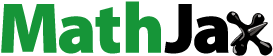
ABSTRACT
Plum slices (diameter 2.5 cm and thickness 0.2 cm) were dried using vertical tray-drying (VTD) and freeze-drying. Models were used to determine the thermal conductivity (k) and specific heat (Cp). In addition, water activity (aw), colour total change (∆E), pectin yield (ypec) and rehydration capacity were investigated. The moisture content of tray- and freeze-dried plum was relatively significant (p < 0.05). No significant difference was found in terms of aw. There was a maximum decrease in k and Cp of 90% and 91.5% during drying process, respectively. In terms of quality, ∆E showed that tray-dried samples had slight darker (browning), whereas the freeze-dried samples had a lighter colouring compared to the fresh fruit. The quality and rehydration characteristics of freeze-dried plum were higher than that of a tray-dried product; however, VTD could be appropriate for industrial production because of its advantages in lower cost and energy consumption.
RESUMEN
Las rebanadas de ciruela (diámetro 2,5 cm y espesor 0,2 cm) fueron secadas usando el secado vertical por charolas (VTD) y la liofilización (FD). Se utilizaron modelos para determinar la conductividad térmica (k) y el calor específico (Cp). Además, la actividad de agua (aw), el cambio total de color (∆E), el rendimiento de pectina (ypec) y la capacidad de rehidratación fueron investigados. El contenido de humedad de la ciruela seca y liofilizada fue relativamente significativo (p < 0,05). No se encontraron diferencias significativas en términos de aw. Hubo un máximo decremento en k y Cp del 90% y 95% durante el proceso de secado, respectivamente. En términos de calidad, ∆E mostró que las muestras secadas en charolas tuvieron un ligero oscurecimiento (pardeamiento), mientras las muestras liofilizadas tuvieron un color más luminoso en comparación con la muestra fresca. La calidad y las características de rehidratación fueron mayores que las de un producto secado por charolas; sin embargo, VTD podría ser apropiado para la producción industrial debido a sus ventajas de bajo costo y consumo energético.
Introduction
The plum (Spondias purpurea L.) is an aromatic fruit native to Central America and Southern Mexico. It is the most cultivated species in the genus Spondias. Plum is a drupe (stone fruit) whose size ranges from 3 to 8 cm in diameter. The colour of the ripe fruit ranges from yellow, green or red flesh, with equally varying skin colour (Maldonado-Astudillo et al., Citation2014). Plums are rich in minerals and vitamins and are mildly laxative. Analyses of fresh fruit showed that the percentage of moisture in the flesh ranged from 76% to 86%, protein and fat were very low and calcium, phosphorus, iron and ascorbic acid were found in appreciable quantities (Lim, Citation2012). Plums have a short harvest season and a limited time of storage under both ambient and refrigeration conditions. Industrialization of plum is necessary to obtain a product with a bigger resistance and consumption diversity to increase its commercialization.
Different methods of foodstuffs preservation are applied in the industry, but drying is commonly practised in traditional and industrial processing. The main purpose of drying is to preserve the quality and extend shelf life of the foodstuffs, by lowering water activity through decrease in moisture content, thus avoiding during storage (Ioannou, Guiga, Charbonnel, & Ghoul, Citation2011). In addition, drying in the food industry is used to (1) obtain the desired physical form, colour, flavour or texture; (2) reduce volume or weight for transportation and (3) produce new products that would not otherwise be feasible (Erbay & Icier, Citation2010; Silva, Almeida, Lima, Silva, & Gomes, Citation2008).
Hot-air drying is the most simple and economical method for food dehydration. A convective hot-air dryer can provide relatively high drying temperature in the range of 40–90°C which is favourable for fruits and vegetables and relative humidity in drying chamber ranges from 8% to 25% (Morales-Delgado et al., Citation2014). There are many disadvantages of this method; the foodstuffs are sensitive to high drying air temperature and long drying time (Villegas‐Santiago, Calderón‐Santoyo, Ragazzo‐Sánchez, Salgado‐Cervantes, & Luna‐Solano, Citation2011), which causes shrinkage, changes in shape, loss of volume and an increased hardness of the product which in many cases result in a decrease of rehydration and a negative impression to customers (Miranda, Maureira, Rodriguez, & Vega-Gálvez, Citation2009). Another drawback of the convective method is the decrease of the content of some valuable components. Pigment degradation is the most common among them, especially disease-fighting compounds like anthocyanin, carotenoid and chlorophyll, as well as browning reactions such as Maillard reaction and oxidation of acid ascorbic (Chen & Martynenko, Citation2013).
Among the drying methods that used in food processing industries, freeze-drying (FD) is considered one of most advanced methods for drying high value products, since it prevents undesirable shrinkage and produces materials with high porosity, good nutritional quality, superior texture, aroma, flavour and colour retention as well as better rehydration capacity (Oikonomopoulou, Krokida, & Karathanos, Citation2011).
