ABSTRACT
In an attempt to positively regulate meat quality at low NaCl concentration, the influence of L-histidine (L-his) introduction on the postmortem phosphorylation of chicken breast muscle protein that had been cured for 16 h at 1% NaCl was investigated at 90 min postmortem in the present study. The 633 different phosphoproteins (﹥1.2-fold) induced by L-his introduction during salting were identified, and a majority of these proteins were involved in glycolysis, calcium signalling pathway and muscle contraction. Myosin, actin, titin, troponin C (TnC), myosin light-chain kinase (MLCK) and γ-phosphorylase kinase (γ-PHK) were the main differentially expressed proteins (DEPs) that were associated with muscle contraction. An advantageous regulation of phosphoglucomutase-1 phosphorylation, fructose-1,6-bisphosphatase and pyruvate dehydrogenase E1 component was obtained in glycolytic metabolism after treatment with L-his.
RESUMEN
En un intento por regular positivamente la calidad de la carne a baja concentración de NaCl, el presente estudio investigó cómo influye la introducción de L-histidina (L-his) en la fosforilación post-mortem de la proteína del músculo de la pechuga de pollo curada durante 16 horas a 1% de NaCl después de 90 minutos post-mortem. Los resultados de este procedimiento permitieron verificar la presencia de 633 fosfoproteínas diferentes (﹥1.2 veces) inducidas por la introducción de L-his durante la salazón; la mayoría de las mismas estaba implicada en la glucólisis, la vía de señalización del calcio y la contracción muscular. Además, se comprobó que las principales proteínas expresadas diferencialmente (DEPs) asociadas con la contracción muscular fueron la miosina, la actina, la titina, la troponina C (TnC), la cadena ligera de miosina quinasa (MLCK) y la γ-fosforilasa quinasa (γ-PHK). Al respecto, tras el tratamiento con L-hisse se obtuvo una regulación ventajosa de la fosforilación de la fosfoglucomutasa-1, la fructosa-1,6-bisfosfatasa y el componente E1 de la enzima piruvato deshidrogenasa en el metabolismo glucolítico.
1. Introduction
Excessive sodium chloride (NaCl) intake has been gradually recognized as a health hazard due to its association with hypertension, cardiovascular disease and bone disease. Meat products represent one of the major sources of NaCl, and consumers urgently demand low-sodium meat products.
Protein phosphorylation is the most common post-translational modification, involved in every aspect of cellular biological processes (Graves & Krebs, Citation1999). In mice, a low-salt diet (0.01% NaCl) was reported to activate the WNK-SPAK-NKCC1 phosphorylation cascade in aortic tissue, resulting in an increase in vascular tone to avoid decreases in blood pressure (Zeniya et al., Citation2013). Furthermore, previous studies have found that protein phosphorylation affected glycolysis (Huang et al., Citation2012), muscle contraction (Stull et al., Citation2011) and muscle protein degradation (Doumit & Bates, Citation2000), indicating that it plays an essential role for meat quality development in postmortem muscle. It was found that troponin T, myosin light chain, actin, and tropomyosin beta chain were identified as differential phosphoproteins induced by 3% NaCl, compared to the 0% NaCl-salted group in the study of Z. Wang et al. (Citation2017), who concluded that NaCl might influence meat quality through protein phosphorylation, which regulated protein function and glycolysis. A study by C. Zhang et al. (Citation2016) showed that NaCl concentrations of 2% and higher significantly reduced myofibrillar protein phosphorylation (P<0.05), and 3% NaCl caused the lowest phosphorylation of myofibrillar proteins after 16 h of salting, indicating that NaCl concentrations could influence protein phosphorylation during meat processing. NaCl was essential and affected the physicochemical phenomena of meat products, while reduced NaCl concentrations led to poor quality. We questioned how we could meet the consumer demand for low-sodium meat products and still achieve a positive regulation of protein phosphorylation under low-NaCl conditions.
In recent years, amino acids have been used as salt substitute components, not only to weaken the off-taste of potassium salts (Campagnol et al., Citation2011; Tahergorabi et al., Citation2012; Zhou et al., Citation2014) but also to ameliorate muscle protein physico-chemical characteristics under low-sodium conditions. L-histidine (L-his) is an alkaline amino acid that might interact with NaCl to contribute to the product’s saltiness (Zhang et al., Citation2014). L-his has the ability to increase myosin solubility (Guo et al., Citation2015), cause a myosin secondary structure transformation (Guo et al., Citation2015), and improve heat-induced gel properties of myosin/myofibrillar protein gels (Chen et al., Citation2016; Zhang et al., Citation2017) at low NaCl/KCl concentrations (1 mmol/L and 0.15 mol/L). Therefore, L-his had great potential to enhance muscle protein processing characteristics when the NaCl concentration was reduced. As is well known, muscle protein processing characteristics directly affect meat quality, and protein phosphorylation plays an important role in the postmortem development of meat quality, including tenderness (Z. Li et al., Citation2017) and colour (Meng et al., Citation2017). We hypothesized that L-histidine might make a difference in the phosphorylation of muscle protein at low NaCl concentrations, causing an improvement in the functional characteristics of muscle protein and meat quality. However, whether the introduction of L-histidine affects the level of muscle protein phosphorylation was unknown, especially under low NaCl conditions. Therefore, the present objective was to investigate the influence of L-histidine on the phosphorylation level of chicken breast muscle, analyse the proteins that were differentially phosphorylated in response to L-histidine and provide a new approach to positively regulate meat quality at low NaCl concentrations for postmortem processing.
