ABSTRACT
In this work, the olive β-glucosidase (β-glu) enzyme was immobilized onto superparamagnetic nanoparticles (SPMNs). Moreover, immobilized enzyme was also used for the debittering process for natural edible olives from Edremit, Turkey. Owing to SPMNs, the system can be easily removed by a simple magnet and reused many times for debittering process. The free olive β-glucosidase (E), β-glucosidase bound SPMNs (IE), free commercial β-glucosidase (CE), and commercial β-glucosidase bound SPMNs (ICE) were comparatively studied for oleuropein removal. In 6 h, the treatment of E, IE, and ICE hydrolyzed the 15.8%, 56.4%, and 80.0% of the oleuropein for 1000 g of olives, respectively, and further treatment showed that the IE and ICE reached the 100% after 22 h treatment. The results revealed that the IE and ICE with biocompatible properties of SPMNs have the economical, fast, and reusable properties for the industrial debittering process in the table olive production.
RESUMEN
En este estudio se inmovilizó la enzima de aceituna β-glucosidasa (β-glu) en nanopartículas superparamagnéticas (SPMN); la enzima inmovilizada se utilizó en el método de desamargado de aceitunas naturales comestibles procedentes de Edremit, Turquía. Debido a las SPMN, el sistema puede ser fácilmente removido mediante un simple imán, siendo posible utilizarlo nuevamente muchas veces en el método de desamargado. Para comparar sus propiedades de eliminación de oleuropeína, se estudiaron la β-glucosidasa libre de aceituna (E), la β-glucosidasa adherida a SPMNs (IE), la β-glucosidasa libre comercial (CE) y la β-glucosidasa comercial adherida a SPMN (ICE). Después de 6 horas, los tratamientos utilizando E, IE e ICE hidrolizaron 15,8%, 56,4% y 80,0% de la oleuropeína de 1 000 g de aceitunas, respectivamente; tratamientos posteriores con IE e ICE alcanzaron 100% después de 22 horas. Estos resultados comprueban que las IE e ICE con propiedades biocompatibles de SPMN reúnen propiedades económicas y de rapidez que, aunadas a su cualidad de reusables, hace favorable su uso en el método de desamargado industrial aplicado a la producción de aceitunas de mesa.
1. Introduction
In Turkey, like other Mediterranean countries, table olives are major for economic and nutritional value (Altınyay & Altun, Citation2006; Guinda, Citation2006). The Mediterranean diet, which includes olives as a part of diet, is associated with the reduction of certain chronic diseases including cardiovascular disease and cancer (Alu’datt et al., Citation2010; Charoenprasert & Mitchell, Citation2012; Jemai, Bouaziz, Fki, El Feki, & Sayadi, Citation2008).
Olive fruit has a bitter taste arising from oleuropein, which is a phenolic glucoside. After the debittering process, olives can be consumed (Ganguli, Van Putte, & Turksma, Citation1999; Guiso & Marra, Citation2005; Kubo, Lunde, & Kubo, Citation1995; Malik & Bradford, Citation2006; Soler-Rivas, Espin, & Wichers, Citation2000). This step comprising the hydrolysis of oleuropein by chemical or biological techniques varies from region to region and also depends on variety (Bianchi, Citation2003; Brenes, Garcia, Garcia, & Garrido, Citation2001; Ciafardini, Zullo, & Cioccia, Citation2005; Esti, Cinquante, & La Notte, Citation1998; Gikas, Papadopoulos, & Tsarbopoulos, Citation2006; Guiso & Marra, Citation2005; Lavermicocca et al., Citation2002; Marsilio, Lanza, & Pozzi, Citation1996; Paiva-Martins & Gordon, Citation2001; Romani, Mulinacci, Pinelli, Vincieri, & Cimato, Citation1999; Romero, Brenes, Garcia, Garcia, & Garrido, Citation2004a; Romero et al., Citation2004b; Romero, Garcia, Brenes, Garcia, & Garrido, Citation2002; Webb et al., Citation2001). The most common method for processing green olives is “Spanish style” (Brenes Balbuena, Garcia Garcia, & Garrido Fernandez, Citation1992; Medina, Garcı´A, Romero, De Castro, & Brenes, Citation2009). NaOH solution can be used for debittering in this method. After chemical hydrolysis of oleuropein, one to three times washing is required to eliminate the alkaline residue. Sometimes, washing water with organic or inorganic acids can be used for neutralizing the alkaline residue in this step. This process leads to damage to the pectin in the fruit tissue, therefore softening could occur. In addition, water soluble compounds that have nutritional value such as phenolics, vitamins, minerals, and pigments could be lost during this process.