FD process consists mainly of two stages: the freezing stage and the sublimation (drying stages). The freezing stage has great influence on the overall efficiency of the FD; the ice crystals create pores and gaps with different characteristics, which affect the heat and mass transfer rates and the sublimation (Oikonomopoulou et al., Citation2011). During primary stage, the temperature of frozen layer is most often at −10°C or lower at absolute pressures of about 2 mmHg or less (Liapis & Bruttini, Citation2006) and at the completion of primary drying, the product can still contain approximately 15–20% of unfrozen water, which is then desorbed during the secondary drying stage, usually at elevated temperature and low pressure, to finally allow the desired low moisture content to be achieved (Kasper & Friess, Citation2011). The main disadvantages of FD are long drying time, and high capital and energy costs due to the refrigeration and vacuum systems (Huang, Zhang, Wang, Mujumdar, & Sun, Citation2012).
In drying process, thermal properties are of great importance, since they are continuously affected by moisture content, density, structure and temperature. Thermal properties are necessary for modelling processes, engineering design of processing equipment and calculating energy demand (Mahapatra, Melton, & Isang, Citation2013). Although the capacities of drying systems can be estimated from the thermal properties of the material being dried, a more thorough understanding of the process requires knowledge of the rate at which moisture moves through the material and the rate at which it is transferred from surface of the material to the surrounding atmosphere.
Drying process has been studied by several researches for a number of species of plum. Fante, Corrêa, Natividade, Lima and Lima (Citation2011) studied the drying of plums (Prunus sp., cv. Gulfblaze) submitted to treatments with KCl and pulsed vacuum osmotic dehydration. Ioannou et al. (Citation2011), Sacilik, Elicin, and Unal (Citation2006) and Živković et al. (Citation2011) studied the kinetics of convective drying of Mirabelle, Uryani and Požegača plums, respectively. Jazini and Hatamipour (Citation2010) studied the effect of physical and chemical pretreatment on drying time of Uryani plums. Michalska, Honke, Łysiak and Andlauer (Citation2016) evaluated the impact of drying methods on selected bioactive compounds in different plum (Prunus domestica L.) cultivars. The authors reported that the progress of the Maillard reaction in plums is strictly dependent on the process temperature. However, the findings on S. purpurea drying are rather scarce. As mentioned above, this fruit is mainly commercialized in local markets and has an incipient postharvest management, with few methods for postharvest conservation (Maldonado-Astudillo et al., Citation2014). The selection of the proper drying technology can affect the final product quality and the drying economic (Beaudry, Raghavan, Ratti, & Rennie, Citation2004). Therefore, the main objective of this study was to evaluate the effect of tray-drying and FD as methods to obtain dried plum. The product quality and drying kinetics of dried plums were investigated; the rehydration capacity and pectin yield were determined as a measure of the injury to the material caused by drying treatment. In addition, models were used to describe the thermal properties of samples.
Materials and methods
Raw material
S. purpurea L. (cv. Beetroot) fruits were obtained from plum Agricultural Group located in the city of Coscomatepec Veracruz, Mexico. Selecting was based on a similar ripening degree (30% green, 70% red) (Muy et al., Citation2010). The plums were then washed and cut into thinly sliced (2.5 cm diameter) of 0.2 cm thickness using a cutting device designed for this purpose. This cutter was used in order to obtain cylinder of same diameters and slices were cut parallel to the fruit axis. The fresh fruit had an initial moisture content of 78% (w.b.) which was measured with an infrared moisture balance (MB35 Halogen, Ohaus, USA). The mean composition of fresh plum was determined as proposed by Miranda et al. (Citation2009), and the main results are shown as follow: carbohydrate: 19.1 g/100 g, protein 0.7 g/100 g, lipids: 0.26 g/100 g and ash: 0.7 g/100 g. The pH 4.02 was measured by means of pH meter (Conductronic, PH 10, USA). All measurements were done in triplicate.
Freezing procedure
Before FD, the raw material was frozen using two methods. The first method involved freezing plum slices in a chamber of freeze dryer (Mod-742002 Labconco, USA) at −20°C for 3 h (quick freezing) without further storage. The second method involved freezing plum slices in a home freezer (FR-061, Daewoo, KR) at −5°C (slow freezing) followed by 48 h storage.
Drying procedure
Air-drying experiments were performed in a pilot scale vertical tray dryer (MOD-SEM-2 Polinox, MX), described previously by Villegas et al. (Citation2011). About 2 kg of sliced plums were dried at air temperature of 50, 65 and 80°C in a tray dryer with air flow rate of 1 m/s. In order to monitor the moisture, aw and colour during drying, samples of approximately 1 g were taken at regular interval of time during a period of 360 min.
The FD was conducted in laboratory scale freeze dryer (Mod-742002 Labconco, USA), which consists of stainless steel chamber with 12 ports, 2.5 l capacity collector chamber, refrigeration system (−84°C) and vacuum control system (set-point 12 Pa). A batch of 100 g sliced plum was taken for each experiment at different vacuum pressures (5 and 10 Pa). The flasks containing the frozen plum slices were removed for specific intervals while FD took place. Changes in the moisture, aw and colour of sample were recorded every 60 min during a period of 360 min.
Analytical methods
Moisture variation during the drying process was monitored with an infrared moisture balance (MB35 Halogen, Ohaus, USA) at 65°C of sample containing approximately 1 g sample. The results were expressed in terms of dry solid (d.s.). Water activity was determined at 25 ± 1°C using water activity meter (Aqualab series 3TE, Decagon, USA). The readings were performed in duplicate.
