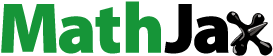
Abstract
Biological pretreatment of lignocellulosic biomass plays an important role for the enzymatic hydrolysis. In this study, a nitrogen-fixing and lignin-degrading strain of bacteria was isolated from an abandoned termite colony. Then, it was identified and named as R. ornithinolytica RS-1. To improve the degradation and enzymatic saccharification in corn stover, we used pretreatment with strain RS-1 to deplete the lignin, combined with a 2.5% mild NaOH pretreatment to further deplete the hemicellulose. After only seven days, lignin was degraded by the bacteria RS-1 with a 19% decrease, while the relative content of cellulose increased with 21%. Furthermore, the corn stover cellulose conversion was up to 48.58% by a two-stage process with 2.5% NaOH pretreatment. Meanwhile, significant lignin and hemicellulose removal were observed. Manganese peroxidase activity was highest on Day 3 with the value 181.0256 U/L and lignin peroxidase activity was highest on Day 5 with the value 37.473 U/L, respectively. Scanning electron microscopy (SEM), Fourier transform infrared spectroscopy (FT-IR) and X-ray diffraction (XRD) analyses showed significant chemical structural changes after the combined pretreatment. Therefore, strain RS-1 provided an efficient strategy to enhance the enzymatic hydrolysis efficiency by pretreatment of corn stover.
Highlights
A nitrogen-fixing and lignin-degrading bacteria R. ornithinolytica RS-1was isolated successfully.
Lignin in corn stover was selectively and effectively degraded in merely seven days.
A relatively high cellulose conversion yield (48.58%) of corn stover was obtained.
Glucose yield of two-stage pretreated corn stover was up to 360.74 µg/mL.
Graphical Abstract
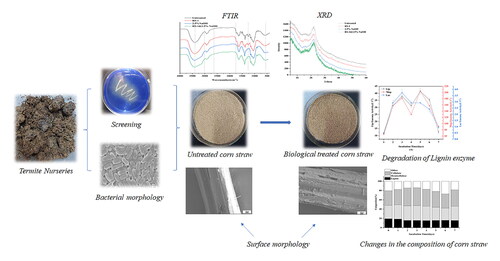
Introduction
Global pollution and energy crisis have aroused great attention to lignocellulose resources utilization and conversion. Lignocellulosic materials are one of the most important renewable sources. As an agricultural residue, corn stover has been widely considered as an abundant lignocellulosic biomass resource in the world [Citation1]. Most corn stover biomass gets burned or discarded every year. Corn stover is mainly composed of lignin, hemicellulose and cellulose [Citation2,Citation3]. All these carbohydrates can be converted into sugars. However, cellulose is tightly wrapped by lignin and hemicellulose, so it is difficult to use corn stover effectively [Citation4–6].
Pretreatment is an important step to upgrade corn stover into more valuable products, including fermentable sugars or biofuels. Generally, physical, chemical, biological or combined methods are utilized to reduce the lignin. Usually, the crystallinity and aggregation of the lignocellulosic material are reduced after physical crushing, which improves the hydrolysis effect and increases the conversion rate of cellulose. Lynd et al. [Citation7] used high-temperature pretreatment and concentrated acid hydrolysis of cellulose and the conversion was up to 85%. Wang et al. [Citation8] reported that twin-screw extrusion pretreatment can increase the glucose yield from 25 to 34 g/L. Lignin depletion is a key factor in the conversion of corn stover biomass to fermentable sugars. It was reported that acids, alkalis or inorganic salt pretreatments can effectively disrupt the structure of lignin [Citation9,Citation10]. Although chemical treatment is fast and effective, it can easily cause environmental pollution. Therefore, it is an effective strategy to find a clean technology to convert lignocellulose.
Biological pretreatment has been widely applied because it is safe, effective, and environmentally friendly. As known, there are many types of microorganisms that can degrade lignocellulose by producing cellulose-degrading enzymes [Citation11]. Lignin-degrading fungi are typically classified into three types: white rot, brown rot and soft rot fungi. White rot fungi effectively degrade the structure of lignin of corn stover; brown rot fungi can significantly degrade cellulose and hemicellulose; while soft rot bacteria degrade non-woody biomass materials [Citation12,Citation13]. In general, fungal pretreatment is more efficient and environmentally friendly, but it takes more time to treat corn stover. Bacteria have stronger environmental adaptability and faster growth rates compared to fungi, which is advantageous for large-scale lignin degradation [Citation14]. Therefore, research of lignin-degrading bacteria is necessary.
In this study, a nitrogen-fixing and lignin-degrading strain of bacteria was isolated successfully. The objective was to understand the efficacy of R. ornithinolytica RS-1 for the pretreatment of corn stover and to optimize the conditions of lignin degradation.
