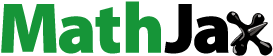
ABSTRACT
The objective of this study was to investigate the effects of various pHs and NaCl concentrations on physicochemical properties of surimi from grass carp during washing. Surimi protein recovery was higher with 0.25% and 0.5% NaCl at the pH 5 or 7 than that with other pHs and NaCl concentrations, while the highest recovery was obtained with 0.25% NaCl at the pH 7. It decreased protein recovery and salt-soluble protein of surimi as NaCl increased by more than 1.0% during washing. The water-soluble protein content was significantly higher at the pH 3 than at other pHs, while the SDS-PAGE of surimi protein exhibited intense sarcoplasmic protein bands at the pH 3. There were significantly fewer hydrophobic groups of actomyosin treated with 0.25% NaCl at the pH 5 or 7 than that with other pHs and NaCl concentrations in washing solution. The α-helix of actomyosin was increased with the increase of NaCl during washing. In general, the washing with 0.25%−0.5% NaCl at the pH 5–7 could significantly increase the yield of surimi from grass carp.
KEYWORDS:
Introduction
Surimi is a muscle protein slurry obtained mainly by head removal, gutting, meat harvesting, cleaning, dehydration, refining, adding cryoprotectant and freezing, while it is used in the production of many high-value reconstituted fish products due to its gel-forming ability and high nutritional value. [Citation1] Since surimi is made from minced meat, it provides the potential opportunity to use different sources of the protein for its production, such as underutilized species with little commercial value including freshwater fish.
Washing process is often considered an important step in determining the quality of surimi by continuously washing the fish with cold water to remove water-soluble protein, fat, endogenous proteases, and other undesirable substances in order to improve myofibrillar protein content and surimi quality.[Citation2,Citation3] The functionality of surimi proteins is influenced by many factors, such as pH, ionic strength, temperature, oxidation, and additives. Among these factors, ionic strength or salt concentration is one of the key factors because myofibrillar protein, the main component in surimi, is considered to be a salt-soluble protein.[Citation4] The salt concentration and pH of the washing medium affected the structural and physicochemical properties of surimi protein and gel properties of the surimi from silver carp (Hypophthalmichthys molitrix).[Citation1,Citation2] The addition of NaCl (0.3–0.6 M) caused the extension and dissolution of myosin by interfering with electrostatic interaction.[Citation5] Na+ of 0.25–0.5% had less effect on the structure of myofibrillar protein from silver carp.[Citation1] The effect of NaCl on meat protein is most likely due to the different binding capacity of Cl− and Na+ to protein increasing the electrostatic repulsion between myosin and actin filaments.[Citation6] More water molecules are bound to the protein as the pH deviates from the isoelectric point (pI), because it causes an increase of protein solubility with the increase of charges and hydration. Salt can make protein solubilizing (salting-in) or precipitation (salting-out).[Citation7] Isolated proteins can be easily separated from unwanted substances due to pH-dependent proteins, while proteins can be re-precipitated with high concentration and purity.[Citation8]
Grass carp (Ctenopharyngodon idella) is a popular species of freshwater fish in China, due to its rapid growth, high production yield, and low price. It can also be processed as surimi-based products.[Citation9] However, there has been no comprehensive study for the effect of washing with various pHs and NaCl concentrations on the physicochemical properties of surimi protein. The objective of this study was to investigate the effects of washing solution with various pHs and NaCl concentrations on the physicochemical properties of surimi from grass carp. Furthermore, the physicochemical changes of grass carp actomyosin were determined to further understand their characteristics during washing with different pHs and NaCl concentrations.
Materials & methods
Materials
Live grass carp (weight: 2.0 ± 0.3 kg) was purchased from a local supermarket (Wuhan, Hubei, China) and transferred to the laboratory within 30 min. On arrival, grass carp was stunned and then immediately headed, skinned, and washed with 4°C water. The white muscle was separated manually from fish body. Fish mince was prepared by a mini chopper (HR7625, Philips, Hong Kong, China) for 1 min. Analytical grade chemicals were used in the experiment. All sample preparations were performed on ice to maintain the temperature below 4°C.
Phenylmethylsulfonyl fluoride and sodium dodecyl sulfate were purchased from Amresco Co., Ltd. (Radnor, Pennsylvania, USA). Bovine serum albumin was purchased from Roche Co., Ltd. (Basel, Switzerland). Other chemical regents were purchased from Sinopharm Group Chemical Reagent Co., Ltd. (Shanghai, China). All chemical reagents were of analytical grade in the experiment.