The colour of fresh and dried plum was measured using colorimeter HunterLab (MiniScan XE plus, HunterLab, USA). The instrument was calibrated using black and white standard tiles. During each run of drying, plums were removed at specified time intervals to describe their colour on the Hunter parameters: L* (lightness/darkness), a* (redness/greenness) and b* (yellowness/blueness). The total colour change (∆E) was calculated according to equation:
where the standard colour value ( 16.24,
16.69,
4.97) was used.
Empirical models are widely popular for the prediction of thermal conductivity and specific heat. The k of plum is a measure of its ability to transmit heat during drying and was estimated using Sweat Equation (2). The Cp of plum is the amount of heat needed to increase the temperature of a unit of mass by one degree. Heldman and Singh (Citation1981) gave a generalized equation for specific heat in kJ/kg °C, which was taken into account the composition of foodstuffs and was used in this study (Equation 3).
where m is the mass (or weight) fraction of each component.
Pectin yield
Pectin extraction from fresh and dried plum was determined according to Kliemann et al. (Citation2009), with modifications. A 5 ± 0.01 g of dried sample was subject to extraction by adding 100 mL water distilled. The pH was adjusted to 2.5 with citric acid. This dispersion was heated at 90°C and extraction was carried out with continuous stirring for 60 min. The extract was filtered through cotton cloth (cheesecloth). Concentrated solution of pectin was coagulated using an equal volume of 96% ethanol and left for 2 h. The coagulated pectin was separated by filtration and washed with 96% ethanol. The wet pectin was dried in oven until constant weight. The pectin yield is calculated using the following equation:
where ypec is the extracted pectin yield in %, P is the amount of extracted pectin in g and Bi is the initial amount of fresh plum in g.
Rehydration capacity
Rehydration experiments were performed in distilled water and milk at 10 and 30°C by immersing. Temperature was kept constant by means of a thermostatic plate. Approximately 0.5–0.6 g of dried sample was placed in flask containing 150 mL of liquid. Rehydration times were 2, 4, 6, 10, 15, 20, 25, 30, 35, 40, 50 and 60 min. At these intervals, samples were removed from liquid, carefully blotted with absorbent paper in order to remove the excess of solution and weighed. Rehydration capacity was calculated as the maximum amount of solution absorbed (g) per g of dry material as determined at the end of the rehydration time for each experiment.
Statistical analysis
summarizes the conditions applied for different drying experiments. All experiments were conducted in duplicate. The data were analysed statistically using analysis of variance (ANOVA) using Minitab Release 14. Mean values were considered significantly different when p < 0.05.
Table 1. Experimental conditions for each drying experiment.
Tabla 1. Condiciones experimentales para cada experimento de secado.
Results and discussion
Drying kinetics and water activity
shows the experimental data of moisture content and water activity as a function of drying time for VTD and FD. As it is expected, it can be seen that the moisture ratio decreased continually with drying time during the initial stages, which later decrease with increase in drying time ()). According to Kumar and Sagar (Citation2014), the presence of falling rate period confirmed that the moisture removed was driven internally by energy absorption and the internal mass which controls the drying process. The results of the analysis of variance (ANOVA) showed that drying temperature had a high significant effect on moisture loss (Dunnettʼs comparisons, p < 0.05). The effect on drying rate was much more pronounced when higher temperatures were applied (65 and 80°C), as compared to lower drying temperature (50°C). The equilibrium moisture content was reached at 180 min for higher temperatures and 240 min for lower temperature, respectively. Similar effects of air temperatures on drying moisture content have been found in the air drying of tomato (Arslan & Özcan, Citation2011), Üryani plums (Sacilik et al., Citation2006), coriander (Silva et al., Citation2008) and Požegača plums (Živković et al., Citation2011).
Figure 1. Variation of moisture content and water activity of plum slices as a function of time (a) tray-drying and (b) freeze-drying.
Figura 1. Variación del contenido de humedad y la actividad de las hojuelas de ciruela en función al tiempo (a) secado por charolas (b) liofilización.
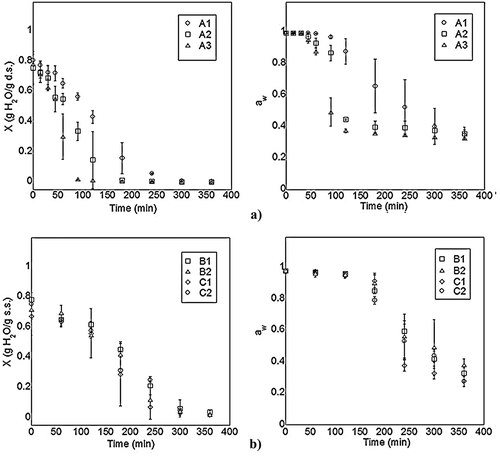
) shows the drying kinetics of freeze-dried samples. For slow freezing, the ANOVA indicated that lowest vacuum pressure had a significant influence (p < 0.05) on moisture content during FD; this behaviour may be attributed to higher vacuum level, due to that it increases the driving force for mass transfer and facilitates the evaporation and volatilization of water in materials. In contrast, for quick freezing, the moisture content was found to be independent of the vacuum pressure employed in FD. In addition, drying rate is higher in the experiments performed on quick frozen samples, which might be due to structural changes (opening) produced by quick freezing stage. Nevertheless, all experiments reached the equilibrium moisture at 300 min of drying process.