Furthermore, the combined pretreatment with 2.5% NaOH was carried out to deplete hemicellulose. Using the cellulose conversion rate as an indicator, the enzymatic hydrolysis of corn stover immediately occurred after each reaction. Scanning electron microscopy (SEM), Fourier transform infrared spectroscopy (FTIR) and X-ray Diffraction (XRD) were used to detect the main structural transformations.
Materials and methods
Isolation, screening and identification of strains
An abandoned termite colony was collected from Guangdong Province, China, and employed for the isolation of bacteria. About 1.0 g of termite nursery was washed in 49 mL of sterile distilled water and incubated for 3–4 h on a rotary shaker (180 rpm) at 28 °C. The supernatant was carefully transferred to a new 1.5 mL centrifuge tube to get bacterial suspension. Then, 1 mL of bacterial suspension was added to 9 mL of sterile water to obtain 10−1 bacterial gradient, and followed the same procedure to obtain the 10−7, 10−8 and 10−9 concentration gradients. For each gradient, 200 µL was added into LB solid medium (tryptone 10 g/L, yeast extract 5 g/L, NaCl 5 g/L, agar22 g/L) and cultured at 28 °C for 24 h. Colonies were picked and restreaked until single colonies were obtained. The obtained single colonies were transferred to a nitrogen-free medium (mannitol 10 g/L, KH2PO4 0.2 g/L, MgSO4·7H2O 0.2 g/L, NaCl 0.2 g/L, CaSO4·2H2O 0.2 g/L, CaCO3 5 g/L) to observe their morphology of growth.
The strains were identified by Shanghai Biotechnology Co., Ltd (Shanghai, China) using 16S rRNA sequencing, and Blast homology similarity comparison was performed. Multiple sequence alignments and phylogenetic analysis were further conducted by the Neighbor-joining method using MEGA5.0 software.
Biological pretreatment for lignin degradation
Corn stover samples were air-dried, collected, sieved through a 36-mesh and then dried at 80 °C for 24 h until constant weight. Furthermore, 2 mL of bacterial suspension was added to corn stover solid medium and placed in sealed culture at 28 °C for 7 d. The experiment was carried out at a constant temperature incubator in an airtight environment.
Bacterial pretreatment was carried out in a 250 mL conical flask. Here, 20 mL of inorganic salt medium (KH2PO4 1.0 g/L, NaCl 0.1 g/L, CaCl2 0.1 g/L, MgSO4·7H2O 0.3 g/L, NaNO3 2.5 g/L, FeCl3 0.1 g/L, pH 7.2) and 10 g of corn stover were mixed and autoclaved at 121 °C, 0.1 MPa for 20 min and then cooled to 25 °C. Then, 2 mL of bacterial suspension was inoculated into the corn stover solid medium and cultivated at 28 °C for 7 d. Lignocellulolytic enzyme activity, xylanase activity and CMC-Na enzyme activity were determined daily during biological pretreatment. Each treatment was performed in triplicate.
Enzyme activities assays
The activities of laccase (Lac), peroxidase (Lip) and manganese peroxidase (Mnp) were investigated using ABTS (2,2′-Azinobis-(3-ethylbenzthiazoline-6-sulphonate)), resveratrol, and manganese sulfate as substrates, respectively [Citation15–17]. Xylanase activity was determined referring to the method of Grigorevski-Lima et al. [Citation18], and one unit of xylanase activity was defined as the amount of enzyme which produces 1 µmol of reducing sugar per minute. The enzymatic activity of carboxymethyl cellulose (CMCase) was determined by incubating a reaction mixture containing 1 mL of appropriately diluted enzyme solution with 1 mL of CMC-Na acetate buffer (50 mmol/L, pH 5) at 50 °C for 30 min. One unit of enzyme activity was the amount of enzyme required to produce reducing sugar equivalent to 1 µmol of glucose per hour [Citation15]. The enzyme activity assay was performed independently three times and the data were presented as means values with standard deviation (±SD).
Different methods for reprocessing corn stover after biological treatment
After biodegradation for 7 d with bacteria, corn stover fermentation by-product was collected and dried to a constant weight at 80 °C and divided into four groups. Then, 1% H2SO4 (v/v), 1% NaOH (v/v) and 1% sulfamic acid (v/v) were added to further reprocess corn stover at a solid-liquid ratio of 1:20 and pretreated at 100 °C for 2 h, which was consistent with the reported protocols [Citation19]. Notably, as a single factor, the effect of NaOH concentrations (1%, 1.5%, 2%, 2.5%, 3% (v/v)) was studied [Citation20,Citation21], respectively. The mixture was filtered to collect the solid product and dried at 80 °C until constant weight to facilitate the determination of composition after alkali treatment and enzymatic hydrolysis.
