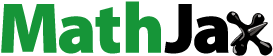
ABSTRACT
Bioactive peptides have been extensively applied for developing health-promoting foods. To improve the value of Laminaria japonica proteins (LJPs), anti-hypertensive peptides were prepared by using controlled enzymatic hydrolysis along with response surface methodology to improve their yields. Eight anti-hypertensive peptides with tyrosine (Tyr) at the C-terminal were determined through an efficient RP-HPLC method and molecular docking was performed to reveal the underlying mechanisms behind their effectiveness of anti-hypertension. According to the results, the strongest activity of hydrolysates was achieved under enzyme ratio (papain: alcalase: trypsin) 1:2:1, pH 8.0, temperature 60°C, substrate concentration 1.7%, enzyme addition 4.4%, and hydrolysis time 5.1 h. Furthermore, molecular docking results displayed that anti-hypertensive peptides could bind with ACE active sites through forming a stable composite structure, thus causing a significant reduction to activities. These results demonstrated the potential applications of LJPs as a source of anti-hypertensive peptides and provided a reference for the industrialized anti-hypertensive productions.
RESUMEN
Los péptidos bioactivos han sido aplicados ampliamente para preparar alimentos que promueven la salud. A fin de mejorar el valor de las proteínas de Laminaria japonica (LJP), en este estudio se prepararon péptidos antihipertensivos utilizando hidrólisis enzimática controlada junto con una metodología de superficie de respuesta destinada a mejorar su rendimiento. Mediante la aplicación de un método eficiente de RP-HPLC, estos procedimientos permitieron identificar ocho péptidos antihipertensivos con tirosina (Tyr) en el extremo C terminal, realizándose un acoplamiento molecular para revelar los mecanismos subyacentes a su eficacia antihipertensiva. De acuerdo con los resultados, la mayor actividad de los hidrolizados se alcanzó con una proporción de enzimas (papaína: alcalasa: tripsina) de 1:2:1, pH 8.0, temperatura de 60°C, concentración de sustrato del 1.7%, adición de enzimas de 4.4% y tiempo de hidrólisis de 5.1 horas. Por otra parte, los resultados de acoplamiento molecular mostraron que los péptidos antihipertensivos pueden unirse a los sitios activos de la ECA mediante la formación de una estructura compuesta estable, lo que provoca una reducción significativa de actividades. Estos resultados dan cuenta de las aplicaciones potenciales de los LJP como fuente de péptidos antihipertensivos y proporcionan una referencia para la producción de antihipertensivos industrializados.
1. Introduction
As a sort of common but serious chronic disease, hypertension is associated with the high risks of cerebrovascular, cardiovascular and renal complications, affecting approximately 25% of adults worldwide at present time and this portion is estimated to 60% by 2025 (García-Mora et al., Citation2017; Ngo et al., Citation2015; Yang et al., Citation2019). Therefore, it is imperative to carry out the prevention and treatment of hypertension.
Angiotensin I-converting enzyme (ACE), a zinc protease, plays a crucial role in regulating blood pressure for its ability of converting angiotensin I to the potent vasoconstrictor angiotensin II in the renin-angiotensin system and the deactivation of the vasodilator bradykinin in the kallikrein-kinin system (Cao et al., Citation2020; Ko et al., Citation2012). The application of ACE inhibitors has been accepted as one of the major therapeutic approaches to reducing hypertension (Wijesekara & Kim, Citation2010). Currently, the detection and identification of ACE inhibitors from foods has attracted increasing attention for safety concerns (Admassu et al., Citation2018; Ko et al., Citation2012). Anti-hypertensive peptides with strong ACE inhibitory activity have been reported to be derived from animals (Kocak et al., Citation2020), fruits (Memarpoor-Yazdi et al., Citation2020), vegetables (Patten et al., Citation2016), eumycetes (Amorim et al., Citation2019) and other marine sources (Pujiastuti et al., Citation2019). Besides, there have been various studies conducted on their activities (García-Mora et al., Citation2017; Majumder & Wu, Citation2015; Raji et al., Citation2020). However, there are still few studies focusing on the anti-hypertensive peptides derived from Laminaria japonica proteins (LJPs).
According to the statistics of global Laminaria japonica production, there were nearly 90% of Laminaria japonica produced in China and the volume of its production exceed 1.5 million tons in 2018, which made it the vitally important marine cash crop (DW, Fishery Bureau of Ministry of agriculture and Rural Affairs of China, Citation2019). However, for the limited processing levels, LJPs are routinely discarded as a sort of by-product, which cause a huge amount of waste and the eutrophication of sea water, or be processed as low-value feed (Carboni et al., Citation2016). Thus, an industrial hydrolysis of LJPs has become an imperative study to derive more anti-hypertensive peptides and improve the utilization value of LJPs. But there is yet to be any efficient solution to improving the yield of bioactive peptides derived from LJPs. On the basis of previous researches, it can be known that the bioactivities of peptides are closely associated with the composition and sequence of their amino acids, especially those peptides comprise 2–20 amino acids, which are regulated according to the type of hydrolases and the hydrolysis conditions (Gu et al., Citation2011; Kristinsson & Rasco, Citation2000; Meisel & FitzGerald, Citation2003). Alcalase tends to hydrolyze the bonds of C-terminal peptide in non-charged side-chain amino acids at a fast pace, such as Phe, Tyr, etc., thus forming the hydrophobic amino acids with strong ACE inhibitory activity at C-terminal (Alashi et al., Citation2014). Trypsin can hydrolyze N-terminal Lys and obtain series of ACE inhibitory peptides with N-terminal Lys, but its enzymatic hydrolysis rate is excessively slow (Cushman et al., Citation1973). Papain shows wide substrate specificity; thus, it can cut off internal peptide bonds and decompose proteins into smaller polypeptides (Gu et al., Citation2011). Based on the catalysis principle and mechanism of proteases above, more active peptides may be obtained through the action of multiple enzymes.
