ABSTRACT
The aim of this study was to assess the ability of the biotransformation of hesperidin from lime peel by Aspergillus saitoi in solid fermentation. The decrease of hesperidin by High-Performance Liquid Chromatography (HPLC) was monitored during 8 days of fermentation. The radical scavenging activity of DPPH· and ABTS·+ increased to 5.8 and 11 times, respectively, after 6 days of fermentation compared with unfermented lime peel. Similarly, the phenolic content of the extract of fermented lime peel was 8.66 times higher than unfermented lime peel, while flavonoids increased 5.14 times. After 6 days of fermentation, A. saitoi biotransformed hesperidin from lime peels and increased its antioxidant activity. These results provide important information concerning the potential of the solid fermentation in the citrus industry waste through the biotransformation of flavonoids, which provides an effective solution for modifying the flavonoid structure and improving their initial bioactivity.
RESUMEN
El objetivo de este estudio fue evaluar la capacidad de transformación de hesperidina a partir de cáscara de lima por Aspergillus saitoi en fermentación sólida. La disminución de hesperidina durante la fermentación se cuantificó durante 8 días utilizando Cromatografía Líquídia de Alta Presión (HPLC). La actividad de atrapamiento de los radicales DPPH• y ABTS•+ incrementó de 5.8 a 11 veces, respectivamente, después de 6 días de fermentación comparado con la cáscara de lima sin fermentar. El contenido fenólico de cáscara de lima fermentada fue 8.66 veces más alta que sin fermentar, mientras que los flavonoides aumentaron 5.14 veces. Después de 6 días de fermentación, A. saitoi biotransformó hesperidina de la cáscara de lima e incrementó su actividad antioxidante. Estos resultados proporcionan información importante concerniente al potencial de la fermentación sólida en los desechos de la industria de los cítricos a través de la biotransformación de flavonoides, los cuales dan una solución efectiva para modificar la esctructura de estos mejorando su actividad inicial.
1. Introduction
The majority of industrialization and exploitation of citrus comprises juice extraction; however, the peel, which represents 50% of the mass of the fruit, may be an important source of bioactive compounds, such as fibers and polyphenols, particularly flavonoids (Girard & Mazza, Citation1998; Liu, Pan, Li, Jie, & Zeng, Citation2017). The characteristic flavonoids in Citrus are flavanones and polymethoxylated flavones, which are relatively rare in other plants and are mostly localized in the peel (Manthey & Grohmann, Citation1996; Xi, Fang, Zhao, Jiao, & Zhou, Citation2014). Flavanones are usually glycosylated and, depending on the Citrus species, this glycosylation may be the rutinoside type, such as hesperidin in the orange (Citrus sinensis) and sweet lime (Citrus limetta), or neohesperidoside type, as in naringin in the grapefruit (Citrus paradise), or neohesperidin in the bitter orange (Citrus aurantium) (Tripoli, La Guardia, Giammanco, Di Majo, & Giammanco, Citation2007). Due to their structure, these flavonoids are highlighted due to their antioxidant activity, i.e., Free Radicals (FR) scavenging activity. FR are generated during the metabolism of the body and are associated with diseases such as cancer, hypertension, myocardial infarct, and diabetes. However, studies have shown that increasing the dietary intake of natural phenolic compounds correlates with the reduction of development of such diseases (De Sousa, Marcadenti, & Portal, Citation2017; Perez-Beltran et al., Citation2017). The antioxidant activity of flavonoids is determined by a combination of structural elements, the presence or absence of glycosidic residues (glycosides or aglycones), the glycosylation site, and the number and position of free and esterified hydroxyl. Principally, three structural groups determine the antioxidant capacity: the ortho-dihydroxy (catechol) structure in the B-ring; the 2,3-double bond, in conjugation with a 4-oxo function, and the presence of both the 3-(a)-and 5-(b)-hydroxyl groups (Benavente-García, Castillo, Marín, Ortuño, & Del Rio, Citation1997; Di Majo et al., Citation2005). Some studies show that flavonoids in aglycone form are absorbed faster and in higher amounts than the glycosylated flavonoids (Miyake, Ito, Itoigawa, & Osawa, Citation2007). In addition to low bioavailability, glycosylated flavonoids exhibit low solubility and stability in commercial preparations, which restricts their use in many applications. The biotransformation of flavonoids includes these problems by altering the structure of the flavonoid while maintaining or improving its original bioactivity when is introduced a hydroxyl group (Sun, Johnson, Wong, & Foo, Citation2017). Additional benefits include an increase in antioxidant activity or metal chelating activity (Mellou et al., Citation2005), the