ABSTRACT
Extensive proteolysis and formation of flavor substances occur during the processing of dry-salted fish due to the action of endogenous proteases. This study analyzed the evolution of the endogenous proteases and the relationship between endogenous protease levels and protein degradation during processing. Cathepsin B and L activity increased significantly during the last two stages, while cathepsin H activity decreased non-significantly (residual activity was 92.27%) compared to initial activity levels in fresh fish. Dipeptidyl peptidase (DPP I, IV) activity decreased overall. The activity of three aminopeptidases (arginine aminopeptidase; alanyl aminopeptidase; leucyl aminopeptidase) initially decreased (P < 0.05) then significantly increased (P < 0.05) during the final stage. Principal component analysis indicated that cathepsins B and L had larger influences than cathepsin H on protein degradation and flavor substance formation in dry-salted fish, but the influence of dipeptidyl peptidases and aminopeptidases on protein degradation and flavor substance formation was limited.
RESUMEN
Durante el procesamiento de pescado seco salado se produce una amplia proteólisis y la formación de sustancias de sabor debido a la acción de proteasas endógenas. El presente estudio analizó cómo evolucionan éstas y la relación existente entre sus niveles y la degradación proteínica durante el procesamiento. La actividad de las catepsinas B y L se elevó de manera significativa durante las dos últimas etapas del procesamiento, mientras que la actividad de la catepsina H disminuyó de manera no significativa (la actividad residual fue 92,27%), en comparación con los niveles de actividad iniciales que presentan en pescado fresco. En general, la actividad de la dipeptidil peptidasa se redujo. Inicialmente, se produjo un descenso de la actividad de tres aminopeptidasas (arginina aminopeptidasa: RAP; alanil aminopeptidasa: AAP; leucil aminopeptidasa: LAP), (p < 0,05), para aumentar significativamente (p < 0,05) durante la última etapa. Aunque el análisis de los componentes principales indicó que las catepsinas B y L ejercieron más influencia que la catepsina H en la degradación proteínica y en la formación de sustancias de sabor en pescado seco salado, la influencia de las dipeptidil peptidasas y las aminopeptidasas en la degradación proteínica y en la formación de sustancias de sabor fue limitada.
1. Introduction
Due to its unique flavor and resistance to storage, dry-salted fish has been favored by consumers deeply. The flavors in dry-salted fish come from variety pathways, such as lipolysis-oxidation, protein degradation, and the Maillard reaction. In the processing of ham, protein degradation can produce large amounts of peptides and free amino acids (FAAs). Small peptides and FAAs can cause bitterness, sweetness, or freshness, and some FAAs and small peptides are precursors to compounds that give ham its flavor (Careri et al., Citation1993). The endogenous proteases in pickled products that can degrade proteins into polypeptides and FAAs are cathepsins, calpains, dipeptidases, and aminopeptidases (Gallego, Mora, Fraser, Aristoy, & Toldrá, Citation2014; Toldra, Aristoy, & Flores, Citation2000). Endogenous cytoplasmic calpains (both u- and m-calpains) and lysosomal cathepsins B, D, H, and L play important roles in post-mortem proteolysis of myofibrils and concomitant meat softening (Ahmeda, Donkora, Street, & Vasiljevic, Citation2015). Cathepsin B plays an important role in textural changes in fish tissue. Chéret, Delbarre-Ladrat, Lamballerie-Anton, and Verrez Bagnis (Citation2007) found that the activity of cathepsins B and L was 29.7 and 4 times higher in fish muscle than in bovine meat, respectively. Calpain and cathepsin D were not active during ham processing; however, the activities of cathepsins B, L, and H were detected throughout the course of processing (Fidel & David, Citation1988; Sárraga, Gil, & García-Regueiro, Citation1993). Four major dipeptidases (DPP I, II, III, and IV) have been identified from porcine skeletal muscle. DPP I and IV had high activity during the processing of ham (Angel & Toldra, Citation2001). Aminopeptidases in ham could degrade proteins and peptides to FAAs (Toldra, Flores, & Sanz, Citation1997). Toldrá et al. (Citation1992) found that alanyl aminopeptidase (AAP), arginine aminopeptidase (RAP), leucyl aminopeptidase (LAP), tyrosinophilin aminopeptidase and pyroglutamine aminopeptidase had a high activity in the curing phase of ham. Toldra, Cerverro, and Part (Citation1993) found that AAP, RAP, and LAP remained highly active throughout ham processing by simulating the processing conditions of dry-cured ham.
