Abstract
Nanoliposomes are microscopic vesicles composed of phospholipid bilayers entrapping one or more aqueous compartments. Their unique properties have triggered numerous applications in several scientific and technological fields. Nanoliposomes can provide controlled release of various bioactive agents, including food ingredients and nutraceuticals, at the right place and the right time. Therefore, they increase the effectiveness and cellular uptake of the encapsulated material. Reactive, sensitive, or volatile additives (vitamins, enzymes, antioxidants, slimming agents, etc.) can be turned into stable ingredients using nanoliposomes. This article reviews various aspects of nanoliposomes including currently available preparation methods, and their application in food technology.
INTRODUCTION
Among the new trends towards a healthier way of living, new functional food items are constantly being developed that require unique technologies for incorporating health-promoting ingredients into food systems. However, these ingredients may slowly degrade and lose their activity, or become hazardous due to oxidation reactions. They can also react with other components in the food system, which may limit bioavailability, or change the color or taste of a product, allowing the food item to become prone to spoilage and deterioration. In many cases, microencapsulation can be used to overcome these challenges.[Citation1]
Microencapsulation is defined as a technology of packing solids, liquids, or gaseous materials in miniature, sealed capsules that can release their contents at controlled rates under specific conditions.[Citation2] The coated material is known as the core material, actives, fill, internal phase, or payload. The coating material can be called the capsule, wall material, membrane, carrier, or shell.[Citation3] Microcapsules are small vesicles that may range from sub-micron to several millimeters in size and are ideally spherical.[Citation2] However, their shape is heavily influenced by the structure of the original encapsulated material and the ingredients from which the capsules are made. The capsule can be made of sugars, gums, proteins, natural and modified polysaccharides, lipids, phospholipids and synthetic polymers.[Citation3–5]
In the food industry, microencapsulation is used to stabilize and control the release of core material (both the rate of release and the commencement of release), and to separate reactive or incompatible components of formulation. The microcapsules offer the food processor many advantages by means of protecting sensitive food components, ensuring against nutritional loss, utilize otherwise sensitive ingredients, incorporate pulsative or time-release mechanisms into the formulation, mask or preserve flavors and aromas, and transform liquids into easily handled solid ingredients.[Citation2,Citation4]
Until recently, the protection of bioactive agents has been investigated exclusively using microencapsulation systems. However, to provide site-specific controlled release is a key functionality that can be provided much more efficiently by employing nanocarrier technologies. Advancements in nanotechnology have made it possible to manufacture and analyse sub-micrometric bioactive carriers on a routine basis. The delivery of bioactives to target sites inside and outside the body and their release properties is directly affected by particle size. Compared to micron-sized carriers, nanocarriers provide more surface area and have the potential to increase solubility, enhance bioavailability, improve controlled release, and enable precision targeting of the encapsulated materials to a greater extent. As a consequence of improved stability and targeting, the amount of material required to exert a specific effect when encapsulated inside the nanocarriers is much less than the amount required when unencapsulated. A time-controlled and targeted release improves the effectiveness of bioactives, broadens their application range and ensures optimal dosage, thereby improving cost-effectiveness of the product. Reactive or sensitive material can be turned into stable ingredients through encapsulation or entrapment by nanocarrier systems such as nanoliposomes.[Citation5]
Numerous encapsulation techniques have been developed on micro-and nano-scales.[Citation2,[Citation5–8] In general, three steps are involved in the encapsulation of bioactive agents: formation of the wall around the material to be encapsulated; ensuring that undesired leakage does not occur; and ensuring that undesired materials are kept out. The encapsulation techniques include spray drying, spray chilling (or spray cooling), extrusion coating, fluidized bed coating, liposome entrapment, coacervation, inclusion complexation, centrifugal extrusion, electrostatic deposition, and rotational suspension separation.[Citation3,Citation4] In this article, we focus on liposome and nanoliposome technologies and their applications in the encapsulation of food ingredients. As an example of nanoliposome application in food area, encapsulation of antioxidants is explained in the final section of the manuscript due to the high importance of antioxidants not only in food area, but also in pharmaceutics and cosmetics as well.
LIPOSOMES AND NANOLIPOSOMES
During the last few years, liposome technology has generated much interest in the food industry. Liposomes are colloidal particles consisting of a membraneous system formed by lipid bilayers encapsulating aqueous space(s). They can be prepared using completely natural ingredients or indigenous molecules found in our body; thus they are biocompatible and are acceptable for human consumption.[Citation3,Citation5,Citation8] A recent definition proposed at a conference in the field of liposomology, describes liposomes as “closed, continuous bilayered structures made mainly of lipid and/or phospholipid molecules”.[Citation9] They are under intensive investigation and development by the pharmaceutical, cosmetic, and food industries as micro- and nanocarrier systems for the protection and delivery of bioactive agents. Recent studies suggest that liposomes are even naturally present in the very first food we take, the breast milk.[Citation10,Citation11]
Liposomes are composed of one or more lipid and/or phospholipid bilayers, and they can contain other molecules such as proteins or carbohydrates in their structure. A liposome composed of a number of concentric bilayers is known as a multilamellar vesicle (MLV), while one composed of many small non-concentric vesicles encapsulated within a single lipid bilayer is known as a multivesicular vesicle (MVV).[Citation12] Another type of liposome is known as a unilamellar vesicle (ULV), which contains one single lipidic bilayer and can be small unilamellar vesicle (SUV, less than 100nm) or large unilamellar vesicle (LUV, larger than 100nm) (). Recently another type of liposome, known as a double-bilayer vesicle, has been introduced, which consists of two bilayer membranes.[Citation13] This type of liposome has the advantages of improved stability and delayed, sustained-release of their contents ().