2. Materials and methods
2.1. Sample preparation
Eighteen Qingjiaoma broilers (90 d old, 1.6 ± 0.1 kg) were transported from the holding area of a commercial plant to the abattoir within approximately 30 min. After resting for one hour, all the broilers were slaughtered according to Guidelines for Experimental Animals at Nanjing Agricultural University. Breast muscle was rapidly removed, cut into cubes (approximately 2 cm×2 cm) and immediately snap-frozen in liquid nitrogen as 0 min post-mortem. The other pieces were randomly divided into five groups for 30 min, 60 min, 90 min, 120 min and 240 min (at 4°C) postmortem analysis in triplicate, and then snap-frozen in liquid nitrogen. All samples were stored at −80°C until analysis.
2.2. pH measurement
The pH values were determined by inserting a calibrated pH probe (Testo205 pH meter, Lenzkirch, Germany) directly into the minced muscle. Each sample was measured in three different locations.
2.3. Glycogen and ATP/ADP/AMP determinations
Muscle glycogen was measured using a commercial kit (Nanjing Jiancheng Bioengineering Institute, China). Each muscle sample was measured in triplicate according to the manufacturers’ instructions. Briefly, 85 mg of sample was mixed with 255 μL of concentrated alkali and heated for 20 min in boiling water. After cooling, the mixture was diluted with 1.36 mL of distilled water to obtain a 5% glycogen assay liquid. Then, 2.0 mL of staining solution was mixed with 0.9 mL of distilled water and 0.1 mL of glycogen assay liquid and heated for 5 min in boiling water and then cooled with running water. Optical density (OD) was determined at 620 nm. Glycogen content was calculated by referring the same volume of standard solution in the kit and distilled water. The ATP, ADP and AMP contents of post-mortem muscle were determined by HPLC analysis as previously described according to S. Wang et al. (Citation2013). Briefly, 1.0 g of frozen muscle sample was homogenized in 5 ml of 7% ice-cold perchloric acid at 13,500 rpm for 30 s (Ultra Turrax T25, IKA, Germany). The homogenate was centrifuged at 15,000 × g for 10 min at 4°C (Avanti J-E, BACKMAN, CA). The supernatant was neutralized with 0.85 M KOH and centrifuged again (15,000 × g, 4°C for 10 min) to remove KClO4. The neutralized supernatant was then passed through a 0.2 μm filter before injection onto the HPLC system (Agilentl100, Agilent Technologies). Ten microliters of filtrate was injected into the chromatography column (Zorbax SB-C18, Particle Size: 5 μm, 0.3 × 150 mm, Agilent). Mobile phase flow rate was 1.0 mL/min and constituted an aqueous (A) and an organic phase (B). Mobile phase A was phosphate buffer (2.5 mM Tetrabutylammonium hydrogen sulfate, 0.04 M potassium dihydrogen orthophosphate and 0.06 M dipotassium hydrogen orthophosphate, pH 7.0). Mobile phase B was methanol. Proportion of mobile phases A and B were 86.5% and 13.5%, respectively. UV detection was carried out at 254 nm. Peaks were identified and quantified by comparison of retention time and peak area with known external standards.
2.4. Protein preparation
2.4.1. Protein preparation for phosphoproteome analysis
The muscle obtained from 30 Qingjiaoma broilers was selected for phosphoproteome analysis after 90 min postmortem. After being sliced, minced and mixed, the muscle samples were divided into two groups for salting treatments with 1% NaCl and 1% NaCl+0.06% L-his for 16 h at 4°C. Then, the samples were ground into powder under liquid nitrogen, and lysed with four volumes of lysis buffer (8 M urea, 1% protease inhibitor (Roche, Hvidovre, Denmark) and 1% phosphatase inhibitor (Roche, Hvidovre, Denmark)) on ice using a high intensity ultrasonic processor (Ningbo Scientz Biotechnology Co., China). The remaining debris was removed by centrifugation at 12000 g at 4°C for 10 min. Finally, the supernatant was collected, and protein concentrations were determined using a BCA kit (Nanjing Jiancheng Bioengineering Institute, China) according to the manufacturer’s instructions.
2.4.2. Trypsin digestion and phosphopeptides affinity enrichment
The protein solution was reduced with 5 mM dithiothreitol for 30 min at 56°C and alkylated with 11 mM iodoacetamide for 15 min at 25°C in the dark. The protein samples were then diluted by adding 100 mM triethylammonium bicarbonate buffer (TEAB) to urea concentration at less than 2 M. Finally, trypsin was added at a 1:50 trypsin-to-protein (w/w) ratio for the first digestion overnight at 37°C and a 1:100 trypsin-to-protein (w/w) ratio for a second 4-h digestion. After digestion, the peptide mixtures were solubilized in a 50% acetonitrile and 6% trifluoroacetic acid buffer and were incubated with an IMAC microspheres suspension for 60 min with vibration. The IMAC microspheres with enriched phosphopeptides were collected by centrifugation and washed with a 50% acetonitrile and 6% trifluoroacetic acid buffer and with a 30% acetonitrile and 0.1% trifluoroacetic acid buffer three times, sequentially. Finally, 10% NH4OH was added and the enriched phosphopeptides were eluted with vibration for 10 min. The supernatant containing the phosphopeptides was collected and lyophilized for further LC-MS/MS analysis.