Based on the above discussion, the research into endogenous proteases related to protein degradation and flavor substance formation mainly focused on the processing of dry-salted ham. However, there have been no reports on the role of endogenous protease activity in aquatic products. The objective of our study was to analyze the evolution of the endogenous proteases (cathepsin B, cathepsin L, cathepsin H, DPP I, DPPIV, AAP, RAP, LAP), the parameters of physical and chemical indicators (water content, salt content, and pH values), the level of protein degradation indicators (nitrogen, PN; non-protein nitrogen, NPN; protein hydrolysis rate, PI), and FAAs during the processing of dry-salted fish. Furthermore, the relationship between endogenous proteinases activity and protein degradation in dry-salted fish was explored by principal component analysis (PCA) using SPSS.
2. Materials and methods
2.1. Salted fish processing and sampling
Chilled round scad (Decapterus maruadsi) were purchased from a local supermarket with live weights of 100 ± 5 g. Dry-salted (20% salt (w/w)) fish was prepared as follows. Salt was evenly spread on the fish, saturated salt water was used to cover the fish, and they were placed in an incubator at 4°C for 36 h. After the wet-cured procedure was complete, the fish were soaked in water for 1 h. This desalination process was repeated for a total of three or four water soaks. Then, fish were air dry-ripened at 28 ± 2°C with 10% relative humidity (flowing air speed, 6 m/s) until the water content of the salted fish was approximately 40%. Specimens were sampled at five points: raw fish (sample A), end of salting (sample B), after desalination (sample C), during low temperature heat pump drying (sample D), and the finished product (sample E). Approximately 10 fish were sampled at each point. Samples were vacuum-packed and stored at −80°C until analysis.
2.2. Endogenous proteinases extraction and activity assays
2.2.1. Cathepsin (B and L) activity assay
A crude cathepsin solution was extracted according to the method described by Garcia-Garrido, Quiles-Zafra, Tapiador, and Luque (Citation2000), and Rosell and Toldrá (Citation1998). The activity of cathepsin B and activity of cathepsins B and L combined were measured as described by Zhao, Zhou, Tian, et al. (Citation2005), and Ge, Xu, Xia, Jiang and Jiang (Citation2016) with few modifications. A portion of the enzyme solution (0.5 mL) was diluted with 0.25 mL reaction buffer (cathepsin B buffer: pH 6.0, containing 352 mmol/L KH2PO4, 4 mmol/L Na2EDTA,48 mmol/L Na2HPO4, 8 mmol/L L-Cys; cathepsin B + L buffer: pH 5.5, containing 340 mmol/L NaAc, 4 mmol/L Na2EDTA, 60 mmol/L acetic acid, 8 mmol/L DTT). The homogenous solution was preheated to 40°C for 5 min using a water bath. Finally, 0.5 mL of substrate buffer (cathepsin B: Z-Arg-Arg-7-amido-4-methylcoumarin; cathepsin B + L: Z-Phe-Arg-7-amido-4-methylcoumarin; 20 µmol/L; Sigma, St. Louis, MO, USA) was added. After incubation at 40°C for 15 min, the reaction was quenched with 2 mL of termination reagent (pH 4.3, 100 mmol/L ClCH2COONa, 70 mmol/L acetic acid, 30 mmol/L NaAc). For the control group, the termination reagent was added before the other solutions. Fluorescence was measured using a fluorescence spectrophotometer (Cary Eclipse, Varian, America) (λex = 340 nm, λem = 440 nm). The activity of cathepsin L = (cathepsin B + L) − (cathepsin B).
2.2.2. Cathepsin H activity assay
L-Arg-AMC can be hydrolyzed by aminopeptidase and cathepsin H, and cysteine protease-specific irreversible inhibitors (E-64) can inhibit cathepsin H activity. The determination of cathepsin H is slightly different than the others. The procedure is as follows: group (A): A portion of the enzyme solution (0.5 mL) was diluted with 20 µL E-64(1 mmol/L) and 0.25 mL reaction buffer (cathepsin H buffer containing 200 mmol/L KH2PO4, 200 mmol/L Na2HPO4, 4 mmol/L Na2EDTA, 8 mmol/L L-Cys; pH 6.8). The homogenous solution was preheated for 60 min at 40°C using a water bath; group (B): A portion of the enzyme solution (0.5 mL) was diluted with 0.25 mL cathepsin H buffer, and the homogenous solution was preheated for 5 min at 40°C using a water bath. Then, 0.5 mL of substrate buffer (cathepsin H: L-Arg-7-amido-4-methylcoumarin 20 μmol/L; Sigma, St. Louis, MO, USA) was added to groups (A) and (B). The next steps are the same as above (2.3.2). Cathepsin H activity = group (A) − group (B).