There is no need to use NaOH or any chemicals for the natural process of table olive production. However, this method requires a long processing time for debittering, aroma, and taste balancing in table olives. Black or green olives can be handled by natural methods. Olives are debittered by brine or water replacement and fermented with environmental microflora. If it is necessary, lactic or citric acid could be added to the brine. As a reflection of the differences in processing methods, some sensorial differences could occur (Savas & Uylaser, Citation2013).
In the enzymatic hydrolysis, the glycosidic linkage of oleuropein is hydrolyzed by β-glucosidase activity, and then glucose and oleuropein aglycone are formed. As a source of the enzyme, some researchers studied the oleuropein hydrolysis by microbial recombinant β-glucosidases (Brenes, Rejano, Garcia, Sanchez, & Hand Garrido, Citation1995; Briante, Patumi, Febbraio, & Nucci, Citation2004). Enzymatic hydrolysis of oleuropein, by preventing the loss of fermentable material during the washing step, constitutes a significant impact on the development of initial lactic acid bacteria in fermentation. By using β-glucosidases to hydrolyse oleuropein, high sensorial-quality end-products can be obtained. For all that, this procedure eliminates the waste water problem and avoids removing phenolic antioxidants from the olive that could occur in other techniques (Restuccia et al., Citation2011). However, the major drawback of the method is that the enzyme is not reusable and it increases the application cost. Immobilization of the enzyme is a technique that is often used in chemical and enzyme engineering to stabilize enzymatic activity (Alahakoon, Koh, Chong, & Lim, Citation2012; Liburdi, Straniero, Benucci, Vittoria Garzillo, & Esti, Citation2012; Onem, Cicek, & Nadaroglu, Citation2016; Yu et al., Citation2012). Binding via carbodiimide activation is a more recently used method owing to its simplicity and high efficiency (Chen & Liao, Citation2002).
Superparamagnetic nanoparticles (SPMNPs), which have a lot of properties for biotechnology, have been utilized to immobilize enzymes (Kockar et al., Citation2010; Zhu et al., Citation2014). These SPMNPs can easily control the reaction and could be separated from the reaction medium by using an external magnetic field (Kumar, Jana, Dhamija, Singla, & Maiti, Citation2013; Yu et al., Citation2012).
In this study, a novel method based on the β-glucosidase bound to the SPMNs for the debittering process in the table olive production is proposed, with economical, fast, and reusable properties. Thus, it is the first time that the β-glucosidase enzyme was immobilized onto SPMNs via Carbodiimide Activation and used for debittering of Turkish variety “Edremit” green olives. The free olive β-glucosidase (E), (IE), free commercial β-glucosidase (CE) and ICE were comparatively studied to monitor oleuropein hydrolysis during the debittering process. Other traditional methods, water treatment (W), scratching method (S), and alkaline treatment (NaOH) (L), were also included as comparative methods.
2. Material and methods
2.1. Chemicals
The commercial β-glucosidase enzyme preparation (Rapidase® 2000) was purchased from DSM Product, France. Reagents for enzymatic activity, extraction, immobilization, debittering reagents, and oleuropein standard were supplied by Sigma-Aldrich.
2.2. Superparamagnetic iron oxide nanoparticles (SPMNS)
The SPMNs have been obtained by co-precipitation in air medium at room temperature (Karaagac, Kockar, Beyaz & Tanrisever, Citation2010). The sizes of SPMNPs are around ~9 nm as obtained from images of Transmission Electron Microscope. Further information for the synthesis and characterizations of the iron oxide nanoparticles can be obtained in the literature, which is out of scope of this investigation (Karaagac & Kockar, Citation2012; Ozel & Kockar, Citation2015; Ozel, Kockar, & Karaagac, Citation2015).