Figure 1 Different types of liposomes based on size and number of lamella. A cross-section of a unilamellar vesicle (ULV) is also shown (see text for details).
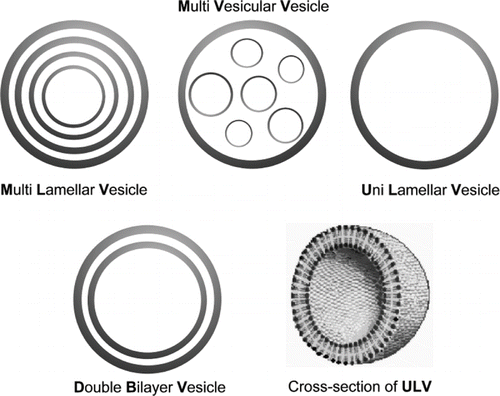
Liposomes were first described by Bangham and coworkers in 1965 at Cambridge University[Citation14] and since then there have been considerable developments in their preparation, characterization and utilization as carriers of drugs and other bioactive materials.[Citation15–17] Owing to the possession of both lipid and aqueous phases, liposomes can be utilised in the entrapment, delivery, and release of water-soluble, lipid-soluble, and amphiphilic materials. This is an important advantage, which not all encapsulation systems possess. The term nanoliposome has recently been introduced to exclusively refer to nanoscale lipid vesicles,[Citation17] since liposome is a general word encompassing many classes of lipid vesicles whose diameter range from tens of nanometers to several micrometers. Nanoliposomes and liposomes possess the same physical, structural, and thermodynamic properties that are mainly determined by their components and environment. Manufacture of both liposomes and nanoliposomes requires input of energy to a dispersion of lipid/phospholipid molecules in an aqueous medium.[Citation8,Citation18] The underlying mechanism for the formation of liposomes and nanoliposomes is basically the hydrophilic–hydrophobic interaction between phospholipids and water molecules. Due to the fact that liposomes are dynamic entities, which tend to aggregate and/or fuse and as a result increase in size, vesicles prepared in nanometric size ranges may end up becoming micrometric particles upon storage. Nevertheless, nanoliposomes should have adequate stability to retain their sizes and could be defined as ‘bilayer lipid vesicles possessing and maintaining nanometric size ranges during storage and application’.[Citation17]
The unique properties of liposomes have triggered numerous applications in different fields of science and technology, from basic studies of membrane structure/function to bioactive agent delivery.[Citation16–19] In agriculture, they can be used to improve the efficacy of different biocides and to deliver some essential nutrients.[Citation19,Citation20] There are many potential applications for liposomes and nanoliposomes in the food industry, ranging from the protection of sensitive ingredients to increasing the efficacy of food additives. An example of liposome application in food is the entrapment of proteolytic enzymes in cheese production.[Citation21–23] Application of liposomal enzymes can produce a cheese with good texture and flavour in half the normal time, with the overall enzyme requirement reduced 100-fold.[Citation24,Citation25] Another advantage of liposomal systems compared to other encapsulation strategies is that they are made of ingredients that are beneficial for our health.[Citation26–28] Recent research into the biological functions of phospholipids and sphingolipids has identified several health benefits, including liver protection,[Citation26,Citation27,Citation29] memory improvement,[Citation30,Citation31] and inhibition of cholesterol absorption.[Citation32]
The types of food ingredients that can be encapsulated in liposomes and nanoliposomes are listed below[Citation3,Citation8,Citation19,Citation23]:
-
flavoring agents such as oils, spices, seasoning, and sweeteners;
-
acids, alkalis, and buffers;
-
essential oils, amino acids, vitamins, and minerals;
-
redox agents (bleaching, maturing), and colorants;
-
enzymes or microorganisms;
-
leavening agents;
-
antioxidants, antimicrobials, and preservatives;
-
cross-linking and setting agents; and
-
agents with undesirable flavors and odors (e.g., omega 3).
LIPOSOME AND NANOLIPOSOME MANUFACTURE
Generally liposomes can be classified either by their structural properties or by their preparation method. The main types of lipid vesicles and their characteristics are outlined in .[Citation2,Citation13,Citation16,Citation17,Citation33] The permeability of liposomes and nanoliposomes can be influenced by their phospholipid content; their method of preparation; and their number of lamellae. Liposome size and morphology has a strong influence on their distribution within food systems. In addition, liposomes that will leak and release their contents at a particular temperature or pH have been designed, and they can be used very conveniently in food systems. The encapsulated material is released under the influence of a specific stimulus at a specified stage. For instance, flavors and nutrients may be released upon consumption, whereas sweeteners that are susceptible to heat may be released toward the end of baking; thus, preventing undesirable caramelization in the baked product.[Citation2,Citation34] Therefore, liposomes have tremendous scope for modification, enabling them to be tailored for a wide variety of different applications and be targeted to the required site inside or outside the body.[Citation5] However, their high cost of preparation limits their application in food industry.[Citation15,Citation35] The cost of manufacture of liposomal products can be brought down by reducing the number of steps, chemicals and time it takes to produce these formulations into products that are suitable for human or animal use.