Preparation of Surimi
The carp mince was subjected to three washing cycles with a water/meat ratio of 3:1 (v/w) and a washing time of 5 min. The washing solution was prepared with various pHs (3, 5, 7, and 9) and NaCl concentrations (0%, 0.25%, 0.5%, 1.0%, and 2.0%). The pH of washing solution was adjusted by 0.5% Na2CO3 and citric acid. The slurry was strained with a double-layer cheesecloth, while the excess water was manually squeezed out. The washed mince was then mixed with cryoprotectants (4% sucrose, 4% sorbitol, and 0.3% sodium tripolyphosphate), while the moisture content was adjusted to 78% by adding cold distilled water (4°C). The surimi was vacuum packed and stored at −20°C until use.
Actomyosin preparation
The extraction of actomyosin was based on the method of Liu et al.[Citation10] with some modification. Washed fish muscle sample (3 g) was mixed in five volumes of cold phosphate buffer (50 mM NaCl, 20 mM sodium phosphate, and 0.05 mM phenylmethylsulfonyl fluoride, pH 7.0). Homogenization was conducted in a homogenizer for 1 min. The homogenate was centrifuged at 5,000 × g for 10 min at 4°C. The precipitate was homogenized with the same buffer and centrifuged once more. The resultant residue was homogenized in 10 volumes of cold phosphate buffer (0.6 M NaCl and 20 mM potassium phosphate, pH 7.0) and then centrifuged at 10,000 × g for 5 min at 4°C. The supernatant was collected and diluted with three volumes of cold distilled water (4°C). The precipitate was collected by centrifugation at 10,000 × g for 10 min at 4°C and then dissolved in 0.6 M NaCl.
Protein recovery
Protein recovery was determined by the method of Lin and Park[Citation11] with some modification. Surimi or fish muscle sample (3 g) was homogenized with 5% (w/v) sodium dodecyl sulfate (SDS) solution to a final volume of 30 mL in a homogenizer (FJ200-SH, Shanghai Specimen and Model Factory, Shanghai, China) for 1 min. The homogenate was incubated in an 80°C water bath for 1 h and then centrifuged (8,000 × g, Avanti J-E, Beckman Coulter, Inc., Brea, CA, USA) for 20 min to remove undissolved debris. Protein concentration was determined by the method of Lowry et al.[Citation12] using bovine serum albumin (BSA) as standard. Absorbance was measured at 750 nm. The protein recovery was calculated from the ratio of protein concentration between surimi and fish muscle:
where Cs and C0 represent protein concentration of surimi and fish muscle; Ms and M0, respectively, are the weight of surimi and fish muscle.
Water-soluble protein and salt-soluble protein content
Based on the method of Lian et al.[Citation13] with slight modification, surimi (5 g) was mixed in 45 mL low-salt phosphate buffer (LSPB: 0.05 M KCl, 0.02 M NaH2PO4, and 0.02 M Na2HPO4, pH 7.0) and 45 mL high-salt phosphate buffer (HSPB: 0.5 M KCl, 0.02 M NaH2PO4 , and 0.02 M Na2HPO4, pH 7.0), respectively. Homogenization was conducted for 1 min. The homogenate was stirred for 30 min and then centrifuged (10,000 × g) at 4°C for 20 min. Due to the interference of potassium ion, the protein concentration of the supernatant was measured by the Bradford method using bovine serum albumin as a standard. The content of water-soluble protein was the concentration of LSPB, while the content of salt-soluble protein was the difference between LSPB and HSPB.
Sodium dodecyl sulfate-polyacrylamide gel electrophoresis (SDS-PAGE)
The SDS-PAGE of surimi protein was determined according to the method of Zhou and Yang[Citation3] with some modification. The washed sample (3 g) was homogenized with 5% (w/v) sodium dodecyl sulfate (SDS) solution to a final volume of 30 mL for 1 min. The homogenate was incubated in an 85°C water bath for 30 min to dissolve all sarcoplasmic and myofibrillar proteins. Samples were then centrifuged (10,000 × g) for 20 min at 4°C to remove undissolved debris. The protein concentration was determined by the method of Lowry et al.[Citation12] The protein concentration of the sample was equilibrated to 1.0–1.5 mg/mL. SDS-PAGE was performed in stacking (5%) and separating (10%) polyacrylamide gels. SDS-PAGE gel was stained in 0.02% Coomassie blue R-250 and distained in 50% methanol and 7.5% acetic acid. There was a mixture of a wide range of molecular weight standards (10–250 kDa) (Thermo Fisher Scientific Inc., Rockford, IL, USA) used in SDS-PAGE.
Intrinsic fluorescence (IF)
Fluorescence spectra of actomyosin were recorded on a Hitachi F-4600 Spectro fluorophotometer (Hitachi High-Tech). Co. Ltd., Tokyo, Japan) in a 10-mm path length quartz cell, according to the method of Wang et al.[Citation1] The concentration of actomyosin was 0.05 mg/mL. The excitation wavelength was set at 297 nm and the emission spectra were recorded in the range of 310–420 nm. It was 5-nm wide for excitation and emission slit.