Iron (II) chloride tetrahydrate, iron (III) chloride hexahydrate, and ammonium hydroxide (25% ammonium in water) were used for the synthesis. 28.4 g ferric and 13.9 g ferrous salts were dissolved in 50 mL of deionized water in total, and base solution was added to this solution under mechanical stirring. The reaction was performed for 20 min at room temperature in the air medium. After the reaction, the precipitate was washed with deionized water and dried in an oven at 65°C for overnight. The magnetic properties of SPMNs, IC, and CIE are measured by vibrating sample magnetometer (VSM, ADE EV9 Model) in field range ±20 kOe (1 Oe intervals) at room temperature, and the results revealed that the obtained particles are superparamagnetic.
2.3. Plant material
Olive fruits (Olea europaea L.) of the “Edremit” cultivar were used in this study. Edremit variety is also known as Ayvalık variety. Fruits in green maturity harvested in 2014 were obtained from Akhisar Region in Turkey. After selecting and sorting, olives were graded as 231–260 pieces per kg and they are placed in glass vessels of 1 L: 65% (v/v) of fruits and 35% (v/v) of tap water for debittering studies.
2.4. Debittering processes
Four table olive debittering treatments, water treatment (W), scratching method (S), alkaline treatment (L) (NaOH) and enzymatic hydrolysis (EH), were conducted in triplicate. For water treatment, olives were rinsed with water only and left in the water for 33 days by changing the water twice a week. As for lye (NaOH) treatment, two different NaOH concentrations (0.5 and 1%, w/v) were used and left for incubation for 22 h. For the scratching method, the washed and sorted olives (Olea europeae L.) after scratching were added to the jar in water containing 1% citric acid (¾ container volumes) and incubated for 24 h at room temperature after scratching.
For enzymatic hydrolysis (EH), two different enzyme sources and four different enzymatic treatment methods have been used: Free Commercial enzyme preparation (Rapidase® 2000) (CE) which is of fungal origin, immobilized commercial β-glucosidase (ICE), Free β-glucosidase purified from Olea europeae L. (E), and immobilized β-glucosidase purified from Olea europeae L. (IE). Olives for enzymatic treatments were left at room temperature for 24 h under continuous agitation, which is a process to prevent the precipitations of magnetic nanoparticles used for immobilization matrix. Samples for HPLC analysis were taken at two different time points, 6 h and 22 h ().
Table 1. Process conditions for debittering Edremit cv. olives.
Tabla 1. Condiciones del método para desamargar aceitunas de Edremit cv.
Owing to the availability of commercial enzyme, three different concentrations of Commercial enzyme were applied for olives debittering, namely 0.25%, 0.5%, and 1% for 6 h. For reusability of β-glucosidase-SPMN (IE and ICE), the magnet was used for removing enzyme source immobilized to SPMNPs.
2.5. Enzyme extraction
Acetone powder was used as an enzyme source. 0.5 g of acetone powder of olive fruits was added into 50 mL of buffer (4°C) with borate (pH 9.0), 5 mM EDTA, 1 mM phenylmethane sulfonyl fluoride (PMSF), and 0.25% (w/v) dithiothreitol (DTT), and solution is stirred using a Micra XRT homogenizer (Kara, Sinan, & Turan, Citation2011). The homogenate was centrifuged at 15,300 rpm for 30 min at 4°C (Thermo), and the solid residue after centrifugation was obtained as the raw extract (Romero-Segura, Sanz, & Perez, Citation2009).
2.6. β-glucosidase purification
The purification of β-glucosidase from olive was achieved in two steps procedure (Kara et al., Citation2011). Specifically, ammonium sulfate precipitation was carried out followed by the hydrophobic interaction chromotography. The crude extract was precipitated with ammonium sulfate—of 0–60% saturation concentration by centrifuging at 15,000 rpm for 30 min (Thermo). The solid residue was dissolved in 50 mM of sodium phosphate buffer (pH 6.8). Final salt concentration was 1 mM before applied into the hydrophobic column. All steps were performed at 4°C. Before loading 3 mL of enzyme solution, the hydrophobic column was balanced with 50 mm of sodium phosphate buffer (pH 6.8). 1 mL fractions of enzyme elution were obtained with the linear gradient of 1.0–0.0 M (NH4)2SO4 at a flow rate of 30 mL/h. Tubes with the highest enzyme activity were pooled for the further steps (Kara et al., Citation2011).