Table 1 The main types of liposomes and their characteristics
There are several methods that may be used to produce liposomes, and a number of excellent books and reviews have been published that provide details of the more common production techniques.[Citation17,Citation18,Citation36,Citation37,Citation38] The standard preparation procedure is through the evaporation of a chloroform/methanol solution of phospholipid, cholesterol, and other hydrophobic compounds, to produce a thin film. Addition of an aqueous phase and hydrophilic compounds followed by input of sufficient amount of mechanical or thermal energy, causes bilayer sheets of the hydrophobic components to separate from the bulk and form liposomes.[Citation17,Citation18,Citation39] The preparation methods of liposomes and nanoliposomes generally involve utilisation of non-food-grade solvents and detergents for solubilisation or dissolution of the lipid and/or phospholipid ingredients.[Citation16,Citation17,Citation18,Citation40,Citation41] Until recently, large-scale production of liposomes and nanoliposomes was limited by poor encapsulation efficiencies, lack of a continuous production process, and the use of organic solvents.[Citation42] These solvents not only affect the structure and stability of the entrapped substance, but they will also remain in the final encapsulation formulation, thus contributing to toxicity and influencing the stability of the liposomal system.[Citation43–47] Another issue in the liposomal encapsulation for the food industry is the scaling up of the preparation method at acceptable levels and costs. These problems can be addressed by employing a new preparation method known as the heating method by which liposomes and nanoliposomes (in addition to some other carrier systems) can be prepared in one-step using a single apparatus in the absence of potentially toxic solvents in less than an hour.[Citation8,Citation18,Citation48,Citation49] The heating method and associated apparatus have also resulted in one patent so far.[Citation50] This method is economical and capable of manufacturing bioactive carriers, including liposomes and nanoliposomes, with a superior monodispersity and storage stability using a simple protocol. Another important feature of the method is that it can be adapted from small to industrial scales. The heating method is obviously most suitable for production of carrier systems for different in vitro and in vivo applications and involves heating and stirring (less than 1000 rpm) the carrier ingredients, in the presence of a plyol, at 40–120°C, based on the properties of the ingredients, presence or absence of cholesterol, and type of material to be entrapped.[Citation8,Citation18,Citation48,Citation49,Citation50] Recently, Mozafari and his team showed that nanoliposomes prepared by the heating method are completely non-toxic towards cultured cells while nanoliposomes prepared by a conventional method using volatile solvents showed significant levels of cytotoxicity.[Citation49] A further improved version of the heating method, called Mozafari method, has recently been employed for the encapsulation and targeted delivery of the food-grade antimicrobial nisin.[Citation51]
Another method of liposome production without using toxic solvents is the microfluidization technique[Citation52–56] using a microfluidizer equipment.[Citation57] This equipment has been traditionally used in the pharmaceutical industry to make liposomal formulations[Citation52] and pharmaceutical emulsions.[Citation58] More recently, Jafari et al. employed the microfluidizer to produce flavor emulsions or homogenized milk.[Citation59] Microfluidization works on the principle of dividing a pressure stream into two parts, passing each part through a fine orifice, and directing the flows at each other inside the chamber of microfluidizer.[Citation59,Citation60] Within the interaction chamber, cavitation, along with shear and impact, reduces size of the particles. The microfluidizer uses high pressures (up to l0000 psi) to guide the flow stream through microchannels toward the impingement area,[Citation53] which creates a very high shearing action that potentially can damage the structure/function of material to be encapsulated.[Citation61–65] Another disadvantage of the microfluidization method is material loss, contamination and being relatively difficult to scale-up.[Citation55] The heating method, on the other hand, has none of these disadvantages and hence is a better choice to be used in the production of pharmaceutical, cosmetic and food preparations.
ENCAPSULATION OF CHEESE-RIPENING ENZYMES
Another unique property of lipid vesicles is the targeted delivery of their content in specific parts of the food system. For instance, it has been shown that liposome-encapsulated enzymes concentrate preferably in the curd during cheese formation, whereas nonencapsulated enzymes are usually distributed evenly in the whole milk mixture, which leads to very low (2–4%) retention of the flavor-producing enzymes in the curd (for a recent review, see Desai and Park[Citation34]). Early attempts at enzyme encapsulation for accelerated cheese ripening involved the use of milk fat. Magee and Olsen evaluated milk fat fractions as an encapsulating system for glucose or peptone solutions.[Citation66] They achieved encapsulation efficiencies of between 80% and 90% for their microcapsules. Using this system, cell free extracts (CFE) derived from L. lactis ssp. diacetylactis were encapsulated and added into a low fat Cheddar type cheese.[Citation66] Approximately eight times more diacetyl and acetoin were produced in cheese cooked at 30 or 33°C containing encapsulated CFE. However, cooking cheese to 35°C disrupted the microcapsules causing early release of CFE with little or no impact on levels of the flavour compounds.
