ABSTRACT
A variety of fructans occurs in plants, having various configuration and chain lengths from 3 up to a few hundred fructose units. The structurally simplest fructan is a linear inulin, where the fructose units are linked by β-2,1 bonds, present mostly in Asteraceae and Cichorioideae (e.g. dahlia). The pharmacological effects of fructans depend mainly on the degree of polymerization (DP). Hence, a controlled DP distribution profile is essential for the expected spectrum of pharmacological activities. The aim of this study was to purify fructans from dahlia (Dahlia x hortensis Guill. cv.‘Ken’s Flame’) tubers with different inulin chain lengths profile. We developed the selective preparative approach, followed by qualitative and quantitative analyses by pulsed amperometry-high performance anion-exchange chromatography. The fructans were obtained by the extraction of dahlia tubers with water, concentrated under vacuum, subsequent precipitation of carbohydrate fraction by ethanol, followed by purification using four different combinations of absorbents such as Dowex® ion-exchange resin, aluminium oxide, calcium carbonate, and activated carbon. The highest content of short-chain inulin (38%) was in the crude fraction and after the separation on Al2O3 and CaCO3. All methods selectively enriched inulin in the middle-length chains. The highest concentration of high-quality long-chain inulin (16%) was obtained by consecutive washing through CaCO3, Al2O3, Dowex, and charcoal. This complex was also the most balanced in terms of the proportion among short-, medium-, and long-chain inulin (33%, 43%, and 16%, respectively).
Introduction
Fructans are the storage polysaccharides in plants, consisting of D-fructose residues; they are an easily formed energy source, which is formed at the initial stage of development of the roots and leaves to restore the normal metabolism after a damage of the above-ground plant parts as a result of frosts, eating herbivores, or cutting.[Citation1,Citation2] Inulins are relatively low molecular weight substances; their degree of polymerization (DP) does not exceed 100, and usually is 30–60. They are widespread in plants that belong to the Asteraceae family. The biosynthesis of fructans is closely associated with the metabolism of sucrose, which serves as a donor and an acceptor of β-D-fructofuranose residues.[Citation3] In the cells of plants, fructans accumulate in vacuoles and serve as a storage material (source of fructose), osmosis regulator, and antifreeze agent. The content of fructans can reach 30% (of the dry weight) in the leaves, and in the specialized storage organs (rhizomes, tubers, and bulbs), they can even exceed 60%.[Citation1,Citation4,Citation5] In recent years, the interest in fruits as prebiotics, immunomodulators, and anti-tumour substances increases constantly.[Citation6–Citation8] Fructans in plants have different chain lengths and configurations (from 3 up to several hundred fructose units). The typical fructan structure is a linear inulin, where the fructose residues are bound to one another by β-2,1 linkages.[Citation9]
The fructans are mainly present in plants, which belong to the Asteraceae family (e.g. Dahlia, Inula helenium, Taraxacum officinale), subfamily Cichoroideae (Cichorium intubus), Campanulaceae (Platycodon grandiflorum, Codonopsis pilosula, Codonopsis lanceolata), and several monocots such as leek, agave, or bananas.[Citation10,Citation11] The content of inulin in chicory and Jerusalem artichoke reaches up to 20%, in elecampane 9–12%, in dandelion root, parsnips, oat root, leeks, and asparagus up to 15%, in onion bulb 2–6%, in banana 0.3–0.7%, and in wheat flour and rice flour 1–4%.[Citation7,Citation12–Citation15]
As the inulin-containing raw material, the Jerusalem artichoke tubers are also used, but the cost of pure inulin from this source is relatively high (from 34,000 $ per ton).[Citation16] This is explained by an expensive purification process of inulin fructans with a lower molecular weight. Such molecules may form a caramel-like substance, and thereby they create a number of problems with the technology of isolation and purification. At the same time, there is a need to inactivate the enzymes present in inulin preparation, by freeze-drying, denaturing by organic solvents, and exposure to high temperatures. The alternative to Jerusalem artichoke tubers is raw materials of dahlia that are used in the United States and in Europe as an alternate to chicory source for the commercial production of inulin.[Citation17,Citation18]
Dahlia, a tuberous perennial plant, is widely cultivated throughout the world as an ornamental flower; it is characterized by a variety of inflorescences forms and colours.[Citation19] The genus Dahlia has more than 35 thousand cultivars, distributed around the world. A homeland of dahlia is South America, where the plant is common in the wild. Dahlias are easy-to-grow plants from the agrotechnical point of view, and the tuber yield may reach 2.5 kg per 1 m2. The content of inulin in tubers of dahlia reaches more than 61%.[Citation17] Since in the tubers of dahlia there are no caramel-producing fructans and no highly active enzymes that promote resinification of polysaccharides, isolation and purification of inulin from this raw material do not require time-consuming and costly techniques.[Citation20] Thus, dahlia tubers are an interesting and promising source of raw material from both points of view – technological and economic.