2.7. Immobilization of β-glucosidase enzymes
Two different enzyme sources were used for immobilization assays, namely olive β-glucosidase (E) and commercial β-glucosidase (CE). SPMNs were individually synthesized and characterized for enzyme immobilization. 25 mg of SPMNs was placed into 2 mL of Buffer A (0.003 M phosphate, pH 6, 0.1 M NaCl), and 0.5 mL of carbodiimide solution (0.025 g/mL in buffer) was placed into the reaction medium. The reaction mixture was sonicated for 10 min (Thermo). 2 mL (22.9 µg) of purified β-glucosidase or commercial enzyme was added, and the mixture was sonicated for 30 min.
2.8. Activity and protein measurements of β-glycosidase
In the extraction, purification, and immobilization steps, β-glucosidase activity was measured at 410 nm using para-nitrophenyl-β-d-glucopyranosides (p-NPG) as the substrate. 70 μL of enzyme solution in 50 mM of sodium acetate, pH 5.5 and 70 µL of the substrate were mixed in the wells of a 96-well microtiter plate in triplicate. Well plate content was incubated at 37°C for 30 min, and the reaction was stopped by adding 70 μL of 0.5 M Na2CO3 and the absorbance was measured spectrophotometrically. Enzyme activity was uttered as μmol p-nitrophenol composed per minute in the reaction medium (Kara et al., Citation2011; Lowry, Rosebrough, Farr, & Randall, Citation1951).
2.9. Samples for HPLC analysis
Oleuropein was extracted from olive pulp, according to the procedure of Altınyay and Altun (Citation2006). 5 g of dried powdered olive samples was extracted in 50 mL of methanol for 2 h at 25°C by shaking (GFL). After filtration, extract was evaporated at 40°C under vacuum. Dryness residues were dispersed in 50 mL of HPLC grade methanol. Solutions were filtered through a 0.45 µm membrane (Millipore), and injection volume was 20 µL. Every sample injection was done in triplicate. Samples analyzed by HPLC are listed in . Raw olive fruits were used for reference of oleuropein in triplicate and extracted in the same manner (Altınyay & Altun, Citation2006).
2.10. HPLC-DAD system for analysis of oleuropein
Oleuropein analysis of olive samples was achieved by diode array detector (DAD), isocratic elution of water–acetonitrile–formic acid (84.6:15:0.4) (v/v/v), with a flow rate of 1.2 mL.min−1, at 240 nm. All solvents were filtered through a 0.45 µm filter before use and degassed in an ultrasonic bath. Injection volume was 20 µL, and the chromatographic run time was 40 min (Agilent).
2.11. Statistical analysis
Data are presented as the mean ± standard deviation of each treatment. Data were analysed for statistical significance using analysis of variance (ANOVA) followed by the Tukey test (p < 0.05) (SigmaPlot 11.0, SPSS Inc., USA).
3. Results and discussion
3.1. The activity of immobilized β-glucosidase
Enzymes from different sources might have different stabilities and properties. Thus, we tried two different enzyme sources, olive β-glucosidase (E) and commercial β-glucosidase (CE), to find the best enzyme sources for this debittering process.
Olea europaea β-glucosidase was purified for the immobilization and debittering process. Specifically, Sepharose-4B-l-tyrosine-1-napthylamine hydrophobic interaction chromatography was used for that purpose. The purification fold was 40.65, with an overall enzyme yield of 13.94%. The molecular mass of the protein was estimated as 65 kDa (data are not shown). The purified β-glucosidase was effectively active on p-/o-nitrophenyl-β-d-glucopyranosides (p-/o-NPG) with Km values of 0.8 mM and Vmax values of 192 EU. 22,6 ug of β-glucosidase purified from olive fruit was immobilized onto 25mg of SPMN. For commercial enzyme immobilization, 100 mg of SPMN was immobilized by 78 µg of commercial enzyme (CE) (). These amounts were chosen from our previous immobilization optimization studies, leading to 100% immobilization effeiciency,
Table 2. Activity and protein amounts of enzyme (β-glucosidase) sources used in debittering process.
