ABSTRACT
The effect of high-pressure carbon dioxide processing on inactivation of aerobic mesophilic bacteria and Escherichia coli ATCC 25922 inoculated in human milk was investigated. The effect of the ratio between sample mass and CO2 (1:0.2; 1:0.6 and 1:1 m/m); depressurization rate (1, 5.5 and 10 MPa/min); and pressure cycling (1, 3 and 5) were the process variables studied. The best reductions in aerobic mesophilic bacteria as well as in E. coli (>6.0 and >5.0 log, respectively) were obtained with a ratio of 1:1, a depressurization rate of 10 MPa/min, and one cycle of pressurization/depressurization. The depressurization rate was found to be an important variable in the inactivation process. The results suggest that high-pressure carbon dioxide processing can be applied to human milk as a safe alternative to the pasteurization employed in human milk banks.
RESUMEN
El presente estudio se orientó a investigar el efecto de la utilización de dióxido de carbono de alta presión en la inactivación de bacterias aerobias mesófilas y Escherichia coli ATCC 25922 previamente inoculadas en leche humana. Durante el proceso se analizaron las siguientes variables: ratio entre la masa de muestra y el CO2 (1:0,2; 1:0,6 y 1:1 m/m); tasa de despresurización (1, 5,5 y 10 MPa/min) y ciclo de presión (1, 3 y 5). La disminución más pronunciada de bacterias aerobias mesófilas y de E. coli (>6,0 y >5,0 log, respectivamente) se logró empleando una ratio de 1:1, una tasa de despresurización de 10 MPa/min y un solo ciclo de presurización/despresurización. Se comprobó que la tasa de despresurización constituye una variable importante para el proceso de inactivación. En este sentido, los resultados permiten constatar que puede aplicarse dióxido de carbono de alta presión a la leche materna como alternativa segura a la pasteurización utilizada en los bancos de leche humana.
1. Introduction
It has been well established that human milk provides all the nutrients and immune factors necessary for the growth and development of newborns and babies (Ballard & Morrow, Citation2013; Souza, Delgadillo, & Saraiva, Citation2016). In normal situations, shortly after birth, newborns are fed directly from their mother`s breasts. However, many infants are fed with milk from human milk banks (HMBs) because sometimes preterm newborns do not have enough strength to suckle. In addition, some mothers show physiological and/or emotional problems, resulting in the reduction of milk production (Serafini et al., Citation2003). In such cases babies are fed by milk donated by women who lactate in excess. In order to meet infants’ needs, the donated milk must have microbiological, chemical, nutritional, immunologic, sensory and structural quality assured (Almeida, Citation2008).
In order to ensure the quality of human milk, the pasteurization process is used to eliminate the contaminating microorganisms, such as Staphylococcus spp., Streptococcus spp., yeasts and molds, and Enterobacteriaceae (Serafini et al., Citation2003). Masiello, Martin, Trmčić, Wiedmann, and Boo (Citation2016) reported that the presence of coliform bacteria in pasteurized human milk mostly indicated contamination after pasteurization or inadequate pasteurization.
Fecal coliform bacteria detection is sequential and dependent on positive results for total coliforms, thus indicating with greater probability the presence of enteric pathogens. Among the microorganisms isolated in the fecal coliform test, Escherichia coli is the one that is most frequently detected, thus becoming the classic indicator of the possible presence of enteric pathogens in food, particularly in human milk (Novak & Almeida, Citation2002)
Novak, Almeida, Asensi, Moraes, and Rodrigues (Citation2001) reported that infections that occur during the first year of life constitute one of the most important causes of elevated morbidity and mortality rates among infants. The frequency and severity of infections caused by E. coli are influenced by the immaturity of the immune system observed for this age group. Therefore, human milk is required to undergo a strict quality control process conducted throughout the collection, processing and distribution of the product in order to ensure its safety and quality (BRAZIL, Citation2008). However, De Oliveira et al. (Citation2016) state that the low-temperature long-time pasteurization (62.5°C /30 min), usually applied in HMBs, modifies biological quality and microstructure of the human milk.
