ABSTRACT
The objective of the present study was to investigate the structural and rheological properties of octenyl succinic anhydrate (OSA) modified potato starch. Potato starch was modified with different concentrations of OSA (0, 1, 3, and 5%, v/v). X-ray results suggested that OSA modification did not disrupt the crystallinity of the native starch, and esterification occurs primarily in the amorphous regions. The use of 1H-NMR spectra confirmed the presence of methyl protons in substituted OSA groups, which interacted with the starch molecules. In steady shear rheological analysis, OSA modified starch pastes showed a pronounced shear thinning behavior (n = 0.47–0.54). The consistency index (K) and yield stress (σoc) values of OSA modified starch pastes were significantly lower than those of the native starch. Dynamic shear rheological tests indicated that OSA modified starch pastes had weak gel-like behavior with storage moduli (G’) higher than loss moduli (G’’). OSA potato starch pastes were more viscous as compared to the native potato starch paste, as evidenced by their higher tan δ values.
Introduction
Potato starch is used by the food industry as an ingredient in processed potato products, including soups, noodles, sauces, snacks, and breads. As the use of native potato starch in the food system is comparatively limited, chemical modifications are necessary to expand the range of potato starch applications in the food industry.[Citation1] Chemical modification involves the introduction of functional groups into the starch molecule, resulting in markedly altered structural, rheological, and physicochemical properties.[Citation2] The structure and rheology properties achieved when modifying starch by chemical substitution depend, interalia on the starch source, type of substituent, extent of substitution, and distribution of the substitutions in the starch molecule. The modification is generally achieved through derivatization such as esterification, etherification, cross-linking, and grafting of starch decomposition.[Citation1,Citation3,Citation4]
Starch alkenylsuccinates, such as dodecenyl succinic anhydride, octadecenyl succinic anhydride, and octenyl succinic anhydride (OSA), are usually prepared commercially by base catalyzed reactions of alkenyl succinic anhydride with granular starch in aqueous suspension.[Citation5] Among the alkenyl succinic anhydrides, OSA has been approved by the US FDA for use in foods at a level of 3.0% [degree of substitution (DS): 0.02]. Shogren et al.[Citation2] reported the preparative conditions, distribution of OSA groups, and properties of OSA-modified waxy maize starch. OSA modification imparted hydrophobic characteristics to starch and is known to weaken the internal bonding that holds the granules together.[Citation6] OSA modified starch has been widely studied for its superior emulsifying property, which is mainly due to the simultaneous presence of hydrophobic alkenyl and hydrophilic carboxyl moieties in the relatively hydrophilic structure of starch molecule.[Citation7,Citation8]
In the literature, OSA modified starches with different DS have been prepared from different starch sources such as corn starch,[Citation9] wheat starch,[Citation10] cassava starch,[Citation11] waxy corn, and amaranth starches,[Citation12] and their physicochemical properties have been intensively studied as a function of DS. On the contrary, relatively few studies have focused on rheological properties in terms of the structural properties except for the study by Ortega-Ojeda et al.,[Citation13] who examined the effect of DS on the rheological properties of OSA modified potato starch under steady shear conditions. Most rheological studies were limited to pasting properties mainly using the Rapid Visco-Analyzer,[Citation14] Brookfield viscometer,[Citation15] Micro Visco-Amylo Graph,[Citation16] or the Brabender Amylograph.[Citation17] No attempt has been made to date to study the steady and dynamic rheological properties of OSA modified potato starch with various DS.
Information on changes in the structural and rheological properties of starches caused by chemical modification is helpful in understanding their physicochemical and functional properties. Moreover, it is necessary to investigate the physical properties of modified starches from other botanical sources. Therefore, in the present study, OSA modified potato starches with different DS (0.0012–0.005) were prepared from Korean potato starch, and their structural, steady, and dynamic rheological properties were investigated as a function of DS.
Materials and methods
Materials
Potato (Solanum tuberosum L.) tubers of variety “Go-un” cured for 15 days (the RH 80% at 20°C) and stored in dark room for 170 days at 4°C were provided by the Highland Agriculture Research Center, National institute of Crop Science (NICS) in Korea. This material was planted on the May 16 2014 and emerged September 5 2014. High purity OSA was purchased from Sigma-Aldrich Chemical Co. (St. Louis, MO, USA). The other chemicals used in the study were of analytical grade.
