Abstract
In construction, the mirroring of knowledge with tasks increasingly limits firm-level abilities to identify and pursue systemic innovation opportunities. Recent research identifies how integration strategies enable individual projects to break from this trap, but much less is known about potential strategic action of firms to enable systemic innovation. To explore this, a longitudinal comparative case study examines how three entrepreneurial firms – DPR construction, RAD Urban, and Project Frog – in the San Francisco Bay Area describe their own strategic evolution and restructuring of firm boundaries over the past five years to enable greater adoption of digital manufacturing. Each firm has developed a different approach – relational, project-based spinoff; vertical integration; or digital systems integration. Benefits and challenges are identified for each. The approaches are theorized as a form of strategic mirror-breaking intended to redefine the current paradigm of knowledge and task dependencies. They enable the firms to develop products with new system architectures and access more opportunities for innovation in digitally-enabled manufacturing. The paper concludes with discussion of how the identification and characterization of strategies for mirror-breaking enriches understanding of integration for systemic innovation in construction at the firm level. The paper identifies common themes for digitally-enabled manufacturing including the limitations of the current industry structure, the emergence of new hybrid positions, and the opportunity for platforms to provide longitudinal integration.
Introduction
Construction needs to change, but systemic innovation is difficult. As a mature industry, construction has become fragmented vertically, with separate parts of the production processes involving specialized firms; horizontally, with many firms striving to compete; and longitudinally, with relationships between suppliers and clients infrequently sustained across projects (Howard et al. Citation1989; Fergusson and Teicholz Citation1996). Fragmentation presents a “never-ending stream of problems that require local incremental innovations” (Levitt Citation2007, p. 624), hence the potential for “systemic” innovations are usually passed over for localized product innovations that offer less global benefit but fit within the existing modules of work and specialization (Katila et al. Citation2018).
This Festschrift paper is motivated by Levitt’s (Citation2007) call to seek understanding of potential future “disruptive paradigms” for the construction industry. We begin from the premise that the North American construction industry structure is not well suited for the implementation of systemic innovations (Taylor and Levitt Citation2007; Katila et al. Citation2018). Scholars have identified barriers to the adoption of systemic innovations, including demand fluctuations, liability concerns, industry fragmentation and broken feedback loops (Sheffer Citation2011). We suggest such norms, standards, and regulations have institutionalized over time (Henisz et al. Citation2012) until the construction industry has found itself caught in a “mirroring trap” (Colfer and Baldwin Citation2016). In such a trap, the knowledge about design, engineering and construction are deeply embedded in specialty firms and their employees’ individual actions. In other words, knowledge about tasks has become tightly aligned with the task dependencies themselves. This “strict mirroring” between knowledge and work tasks traps project design and execution into the prevailing standard system architecture and resists attempts to innovate at the system level (Taylor and Levitt Citation2004, Citation2007; Levitt and Sheffer Citation2011; Katila et al. Citation2018). If the construction industry is indeed stuck in a mirroring trap, one implication is that firms and project teams will be unable or unwilling to take up systemic innovations that offer global benefits but do not align with the prevailing industry structure, project organization and knowledge boundaries.
This paper seeks to identify strategies that construction industry firms can use to break out of the mirroring trap. Existing project scholarship suggests that formal and informal integration strategies can help project teams develop systems-level knowledge to address horizontal and vertical fragmentation and break free from some of the restrictions of the mirroring trap (Bygballe et al. Citation2014; Hall et al. Citation2018). However, this work has emphasized project-based innovation; less construction innovation scholarship has analyzed systemic innovation attempts by firms. There is a need to understand how construction firms can take strategic action over a period of time to “break the mirror” of knowledge and tasks. If successful, this can result in the creation or adoption of new integrated products and/or new system-level architectures.
To understand how this can be done, we look at the adoption of digitally-enabled manufacturing – an emerging systemic innovation in the industry (Lavikka et al. Citation2018). We use digitally-enabled manufacturing to refer to forms of industrialized construction, off-site fabrication, prefabrication, and/or modular construction that contains a digital thread connecting design and construction (Bock and Linner Citation2015). Digitally-enabled manufacturing can create opportunities for new business models that emphasize integrated product-orientation (Lessing and Brege Citation2015). For example, business models for digital manufacturing might take the scope of work of an interior wall system (e.g. the wall framing, electrical system, insulation, drywall and painting) performed by distinct trades and replace it with an integrated product design such as a prefabricated partition wall panel. This is the model used by the company DIRTT1. DIRTT claims to reduce construction cost by up to 50% versus conventional approaches (Ellen MacArthur Foundation Citation2017). As we will later describe, these new products can redefine or require new system architecture. At scale, they cross the existing boundaries of the industry structure and/or require coordinated action in the supply chain for effective implementation.
To develop the contribution, we first articulate the constructs of industry fragmentation, systemic innovation, the mirroring trap, and digitally-enabled manufacturing. We then introduce our research design: a longitudinal comparative case analysis of three construction firms founded in the San Francisco Bay Area. The three firms – DPR Construction, RAD Urban, and Project Frog – can be considered current leaders in the US market for developing new digital manufacturing capabilities. Data was first collected in 2014; we returned again to the same firms in 2019 to understand how their strategic approaches have evolved over the past five years. From the case data, we find participants describe a different strategic approach with distinct benefits and challenges from each of the three firms. The three approaches are 1) spinoff from project-based firm, 2) vertical integration, and 3) digital systems integration. We suggest and describe how each approach can be understood as a strategy for mirror-breaking. By breaking the mirror, the three firms expand knowledge boundaries beyond competitors in the industry. They are able to develop new integrated products within the context of digital manufacturing. Furthermore, we describe how RAD Urban and Project Frog develop entirely new system architectures and – though the use of platforms – enable longitudinal continuity to capture further value from digital manufacturing.
Construction industry structure
The construction industry has long been organized through trilateral governance (Reve and Levitt Citation1984) where a specialized third party (e.g. design firm) assists the project sponsor in completing a transaction. Reve and Levitt (Citation1984) noted the resulting construction industry resembled neither a market nor hierarchy but instead a quasifirm (Eccles Citation1981) or clan relationship. Later scholars formalized this type of organizational form as a network industry structure (Powell Citation1990), citing the construction industry as a prime example. The construction industry network structure is characterized by firms organizing around projects. This has led to extreme fragmentation between stakeholders (Fergusson and Teicholz Citation1996). Vertical fragmentation occurs between project phases (Howard et al. Citation1989). Each phase can have a different set of stakeholders, decision-makers and values. This creates displaced agency – also called “broken agency”– where involved parties will engage in self-interested behavior and pass costs off to stakeholders in a subsequent phase to the detriment of the long-term user (Henisz et al. Citation2012). Horizontal fragmentation occurs between specialists within any given project phase (Howard et al. Citation1989). It is reinforced by the trade-by-trade competitive bidding environment of traditional project deliveries. Longitudinal fragmentation from project to project (Fergusson and Teicholz Citation1996) also occurs in North America and other liberal market economies. Project teams disband at the end of projects and are unlikely to work with the same set of partner firms on future projects. Consequently, team members lose tacit knowledge about how to work together effectively and organizations are unable to build upon ideas accumulated during the project (Dubois and Gadde Citation2002a).
Resistance to systemic innovations in construction
The process of product innovation is well established as important to the health of the construction industry (Nam and Tatum Citation1989). Thus, scholars have attempted to understand what makes it happen in the way that it does in construction. While we recognize that many contributions have been made in this area (e.g. Slaughter Citation1998; Harty Citation2005; Reichstein et al Citation2008; Toole et al. Citation2013; Lindgren Citation2016; Ozorhon et al. Citation2016), we here emphasize the contributions led by Levitt on the relationship between product innovation and construction industry structure. This work also draws on the idea of a dominant system architecture for complex products (Henderson and Clark Citation1990) which then becomes institutionalized within the industry structure.