Both VTD and FD led to a significant reduction in the moisture content of plum slices. The tray- and freeze-dried samples previously freezing at −20°C gave the lowest final moisture content, while freeze-dried plum obtained of plums freezing at −5°C showed high moisture content. However, all experiments reduced the moisture content of product to less than 0.01 g H2O/g d.s.
The initial water activity of fresh plum was 0.997. The aw was reduced to about 0.3 (corresponding to moisture content about 0.02 g H2O/g d.s.) under different drying conditions previously stated. The aw decreased continuously with increase in the time period for both methods, and during initial stages of drying, there was a rapid water removal of the product. Drying curves obtained with VTD showed similar trends ()). As it would expect, the higher drying temperature had much more effect on the aw of product (Bchir et al., Citation2012).
The corresponding values of aw of FD ()) showed a similar trend as the aw variation as a function of time, although the rate of decreasing of aw is slightly higher when quick freezing is used instead of slow freezing, probably due to the generation of smaller pores (left by quick freezing and sublimation) in first case, favouring loss of water (Marques, Ferreira, & Freire, Citation2007). According to the results of treatments, there was no significant difference in terms of aw values (p > 0.05). However, the aw of tray-dried samples was similar to freeze-dried plum frozen at −5°C, in comparison to the values obtained for freeze-dried samples frozen at −20°C.
On basis of literature (Barajas, Cortes‐Rodríguez, & Rodríguez‐Sandoval, Citation2012; Henríquez, Almonacid, Lutz, Simpson, & Valdenegro, Citation2013), foodstuffs with aw < 0.6 and moisture content of approximately 0.18–0.2 g H2O/g d.s. are considered safe and stable with respect to microbial growth, and enzymatic, chemical and physical changes that can lead to food degradation during storage, which means that the values obtained for both drying methods ensure stability of plum.
Thermal properties
In porous solids such as foods, thermal properties depend mostly on composition. In case of drying process, k and Cp decreased with decreasing moisture content due to the formation of pores (air phase) in foods during drying (Sahin & Sumnu, Citation2006). The values of k of plum () obtained ranged from 0.051 to 0.053 W/m °C and 0.060 to 0.073 W/m °C from VTD and FD, respectively. Decreasing moisture content had a significant effect on k values for all treatments; therefore, the k curves exhibit similar trends to the moisture curves. The ANOVA of k showed significant difference (p < 0.05) among the VTD treatments ()). However, the k in tray-dried samples was found to have no significant difference with the freeze-dried samples previously freezing at −20°C. In addition, FD of plum showed significant differences when slow freezing condition (−5°C) was applied ()). This is because the final moisture content directly affects the k of the product; higher final moisture content within the sample when using slow freezing rate for FD led to higher k.
Figure 2. Thermal conductivity and specific heat of plum slices as a function of time (a) tray-drying and (b) freeze-drying.
Figura 2. Conductividad térmica y calor especifico de las hojuelas de ciruela en función del tiempo (a) secado por charolas (b) liofilización.
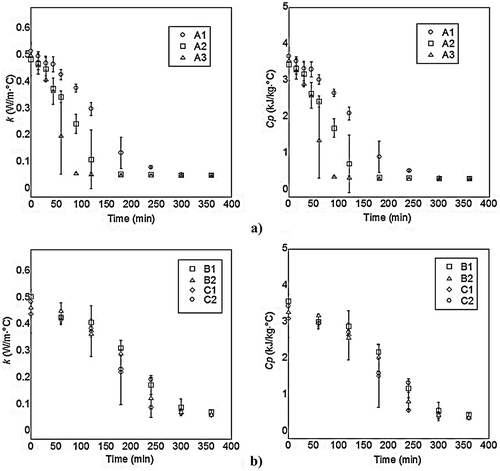
A similar behaviour was also noted by Donsí, Ferrari and Nigro (Citation1996), who evaluated the k of potato and apple at different moisture contents; they found that k is a function of water mass fraction of the samples and it decreases as moisture content decreases. In contrast, Mahapatra et al. (Citation2013) reported the effect of increasing moisture content on thermal properties of cowpea flour; they concluded that k increased with increase in moisture content.
Similarly as thermal conductivity curves, specific heat curves follow from moisture content curve trends. The Cp of tray-dried plum ranged from 0.301 to 0.312 kJ/kg °C, while freeze-dried plum ranged from 0.365 to 0.457 kJ/kg °C. Slow freezing rates for freeze-dried plum produce samples with higher final moisture content resulted in higher Cp (). These values were significantly different (p < 0.05) from tray- and freeze-dried samples obtained from quick freezing condition, which showed lower Cp owing to lower final moisture content.
According to results, changes in the moisture content range had a linear correlation on the thermophysical properties of plums. Phomkong, Srzednicki and Driscoll (Citation2006) concluded that thermophysical properties of stone fruits during drying have strong moisture dependence but were not significantly dependent on temperature. Moreover, the authors observed that major components of the fruit solids, namely sugars, underwent thermal reactions that resulted in changes in the chemical composition that affected the Cp.