Preparation of potato starch
Potato samples were washed, peeled, sliced into 2–3 cm, and lyophilized in a freeze dryer (FDS8508, Ilshin Bio Base, Gyeonggi, Korea). To produce potato powder, the dried sample was ground in a blender (7011, Waring Laboratory Science, Stamford, CT, USA), passed through a 150 μm sieve and stored at -20°C in sealed plastic containers until further use. Potato powder (250 g) was cleaned, steeped in bisulphite solution (1 L) at 58°C for 2 h, washed with distilled water three times, and finally centrifuged at 3,500 rpm for 10 min (VS-5000N, Vision scientific Co. Ltd., Seoul, Korea). After centrifugation, the protein layer was separated. The operations of centrifugation and protein layer separation were repeated until white starch was obtained. The native potato starch was dried at 40°C for 48 h and stored at -20°C in sealed plastic containers until further use.
Chemical composition of native potato starch
The chemical composition of native potato starch including moisture, crude ash, crude protein, and crude lipid contents was determined according to AACCI[Citation18] Approved Methods 44–15A, 08–01, 46–13, and 920.39, respectively. Total starch content in native potato starch was determined based on AOAC[Citation19] Approved Method 996.11. The amylose content of potato starch was analyzed by using the iodine colorimetric method with slight modifications.[Citation15] The starch sample (20 mg, dry weight based) was mixed with 10 mL of 0.5 mol/L KOH by a magnetic stirrer. The starch suspension was then transferred to a 100 mL volumetric flask and diluted to the final volume of 100 mL by adding distilled water. About 10 mL of the diluted solution was transferred to a 50 mL volumetric flask and mixed with 5 mL of 0.1 mol/L HCl and 0.5 mL of iodine reagent (0.2% I2 + 2.0% KI). The solution was diluted to 50 mL with distilled water. The absorbance of color-developed starch solution was measured at 620 nm on a spectrophotometer (Multiskan GO, Thermo Scientific, Massachusetts, USA) after 5 min. The determination of the amylose content was calculated according to a standard curve developed using different ratios of amylose and amylopectin blends.
Production of OSA modified potato starch
OSA modified potato starch samples were obtained using the modified method described by Bhosale and Singhal.[Citation12] Native potato starch (125 g) was suspended in 475 mL distilled water at room temperature, and the slurry was mixed homogeneously with a stirrer. The pH of the slurry was adjusted to pH 9 with 3% (w/v) NaOH solutions. To this mixture, OSA at 1, 3, and 5% based on the weight of the starch was added dropwise for 2 h with further addition after 30 min. During addition of OSA, pH was maintained at 9. The reaction was allowed to continue for 24 h. At the end of reaction, pH of the reaction mixture was adjusted to 6.5 using 3% (v/v) HCl. The resulting OSA modified potato starch was washed with distilled water and centrifuged at 3,000 rpm for 10 min. OSA modified starches were dried in an oven at 45°C for 48 h, sieved through a 150 μm sieve, and stored at -20°C in sealed plastic containers until further use.
Degree of substitution and amylose leaching
The degree of substitution (DS) is the average number of hydroxyl groups substituted per glucose unit. The DS value for OSA starch was determined by the modified method described by Curá et al.[Citation20] OSA starch sample (5 g) was accurately weighed and dispersed by stirring for 30 min in 25 mL of 2.5 mol HCl isopropyl alcohol solution. About 100 mL of 90% (v/v) aqueous isopropyl alcohol solution was added, and the slurry was stirred for an additional 10 min. The suspension was filtered through a glass filter, and the residue was washed with 90% isopropyl alcohol solution until no Cl could be detected any longer (using 0.1 mol AgNO3 solution). The starch was re-dispersed in 300 mL of distilled water, and then, the dispersion was cooked in a boiling water bath for 20 min. The starch solution was titrated with 0.1 mol standard NaOH solution, using phenolphthalein as an indicator. A blank was simultaneously titrated with native starch as a sample. The DS was calculated by the following equation:
where A is the titration volume of NaOH solution (mL), mol is the molarity of NaOH solution, and W is the dry weight (g) of the OSA starch.
The amylose leaching was determined based on the blue value method introduced by Cousidine.[Citation21] Native and OSA modified potato starch (1 g) in centrifuge tubes were suspended in 15 mL of distilled water and shaken in water bath at 80°C for 30 min. The mixture was centrifuged at 3,000 rpm for 10 min. About 1 mL of supernatant was pipette into 100 mL volumetric flask. Then, 1 mL of 1 mol acetic acid and 2 mL of 2% (I2 2% (w/v), KI 0.5% (w/v) iodine solution were added to the supernatant, and the volume was adjusted to 100 mL by distilled water. The solution was placed into dark room for 30 min. The blue color was measured using Elisa reader (Powerwave XS; Bio-TEK Instrument, Winooski, VT, USA) at 620 nm. Potato amylose (St. Louis, MO, USA) was used as standard. The amylose leaching was calculated from amylose standard curve.