In this study, the multi-enzyme mixture of alcalase, trypsin and papain was chosen for hydrolyzation, and dual-response surface methodology (RSM) and Box–Behnken designs (BBD) were performed to optimize the enzymatic conditions to produce ACE-inhibitory peptides from LJPs. Furthermore, the underlying anti-hypertensive mechanism of these peptides was investigated using molecular docking method, so as to identify the specific active sites. These results provided a solution to preparing anti-hypertensive peptides from renewable resources, which is conducive to enhancing the value of Laminaria japonica crude proteins.
2. Materials and methods
2.1. Materials and chemicals
The Laminaria japonica used in this study was bought from Xiapu county, Fujian, China. Hip-His-Leu (HHL) and ACE (from rabbit lung) were purchased from Sigma Chemical Co. (St. Louis, MO, USA). Alcalase was obtained from Novozymes, Beijing, China. Trypsin and Papain were purchased from Sangon Biotech (Shanghai, China) Co., Ltd. Eight hypotensive peptides of Phe-Tyr (FY), Lys-Tyr (KY), Ala-Lys-Tyr (AKY), Gly-Lys-Tyr (GKY), Lys-Phe-Lys-Tyr (KFKY), Phe-Phe-Lys-Tyr (KKFY), Ser-Lys-Thr-Lys (SKTY) and Ala-Lys-Tyr-Ser-Lys (AKYSY) were chemically synthesized by Chinese Peptide Company Ltd (Hangzhou, China). HPLC grade acetonitrile was supplied by Merck Serono Co., Ltd. (Darmstadt, Germany). Other chemicals and reagents were all of analytical grade.
2.2. Pretreatment of Laminaria japonica
The Laminaria japonica was dried, ground and sieved with a 100 mesh after being washed for discarding sand and debris. Removing mannitol, fucoidan and laminarin, the crude LJPs were obtained for the following experiments.
2.3. Selection of enzyme for preparing peptides
Trypsin, papain, and alcalase were used for preparing bioactive peptides. The LJPs were dissolved into different buffer solutions with their optimal pH value until the solutions reached a concentration of 0.5% (w/v). Subsequently, each sample was adjusted to an appropriate temperature and different proteases (trypsin, papain, alcalase, and multi-enzyme mixture of trypsin, papain and alcalase at the ratio of 1:1:1) were added, respectively, at an enzyme/substrate ratio (E/S) of 4% (w/w) for 8 h. Finally, the reaction was stopped by boiling water for 15 min. Then, the protein hydrolysates were cooled to room temperature and centrifuged at 10000 rpm and 4°C for 15 min by using an Avanti J-26XP centrifuge (Beckman Coulter, Inc.). The supernatant was collected and analyzed to detect ACE inhibitory activity.
2.4. Preparation of enzymatic hydrolysates from LJPs
In this study, the hydrolysis power of the multi-enzyme mixture was improved by exploring the variation of pH value (7.0–8.0), temperature (50–60°C) and ratio of the multienzyme mixture chosen from last step (1:2:1, 1:1:1, 2:1:1 for papain, alcalase and trypsin, respectively). Furthermore, the enzymatic hydrolysis efficiency was investigated by varying the level of substrate concentration (1–4%), E/S (2–6%), and hydrolysis time (2–6 hours) to improve the efficiency. All of the samples were deactivated by following the Step 2.3. The supernatant with peptides was collected and determined with ACE inhibitory activity.
2.5. Detection of ACE-inhibitory activity
The ACE-inhibitory activity was measured by the method of Cushman & Cheung with minor modifications (Chen et al., Citation2016; Cushman & Cheung, Citation1971). The standards of ACE and HHL were diluted to 5 mM and 0.1 U·mL−1 respectively by dissolving in 100 mM borate buffer (pH 8.3) with 0.3 M NaCl as a supplement. Each peptides-containing sample (40 μL) was mixed with 5 mM ACE (10 μL) before incubation at 37°C for 5 min to activate the ACE. The mixture was added with 50 μL of HHL and incubated at 37°C for 30 min. Then, it was ended with 200 μL of 1.0 M HCl and centrifuged at 10000 rpm for 5 min. The hippuric acidfrom HHL was detected by HPLC in the supernatant. The mobile phase of ultra-pure water (contained 0.05% TFA and 0.05% triethylamine (pH 2.75–2.8)) and acetonitrile were used at a ratio of 85:15. The analyses were carried out at 0.5 mL·min−1 and 35°C with the column of Waters Xterra MS-C18 column (4.6 × 250 mm I.D., 5 µm). The ACE inhibition of the peptides was calculated according to the following equation:
Where A1 and A2 represent the peak areas of hippuric acid in blank and reaction samples. The IC50 value is defined as the concentration of inhibitor required to inhibit 50% of the ACE activity.
2.6. Optimization of enzymolysis conditions using RSM
Various factors such as temperature, pH and substrate concentration can affect the extraction of peptides from marine source (Lee et al., Citation2014). In general, enzyme activity (i.e. the hydrolysis power) is subjected to influence from the ratio of the multienzyme mixture, temperature and pH, whilst the enzymatic efficiency was closely associated with the level of substrate concentration, E/S, and hydrolysis time. Therefore, dual Box-Behnken designs were performed according to these two characteristics for optimizing the enzymatic conditions of ACE-inhibitory peptides production.