The pharmacological properties of fructans depend mainly on their DP. Control of the DP is important, because according to recent studies, the pharmacological properties of fructans depend on the DP.[Citation21–Citation24] Thus, there are low molecular weight inulins (average DP of 10 and less) and high molecular weight inulins (average DP of 20 and more).[Citation25,Citation26] The physicochemical properties of low molecular weight and high molecular weight inulins are substantially different. Low molecular weight inulins are slightly sweet and dissolve in cold water, whereas high molecular weight inulins have a neutral taste and are difficult to dissolve on boiling. Low molecular weight inulins are amorphous substances, whereas high molecular weight inulins have a crystalline structure.[Citation27] It is quite natural that they differ in the biological properties, too. The general correlation is as follows: a higher average DP means a higher biological activity of the inulin. European experts call low molecular weight inulins as “oligofructose” and this name is mainly used in various sectors of the food industry (dairy products, ice cream, desserts and bakery products, beverages, meat products, fruit and berry fillings, snack and cereal products, baby food, etc.) .[Citation7,Citation28,Citation29] The main technological feature of inulin is its ability to form with water a creamy white opaque gel. The obtained gel has a neutral taste and texture that is very similar to the texture of fat. Therefore, inulin can simulate the presence of fat in low-fat products, improving their texture and organoleptic properties, approaching the indicators of the quality of the products to normal fat content.[Citation30] As an admixture to starch, fructans can improve their processing and consumption properties such as texture, water absorption, and digestibility.[Citation31]
Furthermore, as used in aerated products (mousses, puddings, ice cream, ketchups) and emulsions (dairy spreads, pastes), inulin increases their stability.[Citation32] Thus, the use of inulin in the food industry is as a replacement of fat-containing substances.[Citation33] The high molecular weight inulins have a much wider range of activities. For example, low molecular weight inulins (DP 2–8) play a role as a starter for selective bifidogenic fermentation, whereas high molecular weight inulins (DP 10–65), which are fermented twice slower than low polymerized inulins, save the metabolic activity of the improved flora in more distal parts of the colon.[Citation34–Citation37] Thus, the fermentation of high molecular weight fructooligosaccharides can provide an inhibition of the protein digestion in favour of a more beneficial carbohydrates utilization, which could contribute to a lower formation of carcinogenic metabolites. Moreover, it was found that inulin with DP >23 has the most significant effect on calcium bioavailability.[Citation38] Therefore, a method for producing inulin to a considerable extent should affect the qualitative composition and quantitative content of substances, having an optimal percentage of chains of varying DP and, consequently, influencing their biological activity.[Citation39]
Based on the above, the search for optimal ways to obtain inulin from alternative raw materials – dahlia tubers cultivated in Ukraine – is a relevant issue. The aim of this study was: 1. to obtain a fructan complex from dahlia tubers (variety Ken’s Flame) and to purify it in different ways; 2. to develop a selective, preparative method with subsequent qualitative and quantitative analyses of polyfructans using high-performance anion exchange chromatography with a pulsed amperometric detector (HPAEC-PAD); and 3. to determine the chromatography profiles, indicating the length of the carbohydrate chains.