Enzyme hydrolysis experiment
The effect of pretreatment was assessed by the cellulose conversion. All enzymatic hydrolysis were carried out in 40 mL of sodium citrate buffer (50 mmol/L; pH 4.8) with 1.2 g of corn stover treated by a two-stage process. The residues were hydrolyzed with cellulase (30 FPU/g) and β-glucosidase (30 FPU/g) and shaken at 50 °C in a water-bath 140 rpm/min for 72 h. HPLC was used to measure glucose released from the supernatant, then the corresponding cellulose conversion was calculated. The mixture was filtered through a polyether sulfone aqueous syringe (13 mm, 0.2 µm, Fuzhou Aoyan Lab Equipment Co., Ltd., Fuzhou, Fujian, China), and then immediately frozen at −20 °C.
Analysis of the composition of corn stover
The lignin, cellulose, and hemicellulose contents of corn stover were obtained referring to the method reported by Su et al. [Citation19]. The content of sugars after enzymatic hydrolysis was quantified by HPLC, using a column (Agilent Hi-Plex H, 7.7 × 300 mm, 8 µm) equipped with a refractive index detector (Agilent 1100 Series, Agilent Technologies Ltd., USA). The mobile phase was delivered at a flow rate of 0.5 mL/min and the column temperature was set at 50 °C. The percentage conversion of glucose/xylose was calculated according to the formula in Su et al. [Citation19].
Surface structure changes of corn stover
To better understand the degradation of corn stover, different treatments of corn stover residues were collected, freeze-dried and then observed by scanning electron microscopy (SEM) for surface structure changes. XRD and FTIR were conducted to investigate the changes for crystal structure and their related functional groups, respectively.
SEM analysis
Samples with and without treatment were washed with phosphate buffered saline (PBS) and then dehydrated using a freeze dryer. For SEM analysis, these samples were coated with a 10 nm thick gold layer and subsequently observed under a scanning electron microscope (SU8020, Hitachi, Co., Japan) at 20 kV accelerating voltage.
XRD analysis
The crystallinity of corn stover samples was calculated by XRD using a diffractometer (D/MAX2500VL/PC, Rigaku Science Co., Tokyo, Japan), applying Cu-Kα rays, tube voltage 30 Kv, tube current 40 mA, sample test diffraction angle 2θ range 5 ∼ 40°, and scanning speed 5°/min. The crystallinity was calculated according to the following equation [Citation22]:
Where: I002 is the strongest intensity of diffraction peak in the crystalline region around 2θ = 22°; Iam is the lowest diffraction peak intensity in the non-crystalline region around 2θ = 18°.
FTIR analysis
The infrared spectra of lignocellulosic by-products were collected by the KBr press method. A certain amount of treated and untreated corn stover was taken, mixed at a mass ratio of 1:100 between the sample and KBr and then ground to 200 mesh powder and made into thin slices of uniform thickness. Pure KBr was used as background, resolution of 4 cm−1 and spectral range from 4000 to 400 cm−1. For each pixel, 64 scans were acquired by a FTIR spectrometer (Thermo Nicolet, Madison, WI, USA).
Results and discussion
Screening and identification of lignin-degrading bacteria
Azure B plate assay was used to isolate ligninolytic bacteria. In this study, a nitrogen-fixing and lignin-degrading bacteria was isolated successfully, and obvious decolorization changes were recorded on the surface of azure B agar plate (see Supplemental Figure S1), thus preliminarily confirming the lignin peroxidase activities. As shown in , these bacteria are rod-shaped and capable of spreading across semi-solid surfaces with a conjugative pilus. Meanwhile, the growth of the strain R. ornithinolytica RS-1 entered the logarithmic phase and reached the maximum growth rates at about 24 h, followed by a slow growth period after 24 h (). Additionally, using PCR, 1478 bp fragments of this strain were amplified and sequenced. The sequence alignments showed that the strain was highly similar (98%) with R. ornithinolytica and was thus named R. ornithinolytica RS-1 ().
Composition analysis of bio-pretreated corn stover by R. ornithinolytica RS-1
As noted earlier, many studies have reported the lignin-degrading ability of R. ornithinolytica, which has potential for biotechnological applications. However, these findings usually used different carbon sources [Citation23]. In our current study, nitrogen sources were used to screen the lignin-degrading bacteria, followed by a degradation experiment on corn stover.
As shown in , after treatment for 7 d by R. ornithinolytica RS-1, there was 26.35% of biomass loss, which indicated that some components had been degraded during the treatment process. Of these, cellulose and hemicellulose contents increased from 31.52% and 28.68% to 38.27% and 31.89%, respectively. In addition, the lignin content decreased from 19.37% to 15.69%; variation in the chemical composition was visualized after biological treatment (). An ideal pretreatment approach for enzymatic saccharification should maximize lignin removal and minimize polysaccharides modification [Citation24]. Mohanram et al. [Citation25] also found that the relative lignin content decreased from 21% to 16%. These changes facilitated the enzymatic hydrolysis treatment and these results showed that depletion of lignin using R. ornithinolytica fermentation was technically and economically feasible.
Table 1. Changes in the main components of corn stover after bio-pretreatment.