Circular dichroism spectrum
Circular dichroism (CD) spectrum of actomyosin was measured according to the method of Liu et al.[Citation14] with slight modification. The concentration of actomyosin was 0.1 mg/mL. The actomyosin sample was scanned in the far UV region (197–250 nm) by using a Jasco J-1500 spectrophotometer (Jasco Co., Tokyo, Japan) with a 1-mm optical path. Molar ellipticity of actomyosin was determined using a mean residue weight of 110 g/mol. The content of α-helix structure was determined using the protein secondary structure estimation program (Yang’s method).
Statistical analysis
Analysis of variance was performed using the SPSS package (SPSS 26.0 for Windows, SPSS Inc, Chicago, IL, USA). Differences among the mean values of various treatments were measured by Duncan’s multiple-range test (p < .05). All sample conditions were replicated in triplicates with three repeat measurements for each replication.
Results and discussion
Protein recovery
shows the protein recovery of grass carp surimi prepared by washing with different pHs and NaCl concentrations. Myofibrillar protein is pH sensitive and could be precipitated at the isoelectric point (pI) to collect more protein, thus increasing myofibrillar protein concentration.[Citation15] Without salt, the protein recovery did not significantly change as the pH shifted from acid to alkaline (). Using 0.25% or 0.5% NaCl in washing, protein recovery was lower at the pH 3 or 9 than that at the pH 5 or 7. The highest protein recovery rate was obtained in the washing solution with 0.25% NaCl at the pH 7. However, protein recovery of surimi decreased significantly (p < .05) as salt concentration increased more than 1%. Its protein recovery with 2% NaCl was significantly lower than that with other treatments because higher salt concentration led to protein loss through salt penetration.[Citation16] Due to electrostatic interaction between the protein and ionic environment, higher salt concentration can lead to more protein solubilization, resulting in lower protein recovery.[Citation17,Citation18] The deviation from the protein pI could also cause excess charges between proteins and increase the solubility of proteins, resulting in the loss of protein during washing. With 2% NaCl, protein recovery at the pH 3 was significantly higher than that at other pHs (p < .05, ) because protein–protein interactions (aggregation) were improved and solubility was reduced because protein charges were completely masked by Cl− and electrostatic repulsion was reduced in the acidic pH range.[Citation19] The protein recovery from bigeye snapper (P. tayenus) by the acid-assisted process was significantly higher than that by the alkaline-assisted process.[Citation20] The acid treatment usually showed a higher yield than the alkaline one.[Citation3] Results showed that the pH and salt concentration could effectively influence the protein recovery from grass carp during washing and the highest recovery was obtained with 0.25% NaCl at the pH 7.
Water-soluble protein and salt-soluble protein content
presents the effects of different pHs and NaCl concentrations on the water-soluble protein and salt-soluble protein of surimi from grass carp. The water-soluble protein was significantly higher at the pH 3 than at other pHs (p < .05) because the solubility of sarcoplasmic protein in the acidic condition (pH 3) was less than that in the alkaline condition (pH 9).[Citation21] Without salt, the water-soluble protein was much higher at the pH 3 than other pHs, or there was more precipitate for the water-soluble protein at the pH 3. It indicated that the water-soluble protein might probably have more negative charges on its surface. Therefore, it might counteract the negative charges of the water-soluble protein and reduce its solubility or increase its precipitate at acid environment such as pH 3. Negative surface charge in the protein very strongly correlated with increased protein solubility.[Citation22] At the pH 5–9, the water-soluble protein decreased with increasing NaCl concentrations. The addition of salt could increase electrostatic repulsion of the water-soluble protein, resulting in an increase of its solubility during washing. The increase of salt in water might probably shift the pI of sarcoplasmic protein to acidic,[Citation23] resulting in an decrease of sarcoplasmic protein solubility or the increase of its precipitate. Water-soluble proteins aggregated by hydrophobic interactions could also lead to a higher water-soluble protein in surimi.[Citation24] The washing solution with 0.25%−2% NaCl at the pH 5–9 could effectively reduce the water-soluble protein in surimi from grass carp.
Figure 2. Water-soluble protein and salt-soluble protein concentration of grass carp surimi washed with different pHs and NaCl concentrations.
