Abstract
This paper details the results of a series of tests whose main purpose was to determinate how osmotic drying influences both water loss and color retention in cactus pear fruits. The dehydration process was performed using a 32 experimental design which involved three different sugar concentration levels (40, 50, and 608Brix) and three different temperatures (25, 40, and 558C). A product/solution ratio of 1/15, cactus pear cylindrical slices 5 mm thick, and ten-hour immersion time spans were used for all the experiments. Modeling of the drying curves was done with Fick's second law and Page's equation, which were used to calculate water effective diffusivity (De), drying constant (k), and the b values respectively. The color parameters (L*, a*, b*) of the samples were measured before and after each test to evaluate total color change (ΔE). An analysis of variance (ANOVA) revealed that water diffusivity and Page's parameter b were affected by temperature and sugar concentration while the drying constant (k) was independent of the drying conditions. The total color change was affected only by the temperature. De and b values varied from 4.06×10−11 to 2.95×10−10 m2/s, and from 0.453 to 0.861 respectively. The best color retention was obtained at 25°C independent of the sugar concentration.
INTRODUCTION
Native to the Continent of America prickly pear, or cactus pear, is a tropical fruit that grows in areas with arid or semi-arid climate.[Citation1] Many different species, wild and domesticated, grown in Mexico (Opuntia spp). This fruit is known as “tuna” in Mexico. A cactus pear is a berry covered by a semi-hard peel; it has a very tasty pulp which is full of seeds. This fruit has a similar composition to other fruits, consisting of approximately 85 % water, 14 % sugar, and around 1 % of protein.[Citation2,Citation3] In Mexico, tunas are usually eaten as fresh fruit or in the form of traditional confections and drinks such as tuna cheese, tuna honey, jams, alcoholic drinks, and fermented juice.[Citation1,Citation4] Because of its ascorbic acid content (up to 40 mg/100 g), as well as its fiber and amino acids, this crop has great commercial possibilities.Citation5] Currently, Mexico's tuna production (about 200,000 ton/year) accounts for two thirds of the world's; the remainder is produced by USA, Italy, Spain, Egypt, Morocco, and Israel.[Citation4] Nevertheless, the lack of adequate methods of preservation of cactus pear after they have been harvested, causes storage and transportation to be difficult and, consequently, only a small fraction of Mexico's yearly production reaches the international market.[Citation4] Then, it has been estimated, that more than 60 % of the fruit remains unharvested.
Although more research is still required, partial or full drying of cactus pear seems to offer an adequate way to deal with this situation. More specifically, osmotic dehydration represents a good choice as a pre-treatment method to obtain cactus pears of intermediate moisture content that either can be consumed as is used in food formulations. The method consists in partially removing water from the product by immersing it in aqueous solutions of sugar, salt and spices.[Citation6,Citation7] Additionally, a complementary treatment such as conventional drying, freeze-drying, vacuum drying, or pasteurisation would be necessary in order to provide shelf stability to the product.[Citation8,Citation9] Several authors,[Citation10–12] have reported that the application of an osmotic treatment before a conventional drying has improved texture, flavor, vitamin retention, and color stability in dried fruit and vegetables. This is specially important since color may be a decisive factor in the consumer's acceptance of a food.[Citation13,Citation14] Although abundant information is available on how osmotic drying affects the quality of several fruits and vegetables, little or no literature has been published about the application to cactus pear fruits. Therefore, the present study was performed as a pretreatment method to evaluate the effect of the temperature and sucrose concentration of the osmotic solution on water transfer and on the color retention during the osmotic dehydration of cactus pear.
