ABSTRACT
This study evaluated the effect of Rhizopus oryzae, on essential amino acids and fatty acid profile on lignocellulosic substrates soybean, wheat and rice bran. R. oryzae was isolated from crop of Brazilian south region, genetically characterized and solid-state fermentation was carried on tray reactors during 120 h/30°C. The profile of amino acids and fatty acids was conducted every 24 h by high performance liquid chromatography with fluorescence detector (HPLC-FL) and gas chromatography (GC)-flame ionization detector, respectively. Fatty acids found on rice, wheat and soybean bran biomass showed an increase in the ω3 acids (72%, 64% and 58%, respectively); however, ω6 level was not affected. Fungal biomass on soybean and wheat bran present the most complete essential amino acids profile from a nutritional point of view, increased in Val (34%), Leu (19%), Lys (6%) and Phe (2%) after 96 h of cultivation. The cultivation with R. oryzae triggers changes on essential nutrients of the agro-industrial by-products.
RESUMEN
El presente estudio se orientó a evaluar el efecto provocado por Rhizopus oryzae en el perfil de aminoácidos y ácidos grasos esenciales presentes en los sustratos lignocelulósicos de la soya, el trigo y el salvado de arroz. Para ello se aisló el Rhizopus oryzae de una cosecha procedente de una región del sur de Brasil, sometiéndolo a una caracterización genética, y se realizó la fermentación en estado sólido con reactores de bandeja durante 120 horas a 30°C. Cada 24 horas se determinó el perfil de aminoácidos y de ácidos grasos empleando HPLC-FL y CG-FID, respectivamente. Los ácidos grasos detectados en la biomasa de arroz, trigo y salvado de soya mostraron un aumento de ácidos ω3 (72%, 64% y 58%, respectivamente), sin que se viera afectado el nivel de los ω6. Desde una perspectiva nutricional, se constata que la biomasa micótica de la soya y el salvado de trigo presenta el perfil más completo de aminoácidos esenciales, registrándose incrementos en Val (34%), Leu (19%), Lys (6%) y Phe (2%) después de 96 horas de cultivo. El cultivo con Rhizopus oryzae provoca alteraciones en los nutrientes esenciales de los subproductos agroindustriales.
1. Introduction
The use of agro-industrial by-products and residues as sources of carbon and energy for microorganisms, mainly filamentous fungi, has been a common practice not only to recover compounds and apply on products of interest but also to reduce release of waste on the environment (Oliveira et al., Citation2011; Pandey et al., Citation2000).
Rhizopus oryzae stands out among safe filamentous fungi (generally recognized as safe [GRAS]) because of its intense metabolic activity so it has been commercially explored to yield enzymes and other compounds whose synthesis may be induced throughout cultivation (Kupski et al., Citation2015). In cookery, this microorganism has been used for improving sensory characteristics of texture and flavor of fruits and cereals (Cantabrana, Perise, & Hernandez, Citation2015). Therefore, it is an interesting option to improve functional and nutritional properties on lignocellulosic residues whose production is increasing as consequence of agro-industrial activities around the world (Kupski et al., Citation2012).
Solid-state fermentation of lignocellulosic material by R. oryzae releases hydrolytic exoenzymes which act especially on polymeric constituents of the substrate to use monomer units. Due to this depolymerizing action, compounds that derive from lignin, cellulose, proteins and other components of the vegetable structure are released into the biomass beside fungal metabolites (Kupski et al., Citation2015).
R. oryzae, a GRAS fungal species, has the ability to metabolize polymeric materials; however, to exploit this ability, the fermented biomass must be characterized chemically and functionally at each stage of fungal development and thus determine the parameters that increased yield of the target compound (Kupski et al., Citation2012; Oliveira et al., Citation2011; Schmidt, Goncalves, Prietto, Hackbart, & Furlong, Citation2014; Silveira & Furlong, Citation2007).
Demand for alternative sources of essential nutrients, such as amino acids and fatty acids, is increasing to meet the production of foods for special purposes. Agro-industrial by-products such as rice, soybean and wheat bran are good source of proteins or lipids; however, the bioaccessibility of them is limited for human and animals consumers. Therefore, as substrate for fungal development, under appropriated conditions may be a great solution for recovery essential compounds from lignocellulosic bran and apply on special food formulation.