Scholars have debated whether project-based interorganizational networks – such as those found in the construction industry structure – are loci of innovation and learning (Powell Citation1990) or fall prey to pathways of “evolutionary stagnation” (Lampel and Shamsie Citation2003; Taylor and Levitt Citation2007; Lampel Citation2011). Taylor and Levitt (Citation2004) argue that this is highly dependent on the nature of innovation. The term systemic innovations was introduced by Taylor and Levitt (Citation2004) to refer to construction product innovations that are misaligned with institutionalized project supply chains and system architectures. Systemic innovations reinforce the overall product function. In other words, the core design concept remains the same. However, systemic innovations redefine the boundaries between the units of work traditionally provided by each firm in the supply chain. They require multiple firms in the supply chain network to change their design, prefabrication and/or assembly practices in a coordinated way (Taylor and Levitt Citation2004). They alter the interfaces between individual components or the overall system architecture (Katila et al. Citation2018). Systemic innovations can create increased overall product value or productivity gains. However, they typically induce switching or start-up costs for some participants while reducing or potentially eliminating the role of other participants (Hall et al. Citation2018). Thus, they are resisted by the dominant system architecture established and institutionalized within the industry (Henderson and Clark Citation1990). As described in the introduction, the example of DIRTT’s digitally-manufactured prefabricated wall frames contains integrated components such as plumbing and electrical. The core design concept of a wall remains unchanged. However, the innovation requires a change in the interface between components and a change in the process (e.g. schedule, sequencing, etc.) of the overall system. Such examples cross professional and trade specializations, redefine how work is done in the industry, and break industry standards. For these types of innovations, there is a fundamental mismatch between the construction industry structure and the characteristics of the innovation (Taylor and Levitt Citation2004; Katila et al. Citation2018).
By contrast, scholars find that innovations that align – or “mirror” – the existing divisions or “silos” institutionalized by the construction industry structure are three times more likely to be adopted on construction projects (Katila et al. Citation2018). Such aligned innovations do not cross traditional discipline boundaries and fit within the existing divisions of work and specialization. An example would be a prefabricated timber truss system. Such innovation has already diffused within the industry because it fits within the existing timber supply chain and does not change any of the standardized interfaces for the overall system-level architecture (Taylor and Levitt Citation2005). It does not alter the interface of adjacent construction products or the process of installation within the building. Implementation can be as simple as removing the old components from design and replacing it with the newer component.
This is not to suggest that the diffusion of any type of innovation is easy in construction. As summarized in , demand fluctuations, liability concerns, and vertical fragmentation in decision making inhibit innovations of all kinds (Sheffer Citation2011). However, systemic innovations are additionally impacted by a lack of cross-subsidization in the fixed price competitive bidding system, a diffusion of responsibility due to a lack of strong central coordinating firm and broken learning and feedback loops that occur when inter-firm teams disband at the conclusion of projects (Gann and Salter Citation2000; Dubois and Gadde Citation2002a). Recent research by Katila et al. (Citation2018) empirically verifies the link between project-based organization, industry structure, and the adoption of systemic innovations. Projects with high levels of horizontal and vertical integration are two and a half times more likely to implement “green” systemic innovations than a project with no vertical or horizontal integration, even after controlling for the cost of the innovation.
Figure 1. Structural barriers to innovation in construction (Sheffer Citation2011). 1Nam and Tatum (Citation1988); 2Maisel (Citation1963); 3Gann (Citation1996); 4Reichstein et al. (Citation2005); 5Fergusson (Citation1993); 6Henisz et al. (Citation2012); 7Martishaw and Sathaye (Citation2006); 8Tatum (Citation1986); 9Darley and Latane (Citation1968); 10Hall and Soskice (Citation2001); 11Taylor and Levitt (Citation2004); 12Dubois and Gadde (Citation2002a); 13Stinchcombe (Citation1959); 14Sheffer (Citation2011) defines the term integral innovations as inclusive of the definition of systemic innovations and radical innovations.
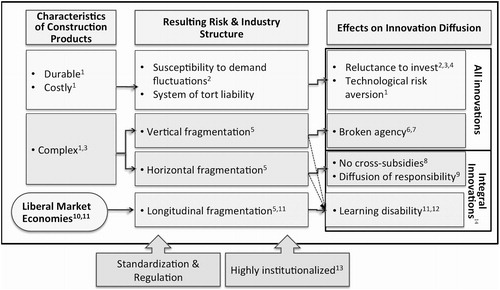
Notably, much research on systemic innovation has occurred at the project level (e.g. Brockmann et al. Citation2016; Hall et al. Citation2018; Katila et al. Citation2018) or at the industry network level (e.g. Taylor and Levitt Citation2007; Toole et al. Citation2013; Bygballe et al. Citation2015). However, less scholarship to our knowledge has looked at the firm-level as the unit of analysis to understand how firms evolve or integrate to implement systemic innovations, although a few exceptions exist (Lindgren et al. Citation2018).
The construction mirroring trap
If systemic innovation can be inhibited by industry structure, rational firms within construction should seek opportunities to restructure the industry and capturing value from systemic innovations. Why has this not happened to a greater degree? One emerging theory is the “mirroring trap” (Colfer and Baldwin Citation2016). We suggest that the institutionalization of the current industry structure (Henisz et al. Citation2012) has deterred systemic innovation attempts and standardized the range of components that can fit within the dominant systems-level architecture. Individuals and firms are incentivized to focus on specialty knowledge aligned with their specific tasks in the fragmented construction supply chain. Given the challenges of coordinating complex interdependent tasks (Thompson Citation1967, Galbraith, Citation1974), the “mirroring” of technical dependencies and organizational ties is an organizational solution that allows firms to conserve scarce cognitive resources (Colfer and Baldwin Citation2016). The concept of information hiding is used to control complexity. Each module in a technical system is informationally isolated from other modules within a framework of system design rules (Baldwin and Clark Citation2000) so that “independent individuals, teams, or firms can work separately on different modules, yet the modules will work together as a whole” (Colfer and Baldwin Citation2016). Furthermore, mirroring is an economical way to set up complex technical systems because it places problem-solving resources in strategic places where these problems are likely to appear (Colfer and Baldwin Citation2016).
However, when this mirroring is too “strict,” it inhibits industry evolution. In an analysis of 142 empirical studies that looked at the linkage between technical dependencies and organizational ties, Colfer and Baldwin (Citation2016) identify 10 longitudinal industry-level studies. For nine of these cases, they find that the organizational structures of firms were initially well-aligned with the dominant technical dependencies. However, when a new system was discovered that offered a better value proposition, incumbent firms either adapted their organizational structure to the new technical dependencies (i.e. break the mirror) or exited the industry. The tenth case – the construction industry – is the only instance where incumbent firms resist organizational change. While scholars have articulated reasons for this resistance – including a prevailing business model of competitive bids, low margins, and over-emphasis on cost minimization rather than new product development (Nam and Tatum Citation1989) – it remains true that the construction industry struggles (e.g. Katila et al. Citation2018) to “access new opportunities involving technical architectures that do not mirror the current industry structure” (Colfer and Baldwin Citation2016). This inability to access new opportunities is referred to as the “mirroring trap”.
This form of “strict mirroring” means that technical tasks and technical knowledge of a complex system are partitioned into modules. These modules are referred to in construction industry language as silos or “islands of automation” (Levitt et al. Citation1991). Silos reduce the amount of knowledge needed by any one person or group. In theory, this should be the most economical way of conserving cognitive resources; team members are only responsible to acquire knowledge about the work within the boundaries of their own silo. In practice, these “islands of automation” allow structural or mechanical engineers to perform their individual work tasks more efficiently. However, silos also reduce capabilities to support concurrent, multidisciplinary decision-making (Levitt et al. Citation1991).
In industries where this overly strict mirroring of knowledge with task dependencies occurs, it prevents firms from seeing opportunities to change their boundaries or restructure the industry (Colfer and Baldwin Citation2016). It is difficult for firms to recognize that a particular innovation is indeed systemic. It is even more difficult to acquire the implicit system architecture knowledge required for implementation (Henderson and Clark Citation1990). Over time, firms develop organizational structures and information channels that are focused on component-level activities (Henderson and Clark Citation1990). They seldom question the overall system. It is possible that strict mirroring can cause firms “not only [to] resist innovative threats, but actually resist all efforts to understand them, preferring to further entrench their positions in the older products” (Utterback Citation1996). Furthermore, especially in periods of dynamic technological change, entrepreneurial firms have the opportunity for aggressive strategic action to “break” the dominant product architecture and build upon systemic innovations through new organizational arrangements (Colfer and Baldwin Citation2016).