formation of new compounds (Dymarska et al., Citation2017) and they may possess amphiphilic properties for expanding their commercial application. Such enzymatic modifications can reduce the astringency and bitterness that are present in foods rich in polyphenols (Degenhardt, Ullrich, Hofmann, & Stark, Citation2007). Biotransformations of polyphenols have been performed with many microorganisms, including Aspergillus species. A fermented product with higher antioxidant activity was able to be obtained from Aspergillus saitoi compared with the other strains (Esaki, Onozuca, Kawakishi, & Osawa, Citation1997; Miyake et al., Citation2007). Two potent antioxidant isoflavones (8-hydroxydaidzein and 8-hydroxygenistein) were obtained from the glycosylated isoflavones (daidzein and genistein) of soybeans fermented with A. saitoi (Esaki, Kawakishi, Morimitsu, & Osawa, Citation1999a). The authors of the other studies have also obtained hydroxyflavones as follows: 8-hydroxyhesperetin, carthamidin (6-hydroxynaringenin) and isocarthamidin (8-hydroxynaringenin) from glycosylated flavones, hesperidin and naringin, extracted from citrus fruit (Miyake et al., Citation2003). In rice fermented with A. saitoi, pyranonigrin-A was found, which is not present in unfermented rice, which is responsible for the increased antioxidant activity (Miyake et al., Citation2007). Research in the biotransformation of flavonoids should be expanded by employing other microorganisms and substrates, in addition to optimizing fermentation conditions for obtain novel, high-yield compounds (Madej, Popłoński, & Huszcza, Citation2014; Ye et al., Citation2009). Therefore, the purpose of this research was to study the biotransformation of hesperidin from lime peel (Citrus limetta Risso) by means of solid fermentation using Aspergillus saitoi, thereby potentiating its antioxidant activity. The product from fermented lime peel could be utilized for the development of high-value bioactive compounds for pharmaceutical and food applications.
2. Materials and methods
2.1. Lime peel flour
The fresh fruits of the sweet lime were harvested in Atotonilco El Alto, Jalisco, Mexico (20° 32′ N, 102° 30′ W, 1761 m of altitude) from November 2007 to February 2008. To remove the peel, lime juice was obtained with an extractor (Bepex, Minneapolis, MN, USA) and subsequently the pulp was removed. The peel was dried utilizing a tunnel dryer (Forced Convection Oven; Despatch Industries, Minneapolis, MN, USA) at a temperature of 40°C. Once dehydrated, this peel was milled using a hammer mill (Pulvex 200, Pulvex, Mexico City, Mexico) and sieved (Ro-Tap Sieve Shaker RX-29; WS Tyler, OJ, USA) to separate particle size, using 20 and 50 meshes, with a particle size between 300 and 850 μm, respectively (Rodríguez-Rivera, Lugo-Cervantes, Winterhalter, & Jerz, Citation2014).
2.2. Starter microorganism
Aspergillus saitoi 22299 was obtained from JCM (Japan Collection of Microorganisms), Riken Bioresource. The fungus was cultured for 7 days on Potato Dextrose Agar (PDA) at 30°C, and then the spores were collected by washing the agar surface with sterile distilled water containing 0.1% Tween 80, and this was adjusted to a concentration of 2 × 108 spores/mL with a Neubauer chamber or hematocytometer, which served as inoculum.
2.3. Fermentation of lime peel with Aspergillus saitoi
For the fermentation of lime peel, the flour of mesh 20 and 50 (1:1) served as solid support. The flour was exposed during 15 min to UltraViolet (UV) light for sterilization; the antioxidant capacity was not affected by this treatment (data not shown). The fermentation condition was selected according to Miyake et al. (Citation2007), and it was used the best condition carried out for this author. Ten grams of sterile flour were placed in Petri dishes, which were impregnated with 25 mL of phosphate buffer (pH 5.0) inoculated with 1 mL of 2 × 108 spores/ml of A. saitoi. The Petri dishes were incubated at 30°C for 7 days in the dark with an initial humidity of 70% and a proportion of N:C of 1:6 obtained from peel composition of Citrus limetta. Samples were taken every 24 h according to Miyake, Minato, Fukumoto, Shimomura, and Osawa (Citation2004) with some modifications: 10 g of fermented lime peel was macerated in 50 mL of methanol at 37°C for 2 days and was centrifuged at 1,500 × g for 30 min at 4°C. Subsequently, the supernatant was recovered and concentrated in a rotary evaporator (RV 10 digital IKA, Staufen, Germany) at 40°C. The concentrate was resuspended in 10 mL of 80% ethanol and then centrifuged at 6,900 × g for 20 min at 4°C. The supernatant was concentrated in a rotary evaporator and the concentrated sample was finally lyophilized, in order to obtain extract yield per g of dry sample. The work sample was prepared at 0.002 g/mL and then, total phenols and flavonoids and the amounts of hesperidin and antioxidant activity were measured.
