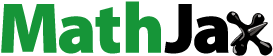
ABSTRACT
Ultrasonic-assisted green synthesis of silver nanoparticles is gaining more attention owing to its benefits over conventional synthesis process. The main objective of this study was to synthesize silver nanoparticles with aqueous cinnamon extract using ultrasound-assisted extraction and their physico-chemical characterization. Total phenolic content, DPPH, FRAP of cinnamon aqueous extract were 315 mg GAE/g, 89% scavenging activity and 9.5 mmol Trolox eq/100 g reducing power respectively. The synthesized silver nanoparticles were characterized by UV-visible spectroscopy, X-rays Diffraction (XRD), Scanning Electron Microscopy (SEM) with Energy Dispersive X-rays (EDX), Fourier transform infrared (FTIR) and Nuclear Magnetic Resonance (NMR). XRD spectra depicted that the nanoparticles were crystallite in structure and 50 nm in size. SEM micrographs showed that nanoparticles were spherical in shape. EDX, FTIR and NMR spectra indicated that the phenolic compounds present in cinnamon aqueous extract played their role in capping and reducing the silver ions. Cinnamon-based silver nanoparticles showed potential antimicrobial activity against Gram negative and Gram-positive bacteria. Thus, cinnamon extract obtained through ultrasonic-assisted extraction could be a valuable source of polyphenols, which actively participated in the reduction and stabilization of silver nanoparticles.
Introduction
Nanotechnology is a field of science which deals with the synthesis of particles having a size range from 1 to 100 nm. With a decrease in particle size, surface area and volume increase which leads toward enhanced chemical, physical and biological properties as compared to the particles having larger sizes.[Citation1,Citation2] Inert metal nanoparticles have immense biochemical, physicochemical and optoelectronic properties. Nanoparticles are widely used in pharmaceuticals and industrial sectors.[Citation3,Citation4] In spite of diverse range of metals which occur naturally, only a few metals (Silver, Gold, Palladium, Platinum) are considered for the synthesis of nano-sized materials.[Citation5,Citation6]
Recently, silver nano-size particles gained much attention owing to their antimicrobial properties and cost effectiveness.[Citation7] Most commonly silver nanoparticles synthesized by the chemical reduction methods by using different chemicals such as ascorbate, sodium borohydride and sodium citrate as reducing agents.[Citation8] However, silver nanoparticles obtained through chemical methods are not popular among consumers due to potential toxicity which restrict their usage in the preservation of foods. Phytochemicals are used to synthesize the safe and environmental friendly silver nanoparticles. Phytochemicals, in genesis of silver nano-particle, act as reducing agents.[Citation7] Here green chemistry plays its role and fulfill the desire that the surface of the AgNPs is shielded with the plant moieties such as tannins, flavonoids, glycosides, alkaloids, polyphenols, enzymes, proteins. Along with shielding and capping effects, plant compounds also work as surfactants and active.[Citation8–13]
In the recent year, ultrasonic-assisted synthesis of silver nanoparticles grabs the attention of the researchers and facilitates the speedy genesis of silver nanoparticles. Sonication is widely used in the manufacturing of metal nanoparticles due to its efficiency to synthesize smaller size of nanoparticles with finer distribution and less time.[Citation14] A recent study revealed that ultrasonic application in the synthesis of AgNP produced small size nanoparticles with strong antimicrobial activity.[Citation15]
Cinnamon Zeylanceum bark is used as medicine in many health problems and in cooking as condiment. Cinnamon bark extract contains considerable amount of antioxidants such as linalool, eugenol and cinnamaldehyd (75%).[Citation16,Citation17] The purpose of this study was to extract CZ with ultrasonic-assisted extraction. Further, cinnamon extract was explored for antioxidant potential and used for the synthesis of silver nanoparticles using ultrasonic technique, as only a few studies are available in which this technique is used for the successful synthesis of silver nanoparticles. Furthermore, silver nanoparticles were characterized by UV-Vis spectroscopy, XRD, FTIR, NMR, and SEM with EDX. Moreover, antimicrobial potential of the silver nanoparticles was explored against human pathogens S.aureus, E coli, S. typhi.