Structural properties
Morphological analysis
The morphological features of native and OSA modified potato starch granules were examined using a field emission scanning electron microscope (FE-SEM, SUPRA 55, Carl Zeiss, Oberkochen, Germany). Before testing, the samples were fixed onto a specimen holder with the help of double sided carbon tape and then sputtered with platinum powder using an ion sputter coater. Each sample was observed at an accelerating potential of 10 kV under a high vacuum condition, and image magnifications of 1,000 and 3,000 were used.
X-ray diffraction
The X-ray patterns of native and OSA modified starch were obtained using an X-ray diffractometer (D8 Advance Bruker, Karlsruhe, Germany) operated at 80 mA and 40 kV. The scanning region for the diffraction angle (2θ) was from 5° to 50°, at 0.02° step size, with a count time of 2 sec.
Nuclear magnetic resonance (NMR) spectroscopy
OSA starch samples or native potato starch (2 g) and α-amylase (Megazyme Int., Wicklow, Ireland) (10 µL) were mixed in 30 mL of distilled water. In some cases, sodium acetate (0.3 g) was added. The slurry was heated in a water bath at 85°C with shaking for 2 h to hydrolyze the starch and then placed in a boiling water bath for 30 min to denature the enzyme. After cooling to room temperature, the hydrolyzed starch was freeze dried. The freeze-dried starch (0.2 g) was dissolved in 1 mL of D2O (99.9 atom % D) and freeze dried to produce D2O-exchanged starch. The procedure was repeated twice. The D2O-exchanged starch (0.01 g) was dissolved in D2O (0.50 mL) for NMR experiments. 1D 1H and broadband proton decoupled 13C NMR spectra were recorded on a 300 NMR spectrometer (JNM-AL300, Tokyo, Japan) at 25°C. The NMR spectrometer was equipped with a 3-mm diameter, triple-resonance, inverse detection, and pulse-field-gradient probe operating at 499.85 MHz for 1H. The 1H spectra were collected in 128 individual scans with a sweep width of 16 ppm and a delay time of 1 sec.
Rheological properties
Sample preparation
OSA starch sample dispersions (3%, w/w) were prepared by mixing starch with distilled water in an Erlenmeyer flask with screw cap. The starch dispersion was appropriately stirred for 30 min at room temperature and then heated in a 90°C water bath for 30 min with constant mild agitation using a magnetic stirrer in order to avoid sedimentation and agglomeration. At the end of the heating period, the hot paste was immediately transferred to the rheometer plate for the measurement of rheological properties.
Steady shear rheological properties
Steady shear rheological properties were measured at 25°C with a MCR 102 rheometer (Anton Paar GmbH, Graz, Austria) using a parallel plate system (50 mm diameter) at a gap of 0.5 mm. Each sample was transferred to the rheometer plate at the desired temperature, and excess material was wiped off with a spatula. Steady shear (shear stress and shear rate) data were obtained over a shear rate range of 0.01–1,000 s−1. To describe the steady shear rheological properties of the samples, the data were fitted to the well-known power law (Eq. 1) and Casson (Eq. 2) models:
where σ is the shear stress (Pa), γ̇ is the shear rate (s−1), K is the consistency index (Pa∙sn), n is the flow behavior index (dimensionless), and (Kc)2 is the Casson plastic viscosity (ηc). Casson yield stress (σoc) according to the Casson model (Eq. 2) was determined as the square of the intercept (Koc) obtained from the linear regression of the square roots of the shear rate-shear stress data. Using the magnitudes of K and n, the apparent viscosity (ηa,100) at 100 s−1 was calculated.
Dynamic shear rheological properties
The dynamic shear rheological properties [storage modulus (G′), loss modulus (G″), complex viscosity (η*), and tan δ (G″/G′)] of native potato starch and OSA modified potato starch were investigated by modifying the procedure described by Lee and Yoo[Citation22] and were determined on a strain controlled Physica MCR 301 Rheometer (Anton Paar, Graz, Austria). Prior to the dynamic shear rheological measurements, a strain sweep test at a constant frequency of 0.63 rad s−1 determined the linear viscoelastic region. The dynamic measurements were performed at a strain value of 0.02 (2%) (within the linear viscoelastic region). Frequency sweep tests of all the samples were performed using plate/plate geometry (50 mm diameter, 0.5 mm gap) at 4°C and frequency (ω) from 0.63 to 63.8 rad s−1
Statistical analysis
All statistical analyses were performed using SAS version 9.1 (SAS Institute Inc., Cary, NC, USA). Analysis of variance (ANOVA) was performed using the general linear models (GLM) procedure to determine significant differences among the samples. Means were compared using Fisher’s least significant difference (LSD) procedure. Significance was defined at the 5% level.