2.6.1. Response surface experiment of enzymatic hydrolysis power
According to the enzymatic hydrolysis power of multi-enzyme, enzyme ratio, pH value and temperature are the main factors to consider and optimize. Three factors at three levels Box–Behnken design (Table S1) was performed to optimize enzymatic hydrolysis power on the ACE inhibitory activity of hydrolysates. The enzymatic treatment was performed under the conditions of liquid-solid ratio of 40, hydrolysis time of 4 h and E/S 4%. A second-order model was established for associating the response variable (ACE inhibitory activity) with the independent variable. The quadratic equation used was shown as follows:
Where Y stands for the predict response variable, Xi and Xj are the levels of the independent variables. The regression coefficients α0, αi, αii, and αij represent the intercept, linear, quadratic, and interaction terms, respectively. The coefficients of the model and the analysis of variance (ANOVA) acquired from the experimental design were performed by the software package Design-Expert 8.0.6 (Stat-Ease, USA).
2.6.2. Response surface experiment of enzymatic hydrolysis efficiency
Based on the optimal enzymatic hydrolysis power, the enzymatic hydrolysis efficiency experiments were also conducted using BBD to optimize the efficiency of enzymatic hydrolysis in terms of substrate concentration, E/S and hydrolysis time, to reach a higher ACE inhibitory activity (Table S2). A second-order model was established to correlate the response variable to the independent variable, and the quadratic equation is expressed as follows:
Where Y stands for IC50 value of ACE inhibitory activity as the response variable too, β0, βi, βii, and βij represent the regression coefficient of the intercept, linear, quadratic, and interaction terms, respectively, and X4, X5 and X6 stand for the levels of independent variables. The coefficients and response surfaces are also obtained from the experimental design.
2.7. Peptides detection
The hydrolysates of LJPs were detected by the RP-HPLC method of Chen et al., which we had established in the previous research (Chen et al., Citation2016). A 20 Å Shimadzu HPLC System (Kyoto, Japan) equipped with a UV spectrophotometric detector was used to carry out the graph of Chromatographic separations at 218 nm. The detection was performed at 45°C and 0.7 mL·min−1 with a Xterra MS-C18 column which was 4.6 × 250 mm I.D. and 5 µm particle size (Waters Corporation, Milford, USA). Finally, the analytical results were collected using Lab solutions software (Shimadzu, Kyoto, Japan).
2.8. Molecular docking
2.8.1. Optimization of receptor protein structure
The crystal structure of tACE (PDB: 1O8A) was obtained from RCSB PDB (Protein Data Bank; http://www.rcsb.org/pdb/home/home.do). As the target protein defined by SYBYL8.1/Bioplymer, crystal water was removed from the ACE model, whereas the cofactor zinc was retained, and the end residues were hydrogenated and charged by default in the protic state. Then, this residual structure was relaxed by limiting part of the structure and the dynamic time was adjusted to 10000 sf before the residual structure was optimized for 1000 steps. The amino acid residues of the known binding site of ACE inhibitors was generated to the prototype molecule as while, for the active pocket of molecule docking in the next step.
2.8.2. Optimal treatment of eight anti-hypertensive peptides
Based on the chemical structure of the anti-hypertensive peptides, the initial structures were constructed by restricting the bond angles of Chiral carbons, optimizing minimal geometric energy, adding a Gasteiser-Huckel charge with the assistance of SYBYL8.1 software, and then optimizing molecular mechanics with convergence limit setting in 0.005 kJ/mol·Å via the tripos force field.
2.8.3. Surflex-dock
The optimized ACE structure taken as receptor and the optimization structures of eight small peptides treated as ligand, Total_Score, Crash and Polar were calculated by SYBYL8.1/Surflex-dock, for determining the intensity of interaction between the ligand and the receptor. The docking results were chosen from the suitable conformation of the four scores.
2.9. Statistical analyses
Statistical analyses were conducted using the software Design-Expert 8.0.6 (Stat-Ease Inc., Minneapolis, MN, USA). All of the experiments were performed in triplicate and the data were indicated by mean ± SD. All of the data were statistically analyzed by ANOVA and the averages were determined by Duncan’s multiple range test (p < .05).
3. Results
3.1. Selection of appropriate protease
The IC50 value for the Laminaria japonica hydrolysates prepared by enzymatic hydrolysis displayed lower than that of the control without enzymatic hydrolysis. In addition, the treatment carried out using multienzyme mixture showed higher ability against ACE activity (IC50 of 6.93 ± 0.33 mg∙mL−1) than if single enzymes were used. The IC50 value of other hydrolysates treated with papain, trypsin and alcalase was shown to be 14.11 ± 0.47 mg∙mL−1, 9.72 ± 0.39 mg∙mL−1, and 7.32 ± 0.31 mg∙mL−1 respectively. The result was consistent with the report that the multienzyme was conducive to the generation of anti-hypertensive peptides, because there were larger amount of peptides with low molecular mass but more active sites obtained (Sato et al., Citation2002). Therefore, the multienzyme is considered more suitable for preparing anti-hypertensive peptides in the following process.
3.2. Optimization of anti-hypertensive peptides’ preparation
3.2.1. Optimization of enzyme activity and its effect on ACE activity
shows the experimental design along with the response values of optimizing enzyme activity to prepare anti-hypertensive peptides. The software Design Expert 8.0.6 (StatEase, Inc, USA) was adopted for experimental design, data analysis, and model construction. A multiple regression equation related to the response function with independent variables is shown as follows:
Table 1. Variables in the Box–Behnken design for optimization of enzyme activity.
Tabla 1. Variables presentes en el diseño Box-Behnken para la optimización de la actividad enzimática
The results and the analysis of variance of the model are summarized in Table S3. The statistical significance of the regression model was checked by the F-value (1330.67) and P-value (<0.0001), indicating that the model was highly statistically significant. The F-value and P-value lack of fit was found to be 1.11 and 0.4418, respectively, suggesting the capability of the model to predict the variations. The coefficient of the quadratic regression model (R2) was determined to be 0.9994, implying that merely 0.06% of the total variations could not be explained by the model. The adjusted determination coefficient (Rad j2 = 0.9987) was close to the R2 value, which demonstrates the high degree of correlation between the experimental and predicted values (Derrien et al., Citation2017). In addition, the interaction terms of X1X2, X1X3 and X2X3 were of significance, suggesting that it was not a simple linear but a nonlinear relationship on three factors and ACE inhibition activity.