Materials and methods
Plant material
Dahlia tubers, collected in October 2013, from a village Tishki (Ukraine, Kharkiv region), were used. The tubers were cut to a particle size of about 1 × 1 cm, dried to air-dry form at ambient temperature, and powdered in a laboratory mill to pass a 1.0 mm sieve.
Solvents and reagents
Ultrapure deionized water was obtained from a Milli-Q Water Purification System (Millipore, Billerica, USA). Sodium acetate, glucose, fructose, and sucrose were obtained from Merck (Darmstadt, Germany), sodium hydroxide and inulin from Alfa Aesar (Karlsruhe, Germany), and lactose was purchased from Sigma. Kestose and nystose were purchased from Wako Chemicals (Neuss, Germany). All other chemicals were from Avantor (Gliwice, Poland).
Sample preparation
The native polyfructan complex – the raw material (500 g) – was extracted for 6 h at 65°C. The extraction was repeated twice. Selecting temperature for the extraction of the tubers can be justified by the fact that long-chain inulin is thermolabile and destroyed by higher temperatures.[Citation36] The extract was filtered and evaporated under vacuum to 1/3 volume. To precipitate the polysaccharides, a five-fold volume of 96% ethanol was added to the solution. The precipitated complex was filtered and dried at ambient temperature. The obtained substance was purified in three different ways.
Purification method no 1 (pur 1): The substance was dispersed in distilled water at 80–85°C (ratio 1: 5). To the suspension sodium sulfite was added (to a concentration of 0.1%), which allows one to get rid of the phenolic compounds. The control was carried out by TLC in a solvent system butanol-acetic acid-water (4:1:2) on pre-coated SG-60 Macherey-Nagel Adamant 20 × 20 glass plates (Carl Roth, Karlsruhe, Germany). Then the solution of fructans was treated by freezing/thawing steps (which allows one to regularize the chain structure in a solution) and concentrated under vacuum to 1/3 volume. The cooled, concentrated solution was finally treated with 96% ethanol at a ratio of concentrate: ethanol of 1:3. The obtained precipitate was filtered, washed with cold distilled water, and then dried.
Purification method no. 2 (pur 2): The native substance was dispersed in distilled water at 80–85°C (ratio 1: 5). The hot water extraction was processed with calcium carbonate at 80–85°C for 60 min and filtered through a pad of belting. The process made it possible to destroy the inulin–pectin complex, to coagulate proteins, to get rid of water-soluble pectins, and partly from organic acids and proteins, without destroying the inulin; the pH of the suspension/solution was maintained close to neutral. Absence of proteins was controlled by TLC (butanol-acetic acid-water (12: 3: 5)) and the absence of pectins was determined by the Ehrlich test. The precipitate was washed with hot water (85°C) to a volume of a stock solution taken. To the filtrate the aluminium oxide was added and the mixture was heated with continuous stirring at 75°C for 30 min and then filtered. This allowed one to remove impurities that adsorbed on aluminium oxide (phenols, polyphenols, their oxidation products) (TLC control). The precipitate was cooled to 45°C and consistently passed through a column of ion-exchange resin Dowex (Dow, USA) with particle size 1 × 8 mm and 50 × 4 mm, which allowed one to get rid of cations and anions of organic and inorganic origins. The eluate was decoloured with activated charcoal (10–15 g carbon per 1 L of liquid) at 70–75°C while stirring for 20–30 min. Then the solution was concentrated under vacuum, cooled, and then treated with 96% ethanol at a ratio of concentrate: ethanol of 1: 3. The obtained precipitate was filtered, washed with cold distilled water, and dried.