2.5. LC-MS/MS
2.5.1. Peptides fractions analysis
Peptide fractions were dissolved in 0.1% formic acid (solution A) and analysed using an EASY-nLC1000 UPLC system (Thermo Scientific). The chromatographic gradient consisted of 0–26 min from 6% to 23% solution B (0.1% formic acid in 98% acetonitrile), 26–34 min from 23% to 35% B, 34–37 min from 35% to 80% B and holding at 80% for the last 3 min at a constant flow rate of 400 nL/min. The peptides were subjected to NSI source followed by tandem mass spectrometry (MS/MS) in Q ExactiveTM Plus (Thermo) coupled online to the UPLC. An MS scan (350–1800 m/z) was acquired and intact peptides were detected in the Orbitrap analyser with a resolution of 70000. Peptides were then selected for MS/MS using NCE setting as 28, and the fragments were detected in the Orbitrap at a resolution of 17500. A data-dependent procedure that alternated between one MS scan followed by 20 MS/MS scans with 15 s dynamic exclusion at automatic gain control (AGC) 5E4.
2.5.2. Database search
The resulting MS/MS data were processed using the MaxQuant search engine (v.1.5.2.8). Trypsin/P was specified as a cleavage enzyme allowing up to two missing cleavages. The mass tolerance for precursor ions was set as 20 ppm in the first search and 5 ppm in the main search, and the mass tolerance for fragment ions was set as 0.02 Da. Carbamidomethyl on Cys was specified as fixed modification and acetylation modification and oxidation on Met were specified as variable modifications. The integrated percolator algorithm was set to a q-value of <0.01, which ensured a peptide-spectrum match false discovery rate (FDR) <0.01.
2.5.3. Bioinformatic and statistical analysis
Proteins were classified into three categories by GO annotation using the UniProt-GOA database (http://www.ebi.ac.uk/GOA/). For each category, a two-tailed Fisher exact test was employed to test the enrichment of each differentially modified protein against all identified proteins. The GO with a corrected p-value <0.05 was considered significant. Analysis of the cluster of orthologous groups (COG) of proteins was performed using the NCBI database (https://www.ncbi.nlm.nih.gov/). The KEGG database (http://www.genome.jp/kegg/) was used to identify enriched pathways by a two-tailed Fisher’s exact test to compare the enrichment of each differentially expressed protein against all identified proteins. The pathway with a corrected p-value <0.05 was considered significant.
All differentially expressed modified protein database accessions or sequences were searched against the STRING database version 10.5 for protein–protein interactions. STRING defines a metric called “confidence score” to define interaction confidence and we fetched all interactions that had a confidence score ≥0.7.
Differences of pH values, ATP, AMP, ADP and glycogen among the samples were analysed using a statistical analysis system (SAS Institute Inc., Cary, NC, USA). An analysis of variance (ANOVA) was performed with a significance level of P < .05. Duncan’s multiple-range test was used to evaluate the differences between the treatments.
3. Results and discussion
3.1. pH value and glycogen, ATP, ADP and AMP content analysis
After slaughter, the pH value in breast was markedly decreased from 6.66 to 5.68 with postmortem time (P<0.05), and not significantly changed after 90 min postmortem (P>0.05) (). This agreed with the result of Perlo et al. (Citation2012) who reported that broiler muscle pH decreased at 2 h (pH 5.91) and it did not change afterwards. It is known that the reduction of muscle pH is usually accompanied by the depletion of ATP during the conversion of muscle to meat after slaughter. ATP, as a phosphate donor, is a necessary energy substance for muscle protein phosphorylation and provides a phosphate group to attach to the amino acids. In the present study, from 0 to 240 min postmortem, a decrease was obtained in the content of glycogen, ATP and ADP of muscle (P<0.05), especially from 120 min to 240 min postmortem, while the AMP content exhibited the converse case, showing that the energy was nearly exhausted after 120 min postmortem. At 90 min postmortem muscle pH was decreased to 5.74 and did not change afterwards, indicating the onset of rigor mortis. In PM bovine muscle, it was reported that myosin regulatory light chain 2 (MyLC2) became phosphorylated during rigor formation (Muroya et al., Citation2007). Based on comprehensive consideration, postmortem 90 min was chosen for phosphoproteome analysis in the present study.
Table 1. Changes of pH, glycogen, ATP, ADP and AMP value with postmortem time (n = 3).
Tabla 1. Cambios de pH, glucógeno, ATP, ADP y valor AMP con el tiempo post-mortem (n = 3)
3.2. Phosphorylation level of DEPs
In this study, a quantitative L-histidine treatment vs. NaCl treatment ratio higher than 1.2 was considered to indicate upregulation, while a quantitative ratio of less than 1/1.2 was considered to indicate downregulation. As shown in ), the addition of L-histidine caused 633 differentially expressed phosphoproteins, of which 307 were upregulated and 326 were downregulated, including 484 upregulated phosphorylation sites and 716 downregulated phosphorylation sites at 1% NaCl. Proteins were upregulated, implying the increase phosphorylation level (Baba et al., Citation2010). The results in the present study indicated that L-histidine introduction decreased phosphorylation level at 1% NaCl.
Figure 1. Regulated phosphoproteins analysis (A) and COG classification (B) of differentially expressed phosphoproteins in 1% NaCl+0.06% L-his vs 1% NaCl treatment.