Cathepsin activity was calculated using a standard curve. One unit of activity (U) was defined as the amount of enzyme hydrolyzing 1 nmol of 4-methylumbelliferyl-oleate per min at 40°C. Enzyme activity is expressed in (U)/g fish.
2.2.3. Dipeptidyl peptidase I and IV activity assay
A crude dipeptidyl peptidase (DPP) solution was extracted according to the method described by Sentandreu and Toldra (Citation2001). The activities of DPP I and IV were measured as described by Bolumar, Bindricha, Toepfl, Toldrá, and Volker Heinz (Citation2014) and Zhao, Zhou, Tian, et al. (Citation2005), with little modification. A portion of the enzyme solution (0.5 mL) was diluted with 0.25 mL of reaction buffer (DPP I: pH 8.0, 50 mmol/L sodium citrate buffer containing 0.5 mmol/L DTT; DPP IV: pH 8.0, 200 mmol/L Tris–HCI buffer; containing 5 mmol/L DTT). The homogenous solution was preheated for 5 min at 40°C using a water bath. Finally, 0.5 mL of substrate buffer (DPP I: H-Gly-Arg-7-amido-4-methylcoumarin, 200 mmol/L Tris–HCI: H-Gly-Pro-7-amido-4-methylcoumarin, 20 µmol/L; Sigma, St. Louis, MO, USA) was added. After incubation at 40°C for 15 min, the reaction was quenched with 5 mL anhydrous ethanol. For the control group, the ethanol was added before the other solutions. Fluorescence was measured using a fluorescence spectrophotometer (λex = 340 nm, λem = 440 nm).
2.2.4. Aminopeptidase activity assay
A crude aminopeptidase solution was extracted according to the method described by Rosell and Toldrá (Citation1998). Aminopeptidase (RAP; AAP; LAP) activity was measured as described by Bolumar et al. (Citation2014), with little modification. A portion of the enzyme solution (0.5 mL) was diluted with 0.25 mL reaction buffer (AAP: pH 7.0, 100 mmol/L sodium phosphate buffer containing 0.33% of 30% Brij 35, 5 mmol/L CaCl2, 1 mmol/L DTT; RAP: pH 6.5, 50 mmol/L sodium phosphate buffer containing 0.33% of 30% Brij 35, 1 mmol/L DTT, 150 mmol/L NaCl; LAP: pH 6.5, 50 mmol/L sodium phosphate buffer containing 0.33% of 30% Brij 35, 1 mmol/L DTT, 5 mmol/L MgCl2). The homogenous solution was preheated for 5 min at 37°C using a water bath. Finally, 0.5 mL of substrate buffer (AAP: Ala-7-amido-4-methylcoumarin; RAP: Arg-7-amido-4-methylcoumarin; LAP: Leu-7-amido-4-methylcoumarin, 20 µmol/L; Sigma, St. Louis, MO, USA) was added. After incubation at 37°C for 15 min, the reaction was quenched with 5 mL anhydrous ethanol. For the control group, the ethanol was added before other solutions. Fluorescence was measured using a fluorescence spectrophotometer (λex = 340 nm, λem = 440 nm).
Proteinases activity was calculated using a standard curve. One unit of activity (U) was defined as the amount of enzyme hydrolyzing 1 nmol 4-methylumbelliferyl-oleate per min at 40°C. Enzyme activities are expressed in (U)/g fish.
2.3. Determination of protein degradation index and FAAs
NPN (%): A portion of the sample (2.00 g) was mixed with 20 mL of ultra-pure water. The solution was heat shocked at 40°C, then 20 mL of 10% TCA solution was added. The new homogenate was continuously shocked for 40 min then centrifuged (10,000g) for 15 min at 4°C using a refrigerated centrifuge (Sigma 3k30, Germany). The supernatants were filtered through glass wool, and the filtrates were collected for further assays. The NPN content of the filtrate was assayed with 3 g catalyst and 12 mL of H2SO4 using a protein autoanalyzer. Total nitrogen (TN; %): A 2.00-g portion of the sample was assayed with 3 g catalyst and 12 mL of H2SO4 using a protein autoanalyzer. Protein Degradation Index (PI) = NPN(%)/TN(%) × 100. FAAs were measured as described by Stavropoulou, Borremans, De Vuyst, De Smet, and Leroy (Citation2015) and Berardo et al. (Citation2017).
2.4. Determination of physiochemical index
Moisture content was measured as described by ISO 1442:1997(E). Salt content was measured as described by the ISO recommended methods 1841-1 (ISO, 1996E). Measurements of pH were taken as described by Jin et al. (Citation2010).