Purification method no. 3 (pur 3): The native fructan complex was dissolved in hot (80–85°C), distilled water at a ratio of 1:5. Hot water extraction was processed by calcium carbonate at 80–85°C for 60 min and filtered hot through a pad of belting. To the filtrate, aluminium oxide was added and the mixture was heated at 75°C with continuous stirring for 30 min and then filtered. The supernatant was concentrated under vacuum, cooled, and treated with 96% ethanol at a ratio of concentrate: ethanol of 1: 3. The obtained precipitate was filtered, washed with cold distilled water, and then dried. The complete procedures are shown in .
HPAEC-PAD analysis
Isolated inulin and commercial inulin samples were analysed by high-performance anion-exchange chromatography with a pulsed amperometric detection (HPAEC-PAD) using the Dionex DX 500 system (Dionex, Sunnyvale, USA). This method is the official method of the International Commission for Uniform Methods of Sugar Analysis (ICUMSA) and was approved in 1994. Approval of this method was based on an international collaborative study involving 11 laboratories.[Citation40] The system used in the present investigation was equipped with the electrochemical detector ED40 (Dionex, Sunnyvale, USA) and CarboPac PA-1 (9 x 250 mm) or CarboPac PA-100 (4 x 250 mm) analytical columns (Dionex, Sunnyvale, USA). Samples of polyfructans (50 μL) were analysed using a gradient of sodium acetate in 0.1 M NaOH (see ); the flow rate was 1.5 mL/min or 0.5 mL/min and the total time to complete one analysis was 70 min. The following pulse potentials and durations were used in the detector: E1 = 0.40 V (t1 = 400 ms), E2 = 1.0 V (t2 = 200 ms), E3 = 0.25 V (t3 = 400 ms). Relative response factors for inulin chains were applied according to Timmermans et al.[Citation41] For the calibration curves, appropriate volumes of standard stock solutions of glucose, fructose, sucrose, kestose, and nystose were diluted with Milli-Q water and analysed at five concentrations – 0.2 ug, 0.5 ug, 1.0 ug, 2.0 ug, and 5.0 ug in one injected sample. The calibration plots showed good linearity with the correlation coefficients of 0.98 for all substances. All analyses were performed in triplicate. comprises four elution methods, used for the separation of inulin preparations (sep1, sep2, sep3, sep4).
Table 1. HPAEC-PAD chromatography conditions.
Calculations
The evaluation of the relative contents of monosaccharides, disaccharides, and polysaccharides was performed by a simple normalization using the following formula:
is the sum of the peak areas of mono- and disaccharides (polysaccharides: short-, medium-, and long-chain inulin)
is the sum of the peak areas of all components
Results and discussion
To assess the qualitative and quantitative compositions of inulin preparations obtained by various methods, an HPAEC-PAD chromatography was used. Uncharged oligo- and polysaccharides with little or no branching are generally separated on either a CarboPac PA1 or CarboPac PA100 column, using sodium acetate gradient in 0.1 M or 0.15 M sodium hydroxide. The role of sodium hydroxide is to ionize the saccharide hydroxyl groups to oxyanions so that the saccharide binds to the CarboPac column, a strong anion exchanger. The acetate ion is a stronger eluent than the hydroxide ion and is generally used to elute the saccharides, bound to the column. Amylopectin and fructan separations demonstrate that column, eluent, and sodium acetate gradients are parameters of choice while establishing the HPAEC-PAD methods for the separation of neutral oligo- and polysaccharides ().
shows a comparison of the elution profiles of dahlia inulin sample obtained using pur 3 procedure. Three separation methods were used: sep 1, sep 2, and sep 3, as indicated. For all three methods, the CarboPac PA-100 column (4 x 250 mm) was used. The fourth separation method (sep 4) was used for the separation of inulin standard preparation using the CarboPac PA-1 column and compared with sep 3 method; the elution profile is shown in .