According to the results of experiments, the response surface diagram and contour diagram were applied to illustrate the effect and interaction of independent variables on the IC50 value of enzymatic hydrolysates (). The contour diagram showed that the IC50 value initially decreased and then increased progressively as the enzyme ratio changed from 2:1:1 to 1:2:1 and the hydrolysis pH was increased from 7.0 to 8.0. Whilst, the pH value had a more significant influence than the enzyme ratio for the curvature of contour diagram towards the pH value. Similarly, the hydrolysis temperature also increased first and then decreased the IC50 value as the temperature rose from 50°C to 60°C. Compared with the enzyme ratio, the temperature altered the IC50 values more pronouncedly as indicated by the curvature of contour diagram. In addition, pH value and temperature showed a similar effect on IC50 values. Therefore, choosing the optimal pH and temperature could contribute to enhance the activity of enzyme significantly for preparing more bioactive peptides.
Figure 1. The combined effect of enzyme ratio and pH on the enzyme activity of the multi-enzyme mixture.
Figura 1. Efecto combinado de la ratio de enzimas y el pH en la actividad enzimática de la mezcla multienzimática
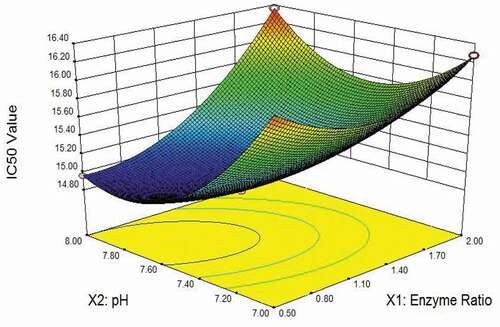
Based on EquationEquation (4)(4)
(4) , the optimum parameters (enzyme ratio 1:2:1, pH 8.0 and 60°C) were acquired to improve enzyme activity and in turn reducing ACE activity to the largest extent. With three parallel experiments were conducted, the average IC50 value was observed to be 14.64 ± 0.53 mg∙mL−1, which was close to the predicted value of 15.01 mg∙mL−1. The experimental value was in good agreement with the prediction results, which indicated that the model of EquationEquation (4)
(4)
(4) was satisfactory and accurate for the efficiency of enzymes. Thus, the optimal parameters obtained by the RSM design were verified as reliable and feasible, which is significant to the next experiment.
3.2.2. Optimization of enzymatic efficiency and its effect on ACE activity
The enzymatic hydrolysis conditions such as substrate concentration, E/S and hydrolysis time also played a crucial role in the production of functional peptides and the suppression of ACE activity. Therefore, the parameters of enzymatic hydrolysis process on the ACE inhibitory activity of LJPs hydrolysates were optimized and the complete design along with response values are shown in . The response variable Y could be characterized by the second-order polynomial equation shown below:
Table 2. Variables in the Box–Behnken design for optimization of enzyme efficiency.
Tabla 2. Variables presentes en el diseño Box-Behnken para optimizar la eficiencia de la enzima
As illustrated in Table S4, the ANOVA analysis of the regression model described the effects of three factors on the IC50 value of ACE inhibition. P-value was less than 0.001, corresponding to the statistical significance of the model P < .0001. The P-value (0.697) for lack of fit far exceeded 0.05, reflecting the residual caused by random error. The R2 value was determined as being close to 1, indicating that the model equation established was effective in reflecting the true behavior of the experiment. The predicted R2 (0.9982) was in accordance with the adjusted R2 (0.9952), implying that the experimental values were highly correlated to the prediction results. Besides, substrate concentration, E/S and hydrolysis time also had significant effects on ACE-inhibitory activities (p < .001), implying that the model was not a simple linear relationship. All these results demonstrate that the model gave a satisfactory mathematical description on the effects of enzymatic hydrolysis conditions for ACE inhibitory activity.
As shown in , the contour diagram in ) shows that at the same hydrolysis time, the IC50 value decreased first and then increased as the substrate concentration rose from 1% to 4%, and the same trend was exhibited as E/S increased from 2% to 6%. The IC50 value declined dramatically and then raised from substrate concentration rising from 1% to 4%, while it declined in 2–5 hours prior to a slight change ()).
Figure 2. The response surface plots for substrate concentration, enzyme–substrate ratio and time: (a) the interaction of substrate concentration and E/S; (b) the interaction of E/S and temperature.
Figura 2. Gráficos de la superficie de respuesta para la concentración del sustrato, la ratio enzima-sustrato y el tiempo: (a) interacción de la concentración de sustrato y la E/S; (b) interacción de la E/S y la temperatura
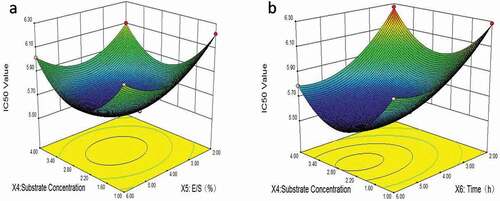
From the predictions of RSM analysis, the optimal conditions of enzymatic hydrolysis were determined as follows: substrate concentration 1.66%, enzyme ratio 4.41%, hydrolysis time 5.14 h. Under the optimum reaction conditions, the predicted IC50 value for ACE inhibitory activity of the enzymatic hydrolysate was 5.64 mg∙mL−1. Considering the feasibility of the practical operations, the optimum conditions were adjusted to be as follows: substrate concentration 1.7%, enzyme ratio 4.4% and 5.1 h. Under three parallel experiments, the average IC50 value was found to be 5.71 ± 0.31 mg∙mL−1, with a 1.24% (<5%) deviation from the predicted value, which implied that the model of EquationEquation (5)(5)
(5) was as accurate as required for the extraction process. The results showed that the parameters of enzymatic hydrolysis conditions as optimized by response surface method are considered to be accurate and reliable.