Digitally-enabled manufacturing
The US construction industry currently finds itself in such a period of dynamic technological change. The technological development and adoption of Building Information Modelling (BIM) in the 1990s and 2000s have provided new and powerful digital tools. These tools have fundamentally motivated project-based firms to develop new project capabilities for digital delivery. This includes the set-up of digital delivery and managing across multiple digital systems, ultimately using digital technologies to create shared identity across the firms involved in the project (Lobo and Whyte Citation2017). Scholars find evidence that for project teams to implement digital technologies such as BIM, new organizational forms and practices need to mirror the data requirements of the 3D model (Dossick et al. Citation2019). Furthermore, because the sub-units of a project can be highly independent and specialized, projects need to adopt formal and informal integrative practices to make visible interdependencies that typically cannot be anticipated in advance (Tee et al. Citation2019). As a result, the digitalization of project information has fundamentally changed project management (Levitt Citation2011; Whyte and Levitt Citation2011) and project delivery models (Whyte Citation2019).
However, this digitalization has not necessarily enabled digital manufacturing. In this paper, we refer to digitally-enabled manufacturing as a combination of digitalization and industrialization for construction. Digitally-enabled manufacturing – also referred to as industrialized, off-site, prefabricated, volumetric, modular, or robotic construction – is a movement to improve productivity and efficiency in construction. A "digital chain" links downstream manufacturing and assembly activities with upstream design constraints. By moving the construction process to a controlled, off-site environment, significant improvements in material and labour efficiency are possible. If done correctly, this could drastically reduce the time and cost of new construction projects. The manufacturing industry – especially that of the automotive sector – is often used as an example in this respect (Gann Citation1996). While industrialization has become an accepted practice in some construction regions (Gann Citation1996; Lessing et al. Citation2015), it has seen slower diffusion in the United States until recently. Today, there is significant momentum for digitally-enabled manufacturing capabilities in the United States, with an unprecedented investment of over $1 billion from venture capital and investment firms in 2018 (Pullen et al. Citation2019). Investors seem to believe the technology offers the opportunity for industry disruption (Steinhardt et al. Citation2019)
Digitally-enabled manufacturing is difficult to achieve. It requires more than just the off-site manufacturing of building parts. It requires high initial capital costs to set up new manufacturing facilities (Gann et al. Citation2018). It also requires new digital design processes with higher levels of precision and less flexibility to make changes later in the project. When new technical solutions are developed for digital manufacturing, they must be developed and partitioned into the appropriate new systems and sub-systems (Lessing Citation2006). To address this, digital manufacturing for construction needs to be managed strategically and not just at the project level. It requires a holistic approach that includes increased development of long-term relations in the supply chain, more understanding of the customer priorities, greater use of systematic performance measuring, and emphasis on continuous improvement (Lessing et al. Citation2015; Lessing and Brege Citation2015). While mega-projects might have enough scope and scale to justify such investment for a single project, the majority of construction projects do not.
To summarize our departure point, digitally-enabled manufacturing should be considered a systemic innovation with the potential for industry disruption. Typically, such systemic innovations are resisted by the structural barriers of the industry. Over time, these barriers have institutionalized to create a dominant system architecture partitioned into specific tasks found in the industry supply chain. Firms and individuals, in order to conserve scarce cognitive resources, have tightly aligned their specific knowledge to “mirror” the tasks in each silo. While advantageous in the short term, such an approach may have resulted in a “mirroring trap” where the industry is unable “break” the dominant system architecture, build upon systemic innovations, or create new system architectures.
If the above is true, there should be strategic opportunities forward for construction firms seeking to implement digital manufacturing to “break the mirror”. Such firms might seek opportunities to restructure the boundaries of the organization in order to mirror a new system architecture that can (potentially) better capture value from a technology such as digital manufacturing. However, there is very little research that we are aware of that shows how construction firms do this, especially looking at the firm-level as the unit of analysis. This paper seeks to answer this point. How can entrepreneurial firms strategically “break the mirror” in order to restructure industry boundaries and develop new products with corresponding new system-level architectures?
Research design
To answer the above question, we conduct a comparative case analysis (Yin Citation2018) of three entrepreneurial firms that have increasingly embraced digital manufacturing in construction. We select three cases from the same regional context – the San Francisco Bay Area. We select the cases for maximum variation as each of the three firms began from very different origins and different capabilities (Eisenhardt Citation1989; Flyvbjerg Citation2006).
Data were collected in two phases. provides a summary of data collection activities. The first round was conducted in 2014 as part of a larger sample for two research studies emphasizing supply chain integration practices (Hall et al. Citation2018) and business models for industrialization (Lessing and Brege Citation2018). This data includes recorded and transcribed interviews, site visits, factory tours, and guest lecture seminars and discussions. This paper specifically seeks to understand how the firms were breaking knowledge and task dependencies within their organizational structure, so we re-coded the 2014 transcripts through this new theoretical lens. The second round occurred in 2019 when the researchers returned to the three firms for additional interviews. In this round, we asked how the firms strategically changed in the past five years since the initial point of contact. The semi-structured interviews averaged between 50 and 75 minutes. Examples from the line of questioning include:
Table 1. Summary of data collection from Phase 1 and Phase 2.
Table 2. Summary of approaches by case firms.
How would you describe your firm to someone else?
In your opinion, what makes your firm different than other firms in construction?
How does your firm learn from project to project?
How would you describe your approach to integration?
What is your relationship with the supply chain?
How is your approach a strategic advantage for your firm?
What are the challenges of your approach?
The second round of interviews was also recorded and transcribed and coded by the first author to understand the evolution of the firm. Altogether, the data collection represents 20 different sources – 12 from 2014 and eight from 2019. During the first round of coding, excerpts were categorized using high-level constructs such as business models, new building products, barriers to innovation, overcoming industry fragmentation, and supply chain coordination. During the second round of coding, data were analyzed and assigned to child codes. For example, under overcoming industry fragmentation, child codes include horizontal integration, vertical integration, longitudinal integration, and strategic partnering. These data represent the basis for the case descriptions and findings made in this paper. In total, transcripts from 14 of the interviews were coded and analyzed. The source of specific excerpts used in the findings sections is indicated with the identification number shown in brackets from (e.g. [1] refers to a quote from the Senior Project Manager, DPR).
During the analysis, we followed an approach of systematic combining between the case data, extant theory, and the parallel development of a theoretical framework (Dubois and Gadde Citation2002b). Our case analysis first seeks to understand – within each case – how the firm strategy has evolved to enable digital manufacturing over the past five years. We proceed to compare across the cases, to examine the benefits and challenges of each approach, and to draw conclusions about cross-case patterns of mirror-breaking (Eisenhardt Citation1989).
The primary approach of this paper is one of theory building. We do not argue that the approaches from the three cases represent the only strategies for digital manufacturing or to “break the mirror.” It is possible and likely that other strategies exist. Furthermore, our analysis is limited to a retrospective view by stakeholders over the last five years. It is possible that managers will re-interpret past actions as justification for current and future strategies. We acknowledge that such post hoc rationalization of enacted strategies is likely to exist in the data collection. In addition, we acknowledge the difficulties in gathering interviews from individuals in a firm and – no matter how senior their position – generalizing their positions to represent the entire company. Nonetheless, from our visits, interviews, and interactions with these three firms, we found clear and distinct patterns and categorizations of strategic approaches as they emerged from our analysis and interpretation.
Findings
Here, we present the evolution of the three firms in their journey to enable digital manufacturing. A summary is provided in .
DPR construction: evolving from “brokers to builders”
DPR construction (DPR) is a commercial general contractor and construction management firm founded in 1990 and currently based in Redwood City, California. They rank as one of the top 50 general contractors in the United States since 1997. In 2018, the company had $6 billion in total revenue and operates from 28 office locations across the United States. DPR describes their value in maintaining a reputation as an industry leader in the coordination of BIM using collaborative practices such as colocation at the early stages of the project to maximize coordination efforts. In addition, they describe themselves as an early adopter of relational contracting project delivery models such as Integrated Project Delivery (IPD). The core of DPR’s business is the management of projects. The firm uses a relational and customer-focused strategy to deliver projects for clients while maintaining positive and collaborative relationships with designers and trade contractors.