An alternative method to encapsulate enzymes is the use of lipid vesicles in the form of liposomes or nanoliposomes.[Citation21,Citation23,Citation24,Citation25] The potential advantages of lipid vesicles over other methods of enzyme encapsulation for cheese applications are (1) they can be made from ingredients that are naturally present in cheese; (2) they protect casein from early hydrolysis during cheese production; (3) partition well in the curd; and (4) they can be manufactured on industrial scales with food grade properties by recently developed procedures such as Mozafari method. The enzyme encapsulation efficiency of lipid vesicles depends upon a number of factors; namely lipid concentration, phospholipid type, and purity of the enzyme to be encapsulated.[Citation23] Enzymes usually orient between bilayers in multilamellar vesicles and are therefore likely to diffuse more quickly than if located in the aqueous core. Most of the lipid vesicles appear to be associated with the milk fat globule membrane (MFGM), some are found in whey pockets while some negatively charged vesicles are found within the casein matrix.[Citation23–25] Those liposomes grouped close to the MFGM appear in time to interact with this membrane resulting in their destabilisation and those in whey pockets tend to aggregate and form large unilamellar vesicles before rupturing.[Citation23–25] Some cheese studies have utilized small unilamellar vesicle type liposomes (SUV) produced from MLV by sonication.[Citation23] However, SUV liposomes have limited potential in dairy applications as their enzyme encapsulation efficiencies are poor due to their small internal core. Consequently, majority of the studies have used MLV type liposomes produced using different methods some of which explained above (also see references[Citation21,Citation24,Citation25,Citation39]).
ENCAPSULATION OF ANTIOXIDANTS
There have been several reports on the use of liposomes and nanoliposomes for the oral administration of drugs such as cyclosporin[Citation67] and insulin[Citation68,Citation69] as an alternative to parenteral drug delivery. Drugs encapsulated into liposomes can be protected from digestion in the stomach and show significant level of absorption in the gastrointestinal tract. This leads to the enhanced bioavailability of drugs and other bioactive agents, as reported by Takahashi and co-workers.[Citation70] Liposomes and nanoliposomes, therefore, have great potential not only in advanced medical and pharmaceutical fields, but also in other fields such as the food industry. Recently, several functional food products have been introduced to the market.[Citation70] However, some of the active ingredients of these products are decomposed by stomach digestion and are not absorbed into the body efficiently.[Citation71–73] In order to improve the absorption of such active material into the body, it is essential to prevent decomposition/degradation and to improve intestinal absorption. Um et al.[Citation74] reported that unilamellar vesicles with mean particle size of 1 μm or less are appropriate for efficient intestinal absorption.
A typical example of nanoliposome application in food area is the encapsulation of antioxidants, which possess many health effects, as well as retarding the oxidation (degradation) of nutrients in foods. These important class of bioactive compounds possess very limited ability to cross cell membranes and are rapidly cleared from cells.[Citation8] A possible method for the protection of antioxidants and enhancing their bioavailability is to employ nanoliposome technology.[Citation75–78] Antioxidants may be utilised for two main purposes, to protect the sensory and nutritive quality of the food and/or to protect the body against certain diseases (for a recent review see Mozafari et al.[Citation8]). The usage of antioxidants has become favorable because of the new trends in the food industry such as the replacement of saturated fat with unsaturated fats in the diet.[Citation79,Citation80] Unfortunately, unsaturated fats are easily oxidized, which can lead to deterioration in food quality and to the generation of toxic products. Furthermore, oxidative degradation can be increased if the lipid is incorporated into food emulsions such as margarines, spreads, and salad dressings, since the aqueous phase can allow the rapid transmission of oxidants such as oxygen and metal ions. These problems can be minimized by incorporating antioxidants into the lipid phase of liposomes, however, the most effective antioxidants are synthetic and there is increasing pressure to phase these out.[Citation25]
The natural lipid-soluble agent α-tocopherol (Vitamin E) can be used as an antioxidant and it can be regenerated from its oxidized form using ascorbic acid. However, in food systems, α-tocopherol is soluble in the lipid phase and cannot interact with water-soluble ascorbate.[Citation79] Consequently, the food industry uses lipid soluble derivatives such as ascorbyl palmitate. However, these derivatives still have solubility problems, requiring heat to dissolve them, and also they are not natural. The effectiveness of α-tocopherol as a food antioxidant can be increased by incorporating it into liposomes[Citation25] or nanoliposomes.[Citation8]
The liposomal system was used as an emulsifier to stabilize an oil-in-water food emulsion.[Citation79] α -tocopherol added in this way was found to be much more effective than when dissolved in the bulk oil phase, presumably because it traps oxygen radicals and interrupts the oxidative chain reaction at an earlier stage.[Citation25] α-Tocopherol was incorporated into the liposome bilayer, whereas the ascorbate is entrapped in the aqueous interior; the encapsulated systems were added to the aqueous phase and encouraged to accumulate at the water-oil interface. Thus, the antioxidant can be targeted at the site where oxidative reactions generally occur, and also avoid the reaction of ascorbate with other food ingredients.[Citation79]
The control of lipid oxidation of foods, primarily for extending shelf life, has been extensively studied over many years.[Citation8,Citation81,Citation82] Many of these studies involved encapsulation to protect the antioxidants from degradation throughout the processing and storage stages. It is becoming increasingly apparent that antioxidants present in foods may also play a role in the control of several chronic and age-related disorders.[Citation83–86] Thus, the design of encapsulation systems that can not only protect foods from oxidation but also play a role in the control of disease is required. Nanoencapsulation systems such as nanoliposomes are especially relevant for oral delivery of antioxidants owing to their stability in the gastrointestinal tract[Citation83–86]. The potential of these nanoencapsulation systems is yet to be explored in the utilisation of the protective effect of antioxidants both in food products and in the body.