Materials and methods
Procurement of raw materials and chemicals
Cinnamon (Cinnamomum zeylancium) was procured from local market of Faisalabad, Pakistan. Silver Nitrate (AgNO3) and ethanol were purchased from Sigma Aldrich and reagents including Folin ciocalteu reagent, sodium carbonate, gallic acid, DPPH solution (2,2-Diphenyl-1-picrylhydrazyl), hydrochloric acid, Iron chloride (FeCl3), Merck Company, and were of analytical grade. Microbial strains including S. aureus and S. typhimurium, and E. coli were kindly provided by Department of Microbiology, Government College University, Faisalabad, Pakistan.
Extraction of cinnamon powder
Extraction of cinnamon powder was done with the method adopted by Gao et al.,[Citation18] with slight modifications. 10 grams of cinnamon powder was extracted in 200 ml DW under sonicator for 25 minutes at an amplitude of 50% (on/off 10s/4s). The resultant mixture was cooled at room temperature and centrifuged at 5000 rpm. Supernatant was collected and filtered through whatmann no. 1 filter paper and stored at 4°C for further use.
Antioxidant potential
Total phenolic content
Total polyphenol content was measured using the Folin-Ciocalteu colorimetric method described previously by Gao et al.[Citation19] Cinnamon extract 100 μL was mixed with 0.2 ml folin reagent and 2 ml water, solution was kept under room temperature for 3 min. In next step, 1 ml 20% sodium carbonate was added in the previously formed mixture in order to determine the polyphenol content after 1 hour of incubation. The absorbance of the mixture was measured at 760 nm with a spectrophotometer (HITACHI UH5300) against the standard curve of gallic acid.
DPPH
The DPPH radical-scavenging activity was determined using the method proposed by Yen and Chen.[Citation20] Briefly, 100 μM DPPH was mixed in 96% ethanol. Freshly prepared DPPH solution (1 ml) was mixed with 1 ml cinnamon extract and ethanol (3 ml), shaken well and rested for ten minutes at ambient temperature and reading was taken at 517 nm.
FRAP
FRAP reagent was obtained by mixing 300 μM acetate buffer pH 3.6 with a solution of TPTZ (10 μM) in HCL (40 μM) and FeCL3 (20 μM) in 10:1:1 volumetrically. Furthermore, 300 μL of stock solution was added to 10 μL sample and shaken constantly for five minutes. The absorbance of the final mixture was noted at 593 nm against a standard curve of Trolox. This assay was based on the reducing power of antioxidant compounds toward ferric salt.[Citation21]
Synthesis of silver nanoparticles
Aqueous cinnamon extract-based synthesis of silver nanoparticles was carried out in 1 mM sliver nitrate solution with the ratio of (1:9) in 1000 ml volume. All the reaction mixtures were sonicated for 20 minutes at 30 amp with same conditions and silver nitrate solution was added dropwise in the mixture in off time. The mixture of silver nitrate and extract was covered with aluminum foil to avoid photo-degradation. The obtained silver nanoparticles were washed with distilled water several times to remove unreacted materials after three time centrifugation at 10,000 rpm.[Citation15]
Nanoparticles characterization
UV-visible spectroscopy
Ultraviolet-visible spectroscopy was used to determine the wavelength of silver nanoparticles at wavelength of 200–800 nm using a cuvette having 1 cm length.
FTIR
Fourier transform infrared (FTIR) spectra of cinnamon-based silver nanoparticles was measured by FTIR spectrometer (Agilent Technologies, Cary 630 FTIR spectrometer). The silver nanoparticles were scanned by infrared and functional groups were detected by the computer-attached detector. Spectra of silver nanoparticles were recorded in the range of 650–4000 cm−1 using 32 scans and a resolution of 4 cm-1.[Citation22]
SEM (Scanning Electron Microscopy) with EDX (Energy Dispersive X-rays)
Silver nanoparticles morphology was observed with Field-Emission Scanning Electron Microscopy (Nova NanoSEM 450 Thermo-Fisher with ETD, TLD detector) at low voltage, without sputter coating. An additional capability of EDX was used for the detection of elemental metallic silver.
XRD
X-rays Diffraction spectra of silver nanoparticles samples was obtained through a D8 Advance Bruker X-ray diffraction spectrometer (Billerica, MA, USA) with Cu-Kα radiation 1.5406 Å. Silver nanoparticles were scanned at 2θ range from 2–60◦.
1H NMR (Proton NMR)
Proton NMR spectra of silver nanoparticles was obtained on NMR spectrometer (Bruker, Avance AMX-600) with the method opted by Vázquez et al.[Citation23] Briefly, Spectra was recorded in NMR spectrometer using a TXI probe using TMS as an internal reference, chloroform as solvent to dissolve the nanoparticle samples and data was processed with topspin 4.0.5.