3.3. Detection of ACE-inhibitory peptides
These anti-hypertensive peptides from LJPs hydrolysate were already verified that they could be determined by RP-HPLC method rapidly and efficiently through replicated experiments and HPLC-MS/MS in our previous work (Chen et al., Citation2016). The detection chromatograms revealed that all of these anti-hypertensive peptides could also be detected in the optimal enzymatic hydrolysates of LJPs.
3.4. Molecular mechanisms of the peptide acting on the target
Anti-hypertensive peptides can suppress ACE activity through binding with ACE active site and then changing the enzyme structures along with a significant loss of enzyme activity (Chaudhary et al., Citation2009; Wu et al., Citation2016). ACE has been reported to contain three main active site pockets: S1, S2 and S1ʹ. S1 pocket is comprised of Ala354, Glu384 and Tyr523 residues, S2 pocket includes Gln281, His353, Lys511, His513 and Tyr520 residues, and S1ʹ contains Glu162 residue (Rohit et al., Citation2012). In order to explore the mechanisms behind the impact of hypotensive peptides on ACE, computational molecular docking was conducted using SYBYL8.1.
As shown in , the N-terminal hydrogen atoms of FY bonded ACE active pockets with Lys511 and His513 in form of hydrogen bond, while they were combined with Gln281 through double hydrogen bonds, thus strengthening the bond and improving its stability. The hydrogen atoms on the FY hydroxyl group were combined with Tyr520 and His387, while the oxygen atoms bonded with Ala365 by forming a hydrogen bond ()). Similarly, the hydrogen atom of KY amino terminal was bound to Gln281 and Glu162 of ACE active pocket, respectively, in the form of two hydrogen bonds, and hydrogen atom of hydroxyl terminal bound to Try520 residue in the same way. However, there were two different hydrogen bonds to Glu384 formed on the hydrogen atom of AKYSY amino terminal and the oxygen atom of hydroxyl terminal, respectively. The hydrogen atom of hydroxyl terminal was bound to His383 residue in the same manner. Likewise, AKY, GKY, KKFY, SKTY and KFKY were all bonded to the different residuals of ACE active pockets by forming the corresponding hydrogen bond, respectively. All of these residues contain a side hydrophilic chain of alcohol-hydroxyl, amino or carboxyl groups, which is conducive to the formation of hydrogen bonds.
Figure 3. The conformation of FY (a), KY (b) and AKYSY (c) bonding with ACE (The green sacs represent ACE active pockets, and the yellow dotted line represents hydrogen bonding between atoms in small peptides and ACE residues.).
Figura 3. Conformación de FY (a), KY (b) y AKYSY (c) enlazando con ECA. (Los sacos verdes representan los paquetes activos de ECA y la línea de puntos amarilla representa el enlace de hidrógeno entre los átomos de los péptidos pequeños y los residuos de ECA)
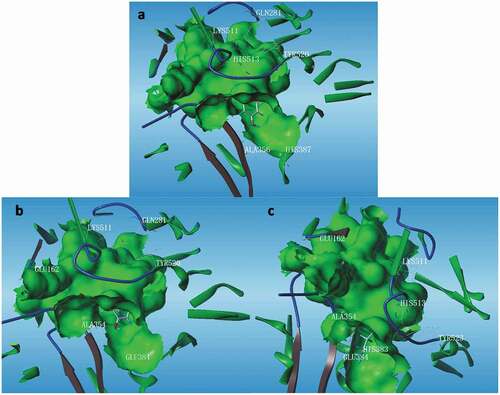
The link between anti-hypertensive peptides and ACE residues is mainly through hydrogen bond, Van der Waals, hydrophobic interaction, and electrostatic force, with hydrogen bond in particular. The interaction sites and the number of hydrogen bonding sites between the eight peptides and ACE residues are presented in . The number of hydrogen bonding sites between KKFY and ACE residues is 13, which is four times more than that of SKTY. Besides, there are eight hydrogen bonding sites existing between AKYSY, KFKY, KY and ACE, respectively, whilst there are only five hydrogen bonding sites binding AKY with ACE, but forming a remarkably stable composite structure. In addition, most of these peptides bind to ACE with the sites of Glu162, Ala354, Glu384 and Lys511 by hydrogen bonding. Therefore, these amino acid residues may be the main active sites of ACE active pockets.
Table 3. The hydrogen bonding sites between small molecules and ACE protein.
Tabla 3. Sitios de enlace de hidrógeno entre las moléculas pequeñas y la proteína ECA
4. Discussion
Despite the huge annual yield of Laminaria japonica, the processing mode still stay in the traditional ways for the preparation of primary products and feeds, or only for the extraction of sodium alginate, mannitol and iodine, which caused a great waste of by-products, especially proteins (approximately 12% of the weight) (Carboni et al., Citation2016). Thus, this study focuses on how to prepare and detect the anti-hypertensive peptides.