CONCLUSIONS
Liposomes and nanoliposomes offer a versatile approach for the encapsulation, protection, and controlled release of sensitive bioactives. They can be used to improve the solubility of lipophilic compounds in aqueous solutions or hydrophilic compounds in hydrophobic systems. Of special interest for the food industry is the fact that they can be prepared from naturally occuring food materials. Recent research into the biological functions of liposomal ingredients (e.g., phospholipids and sphingolipids) has identified a number of health benefits including liver protection, memory improvement, and inhibition of cholesterol absorption. Problems associated with liposome and nanoliposome manufacture are now solved by the development of new preparation techniques, such as the heating method and Mozafari method. These techniques allow one-step, large scale production, and the avoidance of organic solvents and detergents during encapsulation. Taking all these into account, the development of industrial applications of liposomes and nanoliposomes in food processing comparable to those in the fields of pharmaceutics and cosmetics can be expected.
REFERENCES
- Schrooyen , P.M.M. , Van Derr Merr , R. and De Kruif , G. 2001 . Microencapsulation: Its Application in Nutrition . Proceed. Nut. Soc. , 60 : 475 – 479 .
- Dziezak , J.D. 1988 . Microencapsulation and Encapsulated Ingredients . Food Technol. , 42 ( 4 ) : 136 – 151 .
- Gibbs , B.F. , Kermasha , S. , Alli , I. and Mulligan , C.N. 1999 . Encapsulation in the Food Industry: A Review . Int. J. Food Sci. Nut. , 50 : 213 – 224 .
- Vilstrup , P. , ed. 2001 . Microencapsulation of Food Ingredients , Surrey, England : Leatherhead Pub .
- Mozafari , M.R. 2006 . “ Bioactive Entrapment and Targeting using Nanocarrier Technologies: An Introduction ” . In Nanocarrier Technologies: Frontiers of Nanotherapy , Edited by: Mozafari , M.R . 1 – 16 . The Netherlands : Springer .
- Gouin , S. 2004 . Micro-encapsulation: Industrial Appraisal of Existing Technologies and Trends . Trends Food Sci. Technol. , 15 : 330 – 347 .
- Yurdugul , S. and Mozafari , M.R. 2004 . Recent Advances in Micro- and Nano-encapsulation of Food Ingredients . Cell. Mol. Biol. Lett. , 9 ( S2 ) : 64 – 65 .
- Mozafari , M.R. , Flanagan , J. , Matia-Merino , L. , Awati , A. , Omri , A. , Suntres , Z. and Singh , H. 2006 . Recent Trends in the Lipid-based Nanoencapsulation of Antioxidants and their Role in Foods . J. Sci. Food Agricul. , 86 ( 13 ) : 2038 – 2045 .
- Mozafari , M.R. , Reed , C.J. , Rostron , C. , Kocum , C. and Piskin , E. 2002 . Formation and Characterisation of Non-toxic Anionic Liposomes for Delivery of Therapeutic Agents to the Pulmonary Airways . Cell. Mol. Biol. Lett. , 7 : 243 – 244 .
- Keller , B.C. , Faulkner , G. and Lasic , D.D. 2000 . Liposomes in Breastmilk . Agro Food Ind. HiTech , 11 : 6 – 8 .
- Keller , B.C. 2001 . Liposomes in Nutrition . Trends Food Sci. Technol. , 12 : 25 – 31 .
- Kim , S. , Turker , M.S. , Chi , E.Y. , Sela , S. and Martin , G.M. 1983 . Preparation of Multivesicular Liposomes . Biochim. Biophys. Acta , 728 : 339 – 348 .
- Zawada , Z.H. 2004 . Vesicles with a Double Bilayer . Cell. Mol. Biol. Lett. , 9 ( 4A ) : 589 – 602 .
- Bangham , A.D. , Standish , M.M. and Watkins , J.C. 1965 . Diffusion of Univalent Ions Across the Lamellae of Swollen Phospholipids . J. Mol. Biol. , 13 : 238 – 252 .
- Kirby , C.J. 1990 . Delivery System for Enzymes . Chem. Britain , 26 : 847 – 850 .
- New , R.R.C. 1990 . Liposomes a Practical Approach , Oxford, , UK : IRL/Oxford University Press .
- Mozafari , M.R. and Mortazavi , S.M. , eds. 2005 . Nanoliposomes: from Fundamentals to Recent Developments , Oxford, , UK : Trafford Pub. Ltd .
- Mozafari , M.R. 2005 . Liposomes: an Overview of Manufacturing Techniques . Cell. Mol. Biol. Lett. , 10 : 711 – 719 .
- Lasic , D.D. 1993 . Liposomes from Physics to Applications , 507 – 516 . Amsterdam : Elsevier .
- Pons , M. and Estelrich , J. 1996 . Liposomes as an Agrochemical Tool: Optimization of their Production . Ind. Crops Produc. , 5 : 203 – 208 .
- Piard , J.C. , ElSoda , M. , Alkhalaf , W. , Rousseau , M. , Desmazeaud , M. , Vassal , L. and Gripon , J.C. 1986 . Acceleration of Cheese Ripening with Liposome Entrapped Proteinase . Biotechnol. Lett. , 8 : 241 – 246 .