Before 2017, DPR observed successful prefabrication strategies used by trade contractors to bring large pieces of ductwork, wall panels, or utility racks from off-site factories to onsite projects. The motivation for such new products was often driven by a strong owner client wanting to push innovation. During these projects, DPR actively coordinated the off-site work using their advanced BIM capabilities. They gained important knowledge about tolerances, logistics, and design requirements for digital manufacturing. DPR embraced the management of this type of innovation because it enabled observations in a project-based capacity without the need to make high-capital investments into manufacturing assets. It also leveraged DPR’s relational approach and provided new opportunities for the firm to learn.
Despite the use of BIM coordination and relational contracts such as IPD, interviewees from DPR repeatedly describe four types of challenges arising for each project with digital manufacturing. First, each project requires significant design-team coordination. Time is required to get designers comfortable with the manufacturing process, gather design input from multiple stakeholders and ensure the proposed solution meets objectives. Second, trade contractors in the supply chain are difficult to convince:
Subcontractors will do what they know because that is how they make money. If you ask them to step outside of that comfort zone, the cost metrics get affected by that. They are not willing to take a risk [or] to be constrained in their budget because they do not understand it. They have never done it. They probably do not have the bandwidth internally to evaluate it [2].
Third, DPR’s costs are accounted on a project-by-project basis. Project teams consistently ask “how does [prefabrication] impact the project” and are not willing to bet the success of the project on an unproven concept. Fourth, DPR identified more opportunities for increased digital manufacturing – e.g. “the whole building really needs to have an approach that is about prefabrication” [7] – but they struggled to find partners and suppliers to take advantage of such opportunities.
DPR reflects on their 2017 decision to financially invest in digital manufacturing as a strategic decision to become “more builders than brokers in the supply chain” [6]. DPR invested $20 million from a new internal innovation fund into a new spinoff firm. The vision for the new firm – called Digital Building Components (DBC) – is to combine DPR’s knowledge of BIM coordination with its observed learnings about digital manufacturing from past projects. DBC will not “just focus on off-site fabrication” but leverage a “highly-coordinated model” for fabrication in order to “really change the way we build” [7]. DBC operates a large factory in Phoenix, Arizona where the firm manufactures light-gauge steel framing and assembles three new industrialized product lines: (1) a digitally-fabricated load-bearing structural panel system, (2) exterior wall panels and (3) interior wall panels with in-wall MEP systems. Each product line can be customized. DBC takes a productization approach to redefine the value propositions of digitally-enabled manufacturing. This strategy has created challenges to sell the new product to the rest of the construction industry:
We get questions all the time, someone will say, “Well what are the [light-gauge metal] studs worth?” And I stop, because I actually don't get the value of the question. What does it matter what the studs are worth? … it is the suggestion that people know the value of metal studs and their traditional studs, so they basically want to back out all the numbers and be like, “Okay. Is the value of this wall accurate for the market today?”
People want to see what it's worth instead of understanding the full value proposition. Now [with a panel] you no longer have to have the additional soft costs of integrating that trade, or the additional mobilization of bringing someone else on site. [7]
The product development manager at DBC reflects that the decision to give their integrated interior wall panels a product name – the TEKwall – was intentionally done to help customers conceptualize the wall panel as a new product and “realize the value proposition of getting the two components together, instead of trying to break down those into our traditional trade classifications” [7]. Further challenges remain to coordinate design; the model needs fabrication levels of detail with enough lead time for manufacturing. Yet, participants from both DPR and DBC agree that there are benefits to the relational set up between the two firms. They describe that DPR has experience and expertise to fully coordinate the fabrication information that DBC needs to take directly from the model. DPR can take more control of the value chain, expand their knowledge and capabilities, and become “organized learners” [5] without abandoning their core identity of relational project-based management. DPR can learn “what information needs to be provided at what point in time in the project” [7]. They “can start to drive the coordination process differently they would do without this digital fabrication” [7].
Moving forward, both sides express the desire to build on the relational foundation established at DPR. They express the need “to work with partners who will let us bring that learning to the table because we cannot do it on our own” [6]. Meanwhile, DBC would like to pursue additional productization that continues to blur the lines between traditional trade scopes. DBC would like to develop a future product line for a national client – with a repetitive building type and multiple projects – and then identify other trade partners or specialty trades that also embrace prefabrication. Future efforts can focus on a relational network of preferred partners that designs and engineers a new and synergistic system architecture for use on repeat projects with the same client.
RAD Urban – embracing “vertical integration to the core”
The origins of Rad Urban (RAD) come from Nautilus group, founded in 2009 as a project development company with a design-build focus. Nautilus acted as a general contractor with architecture and structural engineering capabilities in-house. When the company decided to enter the residential infill market in 2012, they began further vertical integration of the company. Nautilus purchased a 160,000 SF factory in Lathrop, California for digitally-enabled manufacturing and production and developed a line of steel prefabricated modules called Nemo Building Systems. At the time of interviews in 2014, Nautilus had 20 employees, Nemo had 15, and no projects had yet been completed.
In 2016, Nautilus and Nemo rebranded, forming a new company called RAD Urban. They now employ over 300 people and have opened international offices in India and the Philippines. They have completed several projects, with three projects currently under construction. They have a steady stream of future projects for further expansion. Current construction includes two 29-story towers in downtown Oakland, California that will represent the tallest modular buildings in the United States when complete. In 2018, RAD raised $28 million in financing to grow the company and bring its modular expertise to third-party developers.
In 2014, Nautilus described that motivation for vertical integration was to control the complete process from land acquisition through design, engineering, production, and sales. The vice president and director of operations envisioned success would be driven by their fully integrated firm with one goal in mind:
A very common theme for us is to do as many pieces as possible under our roof. One reason is that no matter how good your consultants are, they have different priorities, different interests and will do things in different ways than we will. So, we want to try to keep control as much as possible. As a design-build developer, we always have been [in the past] in control of our own destiny… [when] you get the paycheck from the same company truly having one goal… that is our biggest advantage! [9]
RAD attributes its recent success to a combination of attracting excellent and innovative people, the ability to prototype and experiment “in their own sandbox”, and their vertical integration. For prototyping, RAD started with their own land acquisition and development of their own projects. The CEO describes a three-story, 5-unit pilot residential project built in 2016 as the most expensive condominium project in Oakland history. However, a project engineer describes the advantage of using such an approach to learn because:
When you have your own project, you can afford to expand the schedule four months or you can go a little bit over budget because you are still trying to figure out detail, a connection, or the right material. You are still trying to figure out your product. Having the luxury of having our own projects to start with from that learning curve has been part of the secret sauce on our end. [13]
RAD describes vertical integration as an enabler to create new building products and components. For example, RAD identified potential cost savings to include the “building skin” (i.e. façade) as part of their steel volumetric module building system. RAD then developed an original panel product called the MegaWall. The MegaWall was designed and specified to the full width and height of their own modules. This product development approach was described as an example of “just one original component” that RAD “developed in order to successfully finish" their new system.
In addition, participants described vertical integration as an enabler to break down traditional roles and knowledge silos. For example, a project engineer described:
It's really a luxury to work in vertical integration. There's no clear delineation of who does what. I submit to the structural engineer, I submit to the architect, there are certain roles that the architect typically does that in my case I would do. There are certain roles that the pre-construction manager typically does that in our case the architect will help out with. It allows for a lot of flexibility and sets the project up for success [13].
RAD describes several challenges of vertical integration. First, the factory requires a steady line of production. The factory is a “hungry beast” burning cash and “needs to be fed” [9]. The alignment between manufacturing schedules and project schedules is difficult to coordinate. Furthermore, when increasing the number of projects, RAD must hire additional team members. Yet, building projects can often be delayed or cancelled on short notice, leaving RAD stuck with a larger team than necessary. This leads to a more conservative approach to scale the team than in a traditional approach where they could “just hire an outside architect, pay him a fee, and if the project doesn't go forward then it’s not my problem” [13]. RAD also needs to hire employees with experience “that is a hybrid between an industrial engineer and a construction engineer,” but state that experienced managers with such a background seldom exist. One participant stated that the greatest challenge is not creating the building system and product itself but organizing the infrastructure and people in place to make vertical integration successful.