Figura 1. Análisis de las fosfoproteínas reguladas (A) y clasificación COG (B) de las fosfoproteínas expresadas diferencialmente en el tratamiento a 1% de NaCl+0.06% L-his vs. 1% NaCl
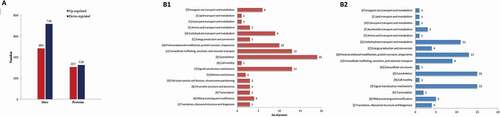
Generally, proteins were phosphorylated on serine, threonine and tyrosine residues sites. In the 1% NaCl+0.06% L-his vs 1% NaCl treatment, serine was the most frequently phosphorylated sites (80.8%) followed by threonine (15.1%) and then tyrosine, which account for only 4.1% of the phosphorylated sites of differentially expressed phosphoprotein. This was in accordance with the results of Liu et al. (Citation2018), who also reported that serine residues were the most phosphorylated sites in caprine muscle. The assignment of the phosphorylation site information of differentially expressed phosphoproteins between NaCl and L-histidine treatments were listed in Table S1 (Supplementary data).
3.3. COG classification of DEPs
Cluster of orthologous groups (COG) analysis was performed to characterize the function classification of the differentially expressed proteins between 1% NaCl+0.06% L-his and 1% NaCl as shown in ). Most of the upregulated (), Table S2) and downregulated proteins (), Table S2) were involved in the cytoskeleton. These were followed by signal transduction mechanisms, mainly including myosin heavy chain (P13538), actin (P68139), alpha-actinin-2 (R9PXQ0), myosin heavy chain 1 (P02604) and serine/threonine protein phosphatase 2A regulatory subunit (E1C5A3). Many downregulated proteins were then involved in posttranslational modification, protein turnover and chaperones, while the upregulated proteins were associated with intracellular trafficking, secretion, and vesicular transport. Furthermore, carbohydrate transport and metabolism were the main functions of the downregulated proteins. Phosphoglucomutase-1 (F1NN63), phosphoglycerate kinase (F1NU17), glyceraldehyde-3-phosphate dehydrogenase (P00356), triosephosphate isomerase (P00940), FBPase (D-fructose-1,6-bisphosphate 1-phosphohydrolase 2, F1NY12) and phosphorylase b kinase regulatory subunit (E1BQ27) were primary proteins involved in carbohydrate transport and metabolism. Proteins were identified that have been reported to participate in glycogen metabolism (Liu et al., Citation2018). The phosphorylation of enzymes involved in glycogen metabolism, especially in glycolysis, affects meat qualities including colour, tenderness and water retention (Batuer et al., Citation2012). Phosphoglucomutase-1 (PGM 1), playing a pivotal role in glycolysis metabolism, is able to catalyse the conversion of glucose-1-phosphate to glucose-6-phosphate and has been related to meat tenderness. It was observed that the phosphorylation level of PGM 1 in tender beef samples was lower than that in tough beef sample (Anderson et al., Citation2014). In high-quality goat longissimus (more tender), the level of phosphorylated PGM 1 was also downregulated (Liu et al., Citation2018). Furthermore, PGM 1 activity was positively regulated by its phosphorylation level (Anderson et al., Citation2014), and high PGM 1 activity was generally correlated with higher PGM 1 expression in cells. Reported that PGM 1 levels were positively correlated with redness and colour stability in M. psoas major from Chinese luxi yellow cattle. A similar phenomenon was found in pigs longissimus in the study of Kwasiborski et al. (Citation2008), meaning that the lower phosphorylation level of PGM 1 was conducive to meat colour stability. The downregulated phosphorylated PGM 1 in the present study indicated that L-his introduction caused a decrease in PGM 1 phosphorylation levels, contributing to improved tenderness and colour, which might be one of the reasons for good TPA properties and colour in cooked pork loin when L-his was used as a salt substitute component (Zhang et al., Citation2018).
3.4. DEPs functional enriched between 1% NaCl and 1% NaCl+0.06%L-His treatments
To further understand the DEPs caused by L-histidine introduction, GO terms enrichment (), Table S3) and KEGG pathway enrichment (), Table S4) were performed. Fisher’s exact test p value (-log10[Fisher’s exact p value]) indicated the enrichment levels of DEPs; a larger p value showed more DEPs enriched in this category. The prominent GO terms enriched by upregulated DEPs between 1% NaCl+0.06% L-his vs 1% NaCl treatment were molecular function and biological process, mainly involving structural components of the cytoskeleton, actin binding, single-organism carbohydrate catabolic and monosaccharide biosynthetic process ( A1). The downregulated DEPs were mainly associated with the terms cellular component and biological process, being associated with terms including myofibril, contractile and supramolecular fibre, supramolecular polymer and complex, contractile fibre part, actin cytoskeleton, myosin complex, and ribose phosphate, nucleoside and nucleoside phosphate metabolic process. In addition, KEGG analysis was performed on the DEPs. The results indicated that the downregulated proteins were mainly enriched in 11 pathways and that glycolysis/gluconeogenesis and calcium signalling were the top 2 enriched pathways. Most of the upregulated proteins were enriched by glycolysis/gluconeogenesis ( B2), and all of the DEPs were significantly enriched in the calcium signalling pathway ( B1). The results of the KEGG pathway enrichment analysis indicated that the DEPs caused by L-histidine introduction were mainly involved in muscle protein cytoskeleton, signalling pathway and carbohydrate metabolism, which was in accordance with the results of COG classification. Many of the differentially expressed phosphoproteins were involved with muscle protein cytoskeleton, myosin heavy chain, actin and alpha-actinin-2 pathways. Their phosphorylation levels play an essential role in regulating muscle contraction post-postmortem. Phosphorylated myosin heavy chain could interact with actin, which was affected by myosin heavy-chain phosphorylation, inducing muscle contraction, and further influence meat tenderness.