- Walde , P. and Ichikawa , S. 2001 . Enzymes Inside Lipid Vesicles: Preparation, Reactivity, and Applications . Biomol. Eng. , 18 : 143 – 177 .
- Wilkinson , M.G. and Kilcawley , K.N. 2005 . Mechanism of Incorporation and Release of Enzymes into Cheese during Ripening . Int. Dairy J. , 15 : 817 – 830 .
- Kirby , C.J. , Brooker , B.E. and Law , B.A. 1987 . Accelerated Ripening of Cheese using Liposome-Encapsulated Enzyme . Int. J. Food Sci. Technol. , 22 : 355 – 375 .
- Kirby , C.J. 1993 . “ Controlled Delivery of Functional Food Ingredients: Opportunities for Liposomes in the Food Industry ” . In Liposome Technology , Edited by: Gregoriadis , G . 215 – 232 . London : CRC Press .
- Thompson , A.K. and Singh , H. 2006 . Preparation of Liposomes from Milk Fat Globule Membrane Phospholipids using a Microfluidizer . J. Dairy Sci. , 89 ( 2 ) : 410 – 419 .
- Thompson , A.K. , Hindmarsh , J.P. , Haisman , D. , Rades , T. and Singh , H. 2006 . Comparison of the Structure and Properties of Liposomes Prepared from Milk Fat Globule Membrane and Soy Phospholipids . J. Agr. Food Chem. , 54 ( 10 ) : 3704 – 3711 .
- Huwiler , A. , Kolter , T. , Pfeilschifter , J. and Sandhoff , K. 2000 . Physiology and Pathophysiology of Sphingolipid Metabolism and Signaling . Biochim. Biophys. Acta , 1485 : 63 – 99 .
- Koopman , J. , Turkish , V. and Monto , A. 1985 . Infant Formula and Gastrointestinal Illness . Am. J. Public Health , 75 : 477 – 480 .
- Crook , T. , Tinklenberg , J. , Yesavage , J. , Petrie , W. , Nunzi , M. and Massari , D. 1991 . Effects of Phosphatidylserine in Age-associated Memory Impairment . Neurology , 41 : 644 – 649 .
- Crook , T. , Petrie , W. , Wells , C. and Massari , D. 1992 . Effects of Phosphatidylserine in Alzheimer's Disease . Psychopharmacol. Bull. , 28 : 61 – 66 .
- Peel , M. 1999 . Liposomes Produced by Combined Homogenization/Extrusion . GIT Lab. J. , 3 : 37 – 38 .
- Segota , S. and Tezak , D. 2006 . Spontaneous Formation of Vesicles . Adv. Colloid Int. Sci. , 121 ( 1–3 ) : 51 – 75 .
- Desai , K.G.H. and Park , H.J. 2005 . Recent developments in microencapsulation of food ingredients . Drying Tech. , 23 ( 7 ) : 1361 – 1394 .
- Thompson , A.K. , Haisman , D. and Singh , H. 2006 . Physical Stability of Liposomes Prepared from Milk Fat Globule Membrane and Soya Phospholipids . J. Agric. Food Chem. , 54 : 6390 – 6397 .
- Watwe , R. and Bellare , J. 1995 . Manufacture of Liposomes: A Review . Curr. Sci. , 68 : 715 – 724 .
- Betageri , G.V. and Kulkarni , S.B. 1999 . “ Preparation of Liposomes ” . In Microspheres, Microcapsules and Liposomes. Vol. 1: Preparation and Chemical Applications , Edited by: Arshady , R . 489 – 521 . London, , UK : Citus Books .
- Frezard , F. 1999 . Liposomes: From Biophysics to the Design of Peptide Vaccines . Braz. J. Med. Biol. Res. , 32 : 181 – 189 .
- Picon , A. , Gaya , P. , Medina , M. and Nunez , M. 1994 . The Effect of Liposome Encapsulation of Chymosin Derived by Fermentation on Manchego Cheese Ripening . J. Dairy Sci. , 77 : 16 – 23 .
- Lasic , D.D. 1988 . The Spontaneous Formation of Unilamellar Vesicles . J. Colloid Interface Sci. , 124 ( 2 ) : 428 – 435 .
- Lasic , D.D. , Joannic , R. , Keller , B.C. , Frederik , P.M. and Auvray , L. 2001 . Spontaneous Vesiculation . Adv. Colloid Interface Sci. , 89–90 : 337 – 349 .
- Jackson , L.S. and Lee , K. 1991 . Microencapsulation and the Food Industry . Lebensm. Wiss. Technol. , 24 : 289 – 297 .
- Vemuri , S. and Rhodes , C.T. 1995 . Preparation and Characterization of Liposomes as Therapeutic Delivery Systems: A Review . Pharm. Acta Helv. , 70 : 95 – 111 .
- Kikuchi , H. , Carlsson , A. , Yachi , K. and Hirota , S. 1991 . Possibility of Heat Sterilisation of Liposomes . Chem. Pharm. Bulletin , 39 ( 4 ) : 1018 – 1022 .
- Kikuchi , H. , Yamauchi , H. and Hirota , S. 1994 . A Polyol Dilution Method for Mass Production of Liposomes . J. Liposome Res. , 4 : 71 – 91 .