Tabla 2. Actividad y cantidades de proteína de fuentes de la enzima (β-glucosidasa) utilizada en el método de desamargado.
Enzymes were monitored for enzyme activity changes after immobilizations (). CE has statistically higher activity than the one from olea europeae origin. CE is derived from a fungal origin. Species-specific enzyme activity differences are a well-known phenomenon and are reported in different studies (Briante et al., Citation2004). There are not any significant differences in the activity of free ezymes, E and CE, and immobilized enzymes, IE and ICE. When compared the suitability of storage conditions required for reuse of both enzyme source, the immobilized enzymes were determined to be more stable (data are not shown).
3.2. Use of different debittering methods
Oleuropein was monitored using HPLC assay to ensure that it was completely hydrolyzed. The summary of HPLC results is shown in . The initial oleuropein amounts of Edremit olive extracts were determined as 4917.0 ± 125.5 mg/kg. According to the Yorulmaz, Poyrazoglu, Özcan, and Tekin (Citation2012), Edremit variety olives have 3956.32 mg/kg of oleuropein (Yorulmaz et al., Citation2012). Dagdelen, and colleques claimed that the initial oleuropein amounts of Ayvalık (Edremit cv) 209.58 mg/kg and reduced during ripening stage of olive fruits (Dagdelen et al., Citation2013).
Table 3. Oleuropein amounts in olives debittered by different methods.
Tabla 3. Cantidad de oleuropeína en aceitunas desamargadas por diferentes métodos.
Oleuropein amounts of olives varied depending on the treatment type (). The duration of the hydrolysis of oleuropein and debittering has changed depending on the concentration of NaOH used (). After 22 h, 0.5% and 1% of NaOH were capable to hydrolase oleuropein by 92.5% and 94.84% respectively. However, NaOH leads to the reduction of other olive phenolics as well as oleuropein (Bianchi, Citation2003). In the fermentation stage, samples treated with lye have lost their eating quality because of the growth of mould. During alkaline treatment and following washing process of industrial table olive production, polar compounds such as reducing sugars and organic acids as well as oleuropein from the olive are removed (Restuccia et al., Citation2011). Meanwhile, the process leads to softening and extracting of carbon source for lactic acid bacteria in the fruit from the pulp. Taste and flavor are formed by the action of environmental lactic acid flora in the traditional fermented table olives. If they could not get sufficient nutrients in the environment, poor flavor development will be inevitable as well as softening (Pistarino et al., Citation2013). Cocolin et al. (Citation2013) declared that, when the debittering process was carried out by NaOH treatment, a decrease in the number of L. plantarum biotypes they require was observed, and those originating from the surface of the olive did not differentiate from the ones present in the amounts of brines (Cocolin et al., Citation2013).
Traditional olive production methods consist of water usage or brine replacement steps for debittering of olives. Both of these methods keep nutritional and sensorial qualities of olives from the tree. However, these methods are not suitable for industrial production, because of long processing time. Similarly, our study also shows, after 22 h, the olive samples debittered with water lost only 0.33% of oleuropein (). This value compared with lye treatment demonstrates the unsuitability for industrial application. Water and scratching treatments caused a considerable reduction of the oleuropein amounts after 33 days ().
Enzymatic debittering provides natural changes in the olives and prepares for natural fermentation of table olives Ciafardini & Zullo, Citation2000) and production of olive oil (Ciafardini & Zullo, Citation2002). In our study, β-glucosidase from olive origin was used in the table olive production as well as β-glucosidase from fungal origin (CE) to choose the best enzyme sources. As far as the effect of immobilization is considered, oleuropein hydrolization by IE was more effective than the one by purified enzyme (C), with 50% reduction of oleuropein after 6 h of incubation time. The amount of oleropein was nearly completely lost after 22 h of incubation time ().