Antimicrobial activity/assay
Antibacterial potential of cinnamon-based silver nanoparticles against S. aureus, S. typhimurium and E. coli was determined by disc diffusion method outlined by CLSI[Citation24] Briefly, Bacterial colonies were transferred to a saline solution. Muller Hilton agar was poured in sterile petri plates and 0.5 ml of bacterial suspension of each strain was transferred on the plates. Petri plates were then incubated for 24 hours at ambient temperature for their growth. Prior to analysis, silver nanoparticles were sonicated and completely in the distilled water. Afterwards, 8 mm filter paper disks were loaded with 50 µg and 100 µg silver nanoparticles and placed over the petri plates having bacterial strains. Inhibitory zones were measured in mm and experiments were repeated three times.
Statistical analysis
Data were analyzed with one way analysis of variance to compare the significant difference (P < .05) among the treatments using Statistics 8.1.
Results and discussion
Antioxidant potential of axtract
Antioxidant activity has a direct relationship with the concentration of phenolic compounds present in the plant extracts, which is generated due to the secondary plant metabolites during biotic and abiotic stress.[Citation25,Citation26] The results of antioxidant potential parameters are summarized in . TPC of ultrasonic-assisted extract of cinnamon was carried out by Folin method and results were expressed as mg gallic acid equivalent per gram of extract. Total phenolic content of cinnamon aqueous extract was found to be 315 mg GAE/g of extract.
Table 1. Antioxidant potential of aqueous cinnamon extract.
DPPH is a stable radical which undergoes rapid reduction by antioxidants. The scavenging activity of cinnamon aqueous extract was 89%. Higher scavenging activity of cinnamon aqueous extract obtained from ultrasonic extraction might be due to the compounds present in the extract which have such high affinity toward the DPPH radical. Ferric reducing antioxidant power (FRAP) is a well-established technique to determine the reducing power of the plant moieties. FRAP values of the obtained extract of cinnamon were found to be 9.5 mmol trolox eq/100 g.
UV-Visible spectroscopy
UV-vis absorbance for the silver nanoparticles was observed in range from 200 to 800 nm. A visible and broader shift was occurred at 439 nm due to the presence of silver nanoparticles in the solution (). In previously reported data, silver absorption band should be occurred at 390 nm and if the band occurs below 390 nm, it indicates the presence of organic impurities.[Citation9,Citation27] The higher shift at nm is associated with the successful growth of AgNPs. According to literature, AgNPs having size <100 nm and spherical shape show surface plasmon resonance in range of 400 to 500 nm in UV-vis spectra, while the shorter peak below 420 nm is due to the excessive amount of capping agents and ligands.[Citation28,Citation29]
Biochemical properties
FTIR (Fourier Transform Infrared Spectroscopy)
depicted the FTIR spectra of cinnamon extract based silver nanoparticles. FTIR spectrum is used to identify the natural moieties present in the cinnamon extract which reduce the silver ions. As a result of FTIR spectrum, different peaks are formed owing to the presence of different chemical groups. The peak at 3244 cm−1 corresponds to O-H stretching for alcohols and phenols, while 2920 cm−1 and 2851 cm−1 are associated to C-H stretch for alkanes. Peaks at 2372 cm−1, 2344 cm−1 correspond to O=C=O stretching and showed the presence of flavonoids on the surface of silver nanoparticles and confirm the formation of metal-flavonoid complex. A stretching peak at 2109 cm−1 is associated to C=C stretching of alkene molecules while 1735 cm−1 –N-H bonding in amines is an indication of the presence of proteins and enzymes in plants and plant extracts which possibly act as reducing and stabilizing agents for silver nanoparticles. Furthermore, the peaks in the region 1684 cm−1,1604 cm−1, 1522 cm−1, 1436 cm−1, 1362 cm−1, 1315 cm−1, 1280 cm−1 and 1243 cm−1 are due to – C-O stretching due to carboxylic acid, primary amines, alkanes, C-O, amine or ester carbonyl groups respectively. The peak between 1000 cm−1 and 1050 cm−1 is represented to polysaccharides. The Peak around 800 cm−1 is ascribed the presence of aromatic group.