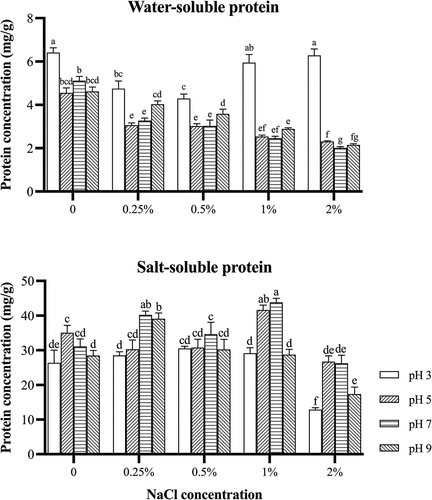
Without salt, the salt-soluble protein was higher at the pH 5 than the pH 3 or 9 (). With 0.25% NaCl in washing water, the salt-soluble protein was significantly higher at the pH 7 or 9 than that at the pH 3 or 5 (p < .05). With 0.5% NaCl, there was no significant difference in the salt-soluble protein at different pHs (p > .05). When NaCl concentration was 1.0% or 2.0%, the salt-soluble protein was significantly higher at the pH 5 or 7 than at the pH 3 or 9 (p < .05). The acidic (pH 3) and alkaline (pH 9) conditions could cause excess positive or negative charges in the washing and increase the electrostatic repulsion of the protein surface, resulting in an increase of salt-soluble protein solubility. Such effect was increased with the increase of NaCl concentration, especially more than 1% (). Changes in the NaCl concentration affected the electrostatic charges of protein molecules and consequently changed their dielectrics.[Citation25] Therefore, the washing under the pH 5 or 7 could obtain more salt-soluble protein from grass carp even under a higher salt washing ().
DS-PAGE pattern
SDS-PAGE is a commonly used technique for protein analysis. SDS-PAGE was used to further confirm the existence of high-molecular-weight aggregates following grass carp surimi prepared at different pHs and NaCl concentrations (). Myosin heavy chain (MHC, 220 kDa) and actin (43 kDa) were two major bands in the SDS-PAGE of grass carp surimi protein. Surimi washed at pH 3 generally produced intense MHC and actin bands probably because acidic condition could generate excess positive charges, which would counteract proteins and increase the aggregation of bands.[Citation26] There were more low-molecular-weight (35–43 kDa and 100–130 kDa) bands appeared at the pH 3 probably because the protein was decomposed by acidic condition or the protease activity was increased by acidic environment, leading to protein decomposition.[Citation27] In addition, the characteristic bands of sarcoplasmic protein were mainly distributed in 25–70 kDa,[Citation28] while the water-soluble protein was higher in surimi washed at the pH 3 (). It tended to decrease intensities of those characteristic bands as the pH increased. It was lower for MHC band intensity of surimi washed without NaCl than that washed with NaCl () because the addition of NaCl could reduce the loss of myofibrillar protein and promote MHC polymerization.[Citation29] Surimi washed with 0.25% or 0.5% NaCl exhibited intense protein bands, indicating that 0.25% or 0.5% NaCl in the washing was less destructive to fish protein. The intensity of the bands increased as NaCl increased upto 1% in the washing, but it decreased as the concentration reached 2%. Myofibrillar protein could dissolve more in the washing as more addition of salt, resulting in the loss of MHC and the removal of actin.[Citation30] MHC and actin band intensities of surimi were significantly intense washed with 1% NaCl than that other concentrations of salt because there was higher salt-soluble protein at 1% NaCl (). Therefore, surimi washed with 0.25% or 0.5% NaCl exhibited intense protein bands. There were much intense bands in the low molecular weights of surimi washed at the pH 3, indicating that there was more water-soluble protein in surimi washed at the pH 3 than other pHs ().
Intrinsic fluorescence of actomyosin
Actomyosin is a major protein in myofibrils and mainly contributes to the gelation of muscle proteins.[Citation10] Proteins are considered to have intrinsic fluorescence, which primarily reflects the contribution from tryptophan (Try) and tyrosine (Tyr) residues.[Citation31] The intrinsic fluorescence of Try and Tyr residues can reflect the tertiary structural changes of the protein.[Citation1] shows the intrinsic fluorescence spectra of actomyosin at different pHs and NaCl concentrations. There was the highest intrinsic fluorescence in all treatments at 333 nm. The fluorescence intensity of actomyosin was decreased at all pHs as NaCl increased from 0% to 0.25% in the washing. NaCl of 0.25% contributed to reduce the exposure of hydrophobic groups of actomyosin probably because 0.25% NaCl had the least effect on the tertiary structure of actomyosin. The fluorescence intensity increased in actomyosin first and then decreased with increasing NaCl concentration (0.25%−2%) at the pH 3, while there was higher fluorescence intensity in actomyosin treated with 0.5% NaCl. The fluorescence intensity of myofibrillar protein was higher at lower ionic strengths (0.25%−0.5%) for silver carp.[Citation1] More addition of salt could increase protein solubility and cause exposure of hydrophobic structural domains of the protein.[Citation32] However, chloride ion had a higher ability to interact with positively charged protein at lower pH, while the reduction in protein–protein electrostatic repulsion led to protein aggregation and reduced exposure to hydrophobic groups.[Citation33] It was generally lower for the fluorescence intensity of actomyosin at the pH 5 or 7, indicating less exposure to internal hydrophobic groups. The fluorescence intensity of actomyosin tended to increase with increasing NaCl concentration from 0.25% to 1% at the pH 7, probably because the increase of NaCl caused changes in the tertiary structure of the protein, which led to the exposure of Try buried inside the structure and the increase of intrinsic fluorescence intensity.[Citation34] The fluorescence intensity of actomyosin was significantly higher at the pH 9 than that at other pHs, especially with 2% NaCl, because the Try residues would be more exposed at the pH 9.[Citation35] The tertiary structural changes of actomyosin resulted in the exposure of internal hydrophobic groups and reflected the structural change of surimi proteins. It was consistent with the protein profile shown by SDS-PAGE, with less hydrolysis of surimi proteins treated with a neutral (pH 5 or 7) and low concentration of NaCl (). Results indicated that the washing solution with 0.25% NaCl at the pH 5 or 7 exposed fewer hydrophobic groups and had less effect on the tertiary structure of actomyosin.