Figure 2. Go enrichment (A1, A2) and KEGG (B1, B2, B3) enrichment analysis of identified different phosphoproteins in 1% NaCl+0.06% L-his vs 1% NaCl treatment.
Figura 2. Análisis de enriquecimiento Go (A1, A2) y KEGG (B1, B2, B3) de las diferentes fosfoproteínas identificadas en el tratamiento con 1% de NaCl+0.06% de L-his vs. 1% de NaCl
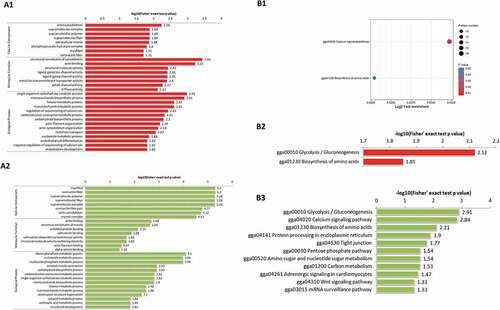
In post-mortem muscle, the exhaustion of ATP was accompanied with pH decline and release of Ca2+, influencing the phosphorylation of sarcoplasmic and myofibrillar proteins, further resulting in protein function change (C. Li et al., Citation2015). The DEPs caused by L-his introduction here were significantly enriched in the calcium signalling pathway (P<0.05), indicating that these DEPs related to the calcium pathway were calcium dependent proteins, which might play roles in rigor mortis development and affect meat quality. Additionally, phosphorylation of PGM 1 identified via COG in the present study might be in response to calcium release and the activation of calmodulin-dependent protein kinase (Lee & Landry, Citation1992).
3.5. Calcium signalling pathway and glycolysis pathway analysis
For postmortem skeletal muscles, Ca2+ ions were released from the sarcoplasmic reticulum to increase the continuous muscle contraction, which contributes to meat tenderness (Huang et al., Citation2012). The most significantly enriched KEGG pathway in the present study was the calcium signalling pathway (). The DEPs associated with muscle contraction were troponin C (TnC), myosin light-chain kinase (MLCK) and γ-phosphorylase kinase (γ-PHK), and their phosphorylation was all decreased. TnC was the protein involved in binding Ca2+ and was reported to interact with troponin T (TnT) to greatly decrease the extent of phosphorylation of TnT, which then increased the TnT degradation by calpains (Di Lisa et al., Citation1995). It was suggested that a decrease in TnC phosphorylation caused by L-histidine introduction could result in a change of TnT phosphorylation and could further influence meat tenderness. MLCK was shown to be an important kinase that regulates myosin light-chain phosphorylation and could be phosphorylated in the absence of Ca2+/calmodulin. Decreased phosphorylation of MLCK could cause an increase in myosin phosphorylation and muscle traction (Conti & Adelstein, Citation1981). Notably, current studies generally found that the experimental factors had an effect on the phosphorylation extent of the phosphorylated substrates, while in the present study, it was observed that L-histidine introduction evidently caused a change in the phosphorylation level of protein kinase, which then phosphorylated the phosphoproteins. MLCK and PHK could interact with calmodulin (Cohen, Citation1982) and γ-PHK was reported to be phosphorylated by a cAMP-dependent kinase (Jesse-Chan et al., Citation1982). Furthermore, cAMP-dependent kinase also phosphorylated TnC (Pratje & Heilmeyer, Citation1972), suggesting that the change in TnC and γ-PHK phosphorylation levels might be due to the effect of L-histidine introduction on the cAMP-dependent kinase properties. Sharma (Citation1991) reported that L-histidine could activate cAMP-dependent kinase resulting in an increased phosphodiesterase phosphorylation rate.
Figure 3. The calcium signaling pathway and glycolysis/gluconeogenesis pathway obtained by KEGG pathway analysis. The red rectangle represented up-regulated proteins. The green rectangle represented down-regulated proteins. The yellow rectangle represented both up and down-regulated proteins.
Figura 3. Vía de señalización del calcio y vía de la glucólisis/gluconeogénesis obtenidas mediante el análisis de vías KEGG. El rectángulo rojo representa las proteínas reguladas al alza. El rectángulo verde representa las proteínas reguladas a la baja. El rectángulo amarillo representa tanto las proteínas reguladas al alza como las reguladas a la baja
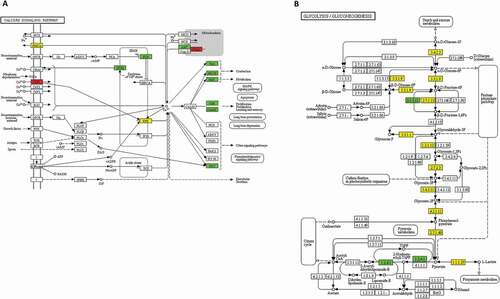
Glycolysis was the critical metabolic pathway to convert glycogen into lactate under anaerobic conditions, and the rapid postmortem glycolysis would cause the development of pale, soft and exudative meat. Several studies have demonstrated that the phosphorylation of glycolytic enzymes would lead to a change in enzyme activity, affecting the rate of glycolysis. Fructose-1,6-bisphosphatase (EC 3.1.3.11) was thought to play an important role in the control of carbohydrate metabolism and could catalyse the hydrolysis of fructose-1,6-bisphosphate to fructose-6-phosphate, controlling the rate of fructose-6-phosphate production in the gluconeogenic pathway. It was reported that phosphorylated fructose-1,6-bisphosphatase had higher enzyme activity than that of the unphosphorylated (Ekdahl & Ekman, Citation1985), and fructose-1,6-bisphosphatase was phosphorylated by cAMP-dependent protein kinase and this phosphorylation caused a 2-fold decrease in the km of the enzyme for fructose 1,6-bisphosphate (Meek & Nimmo, Citation1984), indicating an increase in affinity of the fructose-1,6-bisphosphatase for the fructose 1,6-bisphosphate substrate. The decreased phosphorylation level of fructose-1,6-bisphosphatase in the present study meant a lower affinity and reduced fructose-6-phosphate production, which could slow the rate of glycolysis and pH decline in early postmortem muscle, further increasing tenderness and desirable meat qualities (Immonen & Puolanne, Citation2000).