1H NMR (Proton Nuclear Magnetic Resonance)
An NMR spectrum of cinnamon based silver nanoparticles is shown in . The signal occuring at approximately 0.8 and 0.9 ppm is due to the presence of saturated alkyl group (CH). Sharp signals appeared near 1.2 ppm are due to the presence of CH3-C-O- and C-CH2-C groups attached on the surface of silver nanoparticles. A small signal appeared at 2.3 might be due to the CH2, CH3 and groups of enzymes and proteins. Moreover, CH3 and CH2 groups which are connected to oxygen containing cyclic compounds (CH2-O-, CH3-O-) while CH3, CH2, CH groups connected to nitrogen in proteins and enzymes appeared at 3.5. The signal appeared at 4 ppm is also occurred due to the protein and enzymes which are essential part of plants and plant extracts. It should be noted that the signals appeared at 7.9 or 8 are due to the presence of aromatic compounds present on the surface of silver nanoparticles.
FTIR and 1H NMR spectra depict that the alkanes, alkenes, alkynes, phenols, amines, and alcohols are mainly found on the surface of silver nanoparticles and played the role in capping and stabilizing the silver nanoparticles. Similar studies were carried out by the Ulug et al.[Citation30]; Villalpando et al.,[Citation31] in which they observed the aforementioned chemicals on the surface of silver nanoparticles, which reduced the silver ions and successfully stabilized the particles.
Structural properties
XRD (X-rays Diffraction)
XRD was used to determine the crystalline nature of the materials. The XRD pattern of cinnamon-based silver nanoparticles is shown in . The XRD spectra showed three different divergence peaks at 2θ of 38, 44.80, and 64.5 and these peaks are corresponded to (111), (200) and (220) planes respectively and specifies the fcc (face-centered cubic) structure of cinnamon-based silver nanoparticles and compared with the standard powder card of silver (JCPDS 04–0783). As per the size calculations from full width half maximum (Scherrer equation), average crystallite size is in range from 40 nm to 55 nm. The broadening of peak from the base indicates the formation of small size silver nanoparticles. In a latest study (Nouri et al., 2023), they observed the smaller size of silver nanoparticle with face centered and cubic structure.
= wavelength of X rays
K= Scherrer constant (0.94)
D=FWHM
SEM with EDX
showed the cinnamon-based silver nanoparticles. SEM is usually used to determine the size, shape and morphology of the nanoparticles. SEM micrographs depicted individual nanoparticles, which are mostly spherical in shape and some of aggregates with undefined morphology, which was due to the presence of secondary metabolites of plant extracts. It can be assumed that plant moieties present in aqueous plant extract played a pivotal role in the synthesis of spherical silver nanoparticles. However, the aggregation might be caused by the secondary metabolites present in the extract. SEM images reveal that the size of silver nanoparticles is ranged from 45 to 65 nm (ImageJ software) a difference between size in SEM and XRD is might be due to the different techniques of measuring the size.
EDX was carried out to confirm the synthesis of silver nanoparticles. A peak occurred at 3 keV usually referred as the formation of pure metallic silver nanoparticles due to plasmon resonance.[Citation32,Citation33] Other elements such as carbon and oxygen are also present which is attributed to the presence of functional groups that act as reducing and capping agents in the formation of silver nanoparticles. On the other hand, EDX spectra did not show any nitrogen peak, this is owing to the absence of nitrate ions which formed during the reduction of AgNO3. It is a fact that cinnamon extract completely reduced the silver nitrate and successfully formed the silver nano particles without undergoing any side reactions.
Antimicrobial potential
Among the studied microbes, AgNPs showed the maximum inhibition against E. coli in a dose-dependent manners (50 ug, 20 mm and 100 ug, 25 mm) which is 18 mm followed by S. aureus (13 mm and 17 mm for 50 ug and 100ug) and S. typhimurium (14 mm and 19 mm) respectively (). AgNP showed a prominent inhibition against both gram positive and gram negative bacteria. Possible antimicrobial activity mechanism of AGNP is associated to inhibition and destruction of proteins in the cell wall of pathogens.[Citation34] Further, silver ions are formed from AgNP and generate reactive oxygen which further disrupt the electron transport chain and cause respiratory disorder. Thus production of above discussed factors (ROS, disrupted electron chain, energy transduction) pave a way to cell death.[Citation34–36]
Table 2. Antimicrobial potential of Silver Nanoparticles against E coli, S aureus, S typhi zone of inhibition is measured in mm.