This study aims to follow variation in the profile of essential amino acid and essential fatty acids along the solid state of soybean, wheat and rice bran by R. oryzae CCT 7560 (GRAS), isolated from rice crop of Brazilian south region, in order to identify promising parameter to recover them and apply as ingredients on food chain.
2. Material and methods
Soybean, wheat and rice bran was supplied by agro-industries located in Rio Grande do Sul state, Brazil.
The microorganism R. oryzae CCT 7560 was obtained from the Collection of Cultures at the Fundação André Tosello, located in Campinas, São Paulo, Brazil.
The standard methyl ester (Supelco® 37 Component FAME Mix) and amino acids were purchased from Sigma-Aldrich (USA).
2.1. Chemical characterization
The lipid fraction of the fungal biomasses yielded in the substrates was extracted and quantified by the method proposed by Folch, Lees and Stanley (Citation1957) with some adaptations. The sample (granulometry <0.56 mm) was homogenized by ultrasound agitation for 5 min with the mixture chlorophorm:methanol (2:1, v/v), 1:10 (m/v), centrifuged and partitioned with methanol:H2O (2:1, v/v). The separated organic phase was filtered by anhydrous sodium sulfate and the residue was kept in a laboratory oven for 2 h at 50°C, estimating the lipid percentage on dry bases.
The total nitrogen content of the biomasses collected at different cultivation intervals was determined by AOAC – Association of Official Analytical Chemists (Citation2000) method micro-Kjeldahl no. 920.87. The conversion factor was 6.25 to express the percentage of protein on dry bases. Samples moisture was determined by the procedures no. 934.01 (AOAC – Association of Official Analytical Chemists, Citation2000).
2.2. Genetic characterization and cultivation of R. oryzae
Genetic sequencing of R. oryzae CCT 7560 was evaluated by PCR with Primers ITS 1 (5′TCCGTAGGTGAACCTGCG3′) and ITS 4 (5′TCCTCCGCTTATTGATAT3′) (White, Runs, Lee, & Taylor, Citation1990). The sequence of nucleotides was applied to the software BioEdit v. 7.0.2 whereas identification was carried out in the database GenBank by BLASTN, with 100% complementarity with the database and E-value equal to 0.
Strains of the fungus R. oryzae were kept on an inclined plane at 4°C in potato dextrose agar (PDA). Spores were scraped by Tween 80 (0.2%) and yielded an aqueous emulsion. PDA was used for new spore incubation for 7 days at 30°C in Petri dishes. Spores were scraped with a Drigalski handle, counted with a Neubauer chamber (L. Opitik, Germany) and inoculated in fermentations (Oliveira et al., Citation2010; Schmidt & Furlong, Citation2012).
Cultivation of R. oryzae was carried out in tray reactors (12 × 8 × 4 cm3) in which soybean, wheat and rice bran with standardized granulometry (<0.56 mm) were arranged in 2-cm layers and then autoclaved. Substrates were homogenized with 45 mL nutrient solution (2 g L−1 KH2PO4, 1 g L−1 MgSO4, 1.8 g L−1 NH2CONH2 in HCl 0.4 N) and the humidity of the system was adjusted to 50% with sterile water before the beginning of cultivation (Oliveira et al., Citation2010).
A suspension of R. oryzae containing 4.0 × 106 spores g medium−1 was inoculated on reactors that were covered with sterile gauze to allow ventilation and then incubated at 30°C for 120 h. Sampling was conducted every 24 h and biomass was stored at −18°C up to the chemical determinations (Schmidt & Furlong, Citation2012).
2.3. Microscopic and chemical characterization of fungal biomass
The biomass resulting from R. oryzae on different substrates along cultivation was indicated by the determination of glucosamine content. Therefore, 0.1 g of the sample was hydrolyzed with HCl 6 M (p/v) and the sample of acetylglucosamine was spectrophotometrically determined at 530 nm with a standard curve of glucosamine (Sigma, USA) in water (from 1 to 17 mg mL−1) (Aidoo, Hendry, & Wood, Citation1981; Souza, Prietto, Ribeiro, Souza, & Badiale-Furlong, Citation2011).
Scanning electron microscopy (SEM) was employed to visualize the formation of hyphae in the different substrates. Samples of biomass were dried at 65°C, covered with gold particles and evaluated by a Scanning Electron Microscope Jeol, JSM-6610LV, equipped with energy dispersive spectrometer at 15 kV, Thermo Scientific, Ultra Dry, USA.