- Cortesi , R. , Esposito , E. , Gambarin , S. , Telloli , P. , Menegatti , E. and Nastruzzi , C. 1999 . Preparation of Liposomes by Reverse-phase Evaporation Using Alternative Organic Solvents . J. Microencapsul. , 16 : 251 – 256 .
- Dwivedi , A.M. 2002 . Residual Solvent Analysis in Pharmaceuticals . Pharm. Tech. Europe , 14 : 26 – 28 .
- Mozafari , M.R. , Reed , C.J. and Rostron , C. 2007 . Cytotoxicity Evaluation of Anionic Nanoliposomes and Nanolipoplexes Prepared by the Heating Method Without Employing Volatile Solvents and Detergents . Pharmazie , 62 ( 3 ) : 205 – 209 .
- Mortazavi , S.M. , Mohammadabadi , M.R. , Khosravi-Darani , K. and Mozafari , M.R. 2007 . Preparation of liposomal gene therapy vectors by a scalable method without using volatile solvents or detergents . J. Biotechnol. , 129 : 604 – 613 .
- Mozafari , M.R. Method and apparatus for producing carrier complexes . UK Patent No. GB 0404993.8, Int. Appl. No. PCT/GB05/000825 (03/03/2005) . 2005 .
- Colas , J.C. , Shi , W. , Rao , V.S.N.M. , Omri , A. , Mozafari , M.R. and Singh , H. 2007 . “ Microscopical investigations of nisin-loaded nanoliposomes prepared by Mozafari method and their bacterial targeting ” . In Micron
- Vemuri , S. , Yu , C.D. , Wangsatorntanakun , V. and Roosdorp , N. 1990 . Large-scale Production of Liposome by a Microfluidizer . Drug Dev. Ind. Pharm. , 16 ( 15 ) : 2243 – 2256 .
- Kim , H.Y. and Baianu , I.C. 1991 . Novel Liposome Microencapsulation Techniques for Food Applications . Trends Food Sci. Technol. , 2 : 55 – 61 .
- Chen , R.H. , Win , H.P. and Fang , H.J. 2001 . Vesicle Size, Size Distribution, Stability, and Rheological Properties of Liposomes Coated with Water-soluble Chitosans of Different Molecular Weights and Concentrations . J. Liposome Res. , 11 : 211 – 228 .
- Maa , Y.F. and Hsu , C.C. 1999 . Performance of Sonication and Microfluidization for Liquid-liquid Emulsification . Pharm. Dev. Technol. , 4 : 233 – 240 .
- Zheng , S. , Alkan-Onyuksel , H. , Beissinger , R.L. and Wasan , D.T. 1999 . Liposome Microencapsulation Without Using any Organic Solvent . J. Dispersion Sci. Technol. , 20 : 1189 – 1203 .
- Reineccius , G.A. 1994 . “ Flavour Manufacturing ” . In Source Book of Flavours , Edited by: Reineccius , G.A . 572 – 576 . New York : Chapman and Hall .
- Silvestri , S. , Ganguly , N. and Tabibi , E. 1992 . Predicting the Effect of Nonionic Surfactants on Dispersed Droplet Radii in Submicron Oil-in-water Emulsions . Pharm. Res. , 9 ( 10 ) : 1347 – 1350 .
- Jafari , S.M. , He , Y.H. and Bhandari , B. 2006 . Nano-emulsion Production by Sonication and Microfluidization — A Comparison . Int. J. Food Prop. , 9 ( 3 ) : 475 – 485 .
- Geciova , J. , Bury , D. and Jelen , P. 2002 . Methods for disruption of microbial cells for potential use in the dairy industry – a review . Int. Dairy J. , 12 : 541 – 553 .
- Silvestri , S. , Gabrielson , G. and Wu , L.L. 1991 . Effect of Terminal Block on the Microfluidization Induced Degradation of Model ABA Block Copolymer . Int. J. Pharm. , 71 : 65 – 71 .
- CenciaRohan , L. and Silvestri , S. 1993 . Effect of Solvent System on Microfluidization-induced Mechanical Degradation . Int. J. Pharm. , 95 : 23 – 28 .
- Bodmeier , R. and Chen , H. 1993 . Hydrolysis of Cellulose Acetate and Cellulose Acetate Butyrate Pseudolatexes Prepared by a Solvent Evaporation Microfluidization Method . Drug Dev. Ind. Pharm. , 19 ( 5 ) : 521 – 530 .
- Lagoueyte , N. and Paquin , P. 1998 . Effects of Microfluidization on the Functional Properties of Xanthan Gum . Food Hydrocolloids , 12 ( 3 ) : 365 – 371 .
- Kasaai , M.R. , Charlet , G. , Paquin , P. and Arul , J. 2003 . Fragmentation of Chitosan by Microfluidization Process . Innovative Food Sci. Emerging Tech. , 4 ( 4 ) : 403 – 413 .
- Magee , E.L. and Olsen , N.F. 1981 . Microencapsulation of Cheese Ripening Systems: Formation of Microcapsules . J. Dairy Sci. , 64 : 600 – 610 .
- Al-Meshal , M.A. , Khidr , S.H. , Bayomi , M.A. and Al-Angary , AA. 1998 . Oral Administration of Liposomes Containing Cyclosporine: A Pharmacokinetic Study . Int. J. Pharm. , 168 ( 2 ) : 163 – 168 .