MATERIALS AND METHODS
Raw Material and Osmotic Drying
Cactus pear fruits (variety Opuntia ficus indica) with an average weight of 100 to 110 g and having a similar degree of maturity and commercial sucrose were purchased at a local market. The fruits were carefully washed with water to eliminate the spines from the peel, then hand-peeled and sliced. Six to seven non-seeded slices 5 mm thick were obtained from the middle portion of each fruit, making the cuts perpendicular to the axis of symmetry. In this way, all the slices were cylindrical and approximately equal in diameter (approximately 49 mm). Once the samples had been prepared, they were submerged in a 2000 ml beaker containing about 1500 ml of the osmotic solution to which 2% calcium chloride was added in order to improve firmness in the fruit. Afterwards, the beaker was placed in a large water bath with controlled temperature. A 32 experimental design was used for the osmotic solution, three levels of sugar concentration (40, 50 and 60 °Brix), and three different temperatures (25, 40 and 55 °C). Ten-hour immersion periods under static conditions and a product/solution ratio of 1/15 were used in all the experiments. The samples were taken out of the solution every hour to register weight reduction. All tests were done in duplicate and a total of 18 runs were conducted ().
Table 1 Experimental conditions with Fick's and Page's equations, parameters, and their respective r2 and RMSE values.
Moisture Content and Color Measurements
The initial moisture content of the samples was determined gravimetrically by drying about 2 g of material in a forced convection oven at 104 °C until a constant weight was reached. The value in this way was 9.23 ± 0.72 kg water/kg dry basis (corresponding to 90.2% wet basis). The color parameters (L*, a* and b* Hunter values) of fresh and dried samples were determined by a colorimeter (AccuProbe HH06TM, Accuracy Microsensors, Inc. Pittsford, New York USA) equipped with a circular measuring area 8 mm in diameter. The instrument was calibrated with a white ceramic plate (L*= 99.99, a*= 0.00, b=1.81). Color measurements were done as follows: each slice was scanned at six different points of its surface; then the average value was obtained. Total color change (ΔE) was defined as:
where L o *, a o * and b o * represent the color parameters before the process, and L *, a * and b * are the color parameters after the drying process.
Mathematical Modeling of the Drying Curves
Moisture content (X) was transformed to a dimensionless form referred to as “moisture ratio” (X * ) and then related to the time to derive the drying curves. The drying curves were analyzed in similar fashion to that performed by Park et al.[Citation15] and El-Aouar et al.[Citation16] and consisted in the application of Fick's second law of diffusion, expressed for slab geometry as:
as well as Page's model, given by:
where: X(t),Xo, and Xe are moisture content (kg water/ kg dry matter) at any time, t= 0, and t = ∞, respectively. De is water effective diffusivity (m2/s), Lis half the thickness of the slice (m), k is the drying constant (min−1), which is a phenomenological property representative of heat and mass transport phenomena, b is the dimensionless model parameter and t is the process time (s). In applying Equation (2), it was assumed that a) mass transfer takes place in one direction, b) the initial moisture content and soluble solids of the product are uniform, c) the surface of each slice has already reached moisture equilibrium, d) De is constant e) slice shrinkage is negligible, f) the product/solution ratio is sufficiently high and the syrup concentration can be considered constant, and g) there is no resistance at the surface and a sugar solution film is assumed to exist as a boundary layer. Equation (3) is a modified version of the model by Lewis, which has been widely used to fit drying kinetic of various agricultural products.[Citation17] A non-linear procedure was used to adjust the experimental data for the two models. De was calculated with the first ten terms of the Fourier series in equation (2). The rate of change of moisture (−dX/dt), determined from experimental data was plotted against X, and equilibrium moisture content values (Xe) were obtained from these plots. The coefficient of determination (r2) and the root mean square error analysis (RMSE)[Citation18] were used as the primary criteria to determine which model was more suitable to describe the drying process studied.
Statistical Analyses
Analysis of variance (ANOVA) was done with Modde 7.0 software (by Umetrics Inc). The multiple regression method was used to adjust a quadratic model (Eq. 4) to the experimental data with a confidence interval of 95 %:
where: y = values of water effective diffusivity (De ), drying constant (k), Page's parameter (b) or total color change (ΔΕ); T= temperature (25, 40, and 55 °C); C= sugar concentration (40, 50, and 60 °Brix); β0, β1, β2, and β3 are the regression coefficients of the model.
RESULTS AND DISCUSSION
Moisture Ratio : Experimental and Predicted Values
The experimental values of moisture ratio during osmotic drying of cylindrical slices of cactus pear, at several temperatures and several concentrations, are shown in and Fig. , respectively. The shape of these curves are typical of food products whose drying process is controlled by internal water transfer.