The high-pressure carbon dioxide (CO2) processing is an emerging technology and has been studied as a promising alternative for the reduction of microorganisms in liquid whole egg (Garcia-Gonzalez et al., Citation2009), hami melon juice (Chen et al., Citation2010), milk (Ceni et al., Citation2016), apple juice (Porebska, Sokołowska, Skapska, & Rzoska, Citation2017) and mozzarella-type cheese (Sikin, Walkling-Ribeiro, & Risvi, Citation2016). CO2 is a low-cost inert, non-toxic and non-flammable gas, and it has high density, low viscosity, it is available having high purity, it does not generate waste, it does not create any environmental problems, and also it can be used at temperatures below 50°C (Choi, Bae, Kim, Kim, & Rhee, Citation2009; Zhang et al., Citation2006). To our knowledge, the use of this technology to ensure the safety and microbiological quality of human milk has not been reported in the literature. In this study, the impact of high-pressure carbon dioxide processing on the microbial inactivation of aerobic mesophilic bacteria and E. coli ATCC 25922 inoculated in human milk was determined. Also, the effects of process variables, such as the ratio of CO2 to sample mass ratio, depressurization rate and the number of compression/decompression cycles, were investigated.
2. Experimental
2.1. Selection of voluntary lactating donors
Eight human milk donors, aged 21–33 years, were invited to participate in the study when they sought the CIAM (Center for Breastfeeding Encouragement) at the Maternity Ward of the University Hospital at Federal University of Santa Catarina (UFSC, Florianópolis, Brazil) to relieve breast engorgement. The volunteers received clarification on the purpose of the research and signed an informed written consent. The research project was submitted to the Ethics Committee for Research with Human Beings at UFSC. It was approved on 11 January 2016 and was registered under number 1.386.799.
2.2. Samples
The human milk samples were collected manually into sterilized glass jars, following the hygiene guidelines outlined in the Operating Manual for HMB (BRAZIL, Citation2008) and subsequently grouped to create a pool of human milk. After collection, the samples were immediately frozen and stored in a freezer at −20°C until the time of the analyses, which were conducted within a maximum period of 15 days. For the processing procedures and the analysis of the human milk, the transportation of the samples to the laboratories was made in coolers containing recyclable ice.
2.3. Inoculum preparation
A surrogate E. coli ATCC 25922 stock culture was maintained on Brain–Heart Infusion (Acumedia, Lansing, Michigan, USA) slants at 4°C. The culture for experiment was subcultured twice in Nutrient broth (Oxoid, Basingstoke, Hampshire, England) incubated at 35°C for 18 h until reach the concentration of 108 CFU/mL.
2.4. Contamination of the milk samples
The thawed human milk samples were vigorously mixed and divided into two portions. The first portion (10 mL) was inoculated at a concentration of 8 log CFU/mL of E. coli culture, which reaches about 6 log CFU/mL of E. coli in the human milk samples. The second portion (control sample) was not contaminated, and it was used for calculating the difference of the artificial contamination compared to the natural contamination of the milk.
2.5. Microbiological analyses
In order to confirm the concentration of the inoculum, decimal dilutions of the E.coli suspension were carried out and then were plated in Petrifilm® EC (E. coli/Coliform Count Plate) and incubated at 35°C for 48 h. The determination of aerobic mesophilic bacteria and E. coli was performed in raw human milk with no inoculation (control sample), in raw human milk inoculated with E. coli and after each inactivation processes using Petrifilm® plates AC (Aerobic Count Plate) and EC, according to Association of Official Analytical Chemists International (Citation2002). Replicate counts were expressed as averaged log CFU/mL and the reductions after process as log orders CFU/mL.