Conclusion
In the current study, a cost-effective and environment-friendly green approach was opted for the synthesis of silver nanoparticles. Cinnamon extract obtained from the ultrasonic-assisted extraction was used as reducing and capping agent in the synthesis of silver nanoparticles. The UV-vis spectra and XRD revealed the crystalline structure of silver nanoparticles, while FTIR and NMR spectra of silver nanoparticles confirmed the presence of phytochemicals on the surface of silver nanoparticles. SEM micrographs depicted that nanoparticles are spherical in shape. Moreover, silver nanoparticles exhibited strong antimicrobial activity against E. coli, S. aureus and S. typhimurium. So, ultrasonic assisted extract of cinnamon has a great potential to synthesize small size nanoparticles with great antimicrobial potential and is a substitute for the toxic and expensive materials used in the synthesis of silver nanoparticles.
Disclosure statement
No potential conflict of interest was reported by the author(s).
References
- Dos Santos, C. A.; Seckler, M. M.; Ingle, A. P.; Gupta, I.; Galdiero, S.; Galdiero, M.; Gade, A.; Rai, M. Silver Nanoparticles: Therapeutical Uses, Toxicity, and Safety Issues. J. Pharm. Sci. 2014, 103(7), 1931–1944. DOI: 10.1002/jps.24001.
- Narayanan, K. B.; Sakthivel, N. Biological Synthesis of Metal Nanoparticles by Microbes. Adv. Colloid Interface Sci. 2010, 156(1–2), 1–13. DOI: 10.1016/j.cis.2010.02.001.
- Linic, S.; Aslam, U.; Boerigter, C.; Morabito, M. Photochemical Transformations on Plasmonic Metal Nanoparticles. Nat. Mater. 2015, 14(6), 567–576. DOI: 10.1038/nmat4281.
- Thakkar, K. N.; Mhatre, S. S.; Parikh, R. Y. Biological Synthesis of Metallic Nanoparticles. Nanomed. Nanotechnol. Biol. Med. 2010, 6(2), 257–262. DOI: 10.1016/j.nano.2009.07.002.
- Yoon, W.-J.; Jung, K.-Y.; Liu, J.; Duraisamy, T.; Revur, R.; Teixeira, F. L.; Sengupta, S.; Berger, P. R. Plasmon-Enhanced Optical Absorption and Photocurrent in Organic Bulk Heterojunction Photovoltaic Devices Using Self-Assembled Layer of Silver Nanoparticles. Sol. Energy Mater. Sol. Cells. 2010, 94(2), 128–132. DOI: 10.1016/j.solmat.2009.08.006.
- Yang, Y.; Jin, P.; Zhang, X.; Ravichandran, N.; Du, H.; Yu, C.; Ying, Q.; Xu, Y.; Yin, J.; Wang, K. New Epigallocatechin Gallate (EGCG) Nanocomplexes Co-Assembled with 3-Mercapto-1-Hexanol and β-Lactoglobulin for Improvement of Antitumor Activity. J. Biomed. Nanotechnol. 2017, 13(7), 805–814. DOI: 10.1166/jbn.2017.2400.
- Roy, A.; Bulut, O.; Some, S.; Mandal, A. K.; Yilmaz, M. D. Green Synthesis of Silver Nanoparticles: Biomolecule-Nanoparticle Organizations Targeting Antimicrobial Activity. Rsc. Adv. 2019, 9(5), 2673–2702. DOI: 10.1039/C8RA08982E.
- Wang, W.; Yu, Z.; Alsammarraie, F. K.; Kong, F.; Lin, M.; Mustapha, A. Properties and Antimicrobial Activity of Polyvinyl Alcohol-Modified Bacterial Nanocellulose Packaging Films Incorporated with Silver Nanoparticles. Food Hydrocoll. 2020, 100, 105411. Article 105411 DOI: 10.1016/j.foodhyd.2019.105411.
- Wasilewska, A.; Klekotka, U.; Zambrzycka, M.; Zambrowski, G.; Święcicka, I.; Kalska-Szostko, B. Physico-Chemical Properties and Antimicrobial Activity of Silver Nanoparticles Fabricated by Green Synthesis. Food Chem. 2023, 400, 133960. DOI: 10.1016/j.foodchem.2022.133960.
- Abalkhil, T. A.; Alharbi, S. A.; Salmen, S. H.; Wainwright, M. Bactericidal Activity of Biosynthesized Silver Nanoparticles Against Human Pathogenic Bacteria. Biotechnol. Biotechnol. Equip. 2017, 31(2), 411–417. DOI: 10.1080/13102818.2016.1267594.