Colour analysis
The colour data in terms of the Hunter values (L*, a*, b*, ∆E) of fresh and dried plum were showed in . The ANOVA showed that the treatments had significant effects on colour features of dried plum (p < 0.05).
Table 2. Colour parameters of fresh, tray-dried and freeze-dried Mexican plum slices.
Tabla 2. Parámetros de color de ciruela mexicana fresca, secada por charolas y liofilizada.
Tray-dried samples had a sight lower value of L* compared to fresh sample; these decreases in L* values (lightness) have been correlated with the concentration effect due to the loss of water by evaporation, deformation of surface (shrinkable) and generation of brown pigments. These pigments can be formed in various reactions such as non-enzymatic browning (Maillard reaction and/or auto-oxidation) and enzymatic browning catalysed by polyphenol oxidases, involving phenolic compounds (Marquez, Serratosa, & Merida, Citation2013).
In contrast, for freeze-dried plum, the clearest effect was the increase in sample lightness; this difference may be associated to the removal water by sublimation of ice, preventing enzymatic browning reactions, which results in a relative stability of the colour. In addition, lightness of plum was significantly affected by quick freezing (p < 0.05). Rate of freezing was found to have a marked effect on the lightness of the dried samples as the quick frozen materials maintained a lighter colour than those frozen more slowly. Thus, high rate of freezing leads to small, randomly oriented ice crystals; as a result of this, the lightness of a rapidly frozen product is higher than that of the same product frozen slowly (Ishwarya & Anandharamakrishnan, Citation2015).
The dominant colour in plum is red and hence can be best represented by a* (redness) to distinguish the colour difference of the resulting dried slices as affected by the drying process.
Tray-drying treatments caused a significant decrement of a* values, which suggests that the loss of red colour in the final product occurred in a greater ratio. According to Awuah, Ramaswamy and Economides (Citation2007), naturally occurring pigments in food are susceptible to changes or degradation from heat. The loss in a* values was mainly attributed to partial decomposition of anthocyanin of plum by high processing temperatures as well as the exposure to large amounts of oxygen owing to the long drying time. The degradation of anthocyanin content with decreasing a* value in red-bright or purple colour food is in agreement with a number of other studies (Chen & Martynenko, Citation2013; Morales-Delgado et al., Citation2014). With respect to FD, the more pronounced red colour of samples can be explained by the influence of the freezing step (temperature and freezing rate) and pigment concentration due to water reduction. Besides Shishehgarha, Makhlouf and Ratti (Citation2002) mentioned that anthocyanin colour varies according to changes in pH caused by freezing process. Therefore, a decrease in pH concentration during FD can change the anthocyanin equilibrium, which may increase the red colour intensity. In addition, no significant difference was observed on the b* value (yellowness) between tray- and freeze-dried samples.
It is clear that ∆E was affected significantly by VTD and FD. In general, the tray-dried samples showed the lowest colour difference, with respect to the fresh sample. Less pronounced colour changes for tray-dried samples are related with browning reactions on the surface of product. The browner colour of dried plum can be explained by lower L* and a* values due to higher temperatures associated with VTD. In contrast, the freeze-dried samples had lighter colouring compared with fresh fruit, given that FD increased the lightness (L*) of product, which consequently affected the ∆E value. These results are in good agreement with the earlier studies performed by Chin and Law (Citation2010) on Ganoderma tsugae Murrill, who reported that the ∆E of freeze-dried samples was highest when compared to the samples dried under convective drying processes, due to changes in lightness (L*) and less browning of freeze-dried samples.
Pectin yield
The results of VTD ()) showed that plums dried at 50 and 65°C had similar ypec values. However, there was slight decrease in the ypec when higher air-drying condition was performed. This latter observation is consistent with the findings of Contreras, Martin, Martínez-Navarrete and Chiralt (Citation2005) for structural changes of apple during air-drying treatments. The authors attributed this behaviour to pectin solubilization phenomenon, as increase in water-soluble pectin content and decrease in protopectin fraction with high drying temperatures are employed.
Figure 3. Effect of (a) tray-drying and (b) freeze-drying on pectin yield.
Figura 3. Efecto del (a) secado por charolas y (b) la liofilización en el rendimiento de pectina.
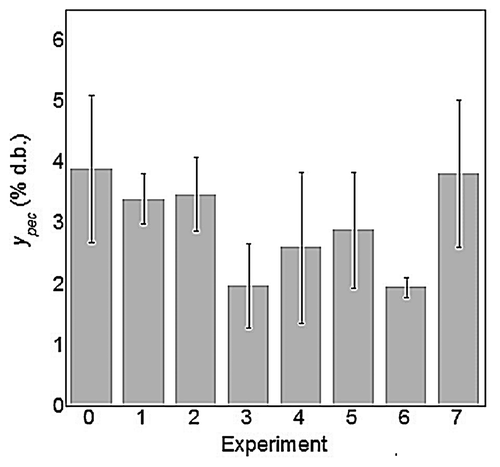
The ypec of tray-dried samples was similar to samples produced by the sequence of slow freezing and FD; and both results were slight lower in comparison with experiments performed on quick frozen samples freeze dried at highest pressures. It is clear that high pressures improved extraction of pectin and other bioactive constituents, as high pressure can cause cell deformation, cell membrane damage, even cell rupture and improve the mass transfer rate, solvent permeability in cells as well as the secondary metabolite diffusion (Guo et al., Citation2012). According to the results obtained, no significant difference (p > 0.05) was found in terms of ypec between VTD and FD conditions. This is consistent with the observations made by Monsoor (Citation2005), who reported that there was no difference in pectin yield for oven-dried and freeze-dried soy hull.