2.4. Amino acid profile along fungal development
Samples of homogenized fungal biomass were washed with Milli-Q water whereas the solid fraction was lyophilized. Ten milligram of sample was weighed and subjected to hydrolysis with 500 μL of 6 M HCl at 110°C for 24 h (Alaiz, Navarro, Giron, & Vioque, Citation1992). The residue was resuspended in Milli-Q water, filtered and lyophilized again. The sample was resuspended in 1 mL Milli-Q water and injected into a liquid chromatographer.
Amino acids were identified and quantified by high-performance liquid chromatographer (Shimadzu, LC-20AD, Tokyo, Japan) coupled to a fluorescence detector and a post-column derivatization device. Wave lengths for detection were 350 nm for excitation and 450 nm for emission. A Shim-pack Amino-Na Column (100 mm × 6 mm) with 45-min elution in gradient mode was used with two solutions prepared in Milli-Q water. One of the solutions was composed of a buffer of citric acid 0.2 mol L−1 pH 3.2 whereas the other one consisted of a buffer of citric acid, boric acid and sodium hydroxide 0.2 mol L−1 pH 10 (both). The post-column derivatization was performed from a buffer solution containing sodium carbonate (0.384 mol L−1), boric acid (0.216 mol L−1) and potassium sulfate (0.108 mol L−1). This buffer solution was divided into two parts, in one was added 1% sodium hypochlorite, and in the other N-acetyl-l-cysteine and orthophthalaldehyde. Identification of amino acids was carried out by comparing retention times of standards and quantification in analytical curves constructed for every amino acid.
2.5. Profile of fatty acids
Lipids were esterified by Metcalfe, Schimtz and Pelke (Citation1966) adapted method, submitted to esterification under reflux for 15 min with methanolic solution KOH 0.5 N catalyzed by BF3 whereas the solvent was evaporated under nitrogen flow. The residue was solubilized in 1 mL dichloromethane for further injection (1 μL) into a gas chromatographer.
Esterified fatty acids were identified and quantified by gas chromatographer GC-2010 Shimadzu Plus equipped with split-mode injector, capillary column Rtx®-1 (30 m × 0.25 mm ID × 0.25 μm df), flame ionization detector and GC solution software program. The stripping gas was helium whose flow rate had linear velocity of 1 mL min−1. The temperature of the injector and the detector was adjusted to 260°C. The initial temperature of the column was 50°C for 2 min; then, it was raised to 200°C at a rate of 6°C min−1. This temperature was kept for 4 min. After that, it was raised to 240°C at a rate of 3°C min−1 for 10 min. The identification of fatty acids profile was carried out by the comparison of retention times with the reference standards, and the quantification was performed by standardization of the peak areas (Massarolo, Souza, Ribeiro, Badiale-Furlong, & Souza-Soares, Citation2016).
2.6. Statistical analysis
All determinations were carried out in triplicate. The statistical analysis of the differences in significance promoted by the treatments of results was conducted by ANOVA and the Tukey’s test (p < 0.05) using the Statistica 7.0 software program.
3. Results and discussion
3.1. Chemical and microscopic characterization of bran
R. oryzae development on the substrates promoted changes on the contents of lipids and proteins (). Protein contents showed significant increase, i.e. 100% in wheat bran (24 h), whereas the increase in soybean and rice bran (72 h) was 65% and 77%, respectively. This behavior shows adaptation of the microorganism to the three substrates, even though at different velocity and biomass production patterns. Similar behaviors have been reported by cultivation of R. oryzae in rice bran, showing a 49% increase in protein content (Oliveira et al., Citation2010). In another study, cultivation with Aspergillus oryzae in wheat bran showed an increase of 11% in protein content (Silveira & Furlong, Citation2007). Other fungal species, such as Aspergillus niger and Trichoderma reesei, cultivated on lignocellulosic materials also shows an increase in the protein content on fermented biomass that can be used to formulate food (Silveira, Oliveira, Costa, & Kalil, Citation2006).
Table 1. Glucosamine content, protein percentage and lipids of Rhizopus oryzae biomass grown on soybean meal, wheat bran and rice bran.
Tabla 1. Contenido de glucosamina, porcentaje de proteínas y de lípidos en la biomasa de Rhizopus oryzae cultivada en harina de soya, salvado de trigo y salvado de arroz.
The fungal biomass on wheat bran showed a 1.6-fold increase in the lipid percentage (24 h); however, it decreased as cultivation went on. The biomass of rice bran had a 1.9-fold increase (120 h) by comparison with the beginning of cultivation. The biomass of soybean bran did not have significant variation in the lipid content along the experiment (p < 0.05).