2.4. Determination of antioxidant activity
2.4.1. Measurements of total phenolic and flavonoid contents
The Folin-Ciocalteu assay (Mullen, Marck, & Crozier, Citation2007; Prior, Wu, & Schaich, Citation2005) was used for the determination of the total phenolic. An aliquot (50-μL) of the sample was taken and added to 3 mL distilled water, 250 μl of Folin (Biochemika, Fluka, D9132), 750 μL of 20% Na2CO3, and 950 μL of distilled water. This was left to stand for 30 min and then absorbance was determined at 765 nm using a spectrophotometer (SINGLE 2800 UV/VIS Spectrophotometer; Dayton, NJ, USA). A calibration curve of Gallic Acid (Sigma, G7384, 97.5%) was performed and the results were expressed as milligrams of Gallic Acid Equivalents per g of dry sample (mg GAE/g ds).
Flavonoid quantification was performed by the method as described by Maksimovic, Malencic, and Covacevic (Citation2005), with some modifications. Aliquots of 1-mL of samples were taken, which were added to 4 mL of deionized water, 30 μL of 5% NaNO2 (sodium nitrite), and 30 μL of 10% of AlCl3 (aluminum trichloride). This was left to stand for 1 min; then, 2 mL of 1 M NaOH (sodium hydroxide) and 2.4 mL of deionized water were added. This was vortexed and absorbance was measured at 405 nm. A calibration curve of rutin was performed. Total flavonoid content was expressed as mg Rutin equivalents per g of dry sample (mg RE/g ds). All tests were conducted in triplicate.
2.4.2. The ABTS (2,2′-azino-bis(3-ethylbenzothiazoline-6-sulfonic acid) diammonium salt) assay
For the ABTS assay, the cation radical solution was prepared by mixing a solution of 7 mM ABTS with 2.4 mM potassium persulfate (Sigma-Aldrich, A1888, 98%). The reaction mixture was allowed to stand for 12–16 h at room temperature. This was then diluted in ethanol (1:30), verifying that the dilution had an absorbance (734 nm) of 0.7 ± 0.01, as measured by a spectrophotometer microplate reader (Multiskan GO Microplate Spectrophotometer; Thermo Scientific, Waltham, MA, USA). In a 96-well microplate was placed 20 μL of sample, to which was added 270 μl of the ABTS•+ solution; after 30 min, absorbance was measured at 734 nm. A calibration curve of Trolox (Sigma-Aldrich, 238813, 97%) as standard was used and the results were expressed as μM Trolox Equivalents per g of dry sample (μM TE/g ds). Values were expressed as the mean of three replicates (Falk et al., Citation2006).
2.4.3. The DPPH (2,2-diphenyl-1-picrylhydrazyl) assay
For the DPPH method, a solution of 150 mM DPPH· (Sigma-Aldrich, D9132) in methanol was prepared. In a 96-well microplate, were placed 20 μL of sample, to which 200 μL of the cationic-radical solution was added. This was left to stand for 30 min and absorbance was measured at 515 nm. The results were reported in μM TE/g ds. (Fukumoto & Mazza, Citation2000).
2.5. Identification of hesperidin
Analyses were conducted by High-Performance Liquid Chromatography (with the HPLC Varian ProStar 230 UV-Vis detector). The diode array detector at 285 nm was used; a Zorbax SB-C18 (250 mm × 4.6 mm × 5 μm) column, and the mobile phase consisted of a solution of A = 0.1% formic acid and B = methanol (A = 40% and B = 60%) at a temperature of 50°C; flow was 1.0 mL/min and the injection volume was 20 μL. Identification of hesperidin was estimated on the basis of the retention time of the standard reagent. For the quantification, the commercial standard hesperidin (Analytical Fluka, 50162, 99%) was employed and a calibration curve was performed.
2.6. Statistical analysis
Multifactorial analysis was performed with three replicates and an Analysis of Variance (ANOVA) and LSD test were applied to observe significant differences during fermentation days using the Statgraphics Centurion XV ver. 2.15.06 statistical software. Differences were considered significant when p < 0.05.