Rehydration capacity
The rehydration data of plum processed using different drying treatments and hydrated in water and milk are presented in . As a result of the analysis, statistically significant influence of drying conditions on rehydration of tray- and freeze-dried samples was observed.
Table 3. Rehydration capacity of dried Mexican plum samples using different immersion media and temperatures.
Tabla 3. Capacidad de rehidratación de las muestras de ciruela mexicana deshidratada usando diferentes medios de inmersión y temperatura.
In case of VTD, samples dried at lower drying temperature (50°C) exhibited lower rehydration capacity. This may be attributed to the prolonged drying period associated with low temperature drying conditions that increased thermal disruption of the cellular organization, which in turn renders impaired rehydratability and a reduction in rehydration capacity (Kumar & Sagar, Citation2014). Moreover, when high drying temperatures are applied (65 and 80°C), the rehydration capacity of plum samples increased, owing to the fact that the rate of the moisture removal at higher temperature is fast and causes less shrinkage of the dried samples.
The rehydration capacity of samples dried by FD, in general, was higher than samples dried by VTD. The FD produced highly porous products that showed a high rehydration capacity, as the internal structure of freeze-dried fruit remains quite undisturbed. This can be attributed to the structural rigidity of the frozen product, which can prevent the collapse of the solid matrix remaining after drying (Beaudry et al., Citation2004). In addition, according to the results obtained, the effect of operating pressure and freezing rates are negligible.
On the other hand, at the equilibrium, the rehydration capacity of the samples dried by both drying techniques was significantly influenced by immersion media and rehydration temperature. Rehydration capacity was higher for samples hydrated in water than those in milk. In water, the absorption of liquid occurred at higher rate in the early stages followed by slower absorption rate in the final stage. This fact occurs due to rapid rehydration of capillaries and cavities near the superficie, which are rapidly filled up with water. In milk, the formation of a layer of lipids and micelles deposited on the food surface creates a major barrier to moisture transfer and explains the slower intake of moisture in product immersed in this liquid media, and the accumulation of fat at the solid matrix surface promotes that the fruit slice retains its cellular structure better (Sacchetti, Pittia, Biserni, Pinnavaia, & Rosa, Citation2003). As expected, the rehydration capacity at 30°C was more rapid than the samples hydrated at 10°C by both immersion media. This is probably due to the fact that higher temperature of immersion media, quicker is the diffusion of liquid into the material, in addition decreasing viscosity of the immersion media and the effects of temperature on the food material structure increase the rehydration ratio.
Conclusions
The characteristics of tray- and freeze-drying of Mexican plum were determined in terms of their physicochemical and thermal properties. A significant difference was observed in the drying time at equilibrium moisture, where the lower time was obtained using convective air drying (180 min) in comparison with freeze-drying (300 min). For both drying methods, aw < 0.6, this ensures microbiological stability of plum. The results showed that the thermal properties of plum varied linearly with moisture content during drying processes. With respect to colour, the air-drying process produced very small changes in colour (∆E) whereas the freeze-drying process originated more colour changes. The values of L* have increased during freeze-drying. For both drying methods, the results of pectin yield showed no significant differences (p < 0.05). In terms of rehydration capacity, freeze-dried plum produced the best acceptable product rehydrated. The results of this study showed that tray-drying and FD are desirable methods to produce dry plum. Tray-drying with media temperature is appropriated for drying industry due to low cost and energy consumption. Therefore, freeze-drying can produce high-quality product with higher rehydration capacity compared to convective drying. Depending on the application or characteristics of final product, a drying method should be chosen to obtain the better product to satisfy the requirements of the market.
Acknowledgements
The authors express acknowledge to the Mexican Consejo Nacional de Ciencia y Tecnología (INFR-2011-3-CONACyT-Proyecto 173906) and TecNM (5124.13-P) by the financial support of this research.
Disclosure statement
No potential conflict of interest was reported by the authors.