Oliveira et al. (Citation2010) observed that R. oryzae cultivation on cereal bran promoted a decrease in lipid contents with cultivation time, thus showing that this behavior may be due to their use in the synthesis of mycelia components. In this study, a little decrease in lipids percentage was found in the soybean fungal biomass.
Variations found in the lipid and protein contents in fungal biomasses cultivated on different substrates were determined by considering the initial content of them. The increase reinforces that the microorganisms depolymerized then and synthesize their own components; consequently, they can be able to activate specific metabolic pathways to adapt to the medium.
The evolution of the fungal biomass was also followed by SEM images since they enable the development of fungal mycelia to be visualized. shows the mycelium ramifications in different substrates, by comparison with the beginning of cultivation. Likewise other genera of the order Mucorales, they grow fast and propagate by hydrophobic sporangiospores. They have tube-like hyphae and no formation of septa (Ma et al., Citation2009).
Figure 1. Scanning microscopy times of the fastest growing Rhizopus oryzae cultivation in soybean meal 0 h (1a), 24h (1b) and 48 h (1c); wheat bran 0 h (2a), 48h (2b) and 72 h (2c); rice bran 0 h (3a), 48h (3b) and 72 h (3c).
Figura 1. Periodos de microscopio de barrido de los cultivos de Rhizopus oryzae de crecimiento más rápido en harina de soya 0 h (1a), 24h (1b) y 48 h (1c); salvado de trigo 0 h (2a), 48h (2b) y 72 h (2c); salvado de arroz 0 h (3a), 48h (3b) y 72 h (3c).
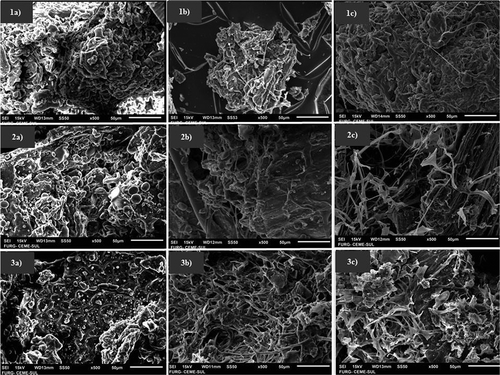
Taking into account that a microscope evaluation does not furnish good quantitative evaluation of fungal biomass evolution, glucosamine accumulated by fungal mycelia was determined in biomass before and along fungal grow. The composition of the substrate and the cultivation conditions affects the metabolic regulation and, consequently, the morphologic formation of the hyphae (Kroll, Pahtz, & Kniemeyer, Citation2014; Scotti, Vergoignan, Feron, & Durand, Citation2001). Glucosamine is present in the R. oryzae structure, whose hyphae have cellular walls composed of chitosan and chitin, rather than glucan and mannan which are typical of other species. Therefore, its content is a reliable indicator of the evolution of fungal mycelia (Souza et al., Citation2011).
Fungal mycelia formation was confirmed by the increase in glucosamine content (). Each bran reached its highest content at different intervals; similarly, it had been seen in the microscopy images and protein production matter. After 48 h of development, on rice and wheat bran substrates, glucosamine content was 9.83 and 7.45 mg, respectively. At 24 h, the highest glucosamine content (6.64 mg) was found on soybean bran substrate, similar to the maximum protein content. This difference, found in the period needed to reach the peak of exponential growth, also reinforces the role of the substrate composition in the evolution of fungal biomass. High protein content present in the soybean bran ended up promoting exponential growth in the shortest interval.
However, rice bran led not only to the highest glucosamine formation (48 h) but also to an increase on protein (72 h) and lipid (96 h) contents. Soybean bran fermented presented a maximum glucosamine content, lower than the ones found on rice and wheat bran, but there was considerable protein accumulation in the biomass and lipids kept constant. The same happened on lipid content in wheat bran.
The cereal bran that possess the chemical compounds essential to R. oryzae evolution and differentiation promoted the longest exponential phase. Therefore, soybean bran is the best substrate when the aim is to get a fast process to generate biomass with high protein content. Amino acids hydrolyzed by microorganism exoproteases may lead an increase in the velocity of the protein synthesis on fungal biomass.