To determine the effect of different amounts of free and immobilized enzyme on the oleuropein hyrolysation, 0.25%, 0.5%, and 1% of CE and ICE were utilized in the fermentation vessels for 6 h (). As shown in , concentration dependent reductions of oleuropein hyrolysation were observed, and it is more obvious for the immobilized enzyme application. Commercial β-glucosidase (CE) and immobilized form (ICE) (1%) caused a decrease in oleuropein amount by 62.05% and 80.01% after 6 h, respectively, and also it is completely reduced after 22 h. Consistent with olive β-glucosidase, ICE enzyme is more effective than free enzyme, CE.
Table 4. Oleuropein amounts in olives debittered by different concentrations of commercial enzyme source for 6 h.
Tabla 4. Cantidades de oleuropeína en aceitunas desamargadas por diferentes concentraciones de fuente comercial de enzimas durante 6 h.
Overall, 1% of immobilized commercial enzyme application seems to be more effective than immobilized olive β-glucosidase after 6 h. However, it should be taken into account that more amount of enzyme is present in the vessel compared to the one with olive E ( and ).
Several authors have previously studied the enzymatic hydrolysis of oleuropein by microbial recombinant β-glucosidases or crude olive extracts (Briante et al., Citation2004). Especially, immobilized forms of these molecules showed high catalytic activity. At the beginning of the fermentation process of olives not treated with alkaline solution, the hydrolysis of oleuropein can be attributed to the β-glucosidase produced by oleuropeinolytic microorganisms (Ruiz-Barba, Brenes-Balbuena, Jiménez-Díaz, García-García, & Garrido Fernández, Citation1993).
3.3. Determination of the number of sequential usages of immobilized enzyme
To evaluate reusability of immobilized enzyme, the immobilized enzyme is used in the debittering process to evaluate reusability. Bissett and Sternberg (Citation1978) immobilized Aspergillus β-glucosidase onto chitosan polymers and reported some difficulties in the use of IE such as low specific activity (Bissett & Sternberg, Citation1978). They also indicated that the immobilized material would have to be reused at least 10 times just to compete economically with using free, β-glucosidase without recovery.
Different amounts of immobilized β-glucosidase were used for 22 h to optimize the usage of immobilized β-glucosidase (IE) to remove the bitterness of olives. During 22 h, the treatment of 30–60 g fruit with the immobilized enzyme (IE) caused a hydrolysis rate of 90–99% of oleuropein in olives (). In some trials, the bitterness of fruits disappeared completely. Then, the same concentrations of IE were used to debitter olives repeatedly (). It was determined that the enzyme is still active after the fourth trial. However, the debittering effect was not equivalent to the first four applications ().
Figure 1. Different amounts of β-glucosidase (BG) and SPMN effects on oleuropein amounts of olives debittered after first, second, third, and fourth trials.
Figura 1. Efectos de diferentes cantidades de β-glucosidasa (BG) SPMN en cantidades de oleuropeínas de aceitunas desamargadas después de 1, 2, 3 y 4 ensayos.
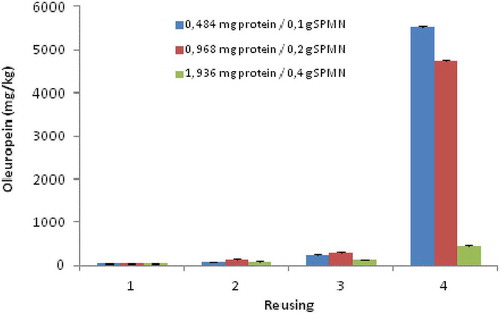
Figure 2. The amount of oleurepein obtained from different immobilisation ratio and the number of trials.
Figura 2. La cantidad de oleurepeína obtenida de diferente tasa de inmovilización y el número de ensayos.