2.5 Statistical analysis
All the data in this study were analyzed using a one-way variance with SPSS statistical software (Version 13, SPSS Inc., Chicago, IL, USA). The level of significance was set at P < 0.05. The relationship between enzyme activity and protein degradation during salted fish processing was analyzed by PCA using SPSS statistical software.
3. Results and discussion
3.1. Endogenous proteinases activity changes during the process of dry-salting fish
The changes in endogenous proteinases activity in the processing of dry-salted fish are shown in . Cathepsins B, L, and H demonstrated activity at all stages. In the final product, the activity of cathepsins B and L increased significantly. This was due to the lysosomes in fish tissue being more likely to rupture, which resulted in more lysosomal proteases being release. Cathepsin H activity decreased but not significantly (residual activity was 92.27%) compared to initial activity levels in fresh fish. Sárraga et al. (Citation1993) found that the activities of cathepsins B, L, and H had decreased substantially by the end of the ham processing (residual activity is only 5–15%).
Table 1. Changes in cathepsin (B, L, H), dipetidyl peptidase, and aminopeptidase activities during processing.
Tabla 1. Cambios en la actividad de las catepsinas (B, L, H), la dipeptidil peptidasa y la aminopeptidasa durante el procesamiento.
Cathepsin B activity changed, but not significantly (P > 0.05), over stages A, B, and C and then increased significantly (P < 0.05) over stages D and E. Cathepsin L activity increased significantly (P < 0.05) at stage B, decreased at stage C, than increased substantially (P < 0.05) over stages D and E. Cathepsin H activity decreased significantly at stage B, then increased gradually in the following steps. The activity of cathepsin B in the process was the most stable. Parreño, Cussó, Gil, and Sárraga (Citation1994) found similar results during the manufacturing process of Spanish dry-cured ham. Zhao, Zhou, Wang, et al. (Citation2005) found that cathepsin L was more stable than cathepsin B during the processing of Jinhua ham. In our study, cathepsin H demonstrated the highest activity throughout the entire processing, followed by cathepsin L, with cathepsin B exhibiting the lowest. In contrast to our conclusion, Flores, Aristoy, Antequera, Barat, and Toldrá (Citation2012) and Flores, Aristoy, Antequera, Barat, and Toldrá (Citation2009) found that cathepsin B + L demonstrated the highest activity, followed by cathepsin B + H, and cathepsin H was found to have the lowest activity throughout the entire process of manufacturing Iberian dry-cured ham.
The physicochemical factors (such as temperature, salt content, and pH) have complex effects on DPP I and IV during the processing of Jinhua ham, and the enzyme activity is controlled by many factors (Zhao, Zhou, Tian, et al., Citation2005). As seen in , although the processing of dry-salted fish could inhibit DPP I and IV activity, DPP I and IV demonstrated activity at all stages. The development of DPP I and IV activity in processing was different. DPP I activity significantly decreased at stage C (P < 0.05) and remained stable at stage C, D, and E. DPP IV activity significantly decreased at stage B (P < 0.05) and then increased at stage E. In general, DPP I and IV activity decreased over the whole processes, and the residual activities in final product was 49.58% and 42.44%, respectively. Flores et al. (Citation2009) also reached a similar conclusion in their investigation into Iberian ham processing. DPP I activity in dry-salted JinHua ham increased significantly (i.e. 64% compared to raw meat), and the residual DPP IV activity was 4.81% in JinHua ham (Zhao, Zhou, Tian, et al., Citation2005).
All three of the aminopeptidases (AAP, LAP, RAP) demonstrated activity during the processing of dry-salted fish, and three kinds of enzyme activities were also detected in dry-cured ham (Flores et al., Citation2012; Toldra et al., Citation2000; Zhao et al., Citation2006, Zhao, Zhou, Tian, et al., Citation2005). The AAP, LAP, and RAP activities were 54%, 62.35%, and 75% compared with the levels in fresh fish. The results showed that RAP was the most stable during the processing of dry-salted fish. The changes in AAP, LAP, and RAP were similar during the manufacturing process. The three aminopeptidase activities initially decreased (P < 0.05) and then significantly increased (P < 0.05) by stage C. The activity in the samples decreased markedly at stage D and significantly increased (P < 0.05) by the final stage. These strong trends in aminopeptidase activity showed the environment during the processing of dry-salted fish greatly influenced the activity of aminopeptidases. Zhao et al. (Citation2006) found that salinity, water, and temperature had a complex effect on aminopeptidase activity during the processing of Jinhua ham.