Nevertheless, RAD believes vertical integration allows them to move faster than the competition in digitally-enabled manufacturing. The prevailing mentality from the interviews can be summarized by the quote that RAD as a firm will “just roll up our sleeves and focus on design and construction. We will do better [than then competition] and go 1000 miles per hour faster” [13].
Project Frog – turning “cycles like a software company in a physical goods environment”
Project Frog was founded in 2006 as a venture capital-backed startup in San Francisco, California. They emphasize design to enable digital production. In its initial years, the firm positioned itself as an alternative solution to the traditional construction fragmentation by providing pre-engineered and prefabricated kits of parts for the efficient assembly of permanent buildings. Project Frog describes one core element of its strategy to not own or operate their own factory. They view themselves as a “product supplier” that utilizes an industry 4.0 approach. Industry 4.0 – also known as the fourth industrial revolution – is characterized by the development of digital factories organized around smart automated systems, mobility, flexibility, integration of customers, and new business models (Jazdi Citation2014). Project Frog partners with manufacturers and suppliers around the world. Project Frog develops the product, develops and details the overall system design, and manages the supply chain and delivery process. Local partner contractors assemble the products at the building site. In 2017, Project Frog completed a Series BB round of venture capital finance to bring the cumulative level of investment funding for the firm to around $74 million.
The company emphasizes horizontal integration through interdisciplinary interactions. In 2014, the 70 employees of Project Frog included architects, designers, structural engineers, mechanical engineers, construction managers, supply chain managers, and business developers.
Having all those interdisciplinary teams underneath one roof allows for deep coordination because you can sit down with these people and say, here’s my architectural system, structural system, and MEP system. With this new architectural idea, it is far easier for the MEP system, far easier for the structural system, and far simpler to bring those elements together to develop a synergistic relationship between historically-siloed parties. [14]
This was described as a reaction to the traditional way of working in the industry where you produce “just enough information and always avoid liability.” The traditional way makes it very difficult to “have the room to research, innovate, own more of the risk and really push the boundaries” [20]. Project Frog’s integrated teams worked to design new products co-created in development with supply chain partners who owned the manufacturing capabilities. They designed new products with the purpose to condense the scope of work and reduce the number of trades required for on-site assembly and coordination.
However, limitations in this product offering became apparent over time. The product did not have enough flexibility and robustness to meet the needs of potential customers. In addition, Project Frog only offered a partial solution. They still relied heavily on partnerships with architectural firms as “we were not stamping drawings and we were not delivering product all the way through the market cycle” [19] despite having a robust construction liaison team. Furthermore, Project Frog suffered from market misalignment. Their primary market of education involved distributed decision-makers who were each responsible for only a few projects each year, making it difficult to scale the business.
In 2016, Project Frog went through major organizational change, replacing company leadership and eventually scaling employees down to around 30 people. The company also evolved its product offering to embrace a new strategy of mass customization. They transitioned away from modular construction toward a flexible kits-of-parts called their “Frog Kit.” Using the principles of mass customization, Project Frog developed a web-based configuration platform called myProjectFrog. The configuration platform draws from a library of Frog Kit parts designed in Autodesk Revit. Using heuristic rules for design and assembly and the logical constraints of shape grammar, myProjectFrog enables designers to manipulate building design on a standardized grid. Since the rules of Project Frog’s products are embedded in the platform, Project Frog has confidence that the resulting design can be manufactured by Project Frog’s partners in the supply chain. The resulting product design also conforms with local building codes and structural requirements. Once the configuration is complete, the platform automates the production of permit drawings, structural calculations, a building information model, and a bill of materials within minutes.
Project Frog now views their role as developing the core infrastructure to allow integration of product designs provided by digital-manufacturing suppliers. The Vice President of Product and Innovation describes a future vision for the development of such a platform which “integrates a product-ready supply chain” and enables a new kind of ecosystem for construction:
There needs to be a platform for AEC, not just fragmented co-opting of products off the shelf today. This kind of common, integrated, and scalable platform is not found in the market today. Every company rolls out their own software and nothing talks to each other. Only a Cloud platform built for AEC will achieve critical mass [20].
Project Frog has never owned its own manufacturing facility. They intentionally avoid committing to high-capital investments or specific forms of manufacturing. They claim to not be “burdened so much with so many factories standing up” but to have the “freedom to think about other things like technology integration and building systems” [19]. This approach enables agility in product design and low capital costs, which allows them to turn “software development cycles with buildings” [20]. Project Frog notes a “blurring of the lines” between architects, engineers, supply chain managers, and programmers. “Some of the architects and engineers start to speak the language of software a little more” [20], a trend that Project Frog predicts will increase as they continue.
Project Frog identifies some challenges to their industry 4.0 approach. They must manage misaligned risk allocation and “have somewhat of a traditional risk-sharing relationship.” They “do not own all the risk in one place.” Project Frog has to work to “find the right manufacturer that will go on the journey with you.” However, they find that there is a trade-off in the absolute level of control you have over cost with this approach and less ability “to innovate and customize components” [19]. In return, however, Project Frog describes that the knowledge share between themselves and manufacturing partners results in a product optimized for scale.
Today, Project Frog believes their platform approach can scale as more of the supply chain for digital manufacturing becomes available and is compatible with configuration and mass customization. They anticipate future products will be available as components that can be configured using the tools created by Project Frog. Project Frog believes they can draw from their horizontal integration and experience with manufacturing to build the infrastructure required to align the physical and technology stack for this new emerging ecosystem. Specifically, Project Frog wants to develop an open platform where they provide the core infrastructure of digital systems integration but welcome other firms to participate:
Because we are not a big vertically integrated closed system, we can help more broadly move the industry forward. We can work with other architects, builders, and corporate developers to get people into the industrialized construction world. We are not here to just hold all the cards to our chest. It is our goal to move the ball forward, helping to develop the infrastructure for the ecosystem and licensing our specific solutions to anyone who wants it. This is what sets us apart from the competition - we want to share! [19].
Different forms of mirror-breaking
In each case, we find evidence of a firm deviating from the institutionalized structure of the project-based industry. We argue that each case presents a different strategy to “break the mirror” (Colfer and Baldwin Citation2016) and counteract the strict mirroring prevalent in construction industry silos. To further the discussion, we unpack how each of the three cases differs in both their strategic approach and their form of mirror-breaking.
DPR first used a relational, project-based strategy to gain knowledge about a new type of systemic innovation. For example, DPR began monitoring digitally-enabled manufacturing through informal project-based mechanisms such as colocation. From this knowledge, DPR later made the strategic investment in the spinoff firm DBC. They retained a close relationship to DBC in order to become “organized learners.” We interpret that DPR began with a strategy of partial mirroring allowing them to explicitly invest in knowledge of technologies beyond their task boundaries. This effort is an “effective way to explore and understand latent interdependencies that are not apparent under the current technical architecture” (Colfer and Baldwin Citation2016) while expanding their knowledge to exceed the scope of their tasks (Brusoni et al. Citation2001). Partial mirroring is often not the most economical strategy, as it violates the principle of information hiding and increases costs of participation (Colfer and Baldwin Citation2016). This is reflected in industry practice, where informal supply chain integration practices (Hall et al. Citation2018) such as team colocation and early involvement of key participants have been found to increase knowledge exchange (Bygballe et al. Citation2014; Papadonikolaki Citation2018) but are criticized for adding costs to project management (Ma et al. Citation2018).
In this case, partial mirroring enabled DPR to explore new interdependencies that is slowly absorbed into practice for their future projects. The partial mirroring strategy can be effective long-term for the network of firms as it allows them to view the current system in a broader context. It enables the project teams to learn and propose more appropriate linkages between knowledge and task interdependencies for their next project (Colfer and Baldwin Citation2016) without investing in significant change to institutionalized contracts, work routines and supply chain arrangements.