Results and discussion
Chemical composition of potato starch
The chemical composition of native potato starch is shown in . The extracted starch contained 8.34% moisture content, 0.61% crude protein content, 0.20% crude fat content, 0.27% crude ash content, and 90.57% total starch content. The isolated native potato starch contained 35.21% of amylose, and the amylopectin content of the native potato starch was observed to be 55.36%.
Table 1. Chemical composition of potato starch.
Degree of substitution
Potato starches modified with different levels of OSA were subjected to DS analysis. DS values of OSA treated potato starches were significantly increased from 0.0012 to 0.0055 with increasing OSA levels from 1 to 5%, respectively (). According to the FDA regulations, the addition of OSA for starch modification must be limited to 3% [DS < 0.02] based on the weight of the starch.
Table 2. Degree of substitution (DS) and amylose leaching for native and octenyl succinic anhydride (OSA) modified potato starch.
Amylose leaching
The extent of amylose leaching for native and OSA modified potato starches at 80°C is presented in . The amylose leaching values of native and OSA modified potato starch with different DS (0.0012–0.0055) was in the range of 47.09–87.32%. OSA starches showed significantly lower values of amylose leaching than native potato starch, and amylose leaching was significantly decreased with an increase in DS. Amylose leaching was influenced by the extent of interaction between amylose–amylose, amylose–amylopectin chains, and the total amylose content.[Citation8] The incorporation of OSA groups in the starch molecules can decrease the number of hydroxyl groups that can potentially bind to water molecules.[Citation23] Therefore, in the present study, OSA groups could inhibit the interaction of amylose chains with water molecules, consequently resulting in the decrease in the amylose leaching.
Structural properties
Field emission scanning electron microscopy
The FE-SEM micrographs of native and OSA modified potato starches at 1,000 and 3,000 X magnifications are presented in . The FE-SEM survey indicated that the OSA treatment brought some changes in the structure of starch granules compared with those of native potato starch. Most of the native potato starch granules were round and oval with smooth surfaces. However, they were remarkably different from OSA modified potato starch granules. For instance, OSA starch granules displayed slightly rough surfaces and their edges lost some definition. The sides of these granules were porous, and several cavities were observed. The particle sizes (40–45 μm) of OSA starches were slightly larger than those (20–30 μm) of native starch, probably due to the extension of chain length during the substitution of OSA with native starch. However, there were no considerable differences among OSA modified starch samples.
Figure 1. Field emission scanning electron microscopy of native and octenyl succinic anhydride modified potato starches with different degree of substitution of native (A, B), 0.0012 (C, D), 0.0031 (E, F), and 0.0055 (G, H). Micrographs of each starch sample were taken at 1,000 and 3,000 X magnifications.
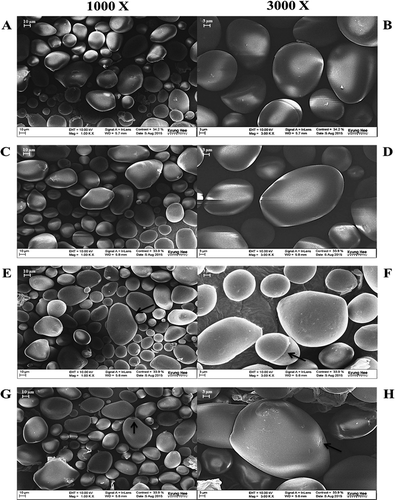
X-ray diffraction
Native and OSA potato starches were measured with powder X-ray diffraction to elucidate the effect of OSA modification on the crystal structure. X-ray powder diffraction scans of native and OSA potato starches are shown in . X-ray results indicated that there were no remarkable differences between native and OSA potato starches, and both starches showed typical B-type diffraction patterns with strong reflection. The earlier studies showed that there is a typical B-type crystallinity peak (about 17°, 2θ) and a few small peaks at around 2θ of 5°, 15°, 20°, and 23° in potato starch.[Citation24] The result suggested that OSA esterification did not disrupt the crystallinity of native potato starch and that esterification occurs primarily in the amorphous regions, leaving the crystalline pattern of starches unchanged. This observation was in agreement with the results of Gao et al.,[Citation25] who mentioned that the esterification of corn starch took place primarily in the amorphous regions. Therefore, the present study results indicated that the esterification did not change the crystalline pattern of potato starch, even though the granules of OSA potato starches have been damaged to some extent by the modification processes.