α-helix of actomyosin
The α-helix structure is mainly stabilized by hydrogen bonds between carbonyl oxygen (CO) and amino hydrogen (NH) of a polypeptide chain, while electrostatic interactions between amino acids also contribute to the stability of secondary structures.[Citation36,Citation37] The α-helix content was used to investigate effect of different pHs and NaCl concentrations on secondary structure of actomyosin (). The concentration of NaCl had a significant effect on the secondary structure of actomyosin. Overall, the α-helix of actomyosin showed an increasing trend with increasing NaCl concentration, except with 1% NaCl at the pH 7 or 9. Adding salt could shield repulsive force[Citation38] so that the content of α-helix was higher as NaCl increased to 2.0%. The structure and stability of proteins could be altered by the net charge of the protein and the charged groups on the surface of proteins.[Citation39] More salt could easily break the interaction on stabilizing the internal structure and increasing the solubility.[Citation40] The α-helix of actomyosin was the lower at the pH 3, except with 1% NaCl. Myosin might have a net positive charge at the pH 3, which led to electrostatic repulsion between protein molecules and contributed to the protein denaturation unfolding.[Citation7] The α-helix of actomyosin was generally higher at the pH 5 or 7 and would slightly decrease as increased the pH upto 9 because the alkaline condition could lead to the unfolding of α-helix.[Citation41] Results indicated that actomyosin had lower α-helix at the pH 3. There was less effect on the α-helix structure of actomyosin treated with 0.25% or 0.5% NaCl at the pH 5 or 7 than that with other pHs and NaCl concentrations in washing solution. Washing with 0.25–0.5% NaCl at pH 5 or 7 showed less effect on the secondary structure of myofibrillar protein from silver carp.[Citation1] The pH and NaCl caused changes in α-helix content by altering the charge and hydrogen bonds on the protein surface, while they also disrupted the internal structure of the protein, leading to the exposure of hydrophobic groups. The α-helix increased () and the intrinsic fluorescence intensity tended to increase () as NaCl increased from 0.25% to 2% in the solution. There was less effect on both secondary and tertiary structures at pH 5–7, which was also consistent with the protein yield results (). Therefore, it was continuously unfolding for the secondary and tertiary structures of actomyosin and might have dissolved more protein into the washing solution and decreased the protein yield (). The washing condition had less effect on the structure of the protein and could have higher protein recovery during the washing. Neutral condition (pH 7) had less effect on the protein structure, and therefore there was higher protein yield and higher salt-soluble protein content (). Thus, the yield of surimi protein was higher in the washing with 0.25%−0.5% NaCl at the pH 7.
Conclusion
This study demonstrated the effects of pH and NaCl concentration on the physicochemical properties of surimi from grass carp. The highest recovery of surimi protein was obtained with 0.25% NaCl at the pH 7. It decreased protein recovery and salt-soluble protein of surimi as NaCl increased by more than 1.0% during washing. The washing caused a higher water-soluble protein into surimi at the pH 3 and exhibited intense sarcoplasmic protein bands in SDS-PAGE. The α-helix increased with increasing NaCl concentration and was higher at the pH 5 or 7. Less effect of α-helix structure was observed in actomyosin treated with 0.25% or 0.5% NaCl at the pH 5 or 7. Therefore, low concentration (0.25%) and neutral condition (pH 7) had less effect on the structure of actomyosin. Results indicated that the proper pH (pH 5–7) and NaCl concentration (0.25%−0.5%) could significantly increase the yield of surimi from grass carp during the washing.