Determination of enzyme activities
As known, the main enzymes associated with lignin degradability are laccase (Lac), lignin peroxidase (Lip) and manganese peroxidase (Mnp). Most previous studies focus on white-rot fungi, which can produce all three enzymes [Citation26]. However, it is a long time to degrade corn stover by white-rot fungi. Therefore, it is important for us to search for an effective bacterial isolate for the high-value comprehensive utilization of corn stover in a short time. In this study, the activities of peroxidase, manganese peroxidase, laccase, xylanase and CMCase of R. ornithinolytica RS-1 were measured during the 7-day fermentation process. As shown in , the variation of lignin degradation activity indicated that peroxidase and manganese peroxidase were mainly responsible for the lignin degradation process. In the first three days of fermentation, there was an increasing trend for peroxidase and manganese peroxidase, with a maximum of 37.473 U/L and 181.0256 U/L, respectively, and then their activity decreased. Compared with peroxidase and manganese peroxidase, the activity of laccase was relatively weak, reaching a maximum of 0.01249 U/L on the third day. In contrast, the enzyme activities of xylanase enzyme and CMCase in showed a decreasing trend in the first three days, then an increasing trend on the fourth day, with a maximum of 0.91249 U/L and 0.0195 U/L, respectively. Based on these findings, we speculated that the lignin enzymes had a certain inhibitory effect on cellulose and hemicellulose enzyme activities in the degradation process.
Figure 3. Changes in enzyme activities during the degradation of corn stover. (a) Lignin-degrading enzyme activity; (b) Cellulase activity. Note: Each value is the mean of three independent replicates.
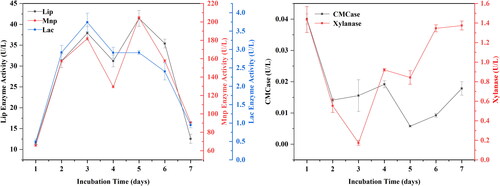
Lip and Mnp are enzymes containing metal ions, and peroxidase. Additionally, Mnp can remove methyl groups to facilitate subsequent degradation and eventual formation of small molecule products [Citation19]. Mei et al. [Citation27] screened Bacillus amyloliquefaciens SL-7, which had a high lignin-degrading enzyme activity. In our current study, we found that all these three enzymes, including peroxidase, manganese peroxidase and laccase had a high activity, which was consistent with previous studies.
Enzymatic saccharification of combined pretreated corn stover
Many previous studies have shown that lignin inhibition is a major barrier against enzymatic hydrolysis, so removing lignin would have a beneficial effect on enzymatic hydrolysis efficiency [Citation28]. For the purpose of studying the efficiency of combined treatments on the enzymatic saccharification of corn stover, the stover was retreated with different acids and alkalis after the bacterial treatment. Here, H2SO4, sulfamic acid and NaOH played an important role in the removal of lignin and hemicellulose. As shown in , in corn stover treated with only 1% H2SO4, 1% NaOH and 1% sulfamic acid the cellulose conversions were 16.76%, 26.39% and 22.74%, respectively. Interestingly, after 72 h of RS-1 digestion, the cellulose conversion values of corn stover pretreated with 1% H2SO4, 1% NaOH and 1% sulfamic acid for 7 d, were 27.44%, 30.99% and 29.59%, which were higher than those of the untreated samples. NaOH was the most effective method to enhance the enzymatic saccharification of corn stover. All these results indicated that R. ornithinolytica RS-1 and NaOH pretreatment result in significant improvement in the efficiency of cellulose conversion, which may be due to its high lignin depletion by bio-pretreatment. Yu et al. [Citation29] showed that the combined pretreatment by hydrogen peroxide and flat mushrooms can increase glucose yield significantly, and this treatment was more effective than biological treatment alone. Wang et al. [Citation30] proved that the enzymatic hydrolysis of poplar was significantly improved to 2.66-fold after pretreatment by L. betulina C5617 compared with that of the untreated group [Citation30]. Similarly, Yu et al [Citation31] found that lignin removal increased the structural pore size of the biomass surface and reduced the ineffective uptake of enzymes. Thus, R. ornithinolytica RS-1 was proposed to be an efficient bacterial strain for improving the enzymatic hydrolysis of lignocellulosic biomass.
Figure 4. Enzymatic effect, cellulose conversion and sugar content of different pretreatments. (a) Cellulose conversion of corn stover treated by different pretreatments; (b) Cellulose conversion of corn stover treated by different concentrations of NaOH; (c) Sugar content of enzyme hydrolysate in different treatments. Cellulose is mainly composed of glucose, while hemicellulose is mainly composed of xylose and a small amount of arabinose. Note: Each value is the mean of three independent replicates.