3.3. Fatty acids profile
According to Silveira and Furlong (Citation2007), R. oryzae synthesizes its lipids from the nutrients found in the medium resulting in 33.6% oleic acid (C18:1 n9), 29.3% palmitic acid (C16:0), 14.6% linoleic acid (C18:2 n6), 8.3% stearic acid (C18:0) and 4.6% linolenic acid. However, the fact that few studies have focused on the profile of fatty acids yielded by fungal species on lignocellulosic substrate motivated the experiments carried out for this evaluation ().
Table 2. Fatty acids during the cultivation of Rhizopus oryzae soybean meal, rice and wheat.
Tabla 2. Ácidos grasos durante el cultivo de Rhizopus oryzae en harina de soya, arroz y trigo.
The main fatty acids found in rice, soybean and wheat bran were the same after R. oryzae cultivation: oleic, linoleic and palmitic acids. At quantitative point, a statistically significant increase (p < 0.05) was found in the percentages of linoleic acid in cis form (11.9% and 14.1%) when substrates were wheat and soybean bran. These substrates also show a decrease in the percentages of oleic acid (7% and 24%, respectively).
Along the experiment, the rice bran biomass yielded eicosenoic (C20:1 n9) and docosahexaenoic (C22:6 n3) acids in 72 h whereas eicosapentaenoic (C20:5 n3) acid was yielded in 96 h. However, all percentages were below 1%, a fact that suggests that these fatty acids are required as substrate for the microorganism structural organization. In wheat bran biomass, arachidonic acid (<0.1%) was detected at 72 h, whereas elaidic acid vanished at 48 h.
According to the Department of Health and Social Security in United Kingdom (Department of Health, Citation1994), the relation between unsaturated and saturated fatty acids (FAU/FAS) in any food is very important for the balance of human cellular metabolism. Therefore, FAU/FAS ratios below 0.45 are not really advisable to health since they may cause heart disorders. R. oryzae biomass in the three bran ratios between 3.4 and 4.9 was found. Thus, they are potential compounds for human diet. Rice bran fermented for 72 h yield an increase of 10% in relation to 0 h and the ratio FAU/FAS became 4.4. In wheat and soybean bran, R. oryzae cultivation was not promising because there were about 15% (24 h) and 17% (120 h) decreases in FAU/FAS, respectively, even though these amounts kept above 0.45. In wheat bran, there was an increase between the lipid content and the FAS happened at 24 h. Although the same behavior was also observed in soybean bran, the total lipid content did not show significant difference in relation to the bran before fermentation.
Regarding nutrition, the beneficial relation ω6/ω3 is in the order of 4:1–10:1. Along rice bran fermentation by R. oryzae, there was a decrease (43%) in this ratio after 120 h, a fact caused by ω3 release, following the increase in the lipid content observed in this period. Although there was a decrease in the numerical value of the ratio, it is still considered above the ideal range (4:1–10:1). It should be pointed out that some countries are planning to change this ratio to 50:1 (Oliveira et al., Citation2011; Simopoulos, Citation2004). In the wheat bran biomass, there was a decrease (35%) in the ω3/ω6 ratio; it still complies with what has been established by most countries. The soybean bran biomass was the only one in which these ratios were below 4:1, a fact that may contribute to the development of allergic, inflammatory and cardiovascular diseases (Martim et al., Citation2006).
shows the lipid profile during the R. oryzae development on the substrate. Solid-state fermentation promotes an essential fatty acids quality improvement on soybean bran, 20% and 24% at 72 and 96 h of fermentation, respectively. Whereas rice bran fermentation by the fungi promoted an increase in the total lipid content, the essential fatty acids were not improved and including were reduced along the time. An improvement of essential fatty acids distribution on bran fermentation was observed; however, the yield was bigger on rice bran. It is important to mention that soybean bran was defatted before fermentation, suggesting it as a strategy for better lipids recover. In wheat bran fermented, the essential fatty acids were improved at 72 h (14%). From a technological point of view, the quality of the lipid extracted improves with the time of cultivation on soybean and wheat bran, but the yield is much higher on rice bran.
Figure 2. Quality (a) and yield (b) of essential fatty acids (ω3 and ω6) during cultivation of Rhizopus oryzae in rice bran, soybean meal and wheat bran.
Figura 2. Calidad (a) y rendimiento (b) de ácidos grasos esenciales (ω3 y ω6) durante el cultivo de Rhizopus oryzae en salvado de arroz, harina de soya y salvado de trigo.