1H NMR analysis
The use of 1H-NMR provides evidence of OSA modified potato starch molecules. The 1H-NMR spectra of native and OSA modified potato starches are illustrated in . The peaks observed in the 1H-NMR spectra for all starches arising from the glucose in starch were assigned according to the literature.[Citation26–Citation29] The anomeric signals of α-1, 4 and α-1, 6 for all starch samples were clearly visible at 5.20 ppm and 5.03 ppm, respectively. Nilsson et al.[Citation26] and Shih et al.[Citation27] confirmed the presence of the anomeric protons of potato starch at 5.11–5.26 ppm for H-1 (α-1, 4) and at 4.94–5.04 ppm for H-1 (α-1, 6). The chemical signals of all starches were present among 3.29, 3.63, 3.09–3.47, and 3.54–3.57 ppm representing hydrogen at positions 2, 3, 4, and 5, respectively, for the internal hydrogen of the glucose units.[Citation26,Citation29]
Figure 3. 1H nuclear magnetic resonance spectroscopy of native and octenyl succinic anhydride (OSA) modified potato starches with different degree of substitution of native (A), 0.0012 (B), 0.0031 (C), and 0.0055 (D).
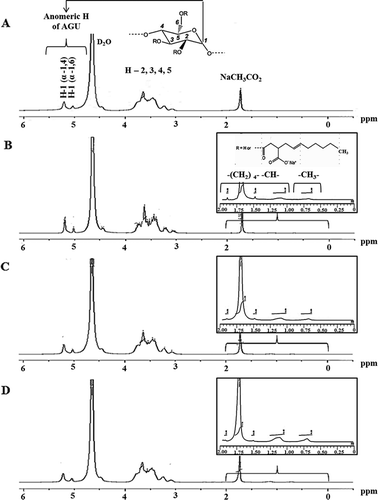
Interestingly, compared with native potato starch, OSA modified potato starches had new signals at 0.70–2.00 ppm, which were not observed in the native starch, subsequently explaining the existence of the OSA groups. According to Shih et al.,[Citation27] and Simsek et al.,[Citation29] the broad peaks around 0.70–0.80 ppm were indicative of the terminal methyl protons in substituted octenyl succinate groups, which are hydrophobic. Moreover, Bai et al.[Citation28] reported that the new peaks around 0.90 ppm were assigned to some methyl protons in substituted OSA groups which interact with the starch molecules. Therefore, in the present study, the peak at 0.70–1.00 ppm can be designated to free and bound forms of terminal methyl protons of OSA. The peaks observed around 1.05–2.00 ppm in OSA modified potato starches were assigned to the protons of the methylene groups of OSA.[Citation4,Citation29]
Rheological analysis
Steady shear rheological analysis
Plots of shear stress (σ) versus shear rate () for native and OSA modified potato starch pastes at different DS (0.0012–0.0055) showed the non-Newtonian (pseudoplastic) nature with yield stress (). The flow behavior index (n) of native and all OSA modified starches, which indicate the extent of shear-thinning behavior as it deviates from 1 (n=1 for Newtonian flow), was in the range of 0.46–0.65 (). The observed high shear-thinning behavior of all starch samples could be associated with greater destruction of the intra- and intermolecular bonding systems in the starch network due to shearing at higher shear rates.[Citation30] The n values of OSA modified potato starches were significantly higher than that of the native starch. The higher shear thinning behavior of the native starch may be due to the lower level of disruption of the intramolecular and intermolecular bonding system in the native starch network as compared to OSA modified starch.[Citation31]
Table 3. Effect of degree of substitution (DS) on apparent viscosity (ƞa,100), consistency index (K), flow behavior index (n), and Casson yield stress (σoc) values of native and octenyl succinic anhydride modified potato starch pastes at 25°C.
Figure 4. Plot of shear stress-shear rate for native and octenyl succinic anhydride modified potato starches with different degree of substitution of native (■), 0.0012 (∆), 0.0031 (○), and 0.0055 (+).
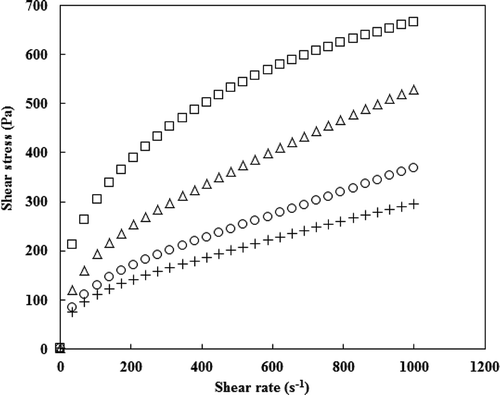
The steady shear rheological data of OSA modified potato starch samples showed that the consistency index (K), Casson yield stress (σoc), and apparent viscosity (ηa,100) of OSA modified potato starch at 30 s−1 were significantly lower than those of the native potato starch and decreased significantly with an increase in DS (). This behavior may be attributed to the disintegration of potato starch granules due to the disruption of hydrogen bonds caused by the incorporation of OSA groups in the starch molecules.[Citation2] Octenylsuccinylation imparts hydrophobic characteristics to starch and is known to weaken the internal bonds that hold the granules together.[Citation6] Another possible reason for the reduction of K, σoc, and ηa,100 values after OSA modification is reduced amylose leaching during gelatinization (). Based on the results on amylose leaching and steady shear rheological properties, the steady shear properties of OSA modified potato starch may be affected by the amount of amylose leached from starch granules during gelatinization.