3.2. Changes in protein degradation and physicochemical index during the processing of dry-salted fish
Studies have found that the characteristic flavor of ham has a close relationship with peptide composition and content (Hansen-Mller & Hinrichsen, Citation1997). Severe protein hydrolysis causes hams to taste bitter because some small peptides with lipophilic side chains can be bitter (Sforza et al., Citation2001). The extent of protein hydrolysis can be expressed as a proteolytic index (PI). shows the variation in NPN, total protein nitrogen (PN), and proteolysis index (PI) during the processing of dry-salted fish. The per cent PN gradually increased with the decreasing moisture content in the whole process. The per cent NPN increased with decreasing water content and protein hydrolysis. PI values increased throughout the whole process. In their study of Parma ham, Careri et al. (Citation1993) found that insufficient protein degradation (PI < 22) made the ham aroma inadequate, and excessive protein degradation (PI > 30) gave hams a noticeable bitterness or metallic aftertaste. In our study, the PI value was 23.99 (22 < PI < 30) indicating no obvious bitterness or metallic aftertaste in the final product. In general, the moisture content decreased during the most of processing stages; in contrast, the salt content increased. Moisture content decreased from 77.18 ± 0.62% (fresh fish) to 45.44 ± 0.32% (final product); salt content increased significantly (P < 0.05) during the salting and air-drying stages. The average salt content of the final product was 8.55 ± 0.1 g/100 g fish.
Table 2. Changing of protein degradation and physicochemical index of Decapterus maruadsi during different methods of dry salted processing.
Tabla 2. Cambios en la degradación proteínica y el índice fisicoquímico de Decapterus maruadsi con la aplicación de distintos métodos del procesamiento destinados a producir pescado seco salado.
3.3. The change in FAAs during the processing of dry-salted fish
For some water-soluble FAAs (Thr, Ser, Glu, Pro, Gly, Ala, Met, Lys, His, Arg) dissolved into saturated brine, TFAA content significantly (P < 0.05) decrease at stage B and C (). However, TFAA content increased significantly (P < 0.05) during stages D and E, indicating that the FAAs in dry-salted fish were mainly produced in the last two stages. The content of caspase, leucine, and arginine was 3.03, 4.26, and 5.30 times higher in final product than in fresh fish. In the study of ham, Toldra et al. (Citation1997) found that FAAs were mainly produced by the hydrolysis of peptides and proteins under the action of aminopeptidase. FAAs were the precursor substances of ham flavoring substances (such as sour, sweet, and bitter) and therefore have a strong correlation with the formation of ham flavoring substances (García, Díez, & Zumalacárregui, Citation1998; Toldra et al., Citation2000). Alanine has a sweet taste, glutamic acid and aspartic acid taste umami. Short peptides contained methionine, leucine, and isoleucine could hydrolyze and release of FAAs thus reduce bitter taste. The percentage of flavored FAAs (PFAA) that contribute to the taste increased steadily with the processing time. PFAA was 2.90 times higher in final product than in fresh fish. The level of FAA (methionine, leucine, and isoleucine) that contributes to reduce the bitterness (BFAA) increased in stages B, D, and E. The level of fresh amino acids (DFAA) increased during the last three stages. DFAA was 2.73 times higher in final product than in fresh fish, which can enhance the umami taste of dry-salted fish. The level of sweet amino acids (SFAA) increased significantly in the early stage of drying (stage D). And, SFAA was 2.58 times higher in final product than in fresh fish, which can enhance the sweet taste of dry-salted fish.
Table 3. Changes in free amino acid levels during different methods of dry salted processing Decapterus maruadsi.
Tabla 3. Cambios en los niveles de aminoácidos libres durante diferentes métodos del procesamiento de Decapterus maruadsi seco salado.
3.4. Relationships between endogenous proteinase, protein degradation, and physicochemical index during dry-salted fish processing
The relationship between endogenous proteinases, protein degradation, physicochemical index, and FAAs during dry-salted fish processing was evaluated by PCA. Two principal components (principal component 1: 63.064%; principal component 2: 27.722%) were extracted, explaining 90.786% of the total variance. shows the loadings of different variables in scatter plots of principal component 1 and principal component 2. The relationship among all variables could be explained by their location in the loading plots.
Figure 1. Loading plots of lipolysis variables and lipid oxidation variables of Decapterus maruadsi during traditional dry salted processing.
Figura 1. Diagramas de carga de las variables de lipólisis y la oxidación de lípidos de Decapterus maruadsi durante el procesamiento tradicional de pescado seco salado.