The phosphorylation of pyruvate dehydrogenase E1 component (EC 1.2.4.1) was reported to regulate pyruvate dehydrogenase activity, and pyruvate dehydrogenase activity was reduced primarily through the increased phosphorylation of its pyruvate dehydrogenase E1 component (Ravindran et al., Citation1996). In the present study, the pyruvate dehydrogenase E1 component exhibited decreased phosphorylation levels, indicating that pyruvate dehydrogenase activity was enhanced. The pyruvate dehydrogenase catalysed the irreversible oxidative decarboxylation of pyruvate, leading to the generation of CO2, NADH, and acetyl-coenzyme A, and linking glycolysis to the tricarboxylic acid cycle (Rardin et al., Citation2009). Pyruvate was also converted to lactate by lactate dehydrogenase catalysis under anaerobic conditions. Based on these previous studies, we supposed that L-histidine introduction may improve the activity of pyruvate dehydrogenase, which accelerated the process of the generation of CO2, NADH, and acetyl-coenzyme A, leading to a reduction in the pyruvate consumption for lactate generation. The lower lactate generation helped to slow down the rate of pH decline in early postmortem meat and led to improved product quality (Immonen & Puolanne, Citation2000).
3.6. Protein–protein interaction network analysis
DEPs obtained from the comparison between 1% NaCl and 1% NaCl+L-His were analysed using string software to explore the protein–protein interaction network (). Proteins usually interact with other proteins to perform their various functions. It has been shown that proteins (mainly MYH1F, MYH1E, ACTN2, MYH1A, MYH1G, MYH1B, MYH1C, TNNI2, TNNC2, ACTA1, ENSGALG00000009089) from the skeleton interact strongly with each other, and proteins (mainly FBP2, MPI, PFKM, PDHA1, LDHA, PGAM1, TPI1, GAPDH, PGK1, PKM2, PGM1, AGL) involved in glycolysis and energy metabolism interact closely. The protein–protein interactions induced by L-histidine in postmortem phosphorylation further demonstrated that the linking proteins between muscle contraction and glycolysis metabolism were FBP2, MPI and PFKM. The regulation of phosphorylation proteins involved in glycolysis and muscle contraction might indirectly affect the glycolysis pathway (Chen et al., Citation2019). In the PPI figure, the red nodes indicate upregulation, and the green nodes indicate downregulation, with the colour intensities proportional to the levels. It was clear that the phosphorylation levels of most phosphoproteins were down regulated in addition to being multi-regulated.
4. Conclusion
L-histidine introduction caused a total of 633 phosphoproteins to be significantly different in chicken breast muscle salted with 1% NaCl, of which 27 phosphoproteins were involved in the cytoskeleton after COG analysis. The DEPs were mainly related to glycolysis, calcium signalling pathway and muscle contraction. Furthermore, most DEPs exhibited down phosphorylation levels, indicating a positive regulation. We have demonstrated that L-his has the potential to improve meat quality through the regulation of phosphorylation at low-NaCl conditions.
Supplemental Material
Download Zip (259.1 KB)Disclosure statement
No potential conflict of interest was reported by the author(s).
Supplementary material
Supplemental data for this article can be accessed on the publisher’s website
Additional information
Funding
References
- Anderson, M. J., Lonergan, S. M., & Huff-Lonergan, E. (2014). Differences in phosphorylation of phosphoglucomutase 1 in beef steaks from the longissimus dorsi with high or low star probe values. Meat Science, 96(1), 379–384. https://doi.org/https://doi.org/10.1016/j.meatsci.2013.07.017
- Baba, T., Kobayashi, H., Kawasaki, H., Mineki, R., Naito, H., & Ohmori, D. (2010). Glyceraldehyde-3-phosphate dehydrogenase interacts with phosphorylated Akt resulting from increased blood glucose in rat cardiac muscle. FEBS Letters, 584(13), 2796–2800. https://doi.org/https://doi.org/10.1016/j.febslet.2010.05.015
- Batuer, A., Patigu, A., & Jueken, A. (2012). Analysis of quality characteristics of lamb from different anatomical locations of Bashbay sheep. Xinjiang Agricultural Sciences, 49(9), 1734–1741. https://doi.org/https://doi.org/10.6048/j..1001-4330.2012.09.026
- Campagnol, P. C. B., dos Santos, B. A., Morgano, M. A., Terra, N. N., & Pollonio, M. A. R. (2011). Application of lysine, taurine, disodium inosinate and disodium guanylate in fermented cooked sausages with 50% replacement of NaCl by KCl. Meat Science, 87(3), 239–243. https://doi.org/https://doi.org/10.1016/j.meatsci.2010.10.018