- Qayyum, S.; Khan, A. U. Biofabrication of Broad Range Antibacterial and Antibiofilm Silver Nanoparticles. IET Nanobiotechnol. 2016, 10(5), 349–357. DOI: 10.1049/iet-nbt.2015.0091.
- Tippayawat, P.; Phromviyo, N.; Boueroy, P.; Chompoosor, A. Green Synthesis of Silver Nanoparticles in Aloe Vera Plant Extract Prepared by a Hydrothermal Method and Their Synergistic Antibacterial Activity. Peer J. 2016, 4, e2589. DOI: 10.7717/peerj.2589.
- Wang, L.; Periyasami, G.; Aldalbahi, A.; Fogliano, V. The Antimicrobial Activity of Silver Nanoparticles Biocomposite Films Depends on the Silver Ions Release Behaviour. Food Chem. 2021, 359, 129859. DOI: 10.1016/j.foodchem.2021.129859.
- Duan, H.; Wang, D.; Li, Y. Green Chemistry for Nanoparticle Synthesis. Chem. Soc. Rev. 2015, 44(16), 5778–5792. DOI: 10.1039/C4CS00363B.
- Nouri, A.; Yaraki, M. T.; Lajevardi, A.; Rezaei, Z.; Ghorbanpour, M.; Tanzifi, M. Ultrasonic-Assisted Green Synthesis of Silver Nanoparticles Using Mentha Aquatica Leaf Extract for Enhanced Antibacterial Properties and Catalytic Activity. Colloid Interface Sci. Commun. 2020, 35, 100252. DOI: 10.1016/j.colcom.2020.100252.
- Anjum, S.; Jacob, G.; Gupta, B. Investigation of the Herbal Synthesis of Silver Nanoparticles Using Cinnamon Zeylanicum Extract. Emergent Mater. 2019, 2(1), 113–122. DOI: 10.1007/s42247-019-00023-x.
- Vasconcelos, N. G.; Croda, J.; Simionatto, S. Antibacterial Mechanisms of Cinnamon and Its Constituents: A Review. Microb. Pathog. 2018, 120, 198–203. DOI: 10.1016/j.micpath.2018.04.036.
- Gao, L.; Mei, S.; Ma, H.; Chen, X. Ultrasound-Assisted Green Synthesis of Gold Nanoparticles Using Citrus Peel Extract and Their Enhanced Anti-Inflammatory Activity. Ultrason. Sonochem. 2022, 83, 105940. DOI: 10.1016/j.ultsonch.2022.105940.
- Gao, X.; Ohlander, M.; Jeppsson, N.; Björk, L.; Trajkovski, V. Changes in Antioxidant Effects and Their Relationship to Phytonutrients in Fruits of Sea Buckthorn (Hippophae Rhamnoides L.) During Maturation. J. Agric. Food. Chem. 2000, 48(5), 1485–1490. DOI: 10.1021/jf991072g.
- Yen, G. C.; Chen, H. Y. Antioxidant Activity of Various Tea Extracts in Relation to Their Antimutagenicity. J. Agric. Food. Chem. 1995, 43(1), 27–32. DOI: 10.1021/jf00049a007.
- Benzie, I. F. F.; Strani, J. J. The Ferric Reducing Ability of Plasma (FRAP) as a Measure of “Antioxidant power”: The FRAP Assay. Anal. Biochem. 1996, 239(1), 70–76. DOI: 10.1006/abio.1996.0292.
- Raza, M. A.; Saeed, F.; Afzaal, M.; Imran, A.; Niaz, B.; Hussain, M.; Al Jbawi, E.; Kashif Mukhtar, M.; Waleed, M.; Al Jbawi, E. Comparative Study of Cross-And Uncross-Linked Arabinoxylans Extracted from Maize Bran with Special Reference to Their Structural and Antioxidant Potential. Int. J. Food Prop. 2022, 25(1), 2495–2504. DOI: 10.1080/10942912.2022.2143524.
- Vázquez, B. E. R.; Rodríguez-Beas, C.; Iñiguez-Palomares, R. A.; Santacruz-Ortega, H.; Mendoza-Cruz, R.; Bazán-Díaz, L. S.; Navarro, R. E.; Rodríguez-León, E.; Navarro, R. E. Spectroscopic Analysis and Nuclear Magnetic Resonance for Silver Nanoparticles Synthesized with Trans-Resveratrol and Cis-Resveratrol. Colloid Polym. Sci. 2022, 300(5), 465–475. DOI: 10.1007/s00396-022-04957-3.