Figure 2. HPAEC-PAD analysis of dahlia inulins, obtained by purification method pur 3; three elution methods were used as indicated: A – sep1; B – sep2; C – sep3 (for details see ).
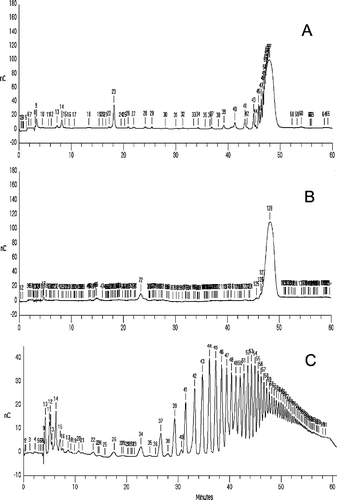
As can be seen in , the HPAEC-PAD chromatography conditions for the separation of polyfructans using method sep3 (, ) gave much better separation and shorter retention times than the other three systems (, B, ); moreover, the spacing between the later-eluting peaks was much more improved. The elution gradient of the method sep3 may improve the reproducibility of integration and this will make it easier to collect individual peaks for further purification or additional analyses. The next stage of our research was to study the inulin profile of native fructan complex and substances separated using the high-performance anion exchange chromatography coupled with pulsed amperometric detection (HPAEC-PAD) method. Among the techniques used for inulin analysis, HPAEC-PAD is the most powerful tool as its resolution power enables one to separate each DP and its isomers (distinction between Fn and GFn-1).[Citation41,Citation42] With the above-described method, glucose, fructose, and sucrose, eluted before inulin and each subsequent peak in a chromatogram, represents a chain with one additional fructose. Identification of individual peaks was carried out by spiking with standards. The HPAEC-PAD method allows one to determine a chain length of more than 70 DP, although molecules having a DP of about 40 have been found in small quantities. In addition, in all the investigated fructan complexes, several small peaks were identified, which, most probably, are fructooligosaccharides ().
Figure 4. HPAEC-PAD chromatograms of dahlia fructans; A. native inulin; B. fructans obtained using sep 1; C. fructans obtained using sep 2 method; and D. fructans obtained using the sep 3 method.
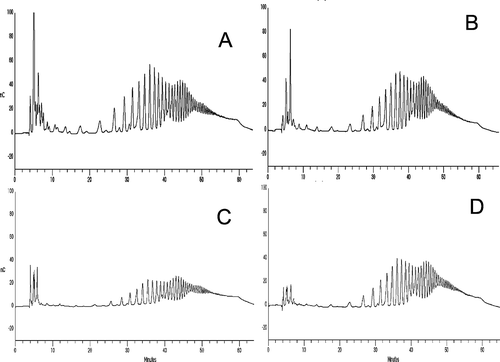
Several methods of obtaining inulin from various materials of Asteraceae plants have been thus far published, including ultrasonic- and microwave-supported techniques.[Citation25,Citation30,Citation43,Citation44] The extraction method used in this study for obtaining inulin from dahlia tubers is simple and does not require specialized technical equipment. The highest content of short-, medium-, and long-chain inulin (39.36%, 35.88%, and 13.00%, respectively) was observed in the fraction obtained by purification method no. 3. This complex was also the most balanced regarding the ratio ().
Table 2. Percentage of monosaccharides, short-, medium-, and long-chain inulin in native fructan and in purified inulin fractions. Results are the mean of three repetitions ± standard deviation.
Conclusion
Using the applied methods, the inulin fractions with varying degrees of purity were obtained. This investigation has shown that the complex produced by purification method no. 3 (sep 3) was the most balanced in terms of content of long- and short-chain inulin and does not require expensive materials. The complex produced by purification method no. 2 (sep 2) was also balanced enough but quite expensive, and hence not industrially feasible. The complex prepared by purification method no. 1 (sep 1) contained a higher amount of middle-chain inulin. The tested methods can be applied to obtain inulin from dahlia tubers, and after proper scale-up and adjustment, they can be further developed for the creation of prebiotic supplements from dahlia tubers.