3. Results and discussion
The content of phenols and flavonoids was measured during fermentation, as well as the behavior of antioxidant activity and the amount of hesperidin. A significant increase of these parameters on day 6 of fermentation was observed in the spore-formation step. The TPC (Total Phenolic Contents) of fermented lime peel was 8.66 times greater than that of unfermented peel, while the TFC (Total Flavonoids Contents) increased 5.14 times (). Similarly, antioxidant activity measured by DPPH• increased 5.8 times, while the ABTS•+ method was up to 11 times higher than that of the unfermented peel (). One possible reason for these increases is that during the solid-state fermentation process, the biotransformation transformed the phenolics presented in substrate into aglycone phenolic molecules with higher antioxidant capacity. Then, the aglycone form of hesperidin has more important effects of bioactivity than glycoside. However, this affirmation cannot be validated, because in all microbial fermentation, the compounds present in the medium are transformed into different chemical structures that participate in the metabolism of the microorganism (Madeira, Nakajima, Macedo, & Macedo, Citation2014). Consequently, is possible that after day 6 the biotransformed structures produced lost or diminished its antioxidant capacity and therefore the affinity with the components of the techniques used decreased.
Figure 1. Total phenolic and flavonoid contents during fermentation kinetics of lime peel by Aspergillus saitoi. GAE, Gallic Acid Equivalents; TPC, Total Phenolic Contents; TFC, Total Flavonoids Content; FL, Fermented Lime; UFL, Unfermented Lime.
Figura 1. Contenido total de fenoles y flavonoids durante la cinética de fermentación de la cáscara de lima por Aspergillus saitoi. GAE, Equivalentes de Ácido Gálico; TPC, Contenido de Fenoles Totales; FL, Lima Fermentada; UFL, Lima sin fermentar.
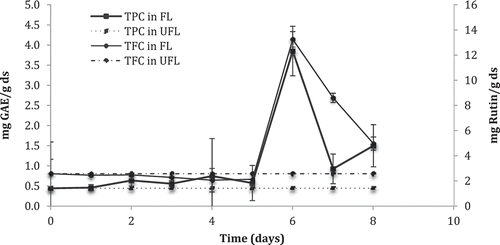
Figure 2. The antioxidant activity of lime peel during fermentation kinetics by Aspergillus saitoi using the DPPH• and ABTS•+ assays. TE, Trolox Equivalents; FL, Fermented Lime; UFL, Unfermented Lime.
Figura 2. Actividad antioxidante de la cáscara de lima durante la cinética de fermentación por Aspergillus saitoi usando los ensayos de DPPH• y ABTS•+. TE, Equivalentes Trolox; FL, Lima Fermentada; UFL, Lima sin fermentar.
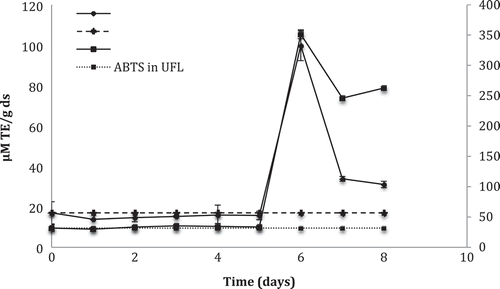
The effect of A. saitoi on the DPPH• radical scavenging activity of lemon peel (Miyake et al., Citation2004), Dangyuja extract (Citrus grandis Osbeck) (Kim, Shin, & Jang, Citation2009), and hesperidin (Osawa, Minato, & Miyake, Citation2009) were previously investigated; the authors of these studies obtained up to 3, 1.33, and 5.3 times higher antioxidant activity, respectively. According to the above, the improvement of antioxidant activity of fermented hesperidin is higher than fermented extracts; thus, in this study, it was reported that increase in fermented lime peel is similar to that of the fermented pure compound.
In the present study, the spores appeared on day 2 of fermentation, which favors the increase in antioxidant activity because, in the spore-formation step, the vegetative mycelia of A. saitoi performed microbial biotransformation while forming spores, ascribing to this a hydroxylation reaction in which a hydroxyl group is added to the 8th position of hesperidin (Osawa et al., Citation2009). Miyake et al. (Citation2003) measured the antioxidant activity in two cultures with naringin and hesperidin, respectively, during the fermentation by A. saitoi. The antioxidant activity of hesperidin increased significantly, from 40 to 71%, after 20 days of fermentation, whereas the antioxidant activity of naringin increased from 30 to 53% after 6 days of fermentation after of sporulation; therefore, the spore-formation step comprises an important factor that determines the biotransformation time. The relationship between increase in antioxidant activity and formation of A. saitoi spores has also been reported in the fermentation of soy and rice (Esaki et al., Citation1999a; Miyake et al., Citation2007), in the fermentation of lemon peel (Miyake et al., Citation2004), and in liquid medium with hesperidin (Miyake et al., Citation2003; Osawa et al., Citation2009).