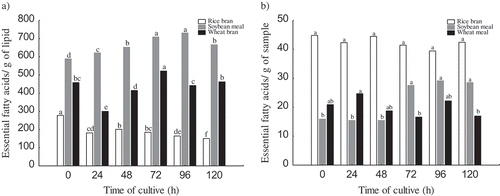
R. oryzae cultivated on rice, wheat and soybean bran is promising since it led to increase in ω3 in 72%, 64% and 58%, respectively. However, ω6-type fatty acids do not undergo strong changes in their contents along the cultivation process in any substrate. It may indicate a metabolic characteristic of fungus species as answer to the nutrients disponibility; however, this property has been explored.
3.4. Amino acids profile
The protein nutritional importance is determined mainly by its essential amino acid composition. The way in which fungal species metabolize their proteins from the composition of the medium has been little investigated; however, this may be interesting to improve the protein biological value. Therefore, this study took into account that the different protein sources used for solid-state fermentation by R. oryzae could affect the development and the profile of amino acids essential in the fungal biomass (). In order to investigate this possibility, were determined in the biomasses threonine (Thr), valine (Val), isoleucine (Ile), leucine (Leu), phenylalanine (Phe), lysine (Lys), histidine (His) and methionine (Met).
Table 3. Amino acid essential profile during cultivation of Rhizopus oryzae soybean meal, wheat and rice.
Tabla 3. Perfil de aminoácidos esenciales durante el cultivo de Rhizopus oryzae en harina de soya, trigo y arroz.
Changes in the amino acid content were compared to the profile of the medium before microbial effect (0 h). The soybean bran fungal biomass showed an increased in Met (98%/72 h), Val (34%), Leu (19%), Lys (6%) and Phe (2%) after 96 h, besides the decrease in His and Ile (6% and 27%). In the rice bran fungal biomass, there was a significant decrease (p < 0.05) in Val (52%), Leu (68%), Phe (82%), His (89%), Ile (100%), Lys (43%), Cys2 (17%) and Met (20%) after 72 h; it coincided with the peak of protein content. In wheat bran, fungal biomass showed a decrease in Leu content (73%/120 h). However, there was increase in Thr (100%/24 h), Lys (43%), Met (94%) and His and Ile (100%) after 72 h, besides Val (100%/96 h).
shows the essential amino acids and protein yield along solid fermentation by R. oryzae.
Figure 3. Quality (a) and yield (b) of essential amino acids during cultivation of Rhizopus oryzae on wheat bran (1), rice bran (2) and soybean meal (3).
Figura 3. Calidad (a) y rendimiento (b) de aminoácidos esenciales durante el cultivo de Rhizopus oryzae en salvado de trigo (1), salvado de arroz (2) y harina de trigo (3).
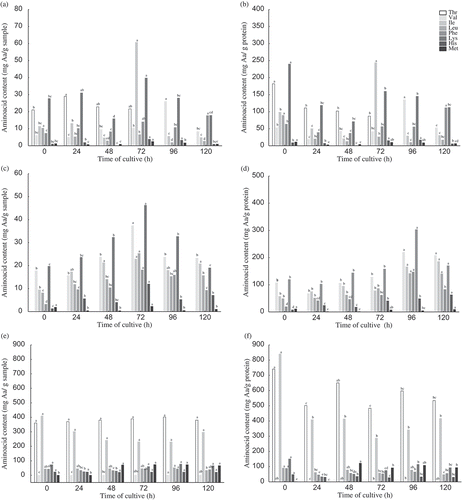
The major yield of essential amino acids per protein on fermented biomass was observed when it was used rice bran as substrate at 72 h ()). The yield was variable along the fermentation process where wheat bran was the substrate. The more variation was observed to Ile, Lys, His and Met level at 72 h ()). Essential amino acid profile in soybean bran under fermentation by R. oryzae (96 h) showed the bigger yield (p < 0.05) ()), but the protein reduced along the fermentation time ()). The best amino acid balance happened in fungal biomass of soybean bran after 96 h and in cultures of wheat and rice bran after 72 h due to the variety versus content of essential amino acids.
Furthermore, the comparison between these compositions and the profile of amino acids of eggs, which for a long time was considered the standard protein by amino acid composition, shows that soybean bran stands out because it presents the appropriated level of all essential amino acids (FAO, Food and Agriculture Organization of the United Nations, Citation1970). The solid fermentation by R. oryzae increased this content, since it got higher than the one found in eggs, except for Val.