CRediT authorship contribution statement
Yudong Wang: Conceptualization, Methodology, Validation, Formal analysis, Writing – Original Draft. Yuxin Tian: Investigation, Software, Data Curation. Jingwen Sun: Investigation, Formal analysis, Software. Hong Yang: Conceptualization, Funding acquisition, Investigation, Methodology, Resources, Supervision, Validation, Visualization, Writing – Review & Editing.
Disclosure statement
No potential conflict of interest was reported by the author(s).
Additional information
Funding
References
- Wang, Y.; Zhuang, Y.; Yan, H.; Lu, Y.; Deng, X.; Hu, Y.; Xiong, S.; Yang, H. The Influence of pH and Monovalent/Divalent Cations on the Structural and Physicochemical Properties of Myofibrillar Protein from Silver Carp. Food Chem. 2023, 404, 134519. DOI: 10.1016/j.foodchem.2022.134519.
- Zhang, L.; Li, Q.; Shi, J.; Zhu, B.; Luo, Y. Changes in Chemical Interactions and Gel Properties of Heat-Induced Surimi Gels from Silver Carp (Hypophthalmichthys molitrix) Fillets During Setting and Heating: Effects of Different Washing Solutions. Food Hydrocolloids. 2018, 75, 116–124. DOI: 10.1016/j.foodhyd.2017.09.007.
- Zhou, Y.; Yang, H. Effects of Calcium Ion on Gel Properties and Gelation of Tilapia (Oreochromis niloticus) Protein Isolates Processed with pH Shift Method. Food Chem. 2019, 277, 327–335. DOI: 10.1016/j.foodchem.2018.10.110.
- Sun, X.; Holley, R. A. Factors Influencing Gel Formation by Myofibrillar Proteins in Muscle Foods. Compr. Rev. Food Sci. Food Saf. 2011, 10(1), 33–51. DOI: 10.1111/j.1541-4337.2010.00137.x.
- Wang, G.; Liu, M.; Cao, L.; Yongsawatdigul, J.; Xiong, S.; Liu, R. Effects of Different NaCl Concentrations on Self-Assembly of Silver Carp Myosin. Food Biosci. 2018, 24, 1–8. DOI: 10.1016/j.fbio.2018.05.002.
- Basso, A. S.; Miguez, F. E.; Laird, D. A.; Horton, R.; Westgate, M. Assessing Potential of Biochar for Increasing Water-Holding Capacity of Sandy Soils. GCB Bioenergy. 2013, 5(2), 132–143. DOI: 10.1111/gcbb.12026.
- Wu, L.; Wu, T.; Wu, J.; Chang, R.; Lan, X.; Wei, K.; Jia, X. Effects of Cations on the “Salt in” of Myofibrillar Proteins. Food Hydrocolloids. 2016, 58, 179–183. DOI: 10.1016/j.foodhyd.2016.02.028.
- Matak, K. E.; Tahergorabi, R.; Jaczynski, J. A Review: Protein Isolates Recovered by Isoelectric Solubilization/Precipitation Processing from Muscle Food By-Products as a Component of Nutraceutical Foods. Food Res. Int. 2015, 77, 697–703. DOI: 10.1016/j.foodres.2015.05.048.
- Tao, L.; Tian, L.; Zhang, X.; Huang, X.; Long, H.; Chang, F.; Li, T.; Li, S. Effects of γ-Polyglutamic Acid on the Physicochemical Properties and Microstructure of Grass Carp (Ctenopharyngodon idellus) Surimi During Frozen Storage. LWT - Food Sci. Technol. 2020, 134, 109960. DOI: 10.1016/j.lwt.2020.109960.
- Liu, R.; Zhao, S. -M.; Yang, H.; D-D, L.; Xiong, S. -B.; Xie, B. -J. Comparative Study on the Stability of Fish Actomyosin and Pork Actomyosin. Meat Sci. 2011, 88(2), 234–240. DOI: 10.1016/j.meatsci.2010.12.026.
- Lin, T. M.; Park, J. W. Effective Washing Conditions Reduce Water Usage for Surimi Processing. J. Aquat. Food Prod. Technol. 1997, 6(2), 65–79. DOI: 10.1300/J030v06n02_06.
- Lowry, O.; Rosebrough, N.; Farr, A. L.; Randall, R. Protein Measurement with the Folin Phenol Reagent. J. Biol. Chem. 1951, 193(1), 265–275. DOI: 10.1016/S0021-9258(19)52451-6.
- Lian, P. Z.; Lee, C. M.; Hufnagel, L. Physicochemical Properties of Frozen Red Hake (Urophycis chuss) Mince as Affected by Cryoprotective Ingredients. J. Food Sci. 2000, 65(7), 1117–1123. DOI: 10.1111/j.1365-2621.2000.tb10249.x.