B, L, H means cathepsin B, L and H activity. DPP-I, DPP-IV mean dipeptidyl peptidase I and IV activity. RAP, AAP and LAP mean arginine aminopeptidase, alanyl aminopeptidase and leucyl aminopeptidase activity. NPN means non-protein nitrogen content percentage. TN means total nitrogen content percentage. PI means Protein Degradation Index. SC means salt content percentage. MC means moisture content percentage. TFAA means the total free amino acid content. BFAA means the free amino acid content contributing to reduce the bitter taste in different processing stages; DFAA means the free amino acid content contributing to the umami taste in different processing stages; SFAA means the free amino acid content contributing to the sweet taste; PFAA means the total level of flavor-giving amino acids/the total of free amino acids in different processing stages.
B,L,H significan actividad de las catepsinas B, L y H. DPP-I, DPP-IV significan actividad de la peptidasa i y iv. RAP, AAP y LAP significan actividad de arginina aminopeptidasa, alanil aminopeptidasa y leucil aminopeptidasa. NPN significa el porcentaje del contenido de nitrógeno no proteínico. TN significa el porcentaje del contenido total de nitrógeno. PI significa el Índice de degradación proteínica. SC significa el porcentaje del contenido de sal. MC significa el porcentaje del contenido de humedad. TFAA significa el contenido total de aminoácidos libres. BFAA significa el contenido de aminoácidos libres que contribuye a reducir el sabor amargo en distintas etapas del procesamiento; DFAA significa el contenido de aminoácidos libres que contribuye al sabor umami en distintas etapas del procesamiento; SFAA significa el contenido de aminoácidos libros que contribuye al sabor dulce; PFAA = el nivel total de aminoácidos que contribuye al sabor/el total de aminoácidos libres en distintas etapas del procesamiento.
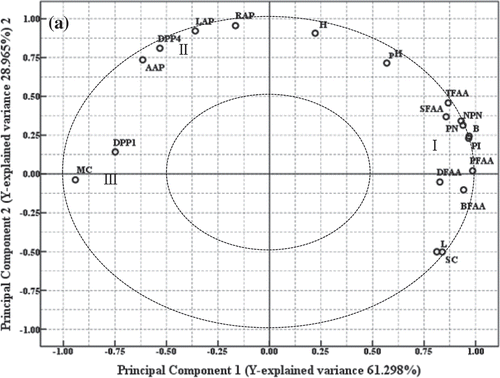
3.4.1. The relationship between endogenous proteinases and protein degradation
As shown in , cathepsin B and L, protein degradation indicators (PI, NPN), and FAAs (TFAA, BFAA, DFAA, SFAA, and PFAA) are clumped together (I). In , we observed positive loadings of NPN (0.939), PI (0.966), BFAA (0.942), PFAA (0.986), SFAA (0.857), DFAA (0.826), and TFAA (0.867) and high positive loadings of cathepsin B (0.968) and cathepsin L (0.812) in principal component 1. The dipeptidases (DPP I and IV) and aminopeptidase (AAP, RAP, LAP) formed a cluster (II) in a separate region with negative loadings (DPP I, −0.749; DPP IV, −0.532; AAP, −0.615; LAP, −0.360; RAP, −0.165). Cathepsin H is located the region between I and II. Thus, cathepsin B and L were more closely related to protein degradation and the generation of FAA than cathepsin H in dry-salted fish processing. Parreño et al. (Citation1994) also found that the contribution of cathepsin H to the proteolysis produced during ham-manufacturing processes was very low. The role of DPP I and IV and aminopeptidases AAP, RAP, and LAP on protein degradation and flavor formation in dry-salted fish was limited. Similar results were reported by Virgili, Parolari, Schivazappa, Soresi, and Borri (Citation1995) who found a significant correlation between NPN and cathepsin B during the processing of Parma ham. Garcia-Garrido et al. (Citation2000) found that the activity of cathepsins B + L was the main reason for the increased level of NPN during the processing of Spanish ham. During the processing of Jinhua ham (Zhao et al., Citation2006, Zhao, Zhou, Tian, et al., Citation2005, Zhao, Zhou, Wang, et al., Citation2005), RAP and LAP were found to have a strong correlation with the release of FAA; however the role of AAP was limited. In addition, DPP I could promote the release of polypeptides, while DPP IV played a minor role.