Solid-state fermentation by R. oryzae is an alternative to increase the amino acid content in lignocellulosic substrates, mainly in soybean and wheat bran. Soybean bran, whose limiting amino acid is methionine, had its amino acid balance improved by its synthesis during microorganism development. The other substrates increased not only the amounts of essential amino acids but also the content of their essential amino acid profile, i.e. lysine.
The cultivation of R. oryzae on agro-industrial residues increases the availability of nutrients through changes that result from its metabolic activity, yielding interesting compounds such as essential amino acids and fatty acids (Oliveira et al., Citation2011; Silveira & Furlong, Citation2007). These compounds are important for human and animal diets, since they are not synthesized, and are required to the metabolism regulation (Martim et al., Citation2006). Besides, to recover, it is interesting to apply them in special foods formulations because it is possible to control the essential components profile by fermentation conditions.
4. Conclusion
Rice bran was the most promising substrate to generate fungal biomass and lipid accumulation, which kept almost constant in the other substrates. Although the lipid content increased in rice bran, the profile of fatty acids underwent changes in its ω3 fatty acids content whereas ω6 fatty acids were almost constant during the process interval in all substrates. The nutritional quality of the lipid improved by fermentation time in soybean meal and wheat bran; however, the yield is higher in rice bran biomass. Soybean bran amino acid balance was improved by its synthesis of its limiting amino acid methionine. The other substrates increased not only the amounts of essential amino acids but also the content of their limiting one, i.e. lysine. Soybean meal fermented by R. oryzae at 96 h is the best source of essential fatty acids and essential amino acids.
Disclosure statement
No potential conflict of interest was reported by the authors.
References
- Aidoo, K.E., Hendry, R., & Wood, B.J.B. (1981). Estimation of fungal growth in a solid-state fermentation system. European Journal of Applied Microbiology and Biotechnology, 12(1), 6–9. doi:10.1007/Bf00508111
- Alaiz, M., Navarro, J.L., Giron, J., & Vioque, E. (1992). Amino-acid-analysis by high-performance liquid-chromatography after rerivatization with diethyl ethoxymethylenemalonate. Journal of Chromatography A, 591(1–2), 181–186. doi:10.1016/0021-9673(92)80236-N
- AOAC – Association of Official Analytical Chemists. (2000). Official methods of analysis (17th ed.) [CD]. Willian Horwitz.
- Cantabrana, I., Perise, R., & Hernandez, I. (2015). Uses of Rhizopus oryzae in the kitchen. International Journal of Gastronomy and Food Science, 2(2), 103–111. doi:10.1016/j.ijgfs.2015.01.001
- Department of Health. (1994). Nutritional aspects of cardiovascular disease. Report on health and social subjects (Vol. 46, pp. 37–46). London: Her Majesty’s Stationer Office (HMSO.
- FAO, Food and Agriculture Organization of the United Nations. (1970). Amino acid content of foods. (Vol. 24). Rome: FAO. Retrieved from http://www.fao.org/docrep/005/AC854T/AC854T00.htm
- Folch, J., Lees, M., & Stanley, G.H.S. (1957). A simple method for the isolation and purification of total lipides from animal tissues. Journal of Biological Chemistry, 226(1), 497–509.