Additional information
Funding
References
- Dey, P.M.; Dixon, R.A.; Biochemistry of Storage Carbohydrates in Green Plants; Academic Press: London, Orlando, San Diego, New York, Toronto, Montreal, Sydney, Tokyo, 1985.
- Van Laere, A.; Van Den Ende, W. Inulin Metabolism in Dicots: Chicory as a Model System. Plant Cell & Environment 2002, 25, 803–813.
- Franck, A.; De Leenheer, L.I. Inulin. In: Biopolymers, Biology, Chemistry, Biotechnology, Applications, Volume 6, Polysaccharides II: Polysaccharides from Eukaryotes. Steinbüchel, A.; De Baets, S.; Vandamme, E.J. (Eds.); Wiley-VCH Verlag, Weinheim, 2005; pp 439–479.
- Livingston, D.P.; Hincha, D.K.; Heyer, A.G. Fructan and Its Relationship to Abiotic Stress Tolerance in Plants. Cellular and Molecular Life Science 2009, 66, 2007–2023.
- Van Arkel, J.; Sévenier, R.; Hakkert, J.C.; Bouwmeester, H.J.; Koops, A.J.; Van Der Meer, I.M. Tailor-Made Fructan Synthesis in Plants: A Review. Carbohydrate Polymers 2013, 93, 48–56.
- Ritsema, T.; Smeekens, S. Fructans: Beneficial for Plants and Humans. Current Opinion in Plant Biology 2003, 6, 223–230.
- Saeed, F.; Pasha, I.; Arshad, M.U.; Anjum, F.M.; Hussain, S.; Rasheed, R.; Nasir, M.A. Physiological and Nutraceutical Perspectives of Fructan. International Journal of Food Properties 2015, 18, 1895–1904.
- Mundi, M.; Mikal, K.M.; Ahmed, O.H.; Sarbini, S.R. A Review on the Effects of Prebiotics on Cell Toxicity and Integrity. International Journal of Food Properties 2017. (published online). 10.1080/10942912.2017.1326937
- Kocsis, L.; Liebhard, P.; Praznik, W. Effect of Seasonal Changes on Content and Profile of Soluble Carbohydrates in Tubers of Different Varieties of Jerusalem Artichoke (Helianthus Tuberosus L.). Journal of Agricultural and Food Chemistry 2007, 55(23), 9401–9408.
- Vergauwen, R.; Van Laere, A.; Van Den Ende, W. Properties of Fructan: Fructan 1-Fructosyltransferases from Chicory and Globe Thistle, Two Asteracean Plants Storing Greatly Different Types of Inulin. Plant Physiology 2003, 133, 391–401.
- Vilhalva, D.A.; Cortelazzo, A.L.; Carvalho, A.M.; Rita De Cássia, L. Histochemistry and Ultrastructure of Campuloclinium Chlorolepis (Asteraceae) Tuberous Roots Accumulating Fructan: Evidences of Functions Other than Reserve Carbohydrate. Australian Journal of Botany 2011, 59(1), 46–52.
- Apolinario, A.C.; De Lima Damasceno, B.P.G.; De Macêdo Beltrão, N.E.; Pessoa, A.; Converti, A.; Da Silva, J.A. Inulin-Type Fructans: A Review on Different Aspects of Biochemical and Pharmaceutical Technology. Carbohydrate Polymers 2014, 101, 368–378.
- Bach, V.; Kidmose, U.; Kjeldsen Bjørn, G.; Edelenbos, M. Effects of Harvest Time and Variety on Sensory Quality and Chemical Composition of Jerusalem Artichoke (Helianthus Tuberosus) Tubers. Food Chemistry 2012, 133(1), 82–89.