In hesperidin content during fermentation is illustrated, during which there was observed an increase in hesperidin content during 1 and 2 days of fermentation. This is due to the release of the phenolic compounds contained in the matrix carbohydrates of the cell wall in which they are embedded, by means of the hydrolytic action of the fungus. Subsequently, the hesperidin content decreased during fermentation, probably because it is being transformed into its aglycone or its hydroxyflavanone. Esaki, Watanabe, Onozuka, Kawakishi, and Osawa (Citation1999b) proposed a mechanism for the formation of ο-dihydroxyisoflavones, it was proved that A. saitoi have the capability for hydrolysis to the aglycone from a flavonoid glycoside and for hydroxylation to ο-dihydroxyflavonoid. The hydroxylase activity increased with the progress of sporulation. It is possible that other phenolic compounds of lower molecular weight are produced by this biotransformation as suggested by Madeira et al. (Citation2014). On days 6 and 7, the amount of hesperidin remains constant until about a 70% decrease on day 8 of fermentation with respect to its highest point (day 2), what leads one to think that the high amount of this glycosylated flavanone was transformed into the aglycone form. Miyake et al. (Citation2004) measured the amount of hesperidin before and after lemon peel fermentation, which decreased to 80% after 7 days of fermentation. The authors identified the 8-hydroxyhesperetin produced in fermented lemon peel and some aglycones, which were not present prior to fermentation, such as eriodictyol, hesperetin, and naringenin. Also, in other studies, catechin and quercetin have been found after fermentation of litchi fruit pericarp by Aspergillus awamori (Lin et al., Citation2012), and carthamidin and isocarthamidin have been found after of oxidation of naringenin by Rhodotorula marina (Madej et al., Citation2014).
Figure 3. Hesperidin content during fermentation kinetics of lime peel by Aspergillus saitoi measured by High-Performance Liquid Chromatography (HPLC) with UltraViolet (UV) detector at 285 nm.
Figura 3. Contenido de hesperidina durante la cinética de fermentación de la cáscara de lima medida por Cromatografía de Alta Presión (HPLC) con detector Ultravioleta (UV) a 285 nm.
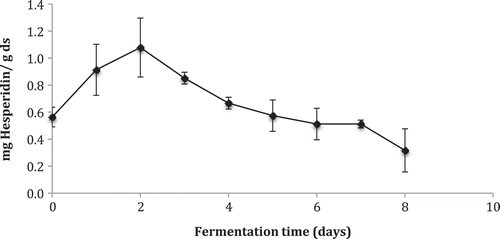
Hesperidin was identified from the retention time of standard for High-Performance Liquid Chromatography (HPLC) analysis (). In the present study, HPLC analysis demonstrated, three peaks in the unfermented lime-peel extract, among which the third was identified as hesperidin. An unidentified compound (Peak 1) did not separate but, after fermentation, Peak 1 was the best defined and slightly increased its concentration, unlike the majority compound (Peak 2) which might have been degraded and converted into other metabolic products. Previous studies show that flavanone glycosides, hesperidin, and eriocitrin (eriodictyol-7-O-rutinoside) exist abundantly in the peel and juice from lemons and limes (Barreca, Bellocco, Caristi, Leuzzi, & Gattuso, Citation2011; Hajimahmoodi et al., Citation2014; Peterson et al., Citation2006; Rodríguez-Rivera et al., Citation2014), in which one is able to observe that the standard of eriocitrin appears before hesperidin; thus, it is probable that Peak 2, detected in this study, could be eriocitrin.
Figure 4. High-Performance Liquid Chromatography (HPLC) chromatograms of unfermented (A) and fermented (B) lime peel by Aspergillus saitoi using UltaViolet (UV) detector at 285 nm. Peak 3 was labeled as ‘hesperidin’, and the remainder, as ‘unidentified’. At the top right of figure is shown an expansion from R = 3 min to R = 14 min.
Figura 4. Cromatogramas del Cromatógrafo de Alta Presión (HPLC) de cáscara de lima sin fermentar (A) y fermentada (B) por Aspergillus saitoi usando detector Ultravioleta (UV) a 285 nm. El pico 3 fue identificado como ‘hesperidina’, y el resto, como ‘no identificado’. En la parte superior derecha de la fiigura se muestra una expansión desde R = 3 min a R = 14 min.