Table 4. Effect of degree of substitution (DS) on storage modulus (G’), loss modulus (G”), complex viscosity (η*) and tan δ at 6.28 rad s−1 of native and octenyl succinic anhydride modified potato starch pastes at 25°C.
Dynamic shear rheological analysis
The changes in storage modulus (G′), loss modulus (G″), and complex viscosity (η*) as a function of the frequency (ω) for native and OSA modified potato starch pastes at different DS values are shown in . For all samples, the magnitudes of G′ and G″ increased with an increase in frequency values (0.63–63.8 rad s−1), while those of η* decreased, showing a high frequency dependency. The strong gels were formed at all samples where the storage modulus G’ was much higher than the loss modulus G’’ over the entire accessible frequency range, and the two moduli exhibited dependence of frequency.
Figure 5. Plots of log G′, log G″, and log tan δ versus log ω (frequency, rad s−1) for native and octenyl succinic anhydride modified potato starch pastes with different degree of substitution: native (■), 0.0012 (∆), 0.0031 (○), and 0.0055 (+) at 25°C.
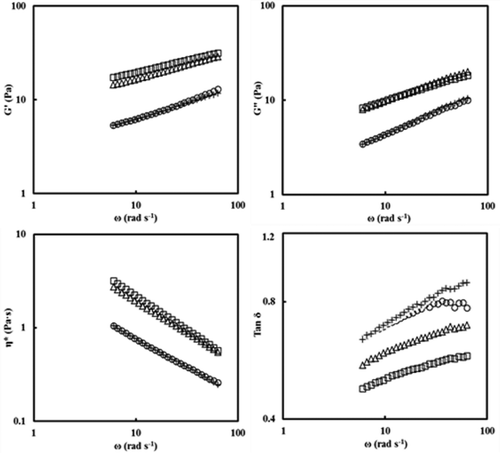
lists the G′, G″, and η* values at 6.28 rad s−1 for native and OSA modified potato starch pastes at 25°C. The G′, G″, and η* values of OSA starch pastes were significantly lower than those of the native starch and were significantly decreased with an increase in DS. Such lower dynamic moduli for OSA potato starch may be attributed to a weaker granular integrity caused by greater water uptake in the swollen granules of the potato starch paste in the presence of OSA groups.[Citation30] Kim et al.[Citation32] also indicated that the decrease of G’, G”, and η* values of sweet potato starch crosslinked with POCl3 may be attributed to the decrease in associative forces within the potato starch granules due to POCl3 esterification.
Table 5. Effect of degree of substitution (DS) on slopes (n′, n″) and intercepts (K′, K″) of log (G′, G″) values versus log ω data of native and octenyl succinic anhydride modified potato starch pastes at 25°C.
The tan δ (ratio of G″/G′) values of all samples were within the range of 0.49–0.66 (), indicating that all samples were more elastic than viscous, which is in good agreement with the results found in waxy corn starch[Citation33] and corn starch.[Citation34] The OSA potato starch pastes were more viscous as compared to the native potato starch paste, as evidenced by their higher tan δ values. These observations suggested that the viscous properties of potato starch pastes can be increased by OSA esterification treatment and the extent of increase depends on DS.
The dynamic rheological data of log (G′, G″) versus log ω were also subjected to linear regression, and the slopes (n′ and n″), intercepts (K′ and K″), and R2 were obtained () using the following equations (Eqs. 3, 4).
The slope values of G′ and G″ for the OSA modified potato starch pastes were positive with high R2 (0.99–1.00) at 25°C. The slopes of G′ (0.26–0.37) and G″ (0.34–0.49) for the OSA modified potato starch pastes were significantly higher than those of the native starch. The slopes of the G′ values for OSA modified potato starch pastes were significantly lower than those of the G″ values, indicating that OSA starch pastes are more elastic than viscous and that G″ is more dependent on frequency than G′, which is typical of weak gels. The K′ values of all OSA starch pastes were always much greater than the K″ values. The magnitudes of K′ and K″ also decreased with the increase in DS, explaining that as the DS increased, the rate of chain rearrangement decreased.[Citation32,Citation34] According to steady and dynamic shear rheological analysis, the values of K, ηa,100, σoc, G’, and G” for native potato starch were significantly reduced after OSA modification because OSA groups could inhibit amylose chain interactions and reduce the formation of junction zones by esterification treatment, resulting in the formation of a weaker gel.