- Yin, T.; He, Y.; Liu, L.; Shi, L.; Xiong, S.; You, J.; Hu, Y.; Huang, Q. Structural and Biochemical Properties of Silver Carp Surimi as Affected by Comminution Method. Food Chem. 2019, 287, 85–92. DOI: 10.1016/j.foodchem.2019.02.066.
- Marmon, S. K.; Krona, A.; Langton, M.; Undeland, I. Changes in Salt Solubility and Microstructure of Proteins from Herring (Clupea harengus) After PH-Shift Processing. J. Agric. Food Chem. 2012, 60(32), 7965–7972. DOI: 10.1021/jf301352s.
- Ooizumi, T.; Kawase, M.; Akahane, Y. Permeation of Sodium Chloride into Fish Meat and Its Effect on Moisture Content as a Function of the Osmotic Pressure of the Soaking Solution. Fish. Sci. 2003, 69(4), 830–835. DOI: 10.1046/j.1444-2906.2003.00694.x.
- Wang, K.; Li, Y.; Zhang, Y.; Luo, X.; Sun, J. Improving Myofibrillar Proteins Solubility and Thermostability in Low-Ionic Strength Solution: A Review. Meat Sci. 2022, 189, 108822. DOI: 10.1016/j.meatsci.2022.108822.
- Liu, H.; Zhang, H.; Liu, Q.; Chen, Q.; Kong, B. Filamentous Myosin in Low-Ionic Strength Meat Protein Processing Media: Assembly Mechanism, Impact on Protein Functionality, and Inhibition Strategies. Trends Food Sci. Technol. 2021, 112, 25–35. DOI: 10.1016/j.tifs.2021.03.039.
- Sun, L. -C.; Lin, Y. -C.; Liu, W. -F.; Qiu, X. -J.; Cao, K. -Y.; Liu, G. -M.; Cao, M. -J. Effect of pH Shifting on Conformation and Gelation Properties of Myosin from Skeletal Muscle of Blue Round scads(Decapterus maruadsi). Food Hydrocolloids. 2019, 93, 137–145. DOI: 10.1016/j.foodhyd.2019.02.026.
- Panpipat, W.; Chaijan, M. Biochemical and Physicochemical Characteristics of Protein Isolates from Bigeye Snapper (Priacanthus Tayenus) Head By-Product Using pH Shift Method. Turk. J. Fish. Aquat. Sci. 2016, 16(1), 41–50. DOI: 10.4194/1303-2712-v16_1_05.
- Tadpitchayangkoon, P.; Park, J. W.; Yongsawatdigul, J. Conformational Changes and Dynamic Rheological Properties of Fish Sarcoplasmic Proteins Treated at Various pHs. Food Chem. 2010, 121(4), 1046–1052. DOI: 10.1016/j.foodchem.2010.01.046.
- Kramer, R. M.; Shende, V. R.; Motl, N.; Pace, C. N.; Scholtz, J. M. Toward a Molecular Understanding of Protein Solubility: Increased Negative Surface Charge Correlates with Increased Solubility. Biophys. J. 2012, 102(8), 1907–1915. DOI: 10.1016/j.bpj.2012.01.060.
- Thawornchinsombut, S.; Park, J. W. Role of Ionic Strength in Biochemical Properties of Soluble Fish Proteins Isolated from Cryoprotected Pacific Whiting Mince. J. Food Biochem. 2005, 29(2), 132–151. DOI: 10.1111/j.1745-4514.2005.00005.x.
- Kim, Y. S.; Yongsawatdigul, J.; Park, J. W.; Thawornchinsombut, S. Characteristics of Sarcoplasmic Proteins and Their Interaction with Myofibrillar Proteins. J. Food Biochem. 2005, 29(5), 517–532. DOI: 10.1111/j.1745-4514.2005.00023.x.
- Feng, D.; Xue, Y.; Li, Z.; Wang, Y.; Yang, W.; Xue, C. Dielectric Properties of Myofibrillar Protein Dispersions from Alaska Pollock (Theragra chalcogramma) as a Function of Concentration, Temperature, and NaCl Concentration. J. Food Eng. 2015, 166, 342–348. DOI: 10.1016/j.jfoodeng.2015.06.038.
- Marchetti, M. D.; Gomez, P. L.; Yeannes, M. I.; Loredo, A. B. G. Structure of Fish Proteins as Modified by Salting Procedures: A Rheological and Ultrastructural Analysis of Hake (Merluccius hubbsi) Fillets. J. Food Sci. 2022, 87(3), 1134–1147. DOI: 10.1111/1750-3841.16056.
- Thawornchinsombut, S.; Park, J. W. Role of pH in Solubility and Conformational Changes of Pacific Whiting Muscle Proteins. J. Food Biochem. 2004, 28(2), 135–154. DOI: 10.1111/j.1745-4514.2004.tb00061.x.