The second strategy used by DPR was one of relational contracts (Colfer and Baldwin Citation2016). DPR attempted to use IPD – a relational contract – to break the supply chain from fears of liability and costs of innovation, in order to co-create knowledge together for prefabrication. Due to space and scope limitations, we cannot fully present and expand on DPR’s use of IPD to enable systemic innovations in this paper, but our analysis aligns with other scholarship that finds the use of IPD enables projects to explore systemic innovation through “project-based” vertical integration (including the project sponsor/owner, designers, general contractor and trade contractors) and horizontal integration (between traditionally separate trade contractors and system designers) (Hall et al. Citation2018). Relational contracts foster high levels of communication and cooperation across firm boundaries (Colfer and Baldwin Citation2016). However, we find that DPR's strategy of IPD was hindered by project-based longitudinal fragmentation, where project teams disband at the conclusion of the project and parties often did not work together again. This hindered learning and broke potential feedback loops (Gann and Salter Citation2000) that are especially important in digital manufacturing. In the end, relational contracts reduced inefficiencies but did not enable DPR to develop new "value-adding products.”
We find that DPR’s third strategy to be one of strategic investment in a special relationship through the use of a spinoff. This strategy has not previously been identified as a mirror-breaking strategy in the literature. Through funding and building a relational partnership with a strategic investment in DBC, DPR repositioned themselves to transition toward becoming “more builders than brokers.” The close relationship between DPR and DBC creates opportunities for mutual learning without changing DPR’s core business model of projects. DPR is learning how to manage the design process to take full advantage of digital manufacturing in a project-based environment. DBC is free to push toward further productization of their digitally-enabled manufacturing capabilities. Together, this strategy offers DPR the opportunity to co-create new technical interdependencies and systemic innovations in the context of a mutual, long-term relationship.
RAD Urban uses a classic strategy of vertical integration. Vertical integration enables a streamlined process of real estate acquisition, design, engineering, off-site fabrication and assembly. We interpret RAD Urban’s strategy as a form of strategic mirror-breaking through redefinition of systems architecture (Colfer and Baldwin Citation2016). They created a new technical building system – a stackable volumetric module made from structural steel – and began developing new products such as the MegaWall that integrate with the new system architecture rules. Horizontal and vertical integration keeps control of product in-house. This enables RAD to self-organize and better align their organizational structure with the emerging technical dependencies of the systemic innovation (Colfer and Baldwin Citation2016).
Longitudinal continuity comes through the development of their proprietary technical building system. The system continuity from project to project serves as the internal product platform in which organizational knowledge can be embedded. Versions of the building system are released in a similar way to versions of mobile phones or software are released (e.g. version 1.0, version 2.0, etc.) (Wikberg et al. Citation2014).
One advantage of RAD’s approach is the ability to drive digitally-enabled manufacturing at a high rate of technical change (Henderson and Clark Citation1990; Baldwin and Clark Citation2000). RAD states an intent to move faster than rivals and push the limits of technical change required to compete in the market (Colfer and Baldwin Citation2016). Assuming their vertical and horizontal integration enables RAD to align their internal organizational configurations with the new system design and the workflows of digital manufacturing, it will position RAD to be a leading innovator in this rapidly shifting market. However, RAD faces the risk of high capital investment and the need to “feed the beast” that is their factory. Dealing with demand fluctuations present in the market (Maisel Citation1963) might be difficult for vertically integrated firms such as RAD to handle. RAD might be vulnerable to economic downturns. Furthermore, RAD could risk prematurely settling on and then organizing around their technical building system architecture. In this time of technological change, RAD risks specifying and investing in a novel product and system architecture that could be sub-optimal to later innovation approaches (Colfer and Baldwin Citation2016).
Project Frog uses a strategy of digital systems integration. They extend ecosystem knowledge boundaries virtually through their development of a mass customization configurator. This industry 4.0 platform acts as a digital link between design and manufacturing. The embedded logic and heuristics of the platform reduces transaction costs for the partners in the supply chain. This is another example of strategic mirror-breaking through the redefinition of systems architecture. However, Project Frog’s mirror-breaking is also notable because it exhibits some characteristics of a core-periphery structure (Colfer and Baldwin Citation2016). As a digital systems integrator, Project Frog provides the “core contributions” by setting up the platform ecosystem. Design occurs within an integrated cloud-based platform to configure projects using mass-customization. Design choices are constrained by the rules sourced from design, manufacturing and construction constraints (Levitt and Kartam Citation1990). They are then embedded in the product configurator used by customers (Malmgren et al. Citation2011). These heuristic rules – or rules of thumb – can act as a “glue” capable of connecting design, engineering and manufacturing (Dym and Levitt Citation1991).
The “periphery” is then made up of the connected suppliers and trusted partners. These partners provide contributions by developing the smaller, localized products which Project Frog inputs into their platform. The partnerships between Project Frog and the periphery are long term, so that the platform retains continuity across projects and product co-development can occur. Project Frog acts as the core digital systems integrator that can organize the supply chain for their new system architecture. Project Frog believes this strategy can enable a new ecosystem of mass-customized, manufactured parts sourced from the periphery supply chain partners. By using a capital-light industry 4.0 approach with no investment in their own manufacturing facilities, Project Frog can remain agile. They can “turn software cycles” that iterate and improve the heuristics and logic rules of the core configuration platform over time.
The challenge for this approach is that it requires the configuration engineering solutions to be constrained to the product offerings of the periphery partners. The control of the system architecture is not confined within the core firm’s organizational structure. Since Project Frog does not design or manufacture the physical products themselves, more time is needed to co-create innovative products with long-term periphery partners.
Characteristics of mirror-breaking in construction
Each of the three firms – DPR, RAD and Project Frog – provide examples of an entrepreneurial firm in construction embracing digitally-enabled manufacturing. The firms each have developed new products – the TEKwall, the MegaWall and the Frog Kit. RAD and Project Frog have done this as part of a restructured systems architecture – e.g. the modular high rises by RAD and the output of the myProjectFrog automated building configurator by Project Frog. DPR and DBC are currently pursuing opportunities for restructured system architecture through formalized relationships with repeat clients and other digital manufacturing partners. From our analysis, it is difficult to judge how intentional such strategies have been. Each of the firms has prided themselves on doing things differently from the traditional industry. However, the specific three strategies seem to have evolved over the past five years as some combination between intentional restructuring of organizational form and the unintentional but necessary restructuring that comes as a consequence of continuous searching to find competitive advantages and access new innovation opportunities (Colfer and Baldwin Citation2016).
Across all three cases, several themes emerged regarding digitally-enabled manufacturing. Participants expressed that the existing paradigm in the construction industry is too strict. They describe an industry structure that resists learning and innovation. They described the implications of this “mirroring trap” as a barrier to digitally-enabled manufacturing. For example, DPR describes the supply chain as “not willing to take risks” and unable to have the internal bandwidth to evaluate innovative opportunities. RAD describes consultants with different priorities who will not push toward the same innovation goals. Project Frog describes an industry where participants are expected to produce “just enough information” against aggressive deadlines without taking on liability, leaving no room for innovation.
Each of three case studies illustrates the need for new employees with hybrid skills and competencies that better align or “mirror” the new system architecture. DBC needs a product designer to “productize” their components and redefine the value proposition. RAD needs a new hybrid position between construction management and industrial engineering. Project Frog sees a “blurring of lines” between architecture and software development.
The cases show that digitally-enabled manufacturing benefits from longitudinal continuity. The existing project-based industry is not well structured to deal with this challenge. DPR has held to a project-based strategy but notes the slow education of designers and contractors as problematic. Liberal market economies such as the US are more fragmented from project to project, and project-based networks will take longer to achieve network-learning (Taylor and Levitt Citation2007). The process of network alignment is crucial to achieving collaboration for digitally-enabled manufacturing (London and Pablo Citation2017) but is slow to occur in these project-based networks (Gann and Salter Citation2000; Taylor and Levitt Citation2004). One implication is that in networked, project-based markets such as the US, mirror-breaking using a firm-level strategy such as vertical integration or core-periphery structures can provide more effective longitudinal integration than the traditional project-based strategy. This is an opportunity for future research. Little construction research to date has looked at the implications of these new organizational forms in the US, despite the recent emergence of many such start-up firms (e.g. Katerra, Plant Prefab, Skender).