- Degim , I.T. , Gumusel , B. , Degim , Z. , Ozcelikay , T. , Tay , A. and Guner , S. 2006 . Oral Administration of Liposomal Insulin . J. Nanosci. Nanotechnol. , 6 ( 9–10 ) : 2945 – 2949 .
- Zhang , N. , Ping , Q.N. , Huang , G.H. and Xu , W.F. 2005 . Investigation of Lectin-modified Insulin Liposomes as Carriers for Oral Administration . Int. J. Pharm. , 294 ( 1–2 ) : 247 – 259 .
- Takahashi , M. , Inafuku , K. , Miyagi , T. , Oku , H. , Wada , K. , Imura , T. and Kitamoto , D. 2007 . Efficient Preparation of Liposomes Encapsulating Food Materials Using Lecithins by a Mechanochemical Method . J. Oleo Sci. , 56 ( 1 ) : 35 – 42 .
- Asp , N.G. , Bjorck , I. and Nyman , M. 1993 . Physiological Effects of Cereal Dietary Fibre . Carbohydr. Polym. , 21 : 183 – 187 .
- Malkki , Y. and Virtanen , E. 2001 . Gastrointestinal Effects of Oat Bran and Oat Gum: A Review . Lebensm.-Wiss. Technol. , 34 ( 6 ) : 337 – 347 .
- Paradkar , A. , Ambike , AA. , Jadhav , B.K. and Mahadik , K.R. 2004 . Characterization of Curcumin-PVP Solid Dispersion Obtained by Spray Drying . Int. J. Pharm. , 271 : 281 – 286 .
- Um , J.Y. , Chung , H. , Kim , K.S. , Kwon , I.C. and Jeong , S.Y. 2003 . In Vitro Cellular Interaction and Absorption of Dispersed Cubic Particles . Int. J. Pharm. , 253 : 71 – 80 .
- Muzykantov , V.R. 2001 . Targeting of Superoxide Dismutase and Catalase to Vascular Endothelium . J. Control. Rel. , 71 : 1 – 21 .
- Stone , W.L. and Smith , M. 2004 . Therapeutic Uses of Antioxidant Liposomes . Mol. Biotechnol. , 27 ( 3 ) : 217 – 230 .
- Kong , B.H. and Xiong , Y.L.L. 2006 . Antioxidant Activity of Zein Hydrolysates in a Liposome System and the Possible Mode of Action . J. Agricul. Food Chem. , 54 ( 16 ) : 6059 – 6068 .
- Wasserman , V. , Kizelsztein , P. , Garbuzenko , O. , Kohen , R. , Ovadia , H. , Tabakman , R. and Barenholz , Y. 2007 . The Antioxidant Tempamine: In Vitro Antitumor and Neuroprotective Effects and Optimization of Liposomal Encapsulation and Release . Langmuir , 23 ( 4 ) : 1937 – 1947 .
- Pothakamury , U.R. and Barbosa-Canovas , G.V. 2000 . Fundamental Aspects of Controlled Release in Foods . Trends Food Sci. Technol. , 6 ( 12 ) : 397 – 406 .
- Hunter , J.E. 2006 . Dietary Trans Fatty Acids: Review of Recent Human Studies and Food Industry Responses . Lipids , 41 ( 11 ) : 967 – 992 .
- Kolanowski , W. , Jaworska , D. and Weissbrodt , J. 2007 . Importance of Instrumental and Sensory Analysis in the Assessment of Oxidative Deterioration of Omega-3 Long-chain Polyunsaturated Fatty Acid-rich Foods . J. Sci. Food Agricul. , 87 ( 2 ) : 181 – 191 .
- Ross , C.F. and Smith , D.M. 2006 . Use of Volatiles as Indicators of Lipid Oxidation in Muscle Foods . Comp. Rev. Food Sci. Food Safety , 5 ( 1 ) : 18 – 25 .
- Stohs , S.J. 1995 . The Role of Free Radicals in Toxicity and Disease . J. Basic Clin. Physiol. Pharmacol. , 6 : 205 – 228 .
- Djordjevic , V.B. 2004 . Free Radicals in Cell Biology . Int. Rev. Cytol. , 237 : 57 – 89 .
- Willcox , J.K. , Ash , S.L. and Catignani , G.L. 2004 . Antioxidants and Prevention of Chronic Disease . Crit. Rev. Food Sci. Nutr. , 44 : 275 – 295 .
- Berger , M.M. 2005 . Can Oxidative Damage be Treated Nutritionally? . Clin. Nutr. , 24 : 172 – 183 .
- Cansell , M. , Nacka , F. and Combe , N. 2003 . Marine Lipid-based Liposomes Increase In Vivo FA Bioavailability . Lipids , 38 ( 5 ) : 551 – 559 .
- Fahr , A. , Van Hoogevest , P. , Kuntsche , J. and Leigh , M.L.S. 2006 . Lipophilic Drug Transfer Between Liposomal and Biological Membranes: What Does it Mean for Parenteral and Oral Drug Delivery? . J. Liposome Res. , 16 ( 3 ) : 281 – 301 .
- Ratnam , D.V. , Ankola , D.D. , Bhardwaj , V. and Sahana-Kumar , M.N.V.R. 2006 . Role of Antioxidants in Prophylaxis and Therapy: A Pharmaceutical Perspective . J. Control. Rel. , 113 ( 3 ) : 189 – 207 .