3.4.2. The relationship between physicochemical index and protein degradation
Salt content (SC) and cathepsins B and L were clustered in the same group (I) in . However, DPP I, DPP IV, AAP, RAP, and LAP activities did not cluster with SC. The results showed that SC had a major effect on the activity of cathepsins L and B (especially the activity of cathepsin L). Elías, Fidel, and José (Citation1991) found that a certain concentration of salt content could not inhibit the activity of cathepsins B and L by single-factor and control experiments. In the processing of Spanish ham, Garcia-Garrido et al. (Citation2000) found that NaCl affected NPN levels by affecting the activity of cathepsin. The PCA results in Section 3.4.1 showed that cathepsins B and L could promote the degradation of proteins, so it can be inferred that the salt content can promote protein degradation and flavor component formation by promoting the activity of cathepsins B and L during the processing of dry-salted fish. Moisture content (MC) and DPP1 were in the same area (III); however, MC did not cluster with other endogenous proteinases in suggesting that MC in the process of dry-salted fish was not significant to the protein degradation or the formation of PFAA and TFAA. Values for pH did not belong to any area in , so the effect of pH values on enzyme activity throughout the whole processes was limited. Thus, the influence of pH on protein degradation and the formation of PFAA and TFAA were not apparent in dry-salted fish.
4. Conclusion
XPCA indicated that cathepsins B and L had larger influences than cathepsin H on protein degradation and flavor substance formation in dry-salted fish, but the influence of DPPs and aminopeptidases on protein degradation and flavor substance formation was limited. Certain concentrations of salt could promote the degradation of proteins and the formation of flavor substances. Water content and pH had no significant effect on protein degradation or FAA formation.
Acknowledgments
This work was supported by the National Natural Science Foundation of China: [Grant Numbers 31371800, 31571869], China Agriculture Research System: [Grant Number CARS-47], Special Scientific Research Funds for Central Non-profit Institutes, South China Sea Fisheries Research Institute, and Chinese Academy of Fishery Sciences:[Grant Number 2015YD02].
Disclosure statement
No potential conflict of interest was reported by the authors.
Additional information
Funding
References
- Ahmeda, Z., Donkora, O., Street, W. A., & Vasiljevic, T. (2015). Calpains and cathepsins-induced myofibrillar changes in post-mortem fish: Impact on structural softening and release of bioactive peptides. Trends in Food Science & Technology, 45, 130–146.
- Angel, S. M., & Toldra, F. (2001). Dipeptidyl peptidase IV from porcine skeletal muscle: Purification and biochemical properties. Food Chemistry, 75, 159–168.
- Berardo, A., Devreese, B., De Maere, H., Stavropoulou, D. A., Van Royen, G., Leroy, F., & De Smet, S. (2017). Actin proteolysis during ripening of dry fermented sausages at different pH values. Food Chemistry, 221, 1322–1332.
- Bolumar, T., Bindricha, U., Toepfl, S., Toldrá, F., & Volker Heinz, V. (2014). Effect of electrohydraulic shockwave treatment on tenderness, muscle cathepsin and peptidase activities and microstructure of beef loin steaks from Holstein young bulls. Meat Science, 98, 759–765.
- Careri, M., Mangia, A., Barbieri, G., Bolzoni, L., Virgili, R., & Parolari, G. (1993). Sensory property relationships to chemical data of Italian-type dry-cured ham. Journal of Food Science, 58, 968–972.
- Chéret, R., Delbarre-Ladrat, C., Lamballerie-Anton, M. D., & Verrez Bagnis, V. (2007). Calpain and cathepsin activities in post mortem fish and meat muscles. Food Chemistry, 101, 1474–1479.
- Elías, R., Fidel, T., & José, F. (1991). Effect of dry-curing process parameters on pork muscle cathepsin B, H and L activity. Zeitschrift für Untersuchung der Nahrungs- und Genußmittel, sowie der Gebrauchsgegenstände, 93, 541–544.
- Fidel, T., & David, J. E. (1988). Examination of cathepsins B, D, H and L activities in dry-cured hams. Meat Science, 23, 1–7.
- Flores, M., Aristoy, M. C., Antequera, T., Barat, J. M., & Toldrá, F. (2009). Effect of prefreezing hams on endogenous enzyme activity during the processing of Iberian dry-cured hams. Meat Science, 82, 241–246.
- Flores, M., Aristoy, M. C., Antequera, T., Barat, J. M., & Toldrá, F. (2012). Effect of brine thawing/salting on endogenous enzyme activity and sensory quality of Iberian dry-cured ham. Food Microbiology, 29, 247–254.
- Gallego, M., Mora, L., Fraser, P. D., Aristoy, M.-C., & Toldrá, F. (2014). Degradation of LIM domain-binding protein three during processing of Spanish dry-cured ham. Food Chemistry, 149, 121–128.