2.6. Experimental design and apparatus
shows the central composite design used to evaluate the effects of the ratio between sample mass and CO2 (1:0.2; 1:0.6 and 1:1 m/m), pressure cycling (1, 3 and 5) and depressurization rate (1, 5.5 and 10 MPa/min) on the total count of viable aerobic mesophilic bacteria and E. coli. The initial working pressure was 8 MPa, at this point the system was kept for a short period to allow stabilization of the system, and then the pressure was increased to 20 MPa (rate of 10 MPa/min), and kept constant up to experiment completion. After the procedure, the system pressure was reduced to 8 MPa at the rate of depressurization pre-established by the experimental design, which also established the number of pressure cycles of each experiment. At the end of this process, the pressure was manually reduced from 8 MPa to atmosphere pressure. For all experiments, the time was set at 120 min and the temperature maintained at 33°C.
Table 1. Factors and their respective levels of the experimental design.
Tabla 1. Factores y sus respectivos niveles del diseño experimental.
A static-synthetic method was used in a variable-volume high-pressure cell to perform the inactivation experiments. This method was previously described by Soares, Lerin, Cansian, Oliveira, and Mazutti (Citation2013). shows an overall view of the apparatus which was used. The experimental set-up, briefly, consisted of: a variable-volume view cell (maximum internal volume = 27 mL); two sapphire windows for visual observation; an absolute pressure transducer (Smar LD 301), with a precision around 0.03 MPa; a portable programmer (Smar, HT 201) for the pressure data acquisition; and a syringe pump (ISCO 260D). The inactivation cell contained a movable piston, which allowed the control of the pressure inside the cell (,)). The cell was surrounded by a metal jacket, and the temperature was maintained using a thermostatic bath, with water as the heating/cooling fluid. At the beginning of the experiment, the inactivation cell was loaded with a certain amount of sample mass (human milk) by means of a sterile syringe at atmosphere pressure. A syringe pump, with a resulting precision of 0.005 g in CO2 loads, helped to load CO2.
Figure 1. Experimental apparatus for human milk processing with supercritical carbon dioxide.
Figura 1. Aparato experimental para el procesamiento de la leche humana con dióxido de carbono supercrítico.
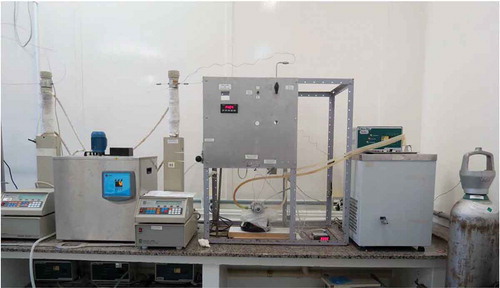
Figure 2. (a) Variable-volume equilibrium cell of the experimental apparatus for human milk processing with supercritical carbon dioxide and (b) a front view of the cell.
Figura 2. (a) Célula de equilibrio de volumen variable del aparato experimental para el procesamiento de la leche humana con dióxido de carbono supercrítico (b) una vista frontal de la célula.
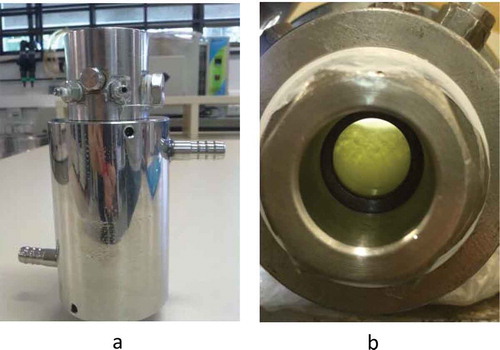
2.7. Statistical analysis
All the results were analyzed using Statistica® 12.0 (Statsoft Inc., Tulsa, OK, USA) considering a significance level of 95% (p < 0.05). Pareto chart was used to better evaluate the effects of each factor, considering a significance level of 95% (p < 0.05).
3. Results and discussion
shows the total count of viable aerobic mesophilic bacteria in human milk with no inoculation (control sample), total count of viable aerobic mesophilic bacteria and E. coli after inoculation, and after high-pressure carbon dioxide processing using different process conditions. In the raw human milk no E. coli was detected (data not shown). The initial aerobic mesophilic bacteria count was about 4 log CFU/mL in human milk and about 7 log CFU/mL in milk inoculated with E. coli. It can be observed a decrease in both aerobic mesophilic bacteria and E. coli after applying the pressurized carbon dioxide using different process conditions.