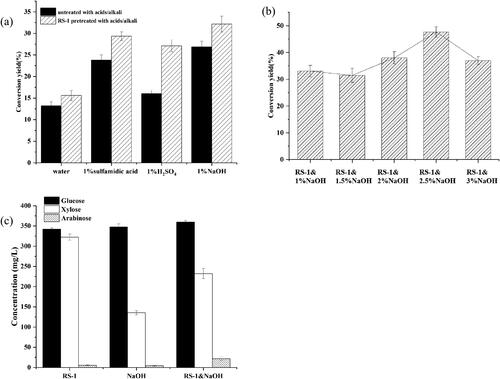
To further enhance the enzymatic saccharification of corn stover, an optimization study was conducted to determine the most appropriate NaOH concentrations. As shown in , the cellulose conversion of the pretreated corn stover increased when the NaOH concentrations increased from 1% to 2.5%, and the cellulose conversion was up to 48.58% when 2.5% NaOH was used. However, the cellulose conversion of corn stover decreased to 37.56% when the concentration was 3%, probably because the high concentration inhibited the subsequent saccharification experiments [Citation32,Citation33]. Therefore, concentration of 2.5% NaOH was the optimal treatment condition. Following solid-liquid separation, the residual streams with NaOH can be either reused to reduce pre-treatment costs or recycled to reduce environmental pollution. From previous studies, it can be understood that in the NaOH treatment of lignocellulosic raw materials such as corn stover, the optimal concentration is mostly in the range of 1% to 10%. Taking into account the recovery of waste liquor and the influence of derivatives produced during the treatment on the subsequent enzymatic effect, etc., a low concentration of 1% to 5% NaOH is used for the treatment, such as Asgher et al. [Citation34]. Niglio et al. [Citation35] used 5% NaOH and ultrasound to pretreat the coffee silver skin (CSS) produced from coffee beans during the roasting process to obtain a higher sugar yield. Zhao et al. [Citation36] established a new and effective NaOH pretreatment process for straw, and then used a Trichoderma reesei and Aspergillus niger mixed culture to produce cellulase; and enzymatic saccharification of pretreated corn stover and rice straw obtained 81.5% and 70.5% yield. The difference from the optimal NaOH concentration used in the above experiments may be due to the different raw materials treated and the different treatment methods.
To further investigate the reasons for the enhanced saccharification of corn stover by the two-step pretreatment process, the contents of glucose, xylose and arabinose in the hydrolysate were determined. Cellulose was mainly composed of glucose, while hemicellulose was mainly composed of a heterogeneous group of sugars, which could reflect the degradation efficiency of the two-step pretreatment. The results in show that the relative contents of glucose, xylose and arabinose after treatment with 2.5% NaOH alone were 347.11, 135.22, 4.13 mg/L, respectively. Interestingly, the contents were 360.74, 230.12, 22.21 mg/L by pretreatment with NaOH and bacteria. Considering the enzyme activity alteration, the bacterial treatment mainly removed lignin, and NaOH further removed lignin and hemicellulose. Furthermore, most of the retained cellulose can effectively improve the saccharification efficiency of corn stover.
NaOH pretreatment can effectively break the linkage between lignin and hemicellulose, thus exposing carbohydrates to enzymes, while alkali pretreatment can effectively improve the delignification of cellulose [Citation37]. Zhao et al. reported that hydrogen peroxide can change the structure of the cell wall to increase the removal of lignin and the digestibility of enzymes [Citation38]. Li et al. also used a two-step pretreatment of dilute hydrochloric acid followed by Fenton oxidation to remove lignin, followed by subsequent enzymatic digestion to obtain glucose, with a relatively high yield 71.3% [Citation39]. Moreover, a two-stage dilute H2SO4 and NaOH pretreatment process was effective to remove lignin, and dextran enzymatic conversion was 98.6% [Citation40]. Both lignin and hemicellulose were removed efficiently by the two-step pretreatment process in our study. In addition, more cellulose would increase the enzymatic saccharification efficiency.
Effect of combined pretreatment on the surface structure of corn stover
The surface structures of corn stover samples with and without biological treatment, NaOH treatment and combined treatment were observed by SEM to visualize the physical changes. shows that the untreated corn stover surface was dense and smooth, fiber bundles were neat and smooth. After biological treatment only or NaOH treatment only, more cracks appeared on the surface and the integrity of the surface was partially destroyed (). As shown in , the changes were more obvious for the two-step pretreatment: the surface had various voids and cracks, and the fiber bundles appeared fractured, rough and cavitated. This indicated that the surface of corn stover changed significantly by the treatment, and that R. ornithinolytica RS-1 can effectively degrade lignocellulose while NaOH treatment mainly broke the chemical bonds. All these results were consistent with the corn stover delignification treatment reported by Sun et al. [Citation9] and Donohoe et al. [Citation41]. Meanwhile, combinations of mild chemical process (2.5% NaOH) along with biological pretreatment can disrupt the corn stover surface to a greater extent, which exposed more cellulose for subsequent enzymatic reactions. Avelino et al. [Citation42] demonstrated that the voids formed in the corn stover after pretreatment made it easier for the enzymes to penetrate, which led to generation of monocaccharides from renewable cellulose with high efficiency of conversion [Citation42].