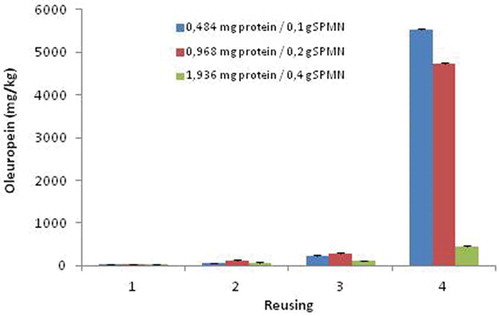
Figure 3. DAD chromatograms at 240 nm of methanol extracts of olive samples debittered by β-glucosidase in different concentrations. (A) 0.25% ICE, 6 h process; (B) 0.5% ICE, 6 h process; (C) 1% ICE, 6 h process; (D) 0.25% CE, 6 h process; (E) 0.5% CE, 6 h process; (F) 1% CE, 6 h process; (G) 0.1% E, 6 h process; (H) SPMN, 6 h process; (I) 1% IE, 6 h process; (J) 1% IE, 22 h process; (K) sctracthing method, 33 days process; (L) debittering with water 33 days process.
Figura 3. Cromatogramas DAD (detector de red de diodos) a 240 nm de extractos de metanol de muestras de aceitunas desamargadas usando β-glucosidasa en distintas concentraciones. (A) 0.25% ICE, proceso de 6 horas; (B) 0.5% ICE, proceso de 6 horas; (C) 1% ICE, proceso de 6 horas; (D) 0.25% CE, proceso de 6 horas; (E) 0.5% CE, proceso de 6 horas; (F) 1% CE, proceso de 6 horas; (G) 0.1% E, proceso de 6 horas; (H) SPMN, proceso de 6 horas; (I) 1% IE, proceso de 6 horas; (J) 1% IE, proceso de 22 horas; (K) método de rascado, proceso de 33 días; (L) desamargado con agua, proceso de 33 días.
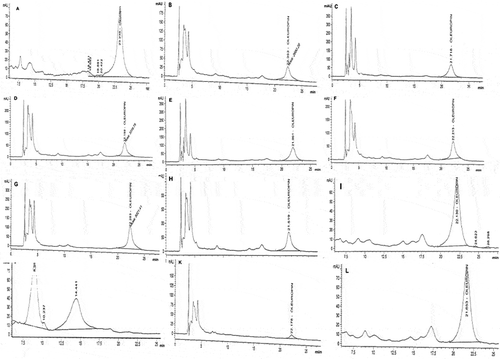
As a result, the use of the 0.1 g nanoparticle-bound enzyme of 91.6 μg completely hydrolyzed oleuropein of 60 g olives up to 22 h treatment.
4. Conclusions
The olive β-glucosidase and commercial β-glucosidase enzymes were immobilized onto SPMNPs by carbodiimide activation. The olive β-glucosidase, β-glucosidase-bound SPMNPs, commercial β-glucosidase, and immobilized commercial enzyme were comparatively studied, and also the enzyme activity was spectrophotometrically quantified. It is found that the commercial β-glucosidase has higher activity than olive β-glucosidase and its immobilized form owing to species-specific properties. In addition, the activities of free and immobilized enzyme sources for both enzyme types were in the same level. After the debittering process, the immobilized enzymes (purified from olive and commercial one) could easily be separated from the reaction medium via a simple magnet. The immobilized forms of the enzymes can be easily and effectively reused more than four times for debittering. A novel method under study was developed with the economical, fast, and reusable properties for the industrial debittering process in the table olive production.
Acknowledgments
This study was supported by The Scientific and Technological Research Council of Turkey Project Grant No. 110O778 and Scientific Research Projects Unit of Balıkesir University Grant No. BAP.2015.0002. The authors would like to thank H. Kara, Ph.D., S. Sinan, Ph.D., and Mr. O. Eren from Central Research Institute of Food and Feed Control, Bursa, Turkey.
Disclosure statement
No potential conflict of interest was reported by the authors.
Additional information
Funding
References
- Alahakoon, T., Koh, J. W., Chong, X. W., & Lim, W. T. (2012). Immobilization of cellulases on amine and aldehyde functionalized Fe2O3 magnetic nano particles. Preparative Biochemistry and Biotechnology, 42, 234.
- Altınyay, Ç., & Altun, M. L. (2006). HPLC analysis of oleuropein in Olea Europaea L. Journal of Faculty of Pharmacy of Ankara University, 35, 1–11.