Platforms for digital manufacturing
Our analysis also finds that the firm-level integration used by RAD and Project Frog emphasizes the development of platforms. Platforms in construction are characterized by the reuse of process and technical solutions and the formation of a stable supply chain (Jansson et al. Citation2014). These platforms act as a transmitter of longitudinal continuity; new knowledge and heuristics can be embedded and managed within the “release” of each new platform version (e.g. v2.0 follows v1.0) (Wikberg et al. Citation2014). This avoids knowledge tacitly embedded within team members who move from project to project (Johnsson Citation2013). This rise of “platformization” has the potential to create new ecosystems for construction that connect suppliers and customers. Platform organization enables different levels of engagement for core firms that act as system integrators and periphery firms that supply products (Colfer and Baldwin Citation2016). Further investigation into understanding platform organization and how it enables longitudinal integration is needed.
One proposition is that future platform development will tend to be open or closed, depending on the level of vertical integration for the firm. Open platforms will be developed by digital systems integrators such as Project Frog. These firms will develop the platform core and leverage the principles of industry 4.0 to organize the periphery into new digital ecosystems. Closed, internal platforms will be developed by vertically integrated firms such as RAD. These firms gain advantage from total control of system architecture and the ability to push the limits of technical change. While such a proposition should be tested, there are already further examples from industry such as Open Systems Lab (Parvin Citation2013) developing BuildX – an open-source software configuration platform – and the vertically-integrated firms BoKlok (Lessing and Brege Citation2018) developing internal closed product platforms. The start-up Katerra can make an interesting deviant case as a vertically-integrated company that began with a closed product platform but has recently positioned its Apollo Construct software platform as a hybrid between open and closed ecosystems. More research is needed to understand how open and closed platforms facilitate collaboration and new ecosystems within a digital manufacturing supply chain in construction. This work can study firm strategies for using platform development to become the dominant “operating system” for digitally-enabled manufacturing.
Conclusion
With this Festschrift paper, we theorize the connections between the construction industry structure, systemic innovation, and the mirroring trap. In so doing, we build on the pioneering work of Professor Raymond E. Levitt on systemic innovation and industry structure. Motivated by the systemic innovation example of digitally-enabled manufacturing currently, we conduct a longitudinal comparative case analysis of three entrepreneurial firms in the San Francisco Bay Area. We find that each of these firms used a different strategic approach to “mirror-breaking.” We unpack how each of the “mirror-breaking” strategies offers different benefits and challenges for the adoption of digitally-enabled manufacturing. All three approaches offer opportunities to redefine the system architecture and develop new products within that system. Furthermore, we note the need for hybrid roles that align with new system architecture knowledge, the emergence of new firm-level integration as opposed to project-based integration and the emergence of platforms as a new organizational structure to enable longitudinal integration.
In setting out our contribution articulating mirror-breaking strategies to enable digital manufacturing, we position this work as the first step in theory building. We do not suggest that the above forms of mirror-breaking are the only ways in which firms enable such systemic innovations. It is possible that other forms of mirror-breaking exist, beyond those observed in the three case studies. Nor do we argue that one approach is better than the others; more research is needed to understand the implications of each approach and to identify when construction firms should intentionally engage in mirror-breaking. The findings are also limited to the post hoc reconstruction of strategies as described by employees of these firms; it remains unclear if these strategies were intentionally undertaken to “break the mirror” or evolved over time in search of competitive advantages. Nonetheless, we find clear evidence that participants describe how their firms have taken distinct actions to break dependence between specialized industry knowledge and required work tasks, have developed systemic innovations to capture value from digital manufacturing technologies, believe their current organizational form is aligned or "re-mirrored" with their new system architecture, and are actively developing new products to capture value from this new system architecture.
Disclosure statement
No potential conflict of interest was reported by the authors.
Note
Notes
References
- Baldwin, C.Y. and Clark, K.B., 2000. Design rules: the power of modularity. Cambridge; London: MIT press.
- Bock, T. and Linner, T., 2015. Robotic industrialization: automation and robotic technologies for customized component, module, and building prefabrication. Cambridge: Cambridge University Press.
- Brockmann, C., Brezinski, H., and Erbe, A., 2016. Innovation in construction megaprojects. Journal of construction engineering and management, 142(11), 04016059.
- Brusoni, S., Prencipe, A., and Pavitt, K., 2001. Knowledge specialization, organizational coupling, and the boundaries of the firm: why do firms know more than they make? Administrative science quarterly, 46(4), 597.
- Bygballe, L.E., Dewulf, G., and Levitt, R.E., 2014. The interplay between formal and informal contracting in integrated project delivery. Engineering project organization journal, 5, 114.
- Bygballe, L.E., Håkansson, H., and Ingemansson, M., 2015. An industrial network perspective on innovation in construction. In: F. Orstavik, A. Dainty, and C. Abbott, eds. Construction innovation. Chichester: Wiley Online Library, 89–101.
- Colfer, L.J., and Baldwin, C.Y., 2016. The mirroring hypothesis: theory, evidence, and exceptions. Industrial and corporate change, 25(5), 709–738.
- Darley, J.M., and Latane, B., 1968. Bystander intervention in emergencies: diffusion of responsibility. Journal of personality and social psychology, 8(4p1), 377.
- Dossick, C., Osburn, L., and Neff, G., 2019. Innovation through practice. Engineering, construction and architectural management. doi:10.1108/ECAM-12-2017-0272
- Dubois, A. and Gadde, L., 2002a. The construction industry as a loosely coupled system: implications for productivity and innovation. Construction management and economics, 20(7), 621–631.
- Dubois, A. and Gadde, L.E., 2002b. Systematic combining: an abductive approach to case research. Journal of business research, 55(7), 553–560.
- Dym, C., and Levitt, R., 1991. Toward the integration of knowledge for engineering modeling and computation. Engineering with computers, 7(4), 209–224.
- Eccles, R.G., 1981. The quasifirm in the construction industry. Journal of economic behavior and organization, 2(4), 335–357.
- Eisenhardt, K.M., 1989. Building theories from case study research. Academy of management review, 14(4), 532–550.
- Ellen MacArthur Foundation, 2017. Achieving “growth within” – a €320-billion circular economy investment opportunity available to Europe up to 2025. Conker House Publishing Consultancy. Available from: https://www.ellenmacarthurfoundation.org/assets/downloads/publications/Achieving-Growth-Within-20-01-17.pdf
- Fergusson, K.J. 1993. Impact of integration on industrial facility quality. Unpublished dissertation. Stanford University.
- Fergusson, K.J. and Teicholz, P.M., 1996. Achieving industrial facility quality: integration is key. Journal of management in engineering, 12, 49–56.
- Flyvbjerg, B., 2006. Five misunderstandings about case-study research. Qualitative inquiry, 12(2), 219–245.
- Galbraith, J.R., 1974. Organization design: an information processing view. Interfaces, 4(3), 28–36.
- Gan, X., et al., 2018. Barriers to the transition towards off-site construction in China: an interpretive structural modeling approach. Journal of cleaner production, 197, 8–18, Elsevier Ltd.
- Gann, D.M., 1996. Construction as a manufacturing process? Similarities and differences between industrialized housing and car production in Japan. Construction management and economics, 14(5), 437–450, Routledge.
- Gann, D.M. and Salter, A.J., 2000. Innovation in project-based, service-enhanced firms: the construction of complex products and systems. Research Policy, 29(7–8), 955–972.
- Hall, D.M., Algiers, A., and Levitt, R.E., 2018. Identifying the role of supply chain integration practices in the adoption of systemic innovations. Journal of management in engineering, 34(6), 04018030.
- Hall, P. and Soskice, D., 2001. Varieties of capitalism: the institutional foundations of comparative advantage. New York: Oxford University Press.
- Harty, C., 2005. Innovation in construction: a sociology of technology approach. Building research and information, 33(6), 512–522.
- Henderson, R.M. and Clark, K.B., 1990. Architectural innovation: the reconfiguration of existing product technologies and the failure of established firms. Administrative science quarterly, 35(1), 9–30.
- Henisz, W.J., Levitt, R.E., and Scott, W.R., 2012. Toward a unified theory of project governance: economic, sociological and psychological supports for relational contracting. Engineering project organization journal, 2(1–2), 37–55.
- Howard, H.C., et al., 1989. Computer integration: reducing fragmentation in AEC industry. Journal of computing in civil engineering, 3(1), 18–32.
- Jansson, G., Johnsson, H., and Engström, D., 2014. Platform use in systems building. Construction management and economics, 32(1–2), 70–82.