The structure alteration of functional groups of corn stover
FTIR was performed to determine the pretreatment effectiveness [Citation43]. By focusing on lignin, cellulose and hemicellulose, bands corresponding to 1800–800 cm−1 were detected. As shown in , compared to the untreated group, the absorption peaks at 1630 to 1405 cm−1, which corresponded to the C = O stretching vibration of aromatic rings in lignin, obviously decreased after bacterial or combined pretreatments, indicating the partial dissolution of lignin. The enhanced intensity of the peaks at 1530 cm−1 in bacterial or combined pretreatments showed that the products of lignin degradation increased, which was related to side chains, demethylation and destruction of the aromatic ring skeleton. The band at 1253 cm−1 (the C–O bond of hemicellulose) decreased after NaOH pretreatment or two-step pretreatment, indicating removal of hemicellulose. Other three bands were monitored: 1160 cm−1 (C–O–C stretching vibration in structure of cellulose and hemicellulose), 1110 cm−1 (crystalline cellulose) and 1058 cm−1 (C–O stretching in structure of cellulose and hemicellulose). The peaks in the spectra changed significantly, which means that the bacterial treatment can alter the main structure of the substrates. Importantly, these three peaks were enhanced by R. ornithinolytica RS-1 pretreatment, NaOH pretreatment and two-step pretreatment, which was consistent with the results of Li et al.. Meanwhile, the absorption peak at 867 cm−1 (amorphous cellulose) was significantly increased, indicating that a large amount of lignin was degraded and the cellulose was exposed [Citation44]. This result suggested that cellulose was better retained, and the two-stage pretreatment process can enhance the enzymatic saccharification of the pretreated corn stover.
Effect of combined pretreatment on the crystal structure of corn stover
Crystallinity is an important structural parameter that can influence the enzymatic hydrolysis of corn stover [Citation45]. Here, the crystal structure of pretreated and unpretreated corn stover were measured by XRD. As shown in , the general trend was very similar while the position of diffraction peak 2θ = 22.2 remained the same, indicating that these three treatments only changed the crystal intensity, accompanied by a small degree of crystallization reorientation [Citation38]. According to the method of Segal [Citation46], the relative crystallinity of corn stover was 17.69%, 14.83%, 21.58% and 6.57% for the group without pretreatment, the groups with R. ornithinolytica RS-1 pretreatment, NaOH pretreatment and two-step pretreatment, respectively. The reduced crystallinity following treatment by R. ornithinolytica RS-1 was mainly due to the ability of the secreted enzymes to degrade the lignin and some hemicellulose of corn stover. These treatments resulted in a decrease in crystallinity, which was consistent with Su et al. [Citation47]. Yet, the increased crystallinity of NaOH pretreatment can increase the content of cellulose [Citation48]. Notably, the crystallinity of corn stover treated by bacteria combined with NaOH decreased significantly probably because the bacterial treatment mainly removed lignin, and NaOH substantially removed lignin and hemicellulose, which reduced the degree of polymerization and crystallinity [Citation36]. Lu et al. [Citation49] reported that the reason for the decrease in substrate crystallinity after NaOH/urea pretreatment was that NaOH can be adsorbed around the cellulose by forming hydrogen bonds, which can destroy cellulose crystallization.
Conclusions
In this study, R. ornithinolytica RS-1 was screened and was demonstrated to be highly effective in reducing the lignin content in during pretreatment of corn stover. It is an effective lignocellulose degrader of corn stover by producing high-activity-level manganese peroxidase and lignin peroxidase enzymes. Compared with pretreatment only by R. ornithinolytica RS-1 and different concentrations of NaOH (1%, 1.5%, 2%, 2.5%, 3%), R. ornithinolytica RS-1 with 2.5% NaOH combined pretreatment produced a better cellulose conversion of 48.58%. SEM, FTIR and XRD analysis further indicated that the two-step pretreatment led to a high sugar yield. This research provided an effective pathway for degradation of lignocellulose biomass and enzymatic saccharification.
Supplemental Material
Download MS Word (369.2 KB)Acknowledgments
This work was supported by the National Science Foundation Project of Anhui (1908085MC63) and National Key R&D Program of China (No. JZ2018ZDYF0541).
Disclosure statement
No potential conflict of interest was reported by the author.
Data availability statement
The datasets used in this study are available from the corresponding author on reasonable request.
Additional information
Funding
References
- Shen XJ, Sun RC. Recent advances in lignocellulose prior-fractionation for biomaterials, biochemicals, and bioenergy. Carbohydr Polym. 2021;261:117884.
- Upton BM, Kasko AM. Strategies for the conversion of lignin to high-value polymeric materials: review and perspective. Chem Rev. 2016;116(4):2275–2306.
- Zhang ZR, Song JL, Han BX. Catalytic transformation of lignocellulose into chemicals and fuel products in ionic liquids. Chem Rev. 2017;117(10):6834–6880.