- Kroll, K., Pahtz, V., & Kniemeyer, O. (2014). Elucidating the fungal stress response by proteomics. Journal of Proteomics, 97, 151–163. doi:10.1016/j.jprot.2013.06.001
- Kupski, L., Cipolatti, E., Rocha, M., Oliveira, M.D., Souza-Soares, L.D., & Badiale-Furlong, E. (2012). Solid-state fermentation for the enrichment and extraction of proteins and antioxidant compounds in rice Bran by Rhizopus oryzae. Brazilian Archives of Biology and Technology, 5(6), 937–942. doi:10.1590/S1516-89132012000600018
- Kupski, L., Silvello, M.A.D., Fontes, M.R.V., Lima, T.S., Treichel, H., & Furlong, E.B. (2015). R. Oryzae Cellulases: A New Approach to Degrading lignocellulosic material. Journal Food Biochemistry, 39(2), 129–138. doi:10.1111/jfbc.12097
- Ma, L.J., Ibrahim, A.S., Skory, C., Grabherr, M.G., Burger, G., Butler, M., … Wickes, B.L. (2009). Genomic analysis of the basal lineage fungus Rhizopus oryzae reveals a whole-genome duplication. Plos Genet, 5(7). doi:10.1371/journal.pgen.1000549
- Martim, C.A., Almeida, V.V., Ruiz, M.R., Visentanier, J.E.L., Matshushita, M., Souza, N.E., & Visentainer, J.V. (2006). Omega-3 and omega-6 polyunsaturated fatty acids: Importance and occurrence in foods. Revista De Nutrição, 19(6), 761–770. doi:10.1590/S1415-52732006000600011
- Massarolo, K.C., Souza, T.D., Ribeiro, A.C., Badiale-Furlong, E., & Souza-Soares, L.A. (2016). Influence of cultivation Rhizopus oryzae on rice bran on lipid fraction: Fatty acids and phospholipids. Biocatalysis and Agricultural Biotechnology, 8, 204–208. doi:10.1016/j.bcab.2016.10.002
- Metcalfe, L.D., Schimtz, A.A., & Pelke, J.R. (1966). Rapid preparation of fatty acid esters from lipids for gas liquid chromatography. Analytical Chemistry, 38, 514–515. doi:10.1021/ac60235a044
- Oliveira, M.D., Feddern, V., Kupski, L., Cipolatti, E.P., Badiale-Furlong, E., & Souza-Soares, L.A. (2010). Physico-chemical characterization of fermented rice bran biomass. Cyta-Journal of Food, 8(3), 229–236. doi:10.1080/19476330903450274
- Oliveira, M.D., Feddern, V., Kupski, L., Cipolatti, E.P., Badiale-Furlong, E., & Souza-Soares, L.A. (2011). Changes in lipid, fatty acids and phospholipids composition of whole rice bran after solid-state fungal fermentation. Bioresource Technology, 102(17), 8335–8338. doi:10.1016/j.biortech.2011.06.025
- Pandey, A., Soccol, C.R., Nigam, P., Soccol, V.T., Vandenberge, L.P.S., & Mohan, R. (2000). Biotechnological potential of agro-industrial residues. II: Cassava bagasse. Bioresource Technology, 74(1), 81–87. doi:10.1016/S0960-8524(99)00143-1
- Schmidt, C.G., & Furlong, E.B. (2012). Effect of particle size and ammonium sulfate concentration on rice bran fermentation with the fungus Rhizopus oryzae. Bioresource Technology, 123, 36–41. doi:10.1016/j.biortech.2012.07.081
- Schmidt, C.G., Goncalves, L.M., Prietto, L., Hackbart, H.S., & Furlong, E.B. (2014). Antioxidant activity and enzyme inhibition of phenolic acids from fermented rice bran with fungus Rhizopus oryzae. Food Chemistry, 146, 371–377. doi:10.1016/j.foodchem.2013.09.101
- Scotti, C.T., Vergoignan, C., Feron, G., & Durand, A. (2001). Glucosamine measurement as indirect method for biomass estimation of Cunninghamella elegans grown in solid state cultivation conditions. Biochemical Engineering Journal, 7(1), 1–5. doi:10.1016/S1369-703x(00)00090-5
- Silveira, C.M., & Furlong, E.B. (2007). Characterization of nitrogenated compounds in solid state fermented bran. Ciência E Tecnologia De Alimentos, 27(4), 805–811. doi:10.1590/S0101-20612007000400021
- Silveira, S.T., Oliveira, M.S., Costa, J.A.V., & Kalil, S.J. (2006). Optimization of glucoamylase production by Aspergillus niger in solid-state fermentation. Applied Biochemistry and Biotechnology, 128(2), 131–139. doi:10.1385/Abab:128:2:131
- Simopoulos, A.P. (2004). Omega-6/omega-3 essential fatty acid ratio and chronic diseases. Journal Food Reviews International, 20(1), 77–90. doi:10.1081/FRI-120028831
- Souza, M.M., Prietto, L., Ribeiro, A.C., Souza, T.D., & Badiale-Furlong, E. (2011). Assessment of the antifungal activity of Spirulina platensis phenolic extract against Aspergillus flavus. Ciência E Agrotecnologia, 35(6), 1050–1058. doi:10.1590/S1413-70542011000600003
- White, T.J., Runs, T., Lee, S., & Taylor, J. (1990). A guide to methods and applications (pp. 315–322). New York: Academic Press. Amplification and direct sequencing of fungal ribosomal RNA genes for phylogenetics. PCR Protocols.