- Chan, K. F., Hurst, M. O., & Graves, D. J. (1982). Phosphorylase kinase specificity. A comparative study with cAMP-dependent protein kinase on synthetic peptides and peptide analogs of glycogen synthase and phosphorylase. Journal of Biological Chemistry, 257(7), 3655–3659. https://doi.org/https://doi.org/10.1016/0165-022X(82)90005-7
- Chen, L., Li, X., Ni, N., Liu, Y., Chen, L., Wang, Z., Shen, Q. W., & Zhang, D. (2016). Phosphorylation of myofibrillar proteins in post‐mortem ovine muscle with different tenderness. Journal of the Science of Food and Agriculture, 96(5), 1474–1483. https://doi.org/https://doi.org/10.1002/jsfa.7244
- Chen, L., Li, Z., Everaert, N., Lametsch, R., & Zhang, D. (2019). Quantitative phosphoproteomic analysis of ovine muscle with different postmortem glycolytic rates. Food Chemistry, 280, 203–209. https://doi.org/https://doi.org/10.1016/j.foodchem.2018.12.056
- Cohen, P. (1982). The role of protein phosphorylation in neural and hormonal control of cellular activity. Nature, 296(5858), 613. https://doi.org/https://doi.org/10.1038/296613a0
- Conti, M. A., & Adelstein, R. S. (1981). The relationship between calmodulin binding and phosphorylation of smooth muscle myosin kinase by the catalytic subunit of 3ʹ: 5ʹcAMP-dependent protein kinase. Journal of Biological Chemistry, 256(7), 3178–3181. https://doi.org/https://doi.org/10.1088/2041-8205/792/1/L2
- Di Lisa, F., De Tullio, R., Salamino, F., Barbato, R., Melloni, E., Siliprandi, N., & Pontremoli, S. (1995). Specific degradation of troponin T and I by μ-calpain and its modulation by substrate phosphorylation. Biochemical Journal, 308(1), 57–61. https://doi.org/https://doi.org/10.1042/bj3080057
- Doumit, M. E., & Bates, R. O. (2000). Regulation of pork water holding capacity, color, and tenderness by protein phosphorylation. Pork Quality, 63(1), 17–22. https://www.researchgate.net/publication/238712632
- Ekdahl, K. N., & Ekman, P. (1985). Fructose-1, 6-bisphosphatase from rat liver. A comparison of the kinetics of the unphosphorylated enzyme and the enzyme phosphorylated by cyclic AMP-dependent protein kinase. Journal of Biological Chemistry, 260(26), 14173–14179. https://doi.org/https://doi.org/10.1016/0165-022X(85)90070-3
- Graves, J. D., & Krebs, E. G. (1999). Protein phosphorylation and signal transduction. Pharmacology & Therapeutics, 82(2–3), 111–121. https://doi.org/https://doi.org/10.1016/S0163-7258(98)00056-4
- Guo, X. Y., Peng, Z. Q., Zhang, Y. W., Liu, B., & Cui, Y. Q. (2015). The solubility and conformational characteristics of porcine myosin as affected by the presence of L-lysine and L-histidine. Food Chemistry, 170, 212–217. https://doi.org/https://doi.org/10.1016/j.foodchem.2014.08.045
- Huang, H., Larsen, M. R., & Lametsch, R. (2012). Changes in phosphorylation of myofibrillar proteins during postmortem development of porcine muscle. Food Chemistry, 134(4), 1999–2006. https://doi.org/https://doi.org/10.1016/j.foodchem.2012.03.132
- Immonen, K., & Puolanne, E. (2000). Variation of residual glycogen-glucose concentration at ultimate pH values below 5.75. Meat Science, 55(3), 279–283. https://doi.org/https://doi.org/10.1016/S0309-1740(99)00152-7
- Kwasiborski, A., Sayd, T., Chambon, C., Santé-Lhoutellier, V., Rocha, D., & Terlouw, C. (2008). Pig Longissimus lumborum proteome: Part II: Relationships between protein content and meat quality. Meat Science, 80(4), 982–996. https://doi.org/https://doi.org/10.1016/j.meatsci.2008.04.032
- Lee, Y. S., & Landry, A. B. (1992). Regulation of Ca2+ release from sarcoplasmic reticulum in skeletal muscles. Molecular and Cellular Biochemistry, 114(1–2), 105–108. https://doi.org/https://doi.org/10.1007/BF00240304
- Li, C., Zhou, G., Xu, X., Lundström, K., Karlsson, A., & Lametsch, R. (2015). Phosphoproteome analysis of sarcoplasmic and myofibrillar proteins in bovine longissimus muscle in response to postmortem electrical stimulation. Food Chemistry, 175, 197–202. https://doi.org/https://doi.org/10.1016/j.foodchem.2014.11.139
- Li, Z., Xin, L., Xing, G., Shen, Q. W., & Zhang, D. (2017). Phosphorylation prevents in vitro myofibrillar proteins degradation by μ-calpain. Food Chemistry, 218, 455–462. https://doi.org/https://doi.org/10.1016/j.foodchem.2016.09.048
- Liu, M., Wei, Y., Li, X., Quek, S. Y., Zhao, J., Zhong, H., Zhang, D., & Liu, Y. (2018). Quantitative phosphoproteomic analysis of caprine muscle with high and low meat quality. Meat Science, 141, 103–111. https://doi.org/https://doi.org/10.1016/j.meatsci.2018.01.001
- Meek, D. W., & Nimmo, H. G. (1984). Effects of phosphorylation on the kinetic properties of rat liver fructose-1, 6-bisphosphatase. Biochemical Journal, 222(1), 125–130. https://doi.org/https://doi.org/10.1042/bj2220125