Table 2. Viable aerobic mesophilic bacteria (AMB) and E. coli in human milk before and after high-pressure carbon dioxide processing using different process conditions.
Tabla 2. Bacterias aerobias mesófilas (AMB) viables y E. Coli presentes en la leche materna antes y después de la aplicación de dióxido de carbono de alta presión bajo condiciones de procesamiento distintas.
The best results were obtained in experiments 5, 6, 7 and 8, with log reductions ranging from 3.6 to >6.0 in relation to mesophilic bacteria, and from 3.7 to >5.0 in relation to E. coli (). The greatest aerobic mesophilic bacteria and E. coli reductions were observed for the depressurization rate of 10 MPa/min, thus suggesting that an increase in depressurization rate favors the inactivation of E. coli in human milk.
Experiments 7 and 8 () showed that the number of cycles had a positive effect only when associated with a higher depressurization rate, for both aerobic mesophilic bacteria and E. coli. Silva et al. (Citation2013) using supercritical carbon dioxide reported that the depressurization rate caused a positive effect on inactivation of E. coli in broth and the number of cycles was an important factor in the inactivation. However, the number of cycles was associated with a longer time of experiment (five cycles, depressurization rate = 11 MPa/min, 120 min), leading to a complete inactivation in exposure times of over 120 min. On the other hand, higher depressurization rates were associated with short time experiments (one cycle, depressurization rate = 11 MPa/min, 20 min and 30 min). In the present study, besides different exposure times were not performed (the experimental time was adjusted to 120 min), our results indicated that the depressurization rate exerts a more positive effect on microbial inactivation than the number of cycles when compared with the results obtained by Silva et al. (Citation2013).
and show the effects of the processing variables on Pareto chart. This is a simple and practical tool, widely used in statistical analysis, to provide information about the effects of main and cross interaction (variables), whether they are positive or negative, as well as a magnitude of the effect. This tool can be obtained directly from the statistical treatment of experimental data of any available commercial software. Given a level of confidence of 95% (p < 0.05), it was possible to observe that the depressurization rate was a significant variable (p < 0.15) in the inactivation of both aerobic mesophilic bacteria and E. coli, while the number of cycles and ratio between milk and CO2 had no significant effect on inactivation of both aerobic mesophilic bacteria and E. coli. The positive effects of a higher depressurization rate were similar when five depressurization cycles were applied, as can be noted in experiments 7 and 8 compared to experiments 3 and 4, for both aerobic mesophilic bacteria and E. coli, as observed in . The best inactivation rates were obtained in experiments 5 and 6 (5.5 and >6.0 for aerobic mesophilic bacteria, respectively; and 4.8 and >5.0 for E. coli, respectively), where higher depressurization rates (10 MPa/min) were used regardless of the amount of CO2. The depressurization causes a sudden expansion of CO2, which has been solubilized within the microbial cells, leading to their rupture and consequent inactivation of microorganisms (Karaman & Erkmen, Citation2001).
Figure 3. Pareto chart showing the effects of independent variables on aerobic mesophilic bacteria reduction after high-pressure carbon dioxide processing obtained in the central composite design (CCD).
Figura 3. Diagrama de Pareto que muestra los efectos de las variables independientes sobre la reducción de las bacterias aerobias mesofilas después del procesamiento de dióxido de carbono a alta presión obtenida en el diseño compuesto central (DCC).
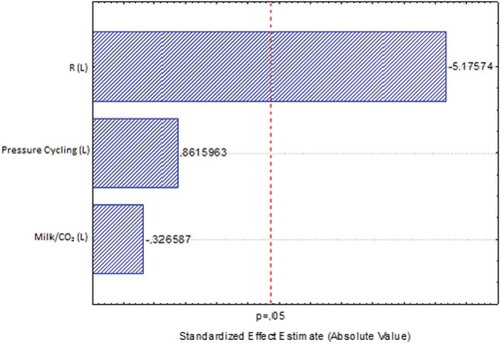
Figure 4. Pareto chart showing the effects of independent variables on microbial survivals after high-pressure carbon dioxide processing of E. coli obtained in the central composite design (CCD).