- Ennaert T, Van Aelst J, Dijkmans J, et al. Potential and challenges of zeolite chemistry in the catalytic conversion of biomass. Chem Soc Rev. 2016;45(3):584–611.
- Rinaldi R, Jastrzebski R, Clough MT, et al. Paving the way for lignin valorisation: recent advances in bioengineering, biorefining and catalysis. Angew Chem Int Ed Engl. 2016;55(29):8164–8215.
- Straathof AJJ. Transformation of biomass into commodity chemicals using enzymes or cells. Chem Rev. 2014;114(3):1871–1908.
- Lynd LR, Elamder RT, Wyman CE. Likely features and costs of mature biomass ethanol technology. Appl Biochem Biotechnol. 1996;57-58(1):741–761.
- Wang ZC, He XJ, Yan LM, et al. Enhancing enzymatic hydrolysis of corn stover by twin-screw extrusion pretreatment. Ind Crops Prod. 2020;143:111960.
- Sun FH, Li J, Yuan YX, et al. Effect of biological pretreatment with Trametes hirsuta yj9 on enzymatic hydrolysis of corn stover. Int Biodeter Biodegrad. 2011;65:934–938.
- Yang L, Ru Y, Xu S, et al. Features correlated to improved enzymatic digestibility of corn stover subjected to alkaline hydrogen peroxide pretreatment. Bioresour Technol. 2021;325:124688.
- Sanchez C. Lignocellulosic residues: biodegradation and bioconversion by fungi. Biotechnol Adv. 2009;27:185–194.
- Dar MA, Shaikh AA, Pawar KD, et al. Exploring the gut of Helicoverpa armigera for cellulose degrading bacteria and evaluation of a potential strain for lignocellulosic biomass deconstruction. Process Biochem. 2018;73:142–153.
- Mishra V, Jana AK, Jana MM, et al. Enhancement in multiple lignolytic enzymes production for optimized lignin degradation and selectivity in fungal pretreatment of sweet sorghum bagasse. Bioresour Technol. 2017;236:49–59.
- Xu C, Su X, Wang J, et al. Characteristics and functional bacteria in a microbial consortium for rice straw lignin-degrading. Bioresour Technol. 2021;331:125066.
- Kumar S, Dheeran P, Singh SP, et al. Kinetic studies of two-stage sulphuric acid hydrolysis of sugarcane bagasse. Renew Energ. 2015;83:850–858.
- Pinto PA, Dias AA, Fraga I, et al. Influence of ligninolytic enzymes on straw saccharification during fungal pretreatment. Bioresour Technol. 2012;111:261–267.
- Chen Y, Wang YY, Xu Z, et al. Enhanced humification of maize straw and canola residue during composting by inoculating phanerochaete chrysosporium in the cooling period. Bioresour Technol. 2019;293:122075.
- Grigorevski-Lima AL, de Oliveira MMQ, do Nascimento RP, et al. Production and partial characterization of cellulases and xylanases from Trichoderma atroviride 676 using lignocellulosic residual biomass. Appl Biochem Biotechnol. 2013;169(4):1373–1385.
- Su YJ, Yu XX, Sun Y, et al. An efficient strategy for enhancing enzymatic saccharification with delignified fungus Myrothecium verrucaria and solid acid. Ind Crops Prod. 2018;121:396–404.
- Wang Q-F, Niu L-L, Jiao J, et al. Degradation of lignin birch sawdust treated by a novel Myrothecium verrucaria coupled with ultrasound assistance. Bioresour Technol. 2017;244(Pt 1):969–974.
- Wang X, Wang G, Yu X, et al. Pretreatment of corn stover by solid acid for D-lactic acid fermentation. Bioresour Technol. 2017;239:490–495.
- Agarwal UP, Ralph SA, Baez C, et al. Effect of sample moisture content on XRD-estimated cellulose crystallinity index and crystallite size. Cellulose. 2017;24(5):1971–1984.
- Li F, Xie RR, Liang N, et al. Biodegradation of lignin via Pseudocitrobacter anthropi MP-4 isolated from the gut of wood-feeding termite Microtermes pakistanicus (Isoptera: Termitidae). BioRes. 2019;14(1):1992–2012.
- Asgher M, Ahmad Z, Iqbal HMN. Alkali and enzymatic delignification of sugarcane bagasse to expose cellulose polymers for saccharification and bio-ethanol production. Ind Crops Prod. 2013;44:488–495.
- Ding DY, Zhou X, Ji Z, et al. How does plant cell wall nanoscale architecture correlate with enzymatic digestibility? Science. 2016;338:415–431.
- Mohanram S, Rajan K, Carrier DJ, et al. Insights into biological delignification of rice straw by Trametes hirsuta and Myrothecium roridum and comparison of saccharification yields with dilute acid pretreatment. Biomass Bioenergy. 2015;76:54–60.
