02.025
- Ul-Islam, M., Shah, N., Ha, J. H., & Park, J. K. (2011). Effect of chitosan penetration on physico-chemical and mechanical properties of bacterial cellulose. Korean Journal of Chemical Engineering, 28(8), 1736–1743. https://doi.org/https://doi.org/10.1007/s11814-011-0042-4
- Ullah, H., Wahid, F., Santos, H. A., & Khan, T. (2016). Advances in biomedical and pharmaceutical applications of functional bacterial cellulose-based nanocomposites. In Carbohydrate Polymers (Vol. 150, pp. 330–352). Elsevier Ltd. https://doi.org/https://doi.org/10.1016/j.carbpol.2016.05.029
- Vilela, C., Pinto, R. J. B., Coelho, J., Domingues, M. R. M., Daina, S., Sadocco, P., Santos, S. A. O., & Freire, C. S. R. (2017). Bioactive chitosan/ellagic acid films with UV-light protection for active food packaging. Food Hydrocolloids, 73, 120–128. https://doi.org/https://doi.org/10.1016/j.foodhyd.2017.06.037
- Wyrwa, J., & Barska, A. (2017). Innovations in the food packaging market: Active packaging. European Food Research and Technology, 243(10), 1681–1692. https://doi.org/https://doi.org/10.1007/s00217-017-2878-2
- Xiao, C., Zhang, Z., Zhang, J., Lu, Y., & Zhang, L. (2003). Properties of regenerated cellulose films plasticized with α-monoglycerides. Journal of Applied Polymer Science, 89(13), 3500–3505. https://doi.org/https://doi.org/10.1002/app.12509
- Yamada, Y., Yukphan, P., Lan Vu, H. T., Muramatsu, Y., Ochaikul, D., Tanasupawat, S., & Nakagawa, Y. (2012). Description of Komagataeibacter gen. nov., with proposals of new combinations (Acetobacteraceae). The Journal of General and Applied Microbiology, 58(5), 397–404. https://doi.org/https://doi.org/10.2323/jgam.58.397
- Yan, H., Chen, X., Song, H., Li, J., Feng, Y., Shi, Z., Wang, X., & Lin, Q. (2017). Synthesis of bacterial cellulose and bacterial cellulose nanocrystals for their applications in the stabilization of olive oil pickering emulsion. Food Hydrocolloids, 72, 127–135. https://doi.org/https://doi.org/10.1016/j.foodhyd.2017.05.044