According to prior studies, the ACE-inhibitory peptides were prepared, identified or designed on the basis of natural foods through fermentation or enzymatic hydrolysis (Daliri et al., Citation2019). This experiment is innovative in applying the controllable enzymatic hydrolysis technology of multi-enzyme mixture to find out that the multi-enzymatic hydrolysis was more effective in preparing the peptides with ACE-inhibitory activity for more cleavage sites than with the single enzyme. The results were in accordance with the published study which demonstrate that two functional enzymes obtained more low-molecular weight bioactive peptides (Garcia-Vaquero et al., Citation2019; Yuan et al., Citation2020). Meanwhile, dual RSM and BBD method was first applied to optimize the enzymatic hydrolysis conditions of LJPs, with the lowest IC50 value (5.71 ± 0.31 mg∙mL−1) of the hydrolysate obtained. Some research have demonstrated that the small ACE-inhibitory peptides with aromatic and hydrophobic amino acid residues at the C-terminal, such as Tyr, Phe, have the potent ability to inhibit ACE activity (Matsui & Matsumoto, Citation2006). In addition, eight small anti-hypertensive peptides with Tyr at the C-terminal had been detected in LJPs hydrolysates, which had been published in our previous study, and the IC50 value of these small peptides has also been determined (Chen et al., Citation2016). By comparing the IC50 value of ACE-inhibitory peptides with that of the hydrolysates, it was not difficult to find that the IC50 of anti-hypertensive peptides was less potent by more than 3 orders of magnitude, which were measured in micromoles. This is possibly because the anti-hypertensive hydrolysates were a mix of many ingredients which inhibited the activity of ACE-inhibitors, or that the content of active peptides in the hydrolysates was roughly lower than 0.1 mg per 100 g of protein (Chen et al., Citation2016). Elavarasan K. et al. found that the IC50 values of oven-dried and freeze-dried ACE inhibitory peptides from Cirrhinus mrigala turned to be 1.15 mg and 1.53 mg of protein per ml, respectively (Elavarasan et al., Citation2016). If protein was taken as the reference unit, the IC50 value of the optimal LJPs hydrolysate was approximately equal to 1.14 mg of protein per gram, which means a greater effectiveness. In order to investigate the underlying mechanism behind the anti-hypertensive capability of those peptides, molecular docking was used to predict the bonding between peptides and ACE active site. The docking results exhibited that these anti-hypertensive peptides inactivated ACE mainly by binding with each other and forming a remarkably stable composite structure through hydrogen bonds. Through a thorough analysis shown in and , most of docking results were found in accordance with the IC50 value of these peptides as detected before (Chen et al., Citation2016). Among these peptides, FY and AKYSY exhibited strong activity in devitalizing ACE whilst the number of hydrogen bonding sites was less. In contrast, KKFY and SKTY had lower inhibitory activity but more hydrogen bonding sites with ACE. The cause for the reverse effect might be associated with the discrepancy of number, sequence, and species. In addition, the bioactivity of these small peptides also had a close relationship with their space conformation and ionization state, which might be another reason affecting the anti-hypertensive activity. Fu et al. and Krichen et al. revealed that the quantity of hydrogen bonds determined the affinity between peptides and ACE residues (Fu et al., Citation2019; Krichen et al., Citation2018). Additionally, the most active sites were observed to be Glu162, Ala354, Glu384 and Lys511, as shown in, which have been identified as the main sites of ACE active pockets (Rohit et al., Citation2012). Furthermore, it appears that the residues of His is the critical binding sites to ACE, which could explain the strong inhibition but a smaller number of active sites for FY and AKYSY, especially His 513. Thus, the docking results provided a reference for further research on their in vitro and in vivo anti-hypertensive activity.
5. Conclusions
In this study, multi-enzyme system and controlled-enzymatic hydrolysis technology were used to prepare anti-hypertensive peptides from LJPs. The multi-enzyme mixture exhibited a greater effectiveness in preparing the peptides with ACE inhibitory activity compared with the single enzyme. After optimization, the strongest ACE-inhibitory activity of LJPs hydrolysates was achieved under the following conditions: Enzyme ratio is 1:2:1 (papain: alcalase: trypsin), pH value is 8.0, temperature is 60°C, substrate concentration is 1.7%, enzyme addition is 4.4%, and hydrolysis time is 5.1 h. The underlying anti-hypertensive mechanism of these peptides was explored by molecular docking to exhibit that hydrogen bond was the main active force to bind peptides with ACE active sites for forming a remarkably stable composite structure to inactivate ACE. The main active sites of ACE active pockets were observed to be His513, Glu162, Ala354, Glu384 and Lys511. However, further research such as separation and purification of the peptides and the in vitro and in vivo anti-hypertensive activity of them will be performed in the future.
Declaration of interest statement
The authors declare no conflict of interest.
Supplemental Material
Download MS Word (25 KB)Acknowldegements
We would like to express our gratitude to all those who gave us the possibility to complete this thesis.
Supplementary material
Supplemental data for this article can be accessed on the publisher’s website.