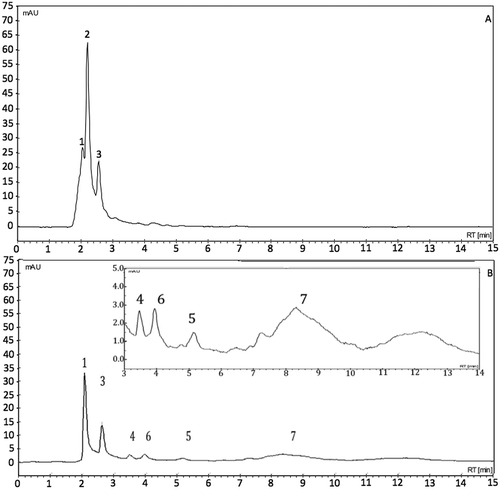
The chromatogram of fermented lime-peel extract exhibits a new, unidentified compound (Peak 7) at 8.4 minutes, which appears on day 6 of fermentation, which may account for the increased in antioxidant activity of fermented lime peel by A. saitoi. Miyake et al. (Citation2003) found a new flavonoid compound (8-hydroxyhesperetin) that was not present prior to fermentation and that is presumed to have a relationship with the increase of antioxidant properties. In this case, it is possible, that this peak could be a hydroxylated flavonoid, but more chemical studies will be carrying out. Other studies of biotransformation, with different microorganisms, have been performed for the utilization of agro-industrial wastes such as cocoa pod, palm cake, cassava peel (Lateef et al., Citation2008), and okara (Zhu, Fan, Cheng, & Li, Citation2008). Similarly, enzymatic biotransformation has been employed in naringin (Hui et al., Citation2009), hesperidin (Ferreira, Macedo, Ribeiro, & Macedo, Citation2013), and in extracts of green tea (Camellia sinensis) and yerba mate (Ilex paraguariensis) (Macedo, Battestin, Ribeiro, & Macedo, Citation2011) for the improvement of antioxidant properties. However, these studies are focused principally in the utilization of agro-industrial wastes to obtain products with other properties as digestibility, elimination of some toxic compounds, etc., while this work was focused in the biotransformation of flavanone to hidroxyflavanone for future use in food or health areas.
4. Conclusions
After 6 days of fermentation, A. saitoi biotransformed the hesperidin present in the lime peel and increased its antioxidant activity from 5.8 to 11 times higher compared with unfermented lime peel. The high antioxidant activity of the fermented lime peel may be related with the biotransformation of hesperidin to its hydroxyflavanone and aglycone considering that some other phenolic compounds of lower molecular weight are produced. Then, this process provides an effective solution to modify the structure of flavonoids and to improve their initial bioactivity for pharmaceutical and food applications.
Acknowledgment
The research for this paper was financially supported by Consejo Nacional de Ciencia y Tecnología (CONACyT) grant no. 268829.
Disclosure statement
No potential conflict of interest was reported by the authors.
Additional information
Funding
References
- Barreca, D., Bellocco, E., Caristi, C., Leuzzi, U., & Gattuso, G. (2011). Flavonoid profile and radical-scavenging activity of Mediterranean sweet lemon (Citrus limetta Risso) juice. Food Chemistry, 129, 417–422.
- Benavente-García, O., Castillo, J., Marín, F. R., Ortuño, A., & Del Rio, J. A. (1997). Uses and properties of Citrus flavonoids. Journal of Agriculture and Food Chemistry, 45, 4505–4515.
- de Sousa, P. A. L., Marcadenti, A., & Portal, V. L. (2017). Effects of olive oil phenolic compounds on inflammation in the prevention and treatment of coronary artery disease. Nutrients, 9(10), 1087.
- Degenhardt, A., Ullrich, F., Hofmann, T., & Stark, T. (2007). Flavonoid sugar addition products, method for manufacture and use thereof. U.S. Patent No. 20070269570. Munich: Kraft Foods R&D Inc.
- Di Majo, D., Giammanco, M., La Guardia, M., Tripoli, E., Giammanco, S., & Finnoti, E. (2005). Flavanones in Citrus fruit — Structure-antioxidant activity relationships. Food Research International, 36, 1161–1166.
- Dymarska, M., Grzeszczuk, J., Urbaniak, M., Janeczko, T., Plaskowska, E., Stepien, L., & Kosstrzewa-Suslow, E. (2017). Glycosylation of 6-methylflavone by the strain Isaria fumosorosea KCH J2. Plos One, 1–14. doi: 10.1371/journal.pone.0184885. Retrieved from http://journals.plos.org/plosone/article/file?id=10.1371/journal.pone.0184885&type=printable
- Esaki, H., Kawakishi, S., Morimitsu, Y., & Osawa, T. (1999a). New potent antioxidative o-dihydroxyisoflavones in fermented Japanese soybean products. Bioscience, Biotechnology, and Biochemistry, 63(9), 1637–1639.