Conclusions
The present study investigated the structural and rheological properties of OSA modified potato starch. FE-SEM results showed that OSA groups brought some changes in the structure of native starch granules and X-ray diffraction spectra indicated that OSA modification caused no change in the crystalline pattern of potato starch. Based on 1H NMR analysis, partial substitutions of OSA groups to the starch granules were observed. It was found that OSA modification can significantly reduce the values of K, ηa,100, σoc, G’, and G” of native potato starch. In conclusion, it is apparent in the present study that OSA modification can considerably affect the structural and rheological properties of potato starch.
Funding
This work was carried out with the support of “Cooperative Research Program for Agriculture Science & Technology Development (Project No. PJ011239032016)” Rural Development Administration, Republic of Korea.
Additional information
Funding
References
- Hui, R.; Qi-He, C.; Ming-liang, F.; Qiong, X.; Guo-qing, H. Preparation and Properties of Octenyl Succinic Anhydride Modified Potato Starch. Food Chemistry 2009, 114, 81–86.
- Shogren, R.L.; Viswanathan, A.; Felker, F.; Gross, R.A. Distribution of Octenyl Succinate Distribution of Octenyl Succinate Groups in Octenyl Succinic Anhydride Modified Waxy Maize Starch. Starch/Stärke 2000, 52, 196–204.
- Chi, H.; Xu, K.; Wua, X.; Chen, Q.; Xue, D.; Song, C.; Zhang, W.; Wang, P. Effect of Acetylation on the Properties of Corn Starch. Food Chemistry 2008, 106, 923–928.
- Bai, Y.; Shi, Y.C. Structure and Preparation of Octenyl Succinic Esters of Granular Starch, Microporous Starch and Soluble Maltodextrin. Carbohydrate Polymers 2011, 83, 520–527.
- Wurzburg, O.B. Modified starches-properties and uses. In Modified Starch: Properties and Uses; Trubiano, P.C. Ed.; CRC Press Inc: Boca Raton, FL, 1986, 131–147.
- Bhosale, R.; Singhal, R.; Effect of Octenylsuccinylation on Physicochemical and Functional Properties of Waxy Maize and Amaranth Starches. Carbohydrate Polymers 2007, 68, 447–456.
- Caldwell, C.G.; Wurzburg, O.B. Polysaccharide Derivatives of Substituted Dicarboxylic Acids. U.S. Patent Office, Pat. 1953; No. 2,661,349.
- Sweedman, M.C.; Tizzotti, M.J.; Schafer, C.; Gilbert, R.G. Structure and Physicochemical Properties of Octenyl Succinic Anhydride Modified Starches: A Review. Carbohydrate Polymers 2013, 92, 905–920.
- Kweon, D.K.; Choi, J.K.; Kim, E.K.; Lim, S.T. Adsorption of Divalent Metal Ions by Succinylated and Oxidized Corn Starches. Carbohydrate Polymers 2001, 46, 171–177.
- Woo, K.; Seib, P.A. Cross-linking of Wheat Starch and Hydroxypropylated Wheat Starch in Alkaline Slurry with Sodium Trimetaphosphate Carbohydrate Polymers 1997, 33, 263–271.
- Athira, G.K.; Jyothi, A.N. Octenyl Succinate Cassava Starch as an Excipient for Controlled Release of Theophylline: Microwave-assisted Synthesis, Characterization and in-vitro Drug Release Studies. International Journal of Pharmaceutical Sciences and Research 2015, 6, 200–211.
- Bhosale, R.; Singhal, R. Process Optimization for the Synthesis of Octenyl Succinyl Derivative of Waxy Corn and Amaranth Starches. Carbohydrate Polymers 2006, 66, 521–527.
- Ortega-Ojeda, F.E.; Larsson, H.; Eliasson, A.C. Gel Formation in Mixtures of Hydrophobically Modified Potato and High Amylopectin Potato Starch. Carbohydrate Polymers 2005, 59, 313–327.
- Wang, J.; Su, L.; Wang, S. Physicochemical Properties of Octenyl Succinic Anhydride‐modified Potato Starch with Different Degrees of Substitution. Journal of the Science of Food and Agriculture 2010, 90(3), 424–429.