- Bosscher, D.; Van Loo, J.; Franck, A. Inulin and Oligofructose as Functional Ingredients to Improve Bone Mineralization. International Dairy Journal 2006, 16(9), 1092–1097.
- Kim, Y.; Faqih, M.N.; Wang, S.S. Factors Affecting Gel Formation of Inulin. Carbohydrate Polymers 2001, 46, 135–145.
- Lopushniak, V.; Sloboda, P. Bioenergetical Appraisal of the Technology of Jerusalem Artichokes Growing at Different Systems of Fertilization in Western Forest and Steppe Regions of Ukraine. Econtechmod. An International Quarterly Journal on Economics. in Technology 2014, 3(4), 29–33.
- Bernal, B.; Calle, J.; Duarte, E.; Pizón, R.; Velásquez, M. Inulina a Partir De Dahlia Imperialis Roetz. Revista Colombiana De Ciencias Químico Farmacéuticas 2009, 34(2), 122–125.
- Wang, H.; Zhang, Z.; Liang, L.; Wen, S.; Liu, C.; Xu, X. A Comparative Study of High-Performance Liquid Chromatography and Colorimetric Method for Inulin Determination. European Food Research and Technology 2010, 230(5), 701–706.
- Elok, Z.; Wilda, A. Comparative Study of Inulin Extracts from Dahlia, Yam, and Gembili Tubers as Prebiotic. Food and Nutrition Sciences 2013, 4, 8–12.
- Ananina, N.A.; Andreeva, O.A.; Mycots, L.P.; et. al. Standardization of Inulin Extracted from Dahlia Single Tubers and Some Physicochemical Properties of Inulin. Pharmaceutical Chemistry Journal 2009, 43, 157–159.
- Abozed, S.S.; Abdelrashid, A.; El-Kalyoubi, M.; Hamad, K.I. Production of Inulin and High-Fructose Syrup from Jerusalem Artichoke Tuber (Helianthus Tuberosus L.). Annals of Agricultural Sciences 2009, 54, 417–423.
- Biedrzycka, E.; Bielecka, M. Prebiotic Effectiveness of Fructans of Different Degrees of Polymerization. Trends in Food Science & Technology 2004, 15(3–4), 170–175.
- Coxam, V.;. Inulin-Type Fructans and Bone Health: State of the Art and Perspectives in the Management of Osteoporosis. British Journal of Nutrition 2005, 93(S1), 111–123.
- López, M.G.; Urías-Silvas, J.E. Agave Fructans as Prebiotics. In Recent Advances in Fructooligosaccharides Research; Shiomi, N., Benkeblia, N., Onodera, S.; Eds; Research Signpost: Kerala, Trivandrum, India, 2007; 1–14.
- Ku, Y.; Jansen, O.; Oles, C.J.; Lazar, E.Z.; Rader, J.I. Precipitation of Inulins and Oligoglucoses by Ethanol and Other Solvents. Food Chemistry 2003, 81, 125–132.
- Panchev, I.; Delchev, N.; Kovacheva, D.; Slavov, A. Physicochemical Characteristics of Inulins Obtained from Jerusalem Artichoke (Helianthus Tuberosus L.). European Food Research and Technology 2011, 233(5), 889–896.
- Zhu, Z.Y.; Lian, H.Y.; Si, C.L.; Liu, Y.; Liu, N.; Chen, J.; Ding, L.N.; Yao, Q.; Zhang, Y. The Chromatographic Analysis of Oligosaccharides and Preparation of 1-Kestose and Nystose in Yacon. International Journal of Food Sciences and Nutrition 2012, 63(3), 338–342.
- Choque Delgado, G.T.; Tamashiro, W.M.S.C.; Pastore, G.M. Review Immunomodulatory Effects of Fructans. Food Research International 2010, 43, 1231–1236.
- De Gennaro, S.; Birch, G.G.; Parke, S.A.; Stancher, B. Studies on the Physicochemical Properties of Inulin and Inulin Oligomers. Food Chemistry 2000, 68(2), 179–183.