- Esaki, H., Onozuca, H., Kawakishi, S., & Osawa, T. (1997). Antioxidative activity and isolation from soybeans fermented with Aspergillus spp. Journal of Agricultural and Food Chemistry, 45, 2020–2024.
- Esaki, H., Watanabe, R., Onozuka, H., Kawakishi, S., & Osawa, T. (1999b). Formation mechanism for potent antioxidative o-dihydroxyisoflavones in soybean fermented with. Aspergillus saitoi. Bioscience, Biotechnology, and Biochemistry, 63(5), 851–858.
- Falk, D. J., De Ruisseau, K. C., Van Gammeren, D. L., Deering, M. A., Kavazis, A. N., & Powers, S. K. (2006). Mechanical ventilation promotes redox status alterations in the diaphragm. Journal of Applied Physiology, 101(4), 1017–1024.
- Ferreira, L. R., Macedo, J. A., Ribeiro, M. L., & Macedo, G. A. (2013). Improving the chemopreventive potential of orange juice by enzymatic biotransformation. Food Research International, 51, 526–535.
- Fukumoto, L. R., & Mazza, G. (2000). Assessing antioxidant and prooxidant activities of phenolic compounds. Journal of Agricultural and Food Chemistry, 48(8), 3597–3604.
- Girard, B., & Mazza, G. (1998). Functional grape and citrus products. In J. Shi, G. Mazza & M. L. Maguer (Eds.), Functional foods, biochemical and processing (pp. 155–178). PA, USA: Technomic Publishing Company.
- Hajimahmoodi, M., Moghaddam, G., Mousavi, S. M., Sadeghi, N., Oveisi, M. R., & Jannat, B. (2014). Total antioxidant activity, and hesperidin, diosmin, eriocitrin and quercetin contents of various lemon juices. Tropical Journal of Pharmaceutical Research, 13(6), 951–956.
- Hui, Y., Haidong, X., Cigang, Y., Yijun, D., Guiyou, L., Wenping, X., & Sheng, Y. (2009). Hydroxylation of naringin by Trichoderma harzianum to dramatically improve its antioxidative activity. Enzyme and Microbial Technology, 45, 282–287.
- Kim, G. N., Shin, J. G., & Jang, H. D. (2009). Antioxidant and antidiabetic activity of Dangyuja (Citrus grandis Osbeck) extract treated with Aspergillus saitoi. Food Chemistry, 117, 35–41.
- Lateef, A., Oloke, J. K., Gueguim, E. B., Oyeniyi, S. O., Onifade, O. R., Oyeleye, A. O., … Oyelami, A. O. (2008). Improving the quality of agro-wastes by solid-state fermentation: Enhanced antioxidant activities and nutritional qualities. World Journal of Microbiology and Biotechnology, 24, 2369–2374.
- Lin, S., Yang, B., Chen, F., Jiang, G., Li, Q., Duan, X., & Jiang, Y. (2012). Enhanced DPPH radical scavenging activity and DNA protection effect of litchi pericarp extract by Aspergillus awamori bioconversion. Chemistry Central Journal, 6, 108.
- Liu, Z., Pan, Y., Li, X., Jie, X., & Zeng, M. (2017). Chemical composition, antimicrobial and anti-quorum sensing activities of pummelo peel flavonoid extract. Industrial Crops and Products, 109, 862–868.
- Macedo, J. A., Battestin, V., Ribeiro, M. L., & Macedo, G. A. (2011). Increasing the antioxidant power of tea extracts by biotransformation of polyphenols. Food Chemistry, 126(2), 491–497.
- Madeira, J. V., Jr., Nakajima, V. M., Macedo, J. A., & Macedo, G. A. (2014). Rich bioactive phenolic extract production bymicrobial biotransformation of Brazilian Citrusresidues. Chemical Engineering Research and Design, 92, 1802–1810.
- Madej, A., Popłoński, J., & Huszcza, E. (2014). Improved oxidation of naringenin to carthamidin and isocarthamidin by Rhodotorula marina. Applied Biochemistry and Biotechnology, 173, 67–73.
- Maksimovic, Z., Malencic, D., & Covacevic, N. (2005). Polyphenol contents and antioxidant activity of Maydis stigma extracts. Bioresource Technology, 96, 873–877.