- García, I., Díez, V., & Zumalacárregui, J. M. (1998). Changes in nitrogen fractions and free amino acids during ripening of spanish dried beef “cecina”. Journal of Muscle Foods, 9, 257–266.
- Garcia-Garrido, J. A., Quiles-Zafra, R., Tapiador, J., & Luque, D. C. M. (2000). Activity of cathepsin B, D, H and L in Spanish dry-cured ham of normal and defective texture. Meat Science, 56, 1–6.
- Ge, L., Xu, Y., Xia, W., Jiang, Q., & Jiang, X. (2016). Differential role of endogenous cathepsin and microorganism in texture softening of ice-stored grass carp (Ctenopharyngodon idella) fillets. Journal of the Science of Food and Agriculture, 96, 3233–3239.
- Hansen-Mller, J., & Hinrichsen, L. (1997). Evaluation of peptides generated in Italian-style dry-cured ham during processing. Journal of Agricultural and Food Chemistry, 45, 3123–3128.
- Jin, G., Zhang, J., Yu, X., Zhang, Y., Lei, Y., & Wang, J. (2010). Lipolysis and lipid oxidation in bacon during curing and drying-ripening. Food Chemistry, 123, 465–471.
- Parreño, M., Cussó, R., Gil, M., & Sárraga, C. (1994). Development of cathepsin B, L and H activities and cystatin-like activity during two different manufacturing processes for Spanish dry-cured ham. Food Chemistry, 49, 15–21.
- Rosell, C. M., & Toldrá, F. (1998). Comparison of muscle proteolytic and lipolytic enzyme levels in raw hams from Iberian and White pigs. Journal of the Science of Food and Agriculture, 76, 117–122.
- Sárraga, C., Gil, M., & García-Regueiro, J. A. (1993). Comparison of calpain and cathepsin (B, L and D) activities during dry-cured ham processing from heavy and light large white pigs. Journal of the Science of Food and Agriculture, 62, 71–75.
- Sentandreu, M. A, & Toldra, F. (2001). Dipeptidyl peptidase activities along the processing of Serrano dry-curedham. European Food Research and Technology, 213, 83–87.
- Sforza, S., Pigazzani, A., Motti, M., Porta, C., Virgili, R., Galaverna, G., … Marchelli, R. (2001). Oligopeptides and free amino acids in Parma hams of known cathepsin B activity. Food Chemistry, 75, 267–273.
- Stavropoulou, D. A., Borremans, W., De Vuyst, L., De Smet, S., & Leroy, F. (2015). Amino acid conversions by coagulase-negative staphylococci in a rich medium: Assessment of inter- and intraspecies heterogeneity. International Journal of Food Microbiology, 212, 34–40.
- Toldrá, F., Aristoy, M., Part, C., Cerveró, C., Rico, E., Motilva, M., & Flores, J. (1992). Muscle and adipose tissue aminopeptidase activities in raw and dry-cured ham. Journal of Food Science, 57, 816–818.
- Toldra, F., Aristoy, M.-C., & Flores, M. (2000). Contribution of muscle aminopeptidases to flavor development in dry-cured ham. Food Research International, 33, 181–185.
- Toldra, F., Cerverro, M. C., & Part, C. (1993). Porcine aminopeptidase activity as affected by curing agents. Journal of Food Science, 58(4). doi:10.1111/j.1365-2621.1993.tb09344.x
- Toldra, F., Flores, M., & Sanz, Y. (1997). Dry-cared ham flavour-enzymatic generation and process influence. Food Chemistry, 59, 523–530.
- Virgili, R., Parolari, G., Schivazappa, C., Soresi, B. C., & Borri, M. (1995). Sensory and texture quality of dry-cured ham as affected by endogenous cathepsin b activity and muscle composition. Journal Of Food Science, 6 (60), 1183-1186.
- Zhao, G. M., Wang, Y. L., Tian, W., Zhou, G. H., Xu, X. L., & Liu, Y. X. (2006). Changes of arginyl and leucyl aminopeptidase activities in biceps femoris along Jinhua ham processing. Meat Science, 74, 450–458.
- Zhao, G. M., Zhou, G. H., Tian, W., Xu, X. L., Wang, Y. L., & Luo, X. (2005). Changes of alanyl aminopeptidase activity and free amino acid contents in biceps femoris during processing of Jinhua ham. Meat Science, 71, 612–619.
- Zhao, G. M., Zhou, G. H., Wang, Y. L., Xu, X. L., Huan, Y. J., & Wu, J. Q. (2005). Time-related changes in cathepsin B and L activities during processing of Jinhua ham as a function of pH, salt and temperature. Meat Science, 70, 381–388.