Figure 1 Evolution of experimental moisture ratio and predicted moisture ratio (with Fick's Eq.) with drying time for osmotic dehydration performed at several temperatures.
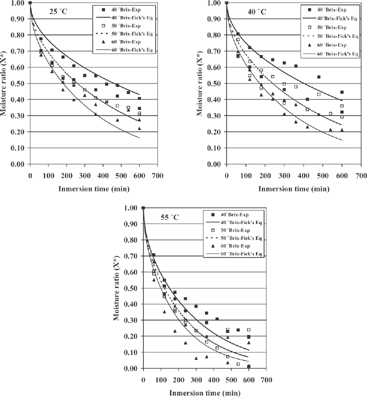
Figure 2 Evolution of experimental moisture ratio and predicted moisture ratio (with Page's Eq.) with drying time for osmotic dehydration performed at several concentrations.
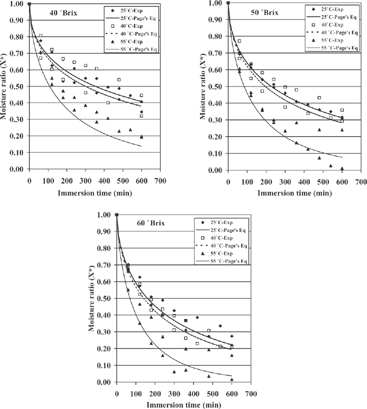
From the observations of and Fig. , it is clear that both the amount of sugar present in the solution and the temperature applied directly influenced moisture loss since, generally speaking, increments in temperature or sucrose concentration normally provoked increments in water loss. According to the literature,[Citation6,Citation7,Citation10] higher sugar concentration in the solution increases the osmotic pressure between the solution and the fruit, consequently making moisture loss higher. Additionally, the application of high temperatures results in a higher solubility of sugar and in a change of the permeability properties of the cellular membrane, which in turn increases mass transfer.Citation19] However, it should be pointed out that the amount of water loss by the samples dried at 40° C was not significantly different (p < 0.05) from that of the samples dried at 25° C. Actually, the experimental curves for 25 and 40° C are practically the same in all three cases (as discussed later), regardless sugar concentration (). A similar phenomenon at temperatures between 25 and 35° C has been reported by Ade-Omowaye et al.,[Citation20] who worked on the osmo-dehydration of bell peppers.
also contains the curves predicted with Fick's equation and the corresponding curves calculated with Page's equation. A good agreement between experimental and predicted values of moisture ratio with r2 values higher than 0.9 was observed. The statistic r2 and the RMSE values for each experimental condition investigated as well as the k, band De values calculated with Page's and Fick's equations are also given in . The results in have shown that the highest values of r2 and the lowest values of RMSE were obtained with the Page's equation. Therefore, theoretically the best model describing the osmotic drying of cactus pear could be Page's equation.
also shows that the effective diffusivity of moisture varied from 4.06×10−11 to 2.95×10−10 m2/s over the sucrose concentration and temperature range studied. This order of magnitude is ten times less than the recompilation reported by Maroulis et al.[Citation21] for water diffusivity in biological products, but close to that reported by Park et al.[Citation15] for osmo-dehydrated pears. The values calculated for the drying constant k (min−1) and the coefficient b are analogous to those reported for osmotic drying of D'anjou[Citation15] pear and papaya,[Citation16] although, kvalues differ from the ones obtained by Krokida et al.[Citation17] This may have been caused by differences in experimental conditions and/or the drying method used.
Further regressions were undertake to identify the effect of the temperature and sugar concentration on the De , k, and b values using the quadratic model, Eq. (4), with a confidence interval of 95%. The regression coefficients for the model and their respective analyses of variance (ANOVA) are shown in . It can be observed that the water effective diffusivity and Page's parameter b were influenced by the temperature and by the sugar concentration. also shows that, according to the lowest p(t) values, the temperature was the most important process variable affecting the water effective diffusivity and parameter b.