- Manthey, J. A., & Grohmann, K. (1996). Concentrations of hesperidin and other orange peel flavonoids in Citrus processing byproducts. Journal of Agricultural and Food Chemistry, 44, 811–814.
- Mellou, F., Lazari, D., Skaltsa, H., Tselepis, A., Kolisis, F., & Stamatis, H. (2005). Biocatalytic preparation of acylated derivatives of flavonoid glycosides enhances their antioxidant and antimicrobial activity. Journal of Biotechnology, 116, 295–304.
- Miyake, Y., Ito, C., Itoigawa, M., & Osawa, T. (2007). Isolation of the antioxidant pyranonigrin-A from rice mold starters used in the manufacturing process of fermented foods. Bioscience, Biotechnology, and Biochemistry, 71(10), 2515–2521.
- Miyake, Y., Minato, K., Fukumoto, S., Shimomura, Y., & Osawa, T. (2004). Radical-scavenging activity in vitro of lemon peel fermented with Aspergillus saitoi and its suppressive effect against exercise-induced oxidative damage in rat liver. Food Science Technology Research, 10(1), 70–74.
- Miyake, Y., Minato, K., Fukumoto, S., Yamamoto, K., Oya-Ito, T., Kawakishi, S., & Osawa, T. (2003). New potent antioxidative hydroxyflavanones produced with Aspergillus saitoi from flavanone glycoside in citrus fruit. Bioscience, Biotechnology, and Biochemistry, 67(7), 1443–1450.
- Mullen, W., Marck, S. C., & Crozier, A. (2007). Evaluation of phenolic compounds in commercial fruit juices and fruit drinks. Journal of Agricultural and Food Chemistry, 55(8), 3148–3157.
- Osawa, T., Minato, K., & Miyake, Y. (2009). Flavonoid compound and process for producing the same. US 7 582 675 B2. Japan: Pokka Corporation, National University Corp. Nagoya University.
- Perez-Beltran, Y. E., Becerra-Verdin, E. M., Sayago-Ayerdi, S. G., Rocha-Guzman, N. E., Garcia-Lopez, E. G., Castaneda-Martinez, A., & Montalvo-Gonzalez, E. (2017). Nutritional characteristics and bioactive compound content of guava purees and their effect on biochemical markers of hyperglycemic and hypercholesterolemic rats. Journal of Functional Foods, 35, 447–457.
- Peterson, J. J., Beecher, G. R., Bhagwat, S. A., Dwyer, J. T., Gebhardt, S. E., Haytowitz, D. B., & Holdenc, J. M. (2006). Flavanones in grapefruit, lemons, and limes: A compilation and review of the data from the analytical literature. Journal of Food Composition and Analysis, 19, S74–S80.
- Prior, R. L., Wu, X., & Schaich, K. (2005). Standardized methods for the determination of antioxidant capacity and phenolics in foods and dietary supplements. Journal of Agricultural and Food Chemistry, 53, 4290–4302.
- Rodríguez-Rivera, M. P., Lugo-Cervantes, E., Winterhalter, P., & Jerz, G. (2014). Metabolite profiling of polyphenols in peels of Citrus limetta Risso by combination of preparative high-speed countercurrent chromatography and LC-ESIMS/MS. Food Chemistry, 158, 139–152.
- Sun, C. Q., Johnson, K. D., Wong, H., & Foo, L. Y. (2017). Biotransformation of flavonoid conjugates with fatty acids and evaluations of their functionalities. Frontiers in Pharmacology, 8, 759.
- Tripoli, E., La Guardia, M., Giammanco, S., Di Majo, D., & Giammanco, M. (2007). Citrus flavonoids: Molecular structure, biological activity and nutritional properties: A review. Food Chemistry, 104, 466–479.
- Xi, W., Fang, B., Zhao, Q., Jiao, B., & Zhou, Z. (2014). Flavonoid composition and antioxidant activities of Chinese local pummelo (Citrus grandis Osbeck.) varieties. Food Chemistry, 161, 230–238.
- Ye, H., Xu, H., Yu, C., Dai, Y., Liu, G., Xu, W., & Yuan, S. (2009). Hydroxylation of naringin by Trichoderma harzianum to dramatically improve its antioxidative activity. Enzyme and Microbial Technology, 45, 282–287.
- Zhu, Y. P., Fan, J. F., Cheng, Y. Q., & Li, L. T. (2008). Improvement of the antioxidant activity of Chinese traditional fermented okara (Meitauza) using Bacillus subtilis B2. Food Control, 19, 654–661.