Additional information
Funding
References
- Admassu, H., Gasmalla, M. A. A., Yang, R., & Zhao, W. (2018). Bioactive peptides derived from seaweed protein and their health benefits: Anti-hypertensive, antioxidant, and antidiabetic properties. Journal of Food Science, 83(1), 6–16. https://doi.org/https://doi.org/10.1111/1750-3841.14011
- Alashi, A. M., Blanchard, C. L., Mailer, R. J., Agboola, S. O., Mawson, A. J., He, R., Malomo, S. A., Girgih, A. T., & Aluko, R. E. (2014). Blood pressure lowering effects of Australian canola protein hydrolysates in spontaneously hypertensive rats. Food Research International, 55, 281–287. https://doi.org/https://doi.org/10.1016/j.foodres.2013.11.015
- Amorim, M., Pinheiro, H., & Pintado, M. (2019). Valorization of spent brewer’s yeast: Optimization of hydrolysis process towards the generation of stable ACE-inhibitory peptides. Lwt-Food Science and Technology, 111, 77–84. https://doi.org/https://doi.org/10.1016/j.lwt.2019.05.011
- Cao, S., Wang, Y., Hao, Y., Zhang, W., & Zhou, G. (2020). Anti-hypertensive effects in vitro and in vivo of novel angiotensin-converting enzyme inhibitory peptides from bovine bone gelatin hydrolysate. Journal of Agricultural and Food Chemistry, 68(3), 759–768. https://doi.org/https://doi.org/10.1021/acs.jafc.9b05618
- Carboni, S., Clegg, S. H., & Hughes, A. D. (2016). The use of biorefinery by-products and natural detritus as feed sources for oysters (Crassostrea gigas) juveniles. Aquaculture, 464, 392–398. https://doi.org/https://doi.org/10.1016/j.aquaculture.2016.07.021
- Chaudhary, S., Vats, I. D., Chopra, M., Biswas, P., & Pasha, S. (2009). Effect of varying chain length between P-1 and P-1 ‘ position of tripeptidomimics on activity of angiotensin-converting enzyme inhibitors. Bioorganic & Medicinal Chemistry Letters, 19(15), 4364–4366. https://doi.org/https://doi.org/10.1016/j.bmcl.2009.05.079
- Chen, J.-C., Wang, J., Zheng, B.-D., Pang, J., Chen, L.-J., Lin, H.-T., & Guo, X. (2016). Simultaneous determination of 8 small antihypertensive peptides with tyrosine at the C-terminal in L aminaria japonica hydrolysates by RP-HPLC method. Journal of Food Processing and Preservation, 40(3), 492–501. https://doi.org/https://doi.org/10.1111/jfpp.12628
- Cushman, D. W., & Cheung, H. S. (1971). Spectrophotometric assay and properties of the angiotensin-converting enzyme of rabbit lung. Biochemical Pharmacology. 20(7), 1637. https://doi.org/https://doi.org/10.1016/0006-2952(71)90292-9
- Cushman, D. W., Pluscec, J., Williams, N. J., Weaver, E. R., Sabo, E. F., Kocy, O., Cheung, H. S., & Ondetti, M. A. (1973). Inhibition of angiotensin-converting enzyme by analogs of peptides from bothrops-jararaca venom. Experientia, 29(8), 1032–1035. https://doi.org/https://doi.org/10.1007/bf01930447
- Daliri, E. B.-M., Ofosu, F. K., Chelliah, R., Park, M. H., Kim, J. H., & Oh, D. H. (2019). Development of a Soy Protein Hydrolysate with an Anti-hypertensive Effect. International Journal of Molecular Sciences, 20 (6), 1496. https://doi.org/https://doi.org/10.3390/ijms20061496
- Derrien, M., Badr, A., Gosselin, A., Desjardins, Y., & Angers, P. (2017). Optimization of a green process for the extraction of lutein and chlorophyll from spinach by-products using response surface methodology (RSM). Lwt-Food Science and Technology, 79, 170–177. https://doi.org/https://doi.org/10.1016/j.lwt.2017.01.010
- Elavarasan, K., Shamasundar, B. A., Badii, F., & Howell, N. (2016). Angiotensin I-converting enzyme (ACE) inhibitory activity and structural properties of oven- and freeze-dried protein hydrolysate from fresh water fish (Cirrhinus mrigala). Food Chemistry, 206, 210–216. https://doi.org/https://doi.org/10.1016/j.foodchem.2016.03.047
- Fishery Bureau, of Ministry of agriculture and Rural Affairs of China. (2019). The China Fishery Yearbook. China Agriculture Press.
- Fu, W., Chen, C., Zeng, H., Lin, J., Zhang, Y., Hu, J., & Zheng, B. (2019). Novel angiotensin-converting enzyme inhibitory peptides derived from Trichiurus lepturus myosin: Molecular docking and surface plasmon resonance study. Lwt-Food Science and Technology, 110, 54–63. https://doi.org/https://doi.org/10.1016/j.lwt.2019.04.053
- García-Mora, P., Martín-Martínez, M., Ma, B., González-Múniz, R., Peñas, E., Frias, J., & Martinez-Villaluenga, C. (2017). Identification, functional gastrointestinal stability and molecular docking studies of lentil peptides with dual antioxidant and angiotensin I converting enzyme inhibitory activities. Food Chemistry, 221, 464–472. https://doi.org/https://doi.org/10.1016/j.foodchem.2016.10.087
- Garcia-Vaquero, M., Mora, L., & Hayes, M. (2019). In vitro and in silico approaches to generating and identifying angiotensin-converting enzyme I inhibitory peptides from green Macroalga Ulva lactuca. Marine Drugs, 17(4), 204. https://doi.org/https://doi.org/10.3390/md17040204
- Gu, R.-Z., Li, C.-Y., Liu, W.-Y., Yi, W.-X., & Cai, M.-Y. (2011). Angiotensin I-converting enzyme inhibitory activity of low-molecular-weight peptides from Atlantic salmon (Salmo salar L.) skin. Food Research International, 44(5), 1536–1540. https://doi.org/https://doi.org/10.1016/j.foodres.2011.04.006
- Ko, S.-C., Kang, N., Kim, E.-A., Kang, M. C., Lee, S.-H., Kang, S.-M., Lee, J.-B., Jeon, B.-T., Kim, S.-K., Park, S.-J., Park, P.-J., Jung, W.-K., Kim, D., & Jeon, Y.-J. (2012). A novel angiotensin I-converting enzyme (ACE) inhibitory peptide from a marine Chlorella ellipsoidea and its antihypertensive effect in spontaneously hypertensive rats. Process Biochemistry, 47(12), 2005–2011. https://doi.org/https://doi.org/10.1016/j.procbio.2012.07.015
- Kocak, A., Sanli, T., Anli, E. A., & Hayaloglu, A. A. (2020). Role of using adjunct cultures in release of bioactive peptides in white-brined goat-milk cheese. Lwt-Food Science and Technology, 123, 109127. https://doi.org/https://doi.org/10.1016/j.lwt.2020.109127
- Krichen, F., Sila, A., Caron, J., Kobbi, S., Nedjar, N., Miled, N., Blecker, C., Besbes, S., & Bougatef, A. (2018). Identification and molecular docking of novel ACE inhibitory peptides from protein hydrolysates of shrimp waste. Engineering in Life Sciences, 18(9), 682–691. https://doi.org/https://doi.org/10.1002/elsc.201800045
- Kristinsson, H. G., & Rasco, B. A. (2000). Fish protein hydrolysates: Production, biochemical, and functional properties. Critical Reviews in Food Science and Nutrition, 40(1), 43–81. https://doi.org/https://doi.org/10.1080/10408690091189266
- Lee, J. K., Jeon, J.-K., & Byun, H.-G. (2014). Anti-hypertensive effect of novel angiotensin converting enzyme inhibitory peptide from chum salmon (Oncorhynchus keta) skin in spontaneously hypertensive rats. Journal of Functional Foods, 7, 381–389. https://doi.org/https://doi.org/10.1016/j.jff.2014.01.021
- Majumder, K., & Wu, J. (2015). Molecular targets of anti-hypertensive peptides: Understanding the mechanisms of action based on the pathophysiology of hypertension. International Journal of Molecular Sciences, 16(1), 256–283. https://doi.org/https://doi.org/10.3390/ijms16010256
- Matsui, T., & Matsumoto, K. (2006). Lead molecules from natural products: Discovery and new trends: Antihypertensive peptides from natural resource, Elsevier North Holland. (Vol. 2, pp. 255–271).