With respect to drying constant (k), significant effect of the drying variables was not observed (). The drying constant did not exhibit a clear trend with an increase in sugar concentration and temperature. On the other hand, the experimental data were better fitted with Page's equation, but this model based on the drying constant was the less appropriate that Fick's equation to describe the effect of the drying conditions during the osmotic dehydration of cactus pear. Moreover, the drying constant describes simultaneously the mechanism of heat and mass transport, while that De describe only the transfer of moisture inside the material. This could explain why k was less sensitive than De to the effect of the experimental conditions tested in this work. The mass transfer model for relating the water effective diffusivity and parameter b in the cactus pear to the temperature and the sugar concentration are given by the following equations:
Table 2 Regression coefficients and variance analysis of the quadratic model Eq. (4) to evaluate the effect of temperature and sucrose concentration on the De , k and b values (p<0.05). The bold character indicates that the corresponding parameter has a significant effect on the properties evaluated.
where T and C are the temperature and concentration, respectively, standardized between –1, 0, 1 in both cases. depicts De versus temperature for the all sugar concentrations studied. There was a significant quadratic and linear effect of the temperature on the values of water effective diffusivity. In , a linear relationship between De and sugar concentration was found. In general, De increased as sucrose concentration and/or temperature increased, although the effect of the temperature is small on the water diffusivity for the temperature interval between 25 and 40° C (). Nevertheless, independent of the sugar concentration, the predicted values of De for the samples dried at 55° C were at least 1.8 to 3 times greater than those obtained at 25° C or 40° C.
Figure 3 Variation of water effective diffusivity (De ) with temperature (a) and sugar concentration (b) in cactus pear during osmotic treatment.
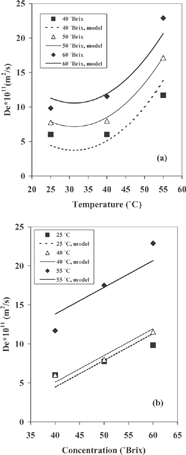
The values of b versus the temperature and sugar concentration were also plotted in . The plot shows that b increased with increasing temperature and concentration and presented a similar tendency that water diffusivity; b was found to have quadratic dependence with temperature and linear dependence with concentration. also shows that b reached its maximum at 55° C- 60 °Brix, and its minimum at 25° C-40 °Brix.
Figure 4 Variation of parameter b with temperature (a) and sugar concentration (b) in cactus pear during osmotic treatment.
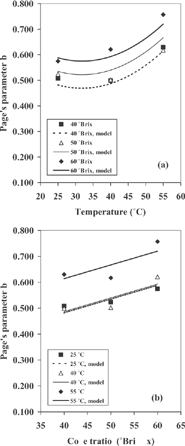
, shows that the drying constant was independent of the temperature and the sucrose concentration. In this case, an average k value of 0.038 could be used in equation (3) to estimate the osmotic drying curves of cactus pear.
Color Retention
Appearance, which is significantly impacted by color, is one of the first attributes used by consumer for acceptability of a food. The data in represent the color parameters (L*, a*, and b*) as well as the total color change (ΔE) values for the fresh and osmodehydrated samples. It is important to indicate that the values of color in fresh fruit represents the mean of the fresh samples used in all the experiments. The appearance of browning in food seems to be directly related to lower values for L.*[Citation13,Citation14,Citation22] This Table shows that the L* values for the osmodehydrated samples were lower that the obtained ones for untreated samples (except in Exp. 7). The scattered data obtained for a* and b* valuès not allowed to have some tendency, therefore, this suggest that the changes in ΔL* were more important and turned out to be the actual determiner of the overall color change. These observations are agrees with that obtained by Tan et al.,Citation23] in their experiments conducted with potato and pineapple. However, a statistical analysi based on the total color change (ΔE) was carried out, using the same procedure and the same model (Eq. 4) utilized for the analysis of De, k, and b. The results of this statistical analysis are given in . This analyses shows that color change was only influenced by the temperature (p<0.05), and no significant effect of the concentration was detected. The experimental and predicted values (with Eq. 4 and coefficients of table 4) of ΔE can be observed in . An analysis of this figure demonstrates that temperature was the strongest factor affecting color, because ΔE augmented as the temperature was raised, reaching a maximum value between 40 and 50° C. This can be attributed to the enzymatic browning, which is one of the most important color reactions that affect fruits and vegetables. Browning is catalyzed by the enzyme polyphenol oxidase (PPO), which is present in fruits with a low value of acidity,[Citation24] and this could be the case with the cactus pear (0.175 % titrable acidity). Additional experiments to determine the concentration of PPO in cactus pear fruit are necessary to confirm this assumption. Nevertheless, the model demonstrates progressive decrease in ΔE values for temperatures above 50° C. At this temperature, there is probably an inactivation of the enzyme PPO. This enzyme is relatively heat labile, and it is feasible that their inactivation by applying temperatures above 50° C.[Citation25] In general, the experiments conducted at 25° C independent of sugar concentration, rendered the lowest ΔE values.