- Lingyun, W.; Wang, J.; Zheng, X.; Teng, D.; Yang, Y.; Cai, C.; Feng, T.H.; Zhang, F. Studies on the Extracting Technical Conditions of Inulin from Jerusalem Artichoke Tubers. Journal of Food Engineering 2007, 79, 1087–1093.
- Zeng, J.; Hu, Y.; Gao, H.; Sun, J.; Ma, H. Fructooligosaccharides Impact on the Hydration and Retro-Gradation of Wheat Starch and Gel. International Journal of Food Properties 2016, 19(12), 2682–2692.
- Mansouripour, S.; Mizani, M.; Rasouli, S.; Gerami, A.; Sharifan, A. Effect of Inulin and Galactooligosaccharides on Particle Size Distribution and Rheological Properties of Prebiotic Ketchup. International Journal of Food Properties 2017, 20(1), 157–170.
- Hernalsteens, S.; Maugeri, F. Properties of Thermostable Extracellular FOS-producing Fructofuranosidase from Cryptococcus Sp. European Food Research and Technology 2008, 228, 213–221.
- López-Molina, D.; Navarro-Martínez, M.D.; Rojas-Melgarejo, F.; Hiner, A.N.; Chazarra, S.; Rodríguez-López, J.N. Molecular Properties and Prebiotic Effect of Inulin Obtained from Artichoke (Cynara Scolymus L.). Phytochemistry 2005, 66, 1476–1484.
- Moerman, F.T.; Van Leeuwen, M.B.; Delcour, J.A. Enrichment of Higher Molecular Weight Fractions in Inulin. Journal of Agricultural and Food Chemistry 2004, 52, 3780–3783.
- Roberfroid, M.B.;. Inulin-Type Fructans: Functional Food Ingredients. Journal of Nutrition 2007, 137, 2493–2502.
- Rubel, I.A.; Pérez, E.E.; Genovese, D.B.; Manrique, G.D. In Vitro Prebiotic Activity of Inulin-Rich Carbohydrates Extracted from Jerusalem Artichoke (Helianthus Tuberosus L.) Tubers at Different Storage Times by Lactobacillus Paracasei. Food Research International 2014, 62, 59–65.
- García-Vieyra, M.I.; Del Real, A.; López, M.G. Agave Fructans: Their Effect on Mineral Absorption and Bone Mineral Content. Journal of Medicinal Food 2014, 17(11), 1247–1255.
- Matusek, A.; Meresz, P.; Le, T.K. D.; Oersi, F. Effect of Temperature and pH on the Degradation of Fructo-Oligosaccharides. European Food Research and Technology 2009, 228, 355–365.
- CarboPac Combined Product Manual. Document № 031824-08/Dionex Corporation. – May 2010. – 80p.
- Timmermans, J.W.; Van Leeuwen, M.B.; Tournois, H.; Wit, D.D.; Vliegenthart, J.F. Quantitative Analysis of the Molecular Weight Distribution of Inulin by Means of Anion Exchange HPLC with Pulsed Amperometric Detection. Journal of Carbohydrate Chemistry 1994, 13(6), 881–888.
- Cooper, P.D.; Masinello, G.R. Rotein a Treatment of Cancer: Activation of Serum Component with Trans-Species anti-B16 Melanoma Activity. International Journal of Cancer 1983, 32(6), 737–744.
- Van Waes, C.; Baert, J.; Carlier, L.; Van Bockstaele, E. A Rapid Determination of the Total Sugar Content and the Average Inulin Lenght in Roots of Chicory (Cichorium Intybus L). Journal of the Science of Food and Agriculture 1998, 76, 107–110.
- Petkova, N.; Ivanov, I.; Vrancheva, R.; Ultrasound, P.A. Microwave-Assisted Extraction of Elecampane (Inula Helenium) Roots. Natural Product Communications 2017, 12(2), 171–174.