- Xia, M.; Chen, Y.; Guo, J.; Huang, H.; Wang, L.; Wu, W.; Xiong, G.; Sun, W. Water Distribution and Textural Properties of Heat-Induced Pork Myofibrillar Protein Gel as Affected by Sarcoplasmic Protein. LWT - Food Sci. Technol. 2019, 103, 308–315. DOI: 10.1016/j.lwt.2019.01.009.
- Núñez-Flores, R.; Cando, D.; Borderías, A. J.; Moreno, H. M. Importance of Salt and Temperature in Myosin Polymerization During Surimi Gelation. Food Chem. 2018, 239, 1226–1234. DOI: 10.1016/j.foodchem.2017.07.028.
- Guo, H.; Yang, Y.; Qiao, Y.; He, J.; Yao, W.; Zheng, W. Heat Stress Affects Fetal Brain and Intestinal Function Associated with the Alterations of Placental Barrier in Late Pregnant Mouse. Ecotoxicol. Environ. Saf. 2021, 227, 112916. DOI: 10.1016/j.ecoenv.2021.112916.
- Li, L.; Bai, Y.; Cai, R.; Wu, C.; Wang, P.; Xu, X.; Sun, J. Alkaline pH-Dependent Thermal Aggregation of Chicken Breast Myosin: Formation of Soluble Aggregates. CyTA - J. Food. 2018, 16(1), 765–775. DOI: 10.1080/19476337.2018.1470576.
- Zhu, C. Z.; Jin, H. Q.; Yin, F.; Cui, W. M.; Zhang, Q. H.; Zhao, G. M. Emulsion-Forming Properties of Heat-Induced Pork Myofibrillar Protein Affected by NaCl. International Journal of Food Science & Technology. 2021, 56(6), 3016–3025. DOI: 10.1111/ijfs.14945.
- Odahara, T.; Odahara, K. Various Salts Employed as Precipitant in Combination with Polyethylene Glycol in Protein/Detergent Particle Association. Heliyon. 2018, 4(12), e01073. DOI: 10.1016/j.heliyon.2018.e01073.
- Ren, Z.; Cui, Y.; Wang, Y.; Shi, L.; Yang, S.; Hao, G.; Qiu, X.; Wu, Y.; Zhao, Y.; Weng, W. Effect of Ionic Strength on the Structural Properties and Emulsion Characteristics of Myofibrillar Proteins from Hairtail (Trichiurus haumela). Food Res. Int. 2022, 157, 111248. DOI: 10.1016/j.foodres.2022.111248.
- Li, J.; Wu, M.; Wang, Y.; Li, K.; Du, J.; Bai, Y. Effect of pH-Shifting Treatment on Structural and Heat Induced Gel Properties of Peanut Protein Isolate. Food Chem. 2020, 325, 126921. DOI: 10.1016/j.foodchem.2020.126921.
- Satoh, Y.; Nakaya, M.; Ochiai, Y.; Watabe, S. Characterization of Fast Skeletal Myosin from White Croaker in Comparison with That from Walleye Pollack. Fish. Sci. 2006, 72(3), 646–655. DOI: 10.1111/j.1444-2906.2006.01195.x.
- Liu, R.; Zhao, S. -M.; Liu, Y. -M.; Yang, H.; Xiong, S. -B.; Xie, B. -J.; Qin, L. -H. Effect of pH on the Gel Properties and Secondary Structure of Fish Myosin. Food Chem. 2010, 121(1), 196–202. DOI: 10.1016/j.foodchem.2009.12.030.
- Haug, I. J.; Draget, K. I.; Smidsrød, O. Physical and Rheological Properties of Fish Gelatin Compared to Mammalian Gelatin. Food Hydrocolloids. 2004, 18(2), 203–213. DOI: 10.1016/S0268-005X(03)00065-1.
- Pace, C. N.; Grimsley, G. R.; Scholtz, J. M. Protein Ionizable Groups: pK Values and Their Contribution to Protein Stability and Solubility*. J. Biol. Chem. 2009, 284(20), 13285–13289. DOI: 10.1074/jbc.R800080200.
- Lanier, T.; Yongsawatdigul, J.; Carvajal-Rondanelli, P. Surimi Gelation Chemistry. In Surimi and Seafood; Boca Raton, Florida: CRC Press, 2013; 101–140. doi:10.1201/b16009-6.
- Li, H.; Zhang, X.; Zhao, C.; Zhang, H.; Chi, Y.; Wang, L.; Zhang, H.; Bai, S.; Zhang, X. Entrapment of Curcumin in Soy Protein Isolate Using the pH-Driven Method: Nanoencapsulation and Formation Mechanism. LWT - Food Sci. Technol. 2022, 153, 112480. DOI: 10.1016/j.lwt.2021.112480.