- Clinical and Laboratory Standards. Performance Standards for Antimicrobial Disk Susceptibility Tests; Approved Standard M2-A8; Clinical and Laboratory Standards Institute; CLSI: Wayne, PA, USA, 2003.
- Lee, S. Y.; Krishnamurthy, S.; Cho, C.-W.; Yun, Y.-S. Biosynthesis of Gold Nanoparticles Usingocimum Sanctumextracts by Solvents with Different Polarity. ACS Sustain. Chem. Eng. 2016, 4(5), 2651–2659. DOI: 10.1021/acssuschemeng.6b00161.
- Surveswaran, S.; Cai, Y.-Z.; Corke, H.; Sun, M. Systematic Evaluation of Natural Phenolic Antioxidants from 133 Indian Medicinal Plants. Food Chem. 2007, 102(3), 938–953. DOI: 10.1016/j.foodchem.2006.06.033.
- Ali, Z. A.; Yahya, R.; Sekaran, S. D.; Puteh, R. (2016). Green Synthesis of Silver Nanoparticles Using Apple Extract and Its Antibacterial Properties. Advances in Materials Science and Engineering, 2016. 10.1155/2016/4102196
- Klekotka, U.; Satula, D.; Nordblad, P.; Kalska-Szostko, B. Layered Magnetite Nanoparticles Modification–Synthesis, Structure, and Magnetic Characterization. Arabian J. Chem. 2020, 13(1), 1323–1334. DOI: 10.1016/j.arabjc.2017.11.002.
- Marhaba, S. Effect of Size, Shape and Environment on the Optical Response of Metallic Nanoparticles. In Noble and Precious Metals-Properties, Nanoscale Effects and Applications, IntechOpen, 2017; 10.5772/intechopen.71574.
- Ulug, B.; Turkdemir, M. H.; Cicek, A.; Mete, A. Role of Irradiation in the Green Synthesis of Silver Nanoparticles Mediated by Fig (Ficus Carica) Leaf Extract. Spectrochim. Acta, Part A: Mol. Biomol. Spectrosc. 2015, 135, 153–161. DOI: 10.1016/j.saa.2014.06.142.
- Villalpando, M.; Gomez-Hurtado, M. A.; Rosas, G.; Saavedra-Molina, A. Ag Nanoparticles Synthesized Using Lavandula Angustifolia and Their Cytotoxic Evaluation in Yeast. Mater. Today Commun. 2022, 31, 103633. DOI: 10.1016/j.mtcomm.2022.103633.
- Singha, S.; Neog, K.; Kalita, P. P.; Talukdar, N.; Sarma, M. P. Biological Synthesis of Silver Nanoparticles by Neptunia Oleraceae. Int. J. Basic Appl. Biol. 2014, 2(2), 55–59.
- Jemal, K.; Sandeep, B. V.; Pola, S. (2017). Synthesis, Characterization, and Evaluation of the Antibacterial Activity of Allophylus Serratus Leaf and Leaf Derived Callus Extracts Mediated Silver Nanoparticles. Journal of Nanomaterials, 2017.
- Yin, I. X.; Zhang, J.; Zhao, I. S.; Mei, M. L.; Li, Q.; Chu, C. H. The Antibacterial Mechanism of Silver Nanoparticles and Its Application in Dentistry. Int. J. Nanomed. 2020, 15, 2555. DOI: 10.2147/IJN.S246764.
- Onodera, A.; Nishiumi, F.; Kakiguchi, K.; Tanaka, A.; Tanabe, N.; Honma, A.; Yayama, K.; Yoshioka, Y.; Nakahira, K.; Yonemura, S. Short-Term Changes in Intracellular ROS Localisation After the Silver Nanoparticles Exposure Depending on Particle Size. Toxicology Reports. 2015, 2, 574–579. DOI: https://doi.org/10.1016/j.toxrep.2015.03.004.
- Slavin, Y. N.; Asnis, J.; H¨afeli, U. O.; Bach, H. Metal Nanoparticles: Understanding the Mechanisms Behind Antibacterial Activity. J. Nanobiotechnol. 2017, 15(1), 1–20. DOI: 10.1186/s12951-017-0308-z.