Additional information
Funding
References
- Arslan, D., & Özcan, M.M. (2011). Drying of tomato slices: Changes in drying kinetics, mineral contents, antioxidant activity and color parameters. CyTA-Journal of Food, 9, 229–236. doi:10.1080/19476337.2010.522734
- Awuah, G.B., Ramaswamy, H.S., & Economides, A. (2007). Thermal processing and quality: Principles and overview. Chemical Engineering and Processing: Process Intensification, 46, 584–602. doi:10.1016/j.cep.2006.08.004
- Barajas, J., Cortes‐Rodríguez, M., & Rodríguez‐Sandoval, E. (2012). Effect of temperature on the drying process of bee pollen from two zones of Colombia. Journal Food Process Enginnering, 35, 134–148. doi:10.1111/j.1745-4530.2010.00577.x
- Bchir, B., Besbes, S., Karoui, R., Attia, H., Paquot, M., & Blecker, C. (2012). Effect of air-drying conditions on physico-chemical properties of osmotically pre-treated pomegranate seeds. Food and Bioprocess Technology, 5, 1840–1852. doi:10.1007/s11947-010-0469-3
- Beaudry, C., Raghavan, G.S.V., Ratti, C., & Rennie, T.J. (2004). Effect of four drying methods on the quality of osmotically dehydrated cranberries. Drying Technology, 22, 521–539. doi:10.1081/DRT-120029999
- Chen, Y., & Martynenko, A. (2013). Computer vision for real-time measurements of shrinkage and color changes in blueberry convective drying. Drying Technology, 31, 1114–1123. doi:10.1080/07373937.2013.775587
- Chin, S.K., & Law, C.L. (2010). Product quality and drying characteristics of intermittent heat pump drying of Ganoderma tsugae Murrill. Drying Technology, 28, 1457–1465. doi:10.1080/07373937.2010.482707
- Contreras, C., Martin, M.E., Martínez-Navarrete, N., & Chiralt, A. (2005). Effect of vacuum impregnation and microwave application on structural changes which occurred during air-drying of apple. LWT-Food Science and Technology, 38, 471–477. doi:10.1016/j.lwt.2004.07.017
- Donsí, G., Ferrari, G., & Nigro, R. (1996). Experimental determination of thermal conductivity of apple and potato at different moisture contents. Journal of Food Engineering, 30, 263–268. doi:10.1016/S0260-8774(96)00044-1
- Erbay, Z., & Icier, F. (2010). A review of thin layer drying of foods: Theory, modeling, and experimental results. Critical Reviews in Food Science and Nutrition, 50, 441–464. doi:10.1080/10408390802437063
- Fante, C., Corrêa, J., Natividade, M., Lima, J., & Lima, L. (2011). Drying of plums (Prunus sp, cv Gulfblaze) treated with KCl in the field and subjected to pulsed vacuum osmotic dehydration. International Journal of Food Science and Technology, 46, 1080–1085. doi:10.1111/j.1365-2621.2011.02619.x
- Guo, X., Han, D., Xi, H., Rao, L., Liao, X., Hu, X., & Wu, J. (2012). Extraction of pectin from navel orange peel assisted by ultra-high pressure, microwave or traditional heating: A comparison. Carbohydrate Polymers, 88, 441–448. doi:10.1016/j.carbpol.2011.12.026
- Heldman, D.R., & Singh, R.P. (1981). Food process engineering. New York, NY: Springer.
- Henríquez, M., Almonacid, S., Lutz, M., Simpson, R., & Valdenegro, M. (2013). Comparison of three drying processes to obtain an apple peel food ingredient. CyTA-Journal of Food, 11, 127–135. doi:10.1080/19476337.2012.703693
- Huang, L.L., Zhang, M., Wang, L.P., Mujumdar, A.S., & Sun, D.F. (2012). Influence of combination drying methods on composition, texture, aroma and microstructure of apple slices. LWT-Food Science and Technology, 47, 183–188. doi:10.1016/j.lwt.2011.12.009
- Ioannou, I., Guiga, W., Charbonnel, C., & Ghoul, M. (2011). Frozen mirabelle plum drying: Kinetics, modelling and impact on biochemical properties. Food and Bioproducts Processing, 89, 438–448. doi:10.1016/j.fbp.2010.07.001
- Ishwarya, S.P., & Anandharamakrishnan, C. (2015). Spray-Freeze-Drying approach for soluble coffee processing and its effect on quality characteristics. Journal of Food Engineering, 149, 171–180. doi:10.1016/j.jfoodeng.2014.10.011
- Jazini, M.H., & Hatamipour, M.S. (2010). A new physical pretreatment of plum for drying. Food and Bioproducts Processing, 88, 133–137. doi:10.1016/j.fbp.2009.06.002
- Kasper, J.C., & Friess, W. (2011). The freezing step in lyophilization: Physico-chemical fundamentals, freezing methods and consequences on process performance and quality attributes of biopharmaceuticals. European Journal of Pharmaceutics and Biopharmaceutics, 78, 248–263. doi:10.1016/j.ejpb.2011.03.010
- Kliemann, E., Simas, D., Nunes, K., Amante, E.R., Prudêncio, E.S., Teófilo, R.F., … Amboni, R.D. (2009). Optimisations of pectin acid extraction from passion fruit peel (Passiflora edulis flavicarpa) using response surface methodology. International Journal of Food Science and Technology, 44, 476–483. doi:10.1111/j.1365-2621.2008.01753.x
- Kumar, P.S., & Sagar, V.R. (2014). Drying kinetics and physico-chemical characteristics of osmo-dehydrated mango, guava and aonla under different drying conditions. Journal of Food Science and Technology, 51, 1540–1546. doi:10.1007/s13197-012-0658-3
- Liapis, A.I., & Bruttini, R. (2006). Freeze drying. In A. Mujundar (Ed.), Handbook of industrial drying (pp. 257–281). Boca Raton: CRC Press.
- Lim, T.K. (2012). Edible medicinal and non-medicinal plants (Vol. 1). New York, NY: Springer.
- Mahapatra, A.K., Melton, S.L., & Isang, E.M. (2013). Effect of moisture content on thermal properties of cowpea flours. Agricultural Engineering International: CIGR Journal, 15, 251–255.