Table 3 Color measurement results in L*a*b* and total color changes (ΔE) for fresh and osmo-pretreated cactus pear.
Table 4 Regression coefficients and variance analysis of the quadratic model (Eq. 4) to evaluate the effect of temperature and sucrose concentration on the total color change (p<0.05). The bold character indicates that the corresponding parameter has a significant effect on the properties evaluated.
CONCLUSIONS
The drying curves of the cactus pear were satisfactorily described by the mathematical models based on Page's Equation and Fick's second law. However, based on the parameters of these models, De has been shown to be more effective that the drying constant to evaluate the effect of the temperature and sugar concentration. In every case when Page's equation was used, the effect of the drying conditions could be explained by the dimensionless parameter b. The water effective diffusivity varied from 4.06×10−11 to 2.95×10−10 m2/s and b from 0.453 to 0.861. The values of De and b showed a quadratic and a linear relationship with temperature and sugar concentration, respectively. With respect to total color change, the statistical analysis of the results showed that temperature was the strongest factor affecting color and ΔE derives principally from ΔL values. The experimental condition conducted at 25° C yielded the best color retention independently of the sugar concentration.
ACKNOWLEDGEMENTS
This research was carried out with the financial support of FAI-UASLP (Project C02-FAI-10-17.57). The authors would like to thank O. Beltrán for the revision of the English version.
REFERENCES
- Saenz , C. 2000 . Processing technologies: an alternative for cactus pear (Opuntia spp.) fruits and cladodes . Journal of Arid Environments , 46 : 209 – 225 .
- Gurbachan , S. and Felker , P. 1998 . Cactus: New world foods . Indian Horticulture , 43 ( 1 ) : 29 – 31 .
- Yasseen , Y.M. , Barringer , S.A. and Splittstoesser , W.E. 1996 . A note on the uses of Opuntia spp in Central/North America . Journal of Arid Environments , 32 : 347 – 353 . [CROSSREF]
- Flores-Valdez , C.A. , Luna-Esquivel , J. M. and Ramírez-Moreno , P. P. 1995 . Mercado mundial de la tuna. In Reporte de la ASERCA-UACH-CIESTAAM 173 México
- Stintzing , F.C. , Schieber , A. and Carle , R. 2001 . Phytochemical and nutritional significance of cactus pear . Food Research and Technology International , 212 : 396 – 407 .
- Sablani , S.S. and Rahman , M.S. 2003 . Effect of syrup concentration, temperature and sample geometry on equilibrium distribution coefficients during osmotic dehydration of mango . Food Research International , 36 : 65 – 71 . [CROSSREF]
- Sablani , S.S. , Rahman , M.S. and Al-Sadeiri , D.S. 2002 . Equilibrium distribution data for osmotic drying of apple cubes in sugar-water solution . Journal of Food Engineering , 52 : 193 – 199 . [CROSSREF]
- Mendoza , R. and Schmalko , M.E. 2002 . Diffusion coefficients of water and sucrose in osmotic dehydration of papaya . International Journal of Food Properties , 5 ( 3 ) : 537 – 546 . [CROSSREF]
- Torringa , E. , Lourenco , F. , Schewewe , I. and Bartels , P. 2001 . “ Application of microwave drying after osmotic dehydration: effect of dielectric properties on heating characteristics ” . In Osmotic Dehydration and Vacuum Impregnation: Application in Food Industries , 1 st , 217 – 224 . Lancaster-Basel, U.S.A : Technomic Publishing Company, Inc. .