- Jazdi, N., 2014. Cyber physical systems in the context of Industry 4.0. 2014 IEEE international conference on automation, quality and testing, robotics. IEEE, Cluj-Napoca, 1–4.
- Johnsson, H., 2013. Production strategies for pre-engineering in house-building: exploring product development platforms. Construction management and economics, 31(9), 941–958.
- Katila, R., Levitt, R.E., and Sheffer, D., 2018. Systemic innovation of complex one-off products: the case of green buildings. In Organization design (Vol. 40). 299–328.
- Lampel, J., 2011. Institutional dynamics of project-based creative organizations: Irving Thalberg and the Hollywood studio system. Advances in strategic management, 28, 445–466.
- Lampel, J. and Shamsie, J., 2003. Capabilities in motion: new organizational forms and the reshaping of the Hollywood Movie Industry*. Journal of management studies, 40(8), 2189–2210.
- Lavikka, R., et al., 2018. Fostering prefabrication in construction projects - case MEP in Finland. In: Proceedings from the European conference on product and process modelling (ECPPM 2018), Copenhagen.
- Lessing, J., 2006. Industrialised house-building: concept and processes. Licentiate thesis. Division of Design Methodology, Department of Construction Sciences, Lund University.
- Lessing, J. and Brege, S., 2015. Business models for product-oriented house-building companies – experience from two Swedish case studies. Construction Innovation, 15(4), 449–472.
- Lessing, J. and Brege, S., 2018. Exploration of industrialized building companies’ business models – a multiple case study of Swedish and North American companies. Journal of construction engineering and management, 144.
- Lessing, J., Stehn, L., and Ekholm, A., 2015. Industrialised house-building – development and conceptual orientation of the field. Construction innovation, 15(3), 378–399.
- Levitt, R.E., 2007. CEM research for the next 50 years: maximizing economic, environmental, and societal value of the built environment. Journal of construction engineering and management, 133(9), 619–628.
- Levitt, R.E., 2011. Towards project management 2.0. Engineering project organization journal, 1(3), 197–210.
- Levitt, R.E., Jin, Y., and Dym, C.L., 1991. Knowledge-based support for management of concurrent, multidisciplinary design. Artificial intelligence for engineering design, analysis and manufacturing, 5(2), 77–95.
- Levitt, R.E. and Kartam, N.A., 1990. Expert systems in construction engineering and management: state of the art. The knowledge engineering review, 5(2), 97–125.
- Levitt, R.E. and Sheffer, D. A. 2011. Innovation in modular industries: implementing energy-efficient innovations in US buildings. Proceedings of the energy, organizations and society conference. University of California, Davis, CA, 1–29.
- Lindgren, J., 2016. Diffusing systemic innovations: influencing factors, approaches and further research. Architectural engineering and design management, 12(1), 19–28.
- Lindgren, J., Emmitt, S., and Widén, K., 2018. Construction projects as mechanisms for knowledge integration. Engineering, construction and architectural management, 25(11), 1516–1533.
- Lobo, S. and Whyte, J., 2017. Aligning and reconciling: building project capabilities for digital delivery. Research policy, 46(1), 93–107.
- London, K. and Pablo, Z., 2017. An actor–network theory approach to developing an expanded conceptualization of collaboration in industrialized building housing construction. Construction management and economics, 35(8–9), 553–577,Routledge.
- Ma, Z., Zhang, D., and Li, J., 2018. A dedicated collaboration platform for integrated project delivery. Automation in construction, 86, 199–209, Elsevier.
- Maisel, S., 1963. A theory of fluctuations in residential construction starts. The American economic review, 53(3), 359–‐383.
- Malmgren, L., Jensen, P., and Olofsson, T., 2011. Product modeling of configurable building systems – a case study. Electronic journal of information technology in construction, 16, 697–712.
- Martishaw, S. and Sathaye, J., 2006. Quantifying the effect of the principal-agent problem in US residential energy use. Berkeley, California: Environmental Energy Technologies Division, University of California.
- Nam, C.H. and Tatum, C.B., 1989. Toward understanding of product innovation process in construction. Journal of construction engineering and management, 115(4), 517–534.
- Nam, C. and Tatum, C., 1988. Major characteristics of constructed products and resulting limitations of construction technology. Construction management and economics, 6(2), 133–147.
- Ozorhon, B., Oral, K., and Demirkesen, S., 2016. Investigating the components of innovation in construction projects. Journal of management in engineering, 32(3), 04015052.
- Papadonikolaki, E., 2018. Loosely coupled systems of innovation: aligning BIM adoption with implementation in Dutch construction. Journal of management in engineering, 34(6), 05018009.
- Parvin, A., 2013. Architecture (and the other 99%): open-source architecture and design commons. Architectural design, 83(6), 90–95.
- Powell, W., 1990. Neither market nor hierarchy: network forms of organization. Vol. 12. In: B.M. Staw and L.L. Cummings, eds. Research in organizational behavior. Greenwich, CT: JAI Press, 295–336.
- Pullen, T., Hall, D. M., and Lessing, J., 2019. White paper: a preliminary overview of emerging trends for industrialized construction in the United States, Version 1. ETH Zurich, Zurich.
- Reichstein, T., Salter, A.J., and Gann, D.M., 2005. Last among equals: a comparison of innovation in construction, services and manufacturing in the UK. Construction management and economics, 23(6), 631–644.
- Reichstein, T., Salter, A. J., and Gann, D. M., 2008. Break on through: sources and determinants of product and process innovation among UK construction firms. Industry and innovation, 15(6), 601–625, Taylor & Francis.
- Reve, T. and Levitt, R.E., 1984. Organization and governance in construction. International journal of project management, 2, 17–25.
- Sheffer, D.A. 2011. Innovation in modular industries: Implementing energy-efficient innovations in US buildings. Dissertation. Stanford University.
- Slaughter, E.S., 1998. Models of construction innovation. Journal of construction engineering and management, 124(3), 226–231.
- Steinhardt, D., et al., 2019. The structure of emergent prefabricated housing industries: a comparative case study of Australia and Sweden. Construction management and economics, 0(0), 1–19.
- Stinchcombe, A., 1959. Bureaucratic and craft administration of production: a comparative study. Administrative science quarterly, 4(2), 168–187.
- Tatum, C.B., 1986. Potential mechanisms for construction innovation. Journal of construction engineering and management, 112(2), 178–191.
- Taylor, J.E. and Levitt, R. E., 2004. Understanding and managing systemic innovation in project-based industries. Innovations: project management research. Newton Square, PA: Project Management Institute.
- Taylor, J.E. and Levitt, R.E. 2005. Inter-organizational knowledge flow and innovation diffusion in project-based industries. Proceedings of the 38th Annual Hawaii International Conference on System Sciences, Big Island, HI, USA: IEEE, 247c.
- Taylor, J. and Levitt, R., 2007. Innovation alignment and project network dynamics: an integrative model for change. Project management journal, 38 (3), 22–35.
- Tee, R., Davies, A., and Whyte, J., 2019. Modular designs and integrating practices: managing collaboration through coordination and cooperation. Research policy, 48(1), 51–61, Elsevier.
- Thompson, E.P., 1967. Time, work-discipline, and industrial capitalism. Past and present, 38(1), 56–97.
- Toole, T.M., Hallowell, M., and Chinowsky, P., 2013. A tool for enhancing innovation in construction organizations. Engineering project organization journal, 3(1), 32–50.
- Utterback, J.M., 1996. Mastering the dynamics of innovation: How companies can seize opportunities in the face of technological change. Boston: Harvard Business School Press.
- Whyte, J., 2019. How digital information transforms project delivery models. Project management journal, 50(2), 177–194, SAGE Publications.
- Whyte, J. and Levitt, R., 2011. Information management and the management of projects. In: P.W.G. Morris, J.K. Pinto, and J. Soderlund, eds. The Oxford handbook of project management. New York, NY: Oxford University Press.
- Wikberg, F., Olofsson, T., and Ekholm, A., 2014. Design configuration with architectural objects: linking customer requirements with system capabilities in industrialized house-building platforms. Construction management and economics, 32(1–2), 196–207.
- Yin, R.K., 2018. Case study research and applications: design and methods. 6th ed. Los Angeles, CA: Sage publications.