- Maldonado-Astudillo, Y.I., Alia-Tejacal, I., Núñez-Colín, C.A., Jiménez-Hernández, J., Pelayo-Zaldívar, C., López-Martínez, V., … Valle-Guadarrama, S. (2014). Postharvest physiology and technology of Spondias purpurea L. and S. mombin L. Scientia Horticulturae, 174, 193–206. doi:10.1016/j.scienta.2014.05.016
- Marques, L.G., Ferreira, M.C., & Freire, J.T. (2007). Freeze-drying of acerola (Malpighia glabra L.). Chemical Engineering and Processing: Process Intensification, 46, 451–457. doi:10.1016/j.cep.2006.04.011
- Marquez, A., Serratosa, M.P., & Merida, J. (2013). Anthocyanin evolution and color changes in red grapes during their chamber drying. Journal of Agricultural and Food Chemistry, 61, 9908–9914. doi:10.1021/jf402263f
- Michalska, A., Honke, J., Łysiak, G., & Andlauer, W. (2016). Effect of drying parameters on the formation of early and intermediate stage products of the Maillard reaction in different plum (Prunus domestica L.) cultivars. LWT-Food Science and Technology, 65, 932–938. doi:10.1016/j.lwt.2015.09.015
- Miranda, M., Maureira, H., Rodriguez, K., & Vega-Gálvez, A. (2009). Influence of temperature on the drying kinetics, physicochemical properties, and antioxidant capacity of Aloe Vera (Aloe Barbadensis Miller) gel. Journal of Food Engineering, 91, 297–304. doi:10.1016/j.jfoodeng.2008.09.007
- Monsoor, M.A. (2005). Effect of drying methods on the functional properties of soy hull pectin. Carbohydrate Polymers, 61, 362–367. doi:10.1016/j.carbpol.2005.06.009
- Morales-Delgado, D.Y., Téllez-Medina, D.I., Rivero-Ramírez, N.L., Arellano-Cárdenas, S., López-Cortez, S., Hernández-Sánchez, H., … Cornejo-Mazón, M. (2014). Effect of convective drying in total anthocyanin content, antioxidant activity and cell morphometric parameters of strawberry parenchymal tissue (Fragaria x ananassa Dutch). Revista Mexicana De Ingeniería Química, 13, 179–187.
- Muy, B., Contreras, P., Martínez, C., Rubio, S., Osuna, V.D.L.R., & Sañudo. 2010. Calidad de la ciruela roja mexicana deshidratada mediante sol y calor seco. [Quality of Mexican red plum dzehydrated by sun and dry heat] Retrieved from Fundación produce Sinaloa website: http://www.fps.org.mx/portal/index.php/pub licaciones/100-frutales/1242-calidad-de-ciruela-roja-mexicana-deshidratada-mediante-sol-y-calor-seco.
- Oikonomopoulou, V.P., Krokida, M.K., & Karathanos, V.T. (2011). The influence of freeze drying conditions on microstructural changes of food products. Procedia Food Science, 1, 647–654. doi:10.1016/j.profoo.2011.09.097
- Phomkong, W., Srzednicki, G., & Driscoll, R.H. (2006). Thermophysical properties of stone fruit. Drying Technology, 24, 195–200. doi:10.1080/07373930600559100
- Sacchetti, G., Pittia, P., Biserni, M., Pinnavaia, G.G., & Rosa, M.D. (2003). Kinetic modelling of textural changes in ready‐to‐eat breakfast cereals during soaking in semi‐skimmed milk. International Journal of Food Science and Technology, 38, 135–143. doi:10.1046/j.1365-2621.2003.00654.x
- Sacilik, K., Elicin, A.K., & Unal, G. (2006). Drying kinetics of Üryani plum in a convective hot-air dryer. Journal of Food Engineering, 76, 362–368. doi:10.1016/j.jfoodeng.2005.05.031
- Sahin, S., & Sumnu, S.G. (2006). Physical properties of foods. New York: Springer.
- Shishehgarha, F., Makhlouf, J., & Ratti, C. (2002). Freeze-drying characteristics of strawberries. Drying Technology, 20, 131–145. doi:10.1081/DRT-120001370
- Silva, A.S., Almeida, F.D.A., Lima, E.E., Silva, F.L.H., & Gomes, J.P. (2008). Drying kinetics of coriander (Coriandrum sativum) leaf and stem. Cyta-Journal of Food, 6, 13–19.
- Villegas‐Santiago, J., Calderón‐Santoyo, M., Ragazzo‐Sánchez, A., Salgado‐Cervantes, M.A., & Luna‐Solano, G. (2011). Fluidized bed and tray drying of thinly sliced mango (Mangifera indica) pretreated with ascorbic and citric acid. International Journal of Food Science and Technology, 46, 296–1302. doi:10.1111/j.1365-2621.2011.02637.x
- Živković, M., Rakić, S., Maletić, R., Povrenović, D., Nikolić, M., & Kosanović, N. (2011). Effect of temperature on the physical changes and drying kinetics in plum (Prunus domestica L.) Požegača Variety. Chemical Industry and Chemical Engineering Quarterly/CICEQ, 17, 283–289. doi:10.2298/CICEQ101109013Z