Figura 4. Diagrama de Pareto que muestra los efectos de las variables independientes sobre la supervivencia microbiana después del procesamiento de dióxido de carbono a alta presión de E. coli obtenido en el diseño compuesto central (DCC).
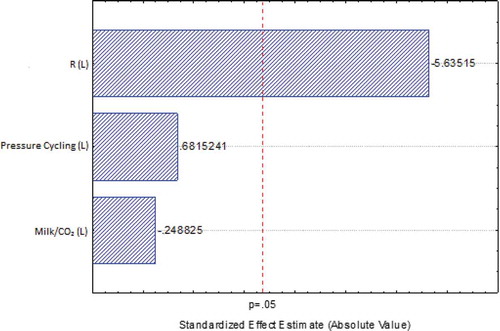
Soares et al. (Citation2013) reported a higher inactivation rate of a culture of Listeria monocytogenes using a depressurization rate of 10 MPa/min while Matos (Citation2013) reported the inactivation of aerobic mesophilic bacteria, Vibrio spp. and Vibrio parahaemolyticus in oysters using one processing cycle, small amounts of CO2 (1:0.2) and a low depressurization rate (1 MPa/min). However, when the amount of CO2 was higher (1:0.8), the highest inactivation rate occurred at the highest depressurization rate (10 MPa/min).
Erkmen (Citation1997) achieved total inactivation of aerobic bacteria and Staphylococcus aureus artificially inoculated in whole cow’s milk treated at 14.6 MPa for 5 h, and in skimmed milk treated at 9 MPa for 2 h, both at 25°C. The same author evaluated the inactivation of E. coli in whole and in skimmed milk, with a reduction of 6.42 and 7.24 log, respectively. In both studies, the milk fat may have increased the resistance to the penetration of CO2 into the microbial cells. It may also have influenced on the microbial inactivation in the present study since the fat content in human milk ranges between 3.2 and 3.6 g/mL (Ballard & Morrow, Citation2013).
Werner and Hotchkiss (Citation2006) evaluated the effects of subcritical and supercritical CO2 in a continuous flow system on the reduction of naturally occurring psychrotrophic microorganisms and of bacterial spores and Pseudomonas fluorescens that were artificially inoculated in skimmed milk. These authors noted a higher mortality rate when the milk was treated at supercritical CO2 conditions. For the total microbial population, maximum reductions of 5.36 and 5.02 log were noted for P. fluorescens, at 35°C and 20.7 MPa, and an average reduction of 3.81 and 2.93 log, respectively, at 30°C, with pressures between 10.3 and 20.7 MPa. Liao, Kui, Xiaojun, and Xiaosong (Citation2014) evaluated the inactivation of microorganisms naturally present in raw cow’s milk and obtained a maximum decrease of 4.96 log in aerobic bacteria after a period of 70 min of exposure, at 25 MPa and 50°C. Yeast and mold were completely inactivated after 70 min of exposure, at 40°C and 25 MPa, or 50 min at 50°C and 25 MPa. The coliform bacteria were completely inactivated by the treatments at 25 MPa for 20, 30, 50 and 70 min at 50, 40, 30 and 20°C, respectively.
In this study, a significant decrease in the microbial count of aerobic mesophilic bacteria and of E. coli was observed (). Ceni et al. (Citation2016) reported a sharp decrease in the microbial count when a residence time of more than 20 min was applied. The greater microbial reduction obtained was a result of high temperature, pressure and exposure time applied. Besides, the amount of pressurized gas, in turns, implies in an increased interaction with the microorganisms, thus being able to promote the inactivation of the existing microbiota (Matos, Citation2013). Although different parameters were used to evaluate the inactivation of the same microorganism in the present study, it is possible to suggest that the time of treatment (maintained at 120 min) and the pressure (20 MPa) that were applied have a positive effect on microbial inactivation.