- Meng, L. A., Xin, L. A., Jx, A., Zheng, L. A., Gl, A., & Yan, Z. A. (2017). Effects of protein phosphorylation on color stability of ground meat. Food Chemistry, 219, 304–310. https://doi.org/https://doi.org/10.1016/j.foodchem.2016.09.151
- Muroya, S., Ohnishi-Kameyama, M., Oe, M., Nakajima, I., Shibata, M., & Chikuni, K. (2007). Double phosphorylation of the myosin regulatory light chain during rigor mortis of bovine longissimus muscle. Journal of Agricultural and Food Chemistry, 55(10), 3998–4004. https://doi.org/https://doi.org/10.1021/jf063200o
- Perlo, F., Bonato, P., Fabre, R., Teira, G., & Tisocco, O. (2012). Combined effect of electrical stimulation, aging time and marination on quality of chicken breast fillet processed under commercial conditions. Journal of the Science of Food and Agriculture, 92(10), 2183–2187. https://doi.org/https://doi.org/10.1042/bj2220125
- Pratje, E., & Heilmeyer, L. M. G. (1972). Phosphorylation of rabbit muscle troponin and actin by a 3′, 5′‐c‐AMP‐dependent protein kinase. FEBS Letters, 27(1), 89–93. https://doi.org/https://doi.org/10.1016/0014-5793(72)80416-2
- Rardin, M. J., Wiley, S. E., Naviaux, R. K., Murphy, A. N., & Dixon, J. E. (2009). Monitoring phosphorylation of the pyruvate dehydrogenase complex. Analytical Biochemistry, 389(2), 157–164. https://doi.org/https://doi.org/10.1016/j.ab.2009.03.040
- Ravindran, S., Radke, G. A., Guest, J. R., & Roche, T. E. (1996). Lipoyl domain-based mechanism for the integrated feedback control of the pyruvate dehydrogenase complex by enhancement of pyruvate dehydrogenase kinase activity. Journal of Biological Chemistry, 271(2), 653–662. https://doi.org/https://doi.org/10.1074/jbc.271.2.653
- Sharma, R. K. (1991). Phosphorylation and characterization of bovine heart calmodulin-dependent phosphodiesterase. Biochemistry, 30(24), 5963–5968. https://doi.org/https://doi.org/10.1021/bi00238a021
- Stull, J. T., Kamm, K. E., & Vandenboom, R. (2011). Myosin light chain kinase and the role of myosin light chain phosphorylation in skeletal muscle. Archives of Biochemistry and Biophysics, 510(2), 120–128. https://doi.org/https://doi.org/10.1016/j.abb.2011.01.017
- Tahergorabi, R., Beamer, S. K., Matak, K. E., & Jaczynski, J. (2012). Salt substitution in surimi seafood and its effects on instrumental quality attributes. LWT-Food Science and Technology, 48(2), 175–181. https://doi.org/https://doi.org/10.1016/j.lwt.2012.03.004
- Wang, S., Li, C., Xu, X., & Zhou, G. (2013). Effect of fasting on energy metabolism and tenderizing enzymes in chicken breast muscle early postmortem. Meat Science, 93(4), 865–872. https://doi.org/https://doi.org/10.1016/j.meatsci.2012.11.053
- Wang, Z., Zhang, C., Li, Z., Shen, Q., & Zhang, D. (2017). Comparative analysis of muscle phosphoproteome induced by salt curing. Meat Science, 133, 19–25. https://doi.org/https://doi.org/10.1016/j.meatsci.2017.05.018
- Zeniya, M., Sohara, E., Kita, S., Iwamoto, T., Susa, K., Mori, T., Oi, K., Chiga, M., Takahashi, D., Yang, -S.-S., Lin, S.-H., Rai, T., Sasaki, S., & Uchida, S. (2013). Dietary salt intake regulates WNK3–SPAK–NKCC1 phosphorylation cascade in mouse aorta through angiotensin II. Hypertension, 62(5), 872–878. https://doi.org/https://doi.org/10.1161/HYPERTENSIONAHA.113.01543
- Zhang, C., Wang, Z., Li, Z., Shen, Q., Chen, L., Gao, L., & Zhang, D. (2016). Phosphoproteomic profiling of myofibrillar and sarcoplasmic proteins of muscle in response to salting. Food Science and Biotechnology, 25(4), 993–1001. https://doi.org/https://doi.org/10.1007/s10068-016-0161-0
- Zhang, Y., Cheng, Q., Yao, Y., Guo, X., Wang, R., & Peng, Z. (2014). A preliminary study: Saltiness and sodium content of aqueous extracts from plants and marine animal shells. European Food Research and Technology, 238(4), 565–571. https://doi.org/https://doi.org/10.1007/s00217-013-2136-1
- Zhang, Y., Guo, X., Liu, T., & Peng, Z. (2018). Effects of substitution of NaCl with KCl, L-histidine, and L-lysine on instrumental quality attributes of cured and cooked pork loin. CyTA-Journal of Food, 16(1), 877–883. https://doi.org/https://doi.org/10.1080/19476337.2018.1493538
- Zhang, Y., Wu, J., Jamali, M. A., Guo, X., & Peng, Z. (2017). Heat-induced gel properties of porcine myosin in a sodium chloride solution containing l-lysine and l-histidine. LWT-Food Science and Technology, 85, 16–21. https://doi.org/https://doi.org/10.1016/j.lwt.2017.06.059
- Zhou, C., Li, J., & Tan, S. (2014). Effect of L-lysine on the physicochemical properties of pork sausage. Food Science and Biotechnology, 23(3), 775–780. https://doi.org/https://doi.org/10.1007/s10068-014-0104-6