- Meisel, H., & FitzGerald, R. J. (2003). Biofunctional peptides from milk proteins: Mineral binding and cytomodulatory effects. Current Pharmaceutical Design, 9(16), 1289–1295. https://doi.org/https://doi.org/10.2174/1381612033454847
- Memarpoor-Yazdi, M., Zare-Zardini, H., Mogharrab, N., & Navapour, L. (2020). Purification, characterization and mechanistic evaluation of angiotensin converting enzyme inhibitory peptides derived from Zizyphus Jujuba fruit. Scientific Reports, 10(1), 3976. https://doi.org/https://doi.org/10.1038/s41598-020-60972-w
- Ngo, D.-H., Kang, K.-H., Ryu, B., Vo, T.-S., Jung, W.-K., Byun, H.-G., & Kim, S.-K. (2015). Angiotensin-I converting enzyme inhibitory peptides from anti-hypertensive skate (Okamejei kenojei) skin gelatin hydrolysate in spontaneously hypertensive rats. Food Chemistry, 174, 37–43. https://doi.org/https://doi.org/10.1016/j.foodchem.2014.11.013
- Patten, G. S., Abeywardena, M. Y., & Bennett, L. E. (2016). Inhibition of angiotensin converting enzyme, angiotensin II receptor blocking, and blood pressure lowering bioactivity across plant families. Critical Reviews in Food Science and Nutrition, 56(2), 181–214. https://doi.org/https://doi.org/10.1080/10408398.2011.651176
- Pujiastuti, D. Y., Amin, M. N. G., Alamsjah, M. A., & Hsu, J.-L. (2019). Marine organisms as potential sources of bioactive peptides that inhibit the activity of angiotensin I-converting enzyme: A review. Molecules, 24(14), 2541. https://doi.org/https://doi.org/10.3390/molecules24142541
- Raji, V., Loganathan, C., Sadhasivam, G., Kandasamy, S., Poomani, K., & Thayumanavan, P. (2020). Purification of fucoxanthin from sargassum wightii greville and understanding the inhibition of angiotensin 1-converting enzyme: An in vitro and in silico studies. International Journal of Biological Macromolecules, 148, 696–703. https://doi.org/https://doi.org/10.1016/j.ijbiomac.2020.01.140
- Rohit, A. C., Sathisha, K., & Aparna, H. S. (2012). A variant peptide of buffalo colostrum beta-lactoglobulin inhibits angiotensin I-converting enzyme activity. European Journal of Medicinal Chemistry, 53, 211–219. https://doi.org/https://doi.org/10.1016/j.ejmech.2012.03.057
- Sato, M., Hosokawa, T., Yamaguchi, T., Nakano, T., Muramoto, K., Kahara, T., Funayama, K., Kobayashi, A., & Nakano, T. (2002). Angiotensin I-converting enzyme inhibitory peptides derived from wakame (Undaria pinnatifida) and their antihypertensive effect in spontaneously hypertensive rats. Journal of Agricultural and Food Chemistry, 50(21), 6245–6252. https://doi.org/https://doi.org/10.1021/jf020482t
- Wijesekara, I., & Kim, S.-K. (2010). Angiotensin I-converting enzyme (ACE) inhibitors from marine resources: Prospects in the pharmaceutical industry. Marine Drugs, 8(4), 1080–1093. https://doi.org/https://doi.org/10.3390/md8041080
- Wu, Q., Du, J., Jia, J., & Kuang, C. (2016). Production of ACE inhibitory peptides from sweet sorghum grain protein using alcalase: Hydrolysis kinetic, purification and molecular docking study. Food Chemistry, 199, 140–149. https://doi.org/https://doi.org/10.1016/j.foodchem.2015.12.012
- Yang, Y., Li, A., Zhong, Z., & Xie, M. (2019). Angiotensin converting enzyme inhibitory peptide fractions from Tibet wild peach kernel protein hydrolysates. Acta Alimentaria, 48(4), 495–506. https://doi.org/https://doi.org/10.1556/066.2019.48.4.11
- Yuan, J., Zheng, Y., Wu, Y., Chen, H., Tong, P., & Gao, J. (2020). Double enzyme hydrolysis for producing antioxidant peptide from egg white: Optimization, evaluation, and potential allergenicity. Journal of Food Biochemistry, 44(2), e13113. https://doi.org/https://doi.org/10.1111/jfbc.13113