4. Conclusions
The best reductions in the counts of both aerobic mesophilic bacteria and E. coli were obtained when the following parameters were used: carbon dioxide: sample mass ratio of 1:1, depressurization rate of 10 MPa/min and one pressurization/depressurization cycle. Results obtained in this work indicated that high-pressure carbon dioxide processing is a promising alternative for replacing the current human milk pasteurization process using low temperatures. The depressurization rate was an important variable in the inactivation of E. coli in human milk. Further studies on high pressure carbon dioxide processing should be conducted towards assessing the effects of even faster depressurization rates and also kinetic inactivation profile on the natural contamination of human milk as well as on its nutrients, to ensure their safety and quality.
Disclosure statement
No potential conflict of interest was reported by the authors.
References
- Almeida, S.D.S. (2008). Somatic cell count, total bacterial and human milk composition ( Master thesis). Retrieved from https://ppgca.evz.ufg.br/up/67/o/Dissertacao2008_Suzy_Darlen.pdf
- Association Official Analytical Chemists International. (2002). Official methods of analysis (17th ed.). Washington, DC: Author.
- Ballard, O., & Morrow, A.L. (2013). Human milk composition: Nutrients and bioactive factors. Pediatric Clinics of North America, 60, 49–74. doi:10.1016/j.pcl.2012.10.002
- BRAZIL. (2008). National Health Surveillance Agency [Brazil], Human milk bank: Operation, prevention and control of risks (pp. 160). Brasília.
- Ceni, G., Silva, M.F., Valério, C., Jr, Cansiana, R.L., Oliveira, J.V., Rosa, C.D., & Mazuti, M.A. (2016). Continuous inactivation of phosphatase and Escherichia coli in milk using compressed carbon dioxide as inactivating agent. Journal of CO2 Utilization, 13, 24–28. doi:10.1016/j.jcou.2015.11.003
- Chen, J.I., Zhang, J., Song, L., Jiang, Y., Wu, J., & Hu, X.S. (2010). Changes in microorganism, enzyme, aroma of hami melon (Cucumis melo L.) juice treated with dense phase carbon dioxide and stored at 4°C. Innovative Food Science & Emerging Technologies, 11, 623–629. doi:10.1016/j.ifset.2010.05.008
- Choi, Y.M., Bae, Y.Y., Kim, K.H., Kim, B.C., & Rhee, M.S. (2009). Effects of supercritical carbon dioxide treatment against generic Escherichia coli, Listeria monocytogenes, Salmonella typhimurium, and E. coli O157: H7in marinades and marinated pork. Meat Science, 82, 419–424. doi:10.1016/j.meatsci.2009.02.016
- de Oliveira, S.C., Bourlieu, C., Ménard, O., Bellanger, A., Henry, G., Rousseau, F., … Deglaire, A. (2016). Impact of pasteurization of human milk on preterm newborn in vitro digestion: Gastrointestinal disintegration, lipolysis and proteolysis. Food Chemistry, 211, 171–179. doi:10.1016/j.foodchem.2016.05.028
- Erkmen, O. (1997). Antimicrobial effect of pressurized carbon dioxide on Staphylococcus aureus in broth and milk. {LWT} - Food Science and Technology, 30, 826–829. doi:10.1006/fstl.1997.0277
- Garcia-Gonzalez, L., Geeraerd, A.H., Elst, K., Van Ginneken, L., Van Impe, J.F., & Devlieghere, F. (2009). Inactivation of naturally occurring microorganisms in liquid whole egg using high pressure carbon dioxide processing as an alternative to heat pasteurization. The Journal of Supercritical Fluids, 51, 74–82. doi:10.1016/j.supflu.2009.06.020