- Kaur, L.; Singh, N.; Sodhi, N. S. Some Properties of Potatoes and their Starches II. Morphological, Thermal and Rheological Properties of Starches. Food Chemistry 2002, 79, 183–192.
- Suh, D.S.; Jane, J.L. Comparison of Starch Pasting Properties at Various Cooking Conditions using the Micro Visco-amylo-graph and the Rapid Visco Analyser. Cereal Chemistry Journal 2003, 80, 745–749.
- Bahnassey, Y.A.; Breene, W.M. Rapid Visco‐Analyzer (RVA) Pasting Profiles of Wheat, Corn, Waxy Corn, Tapioca and Amaranth Starches (A. hypochondriacus and A cruentus) in the Presence of Konjac Flour, Gellan, Guar, Xanthan and Locust Bean Gums. Starch/Stärke 1994, 46, 134–141.
- American Association of Cereal Chemists (AACC), Approved Method of the AACC, Methods 44-15A, 08-01, 46-13, and 920.39, AACC International; St. Paul, MN, USA 2000; 10th ed.
- Association of Official Analytical Chemists (AOAC), Official Method of Analysis of AOAC Method 996.11, AOAC International; Gaithersburg, MD, USA 2005; 18th ed.
- Curá, J.A.; Jansson, P.E.; Krisman, C.R. Amylose is not Strictly Linear. Starch/Stärke 1995, 47, 207–209.
- Cousidine, D.M. Foods and Food Production Encyclopedia; John Wiley Inc: New York, 1982.
- Lee, H.L.; Yoo, B. Effect of Hydroxypropylation on Physical and Rheological Properties of Sweet Potato Starch. Korean Journal of Food Science and Technology 2011, 44, 765–770.
- Song, X.Y.; He, G.Q.; Ruan, H.; Chen, Q. Preparation and Properties of Octenyl Succinic Anhydride Modified Early Indica Rice Starch. Starch/Starke 2006, 58, 109–117.
- Xu, J.; Zhou, C.W.; Wang, R.Z.; Yang, L.; Du, S.S.; Wang, F.P.; He, G.Q. Lipase-coupling Esterification of Starch with Octenyl Succinic Anhydride. Carbohydrate Polymers 2012, 87, 2137–2144.
- Gao, Y.; Wang, L.; Yue, X.; Xiong, G.; Wu, W.; Qiao, Y.; Liao, L. Physicochemical Properties of Lipase-catalyzed Laurylation of Corn Starch. Starch/Stärke 2014, 66, 450–456.
- Nilsson, G.S.; Bergquist, K.E.; Nilsson, U.; Gorton, L. Determination of the Degree of Branching in Normal and Amylopectin Type Potato Starch with 1H-NMR Spectroscopy. Starch/Stärke 1996, 48, 352–357.
- Shih, F.F.; Daigle, K.W. Gelatinization and Pasting Properties of Rice Starch Modified with 2-octen-1-ylsuccinic anhydride. Nahrung/Food 2003, 47, 64–67.
- Bai, Y.; Shi, Y.C.; Herrera, A.; Prakash, O. Study of Octenyl Succinic Anhydride-modified Waxy Maize Starch by Nuclear Magnetic Resonance Spectroscopy. Carbohydrate Polymers 2011, 83, 407–413.
- Simsek, S.; Ovando-Martinez, M.; Marefati, A.; Sjӧӧ, M.; Rayner, M. Chemical Composition, Digestibility and Emulsification Properties of Octenyl Succinic Esters of Various Starches. Food Research International 2015, 75, 41–49.
- Thirathumthavorn, D.; Charoenrein, S. Thermal and Pasting Properties of Native and Acid-treated Starches Derivatized by 1-octenyl Succinic Anhydride. Carbohydrate Polymers 2006, 66, 258–265.
- Cho, S.A.; Kim B.Y.; Yoo., B. Flow Behavior of Sweet Potato Starch in Mixed Sugar System. Journal of the Korean Society of Food Science and Nutrition 2008, 13, 249–252.
- Kim, B.Y.; Yoo, B. Effects of Cross‐linking on the Rheological and Thermal Properties of Sweet Potato Starch. Starch/Stärke 2010, 62, 577–583.
- Krstonosic, V.; Doklc, L.; Milanovic, J. Micellar Properties of OSA Starch and Interaction with Xanthan Gum in Aqueous Solution. Food Hydrocolloids 2011, 25, 361–367.
- Park, S.; Chung, M.G.; Yoo, B. Effect of Octenylsuccinylation on Rheological Properties of Corn Starch Pastes. Starch‐Stärke 2004, 56, 399–406.