- Nieuwenhuijzen , N.H. , Zareifard , M.R. and Ramaswamy , H.S. 2001 . Osmotic drying kinetics of cylindrical apple slices of different sizes . Drying Technology , 19 ( 3–4 ) : 525 – 545 . [CROSSREF]
- Krokida , M.K. , Karathanos , V.T. and Maroulis , Z.B. 2000 . Effect of osmotic dehydration on color and sorption characteristics of apple and banana . Drying Technology , 18 ( 4–5 ) : 937 – 950 .
- Krokida , M.K. , Kiranoudis , C.T. , Maroulis , Z.B. and Marinos-Kouris , D. 2000 . Effect of pretreatment on color of dehydrated products . Drying Technology , 18 ( 6 ) : 1239 – 1250 .
- Koyuncu , T. , Tosun , I. and Ustun , N.S. 2003 . Drying kinetics and color retention of dehydrated rosehips . Drying Technology , 21 ( 7 ) : 1369 – 1381 . [CROSSREF]
- Singh-Gujral , H. and Singh-Brar , S. 2003 . Effect of hydrocolloids on the dehydration kinetics,color, and texture of mango leather . International Journal of Food Properties , 6 ( 2 ) : 269 – 279 . [CROSSREF]
- Park , K.J. , Bin , A. , Brod , F.P.R. and Brandini-Park , T.H. 2002 . Osmotic dehydration kinetics of pear D'anjou (Pyrus communis L) . Journal of Food Engineering , 52 : 293 – 298 . [CROSSREF]
- El-Aouar , A.A. , Azoubel , P.M. and Murr , F.E. X. 2003 . Drying kinetics of fresh and osmotically pre-treated papaya (Carica papaya L) . Journal of Food Engineering , 59 ( 1 ) : 85 – 91 . [CROSSREF]
- Krokida , M.K. , Foundoukidis , E. and Maroulis , Z. 2004 . Drying constant: literature data compilation for foodstuffs . Journal of Food Engineering , 61 : 321 – 330 . [CROSSREF]
- Togrul , T.I. and Pehlivan , D. 2003 . Modelling of drying kinetics of single apricot . Journal of Food Engineering , 58 : 23 – 32 . [CROSSREF]
- Saputra , D. 2001 . Osmotic dehydration of pineapple . Drying Technology , 19 ( 2 ) : 415 – 425 . [CROSSREF]
- Ade-Omowaye , B.I.O. , Rastogi , N.K. , Angersbach , A. and Knorr , D. 2002 . Osmotic dehydration of bell peppers: Influence of high intensity electric field pulses and elevated temperature treatment . Journal of Food Engineering , 54 : 35 – 43 . [CROSSREF]
- Maroulis , Z.B. , Saravacos , G.D. , Panagiotou , N.M. and Krokida , M.K. 2001 . Moisture diffusivity data compilation for foodstuffs: Effect of material moisture content and temperature . International Journal of Food Properties , 4 ( 2 ) : 225 – 237 . [CROSSREF]
- Krokida , M.K. , Maroulis , Z.B. and Saravacos , G.D. 2001 . The effect of the method of drying on the colour of dehydrated products . International Journal of Food Science and Technology , 36 : 53 – 59 . [CROSSREF]
- Tan , M. , Chua , K.J. , Mujumdar , A.S. and Chou , S.K. 2001 . Effect of osmotic pre-treatment and infrared radiation on drying rate and color changes during drying of potato and pineapple . Drying Technology , 19 ( 9 ) : 2193 – 2207 . [CROSSREF]
- Badui , S. 1993 . “ Enzimas ” . In Qui´mica de los alimentos , 279 – 326 . México : Addison Wesley Longman .
- Martinez , M.V. and Whitaker , J.R. 1995 . The biochemistry and control of enzymatic browning . Trends in Food Science and Technology , 6 ( 6 ) : 195 – 200 . [CROSSREF]