- Karaman, H., & Erkmen, O. (2001). High carbon dioxide pressure inactivation kinetics of Escherichia coli in broth. Food Microbiology, 18, 11–16. doi:10.1006/fmic.2000.0371
- Liao, H., Kui, Z., Xiaojun, L., & Xiaosong, H. (2014). Inactivation of microorganisms naturally present in raw bovine milk by high-pressure carbon dioxide. International Journal of Food Science and Technology, 49, 696–702. doi:10.1111/ijfs.12352
- Masiello, S.N., Martin, N.H., Trmčić, A., Wiedmann, M., & Boo, K.J. (2016). Identification and characterization of psychrotolerant coliform bacteria isolated from pasteurized fluid milk. Journal of Dairy Science, 99, 130–140. doi:10.3168/jds.2015-9728
- Matos, K.H.O. (2013). Inativação microbiana em ostras (crassostrea gigas) empregando dióxido de carbono supercrítico ( Doctoral dissertation). Retrieved form https://repositorio.ufsc.br/xmlui/handle/123456789/106994
- Novak, F.R., & Almeida, J.A.G. (2002). Teste alternativo para detecção de coliformes em leite humano ordenhado. Jornal De Pediatria, 78, 193–196. doi:10.1590/S0021-75572002000300005
- Novak, F.R., Almeida, J.A.G., Asensi, M.A., Moraes, B.A., & Rodrigues, D.P. (2001). Resistência antimicrobiana de coliformes isoladas de leite humano ordenhado. Cadernos De Saúde Pública, 17, 713–717. doi:10.1590/S0102-311X2001000300026
- Porebska, I., Sokołowska, B., Skapska, S., & Rzoska, S.J. (2017). Treatment with hydrostatic pressure and supercritical carbon dioxide to control Alicyclobacillus acidoterrestris spores in apple juice. Food Control, 73, 24–30. doi:10.1016/j.foodcont.2016.06.005
- Serafini, A.B., André, M.C.D.P.B., Rodrigues, M.A.V., Kipnis, A., Carvalho, C.O., Campos, M.R.H., … Jubé, T.F.N. (2003). Qualidade microbiológica de leite humano obtido em banco de leite. Revista De Saúde Pública, 37, 775–779. doi:10.1590/S0034-89102003000600013
- Sikin, A.M., Walkling-Ribeiro, M., & Risvi, S.S.H. (2016). Synergistic effect of supercritical carbon dioxide and peracetic acid on microbial inactivation in shredded mozzarella-type cheese and its storage stability at ambient temperature. Food Control, 70, 174–182. doi:10.1016/j.foodcont.2016.05.050
- Silva, J.M., Rigo, A.A., Dalmolin, I.A., Debien, I., Cansian, R.L., Oliveira, J.V., & Mazutti, M.A. (2013). Effect of pressure, depressurization rate and pressure cycling on the inactivation of Escherichia coli by supercritical carbon dioxide. Food Control, 29, 76–81. doi:10.1016/j.foodcont.2012.05.068
- Soares, D., Lerin, L.A., Cansian, R.L., Oliveira, J.V., & Mazutti, M.A. (2013). Inactivation of Listeria monocytogenes using supercritical carbon dioxide in a high-pressure variable-volume reactor. Food Control, 31, 514–518. doi:10.1016/j.foodcont.2012.11.045
- Souza, S.G., Delgadillo, I., & Saraiva, J.A. (2016). Human milk composition and preservation: Evaluation of high-pressure processing as a nonthermal pasteurization technology. Critical Reviews in Food Sciences and Nutrition, 56, 1043–1060. doi:10.1080/10408398.2012.753402
- Werner, B.G., & Hotchkiss, J.H. (2006). Continuous flow nonthermal CO2 processing: The lethal effects of subcritical and supercritical CO2 on total microbial populations and bacterial spores in raw milk. Journal of Dairy Science, 89, 872–881. doi:10.3168/jds.S0022-0302(06)72151-8
- Zhang, J., Davis, T.A., Matthews, M.A., Drews, M.J., Laberge, M., & An, Y.H. (2006). Sterilization using high-pressure carbon dioxide. The Journal of Supercritical Fluids, 38, 354–372. doi:10.1016/j.supflu.2005.05.005