- Manavalan T, Manavalan A, Heese K. Characterization of lignocellulolytic enzymes from white-rot fungi. Curr Microbiol. 2015;70(4):485–498.
- Mei JF, Shen XB, Gang LP, et al. A novel lignin degradation bacteria-Bacillus amyloliquefaciens SL-7 used to degrade straw lignin efficiently. Bioresour Technol. 2020;310:123445.
- Zhu L, O’Dwyer JP, Chang VS, et al. Structural features affecting biomass enzymatic digestibility. Bioresour Technol. 2008;99(9):3817–3828.
- Yu J, Zhang JB, He J, et al. Combinations of mild physical or chemical pretreatment with biological pretreatment for enzymatic hydrolysis of rice hull. Bioresour Technol. 2009;100(2):903–908.
- Wang W, Yuan TQ, Wang K, et al. Combination of biological pretreatment with liquid hot water pretreatment to enhance enzymatic hydrolysis of Populus tomentosa. Bioresour Technol. 2012;107:282–286.
- Yu HB, Guo GN, Zhang XY, et al. The effect of biological pretreatment with the selective white-rot fungus Echinodontium taxodii on enzymatic hydrolysis of softwoods and hardwoods. Bioresour Technol. 2009;100(21):5170–5175.
- Kumar G, Bakonyi P, Periyasamy S, et al. Lignocellulose biohydrogen: practical challenges and recent progress. Renew Sust Energ Rev. 2015;44:728–737.
- Kumar S, Sharma HK, Sarkar BC. Effect of substrate and fermentation conditions on pectinase and cellulase production by Aspergillus niger NCIM 548 in submerged (SmF) and solid-state fermentation (SSF). Food Sci Biotechnol. 2011;20(5):1289–1298.
- Niglio S, Procentese A, Russo ME, et al. Combined pretreatments of coffee silver skin to enhance fermentable sugar yield. Biomass Conv Bioref. 2020;10(4):1237–1249.
- Zhao C, Shao QJ, Ma ZQ, et al. Physical and chemical characterizations of corn stalk resulting from hydrogen peroxide presoaking prior to ammonia fiber expansion pretreatment. Ind Crops Prod. 2016;83:86–93.
- Huang CX, He J, Wang Y, et al. Associating cooking additives with sodium hydroxide to pretreat bamboo residues for improving the enzymatic saccharification and monosaccharides production. Bioresour Technol. 2015;193:142–149.
- Zhao C, Zou ZS, Li JS, et al. Efficient bioethanol production from sodium hydroxide pretreated corn stover and rice straw in the context of on-site cellulase production. Renew Energy. 2018;118:14–24.
- Li WZ, Liu QY, Ma QZ, et al. A two-stage pretreatment process using dilute hydrochloric acid followed by fenton oxidation to improve sugar recovery from corn stover. Bioresour Technol. 2016;219:753–756.
- Li K, Wan JM, Wang X, et al. Comparison of dilute acid and alkali pretreatments in production of fermentable sugars from bamboo: effect of tween 80. Ind Crops Prod. 2016;83:414–422.
- Donohoe BS, Vinzant TB, Elander RT, et al. Surface and ultrastructural characterization of raw and pretreated switchgrass. Bioresour Technol. 2011;102(24):11097–11104.
- Goncalves FA, Ruiz HA, Nogueira CD, et al. Comparison of delignified coconuts waste and cactus for fuel-ethanol production by the simultaneous and semi-simultaneous saccharification and fermentation strategies. Fuel. 2014;131:66–76.
- Karimi K, Taherzadeh MJ. A critical review of analytical methods in pretreatment of lignocelluloses: composition, imaging, and crystallinity. Bioresour Technol. 2016;200:1008–1018.
- Mou HY, Li B, Fardim P. Pretreatment of corn stover with the modified hydrotropic method to enhance enzymatic hydrolysis. Energy Fuels. 2014;28(7):4288–4293.
- Van Dyk JS, Pletschke BI. A review of lignocellulose bioconversion using enzymatic hydrolysis and synergistic cooperation between enzymes-factors affecting enzymes, conversion and synergy. Biotechnol Adv. 2012;30(6):1458–1480.
- Nam S, French AD, Condon BD, et al. Segal crystallinity index revisited by the simulation of X-ray diffraction patterns of cotton cellulose I beta and cellulose II. Carbohydr Polym. 2016;135:1–9.
- Sun QN, Khunsupat R, Akato K, et al. A study of poplar organosolv lignin after melt rheology treatment as carbon fiber precursors. Green Chem. 2016;18:5051–5024.
- Heinigen AV, Tunc MS, Gao Y, et al. Relationship between alkaline pulp yield and the mass fraction and degree of polymerization of cellulose in pulp. J Pulp Paper Sci. 2004;30(8):211–217.
- Lu A, Liu YT, Zhang LN, et al. Investigation on metastable solution of cellulose dissolved in NaOH/urea aqueous system at low temperature. J Phys Chem B. 2011;115(44):12801–12808.