- Alu’datt, M. H., Alli, I., Ereifej, K., Alhamad, M., Al-Tawaha, A. R., & Rababah, T. (2010). Optimisation, characterisation and quantification of phenolic compounds in olive cake. Food Chemistry, 123, 117–122.
- Bianchi, G. (2003). Lipids and phenols in table olives. European Journal of Lipid Science and Technology, 105, 229–242.
- Bissett, F., & Sternberg, D. (1978). Immobilization of Aspergillus beta-glucosidase on chitosan. Applied and Environmental Microbiology, 35, 50–755. 0099-2240/78/0035-0750/$02.00/0
- Brenes Balbuena, M., Garcia Garcia, P., & Garrido Fernandez, A. (1992). Phenolic compounds related to the black color for medduring the processing of ripe olives. Journal of Agricultural and Food Chemistry, 40, 1192–1196.
- Brenes, M., Garcia, A., Garcia, P., & Garrido, A. (2001). Acid hydrolysis of secoiridoid aglycons during storage of virgin olive oil. Journal of Agricultural and Food Chemistry, 49, 5609–5614.
- Brenes, M., Rejano, L., Garcia, P., Sanchez, A., & Hand Garrido, A. (1995). Biochemical changes in phenolic compounds during Spanish-style green olive processing. Journal of Agricultural and Food Chemistry, 43, 2702–2706.
- Briante, R., Patumi, M., Febbraio, F., & Nucci, R. (2004). Production of highly purified hydroxytyrosol from Olea europaea leaf extract biotransformed by hyperthermophilic β-glycosidase. Journal of Biotechnology, 111, 67–77.
- Charoenprasert, S., & Mitchell, A. (2012). Factors INflUENCING PHENOLIC COMPOUNDS IN TABLE OLIVES (Olea europaea). Journal of Agricultural and Food Chemistry, 60, 7081−7095.
- Chen, D.-H., & Liao, M.-H. (2002). Preparation and characterization of YADH-bound magnetic nanoparticles. Journal of Molecular Catalysis B: Enzymatic, 16, 283–291.
- Ciafardini, G., & Zullo, B. (2000). β-Glucosidase activity in olive brine during the microbiological debittering process. Advances in Food Sciences, 22, 100–106. 10.1.1.509.5911
- Ciafardini, G., & Zullo, B. A. (2002). Microbiological activity in stored olive oil. International Journal of Food Microbiology, 75, 111–118.
- Ciafardini, G., Zullo, B. A., & Cioccia, G. (2005). Effect of lipase-producing yeast on the oily fraction of microbiologically debittered table olives. European Journal of Lipid Science and Technology, 107, 851–856.
- Cocolin, L., Alessandria, V., Botta, C., Gorra, R., De Filippis, F., Ercolini, D., & Rantsiou, K. (2013). NaOH-debittering induces changes in bacterial ecology during table olives fermentation. PloS One, 8(7), e69074.
- Dagdelen, A., Tümen, G., Özcan, M. M., & Dündar, E. (2013). Phenolics profiles of olive fruits (Olea europaea L.) and oils from Ayvalık, Domat and Gemlik varieties at different ripening stages. Food Chemistry, 136, 41–45.
- Esti, M., Cinquante, L., & La Notte, E. (1998). Phenolic compounds in different olive varieties. Journal of Agricultural and Food Chemistry, 46, 32–35.
- Ganguli, K. L., Van Putte, K. P., & Turksma, H. (1999). Debittering of Olive Oil, US Patent, 5,998,641.
- Gikas, E., Papadopoulos, N., & Tsarbopoulos, A. (2006). Kinetic study of the acidic hydrolysis of oleuropein, the major bioactive metabolite of olive oil. Journal of Liquid Chromatography & Related Technologies, 29, 497–508.
- Guinda, Á. (2006). Grasas Y Aceites. Use of Solid Residue from the Olive Industry, (1), Enero-Marzo, 107–115.
- Guiso, M., & Marra, C. (2005). Highlights in oleuropein aglycone structure. Natural Product Research, 19, 105–109.
- Jemai, H., Bouaziz, M., Fki, I., El Feki, A., & Sayadi, S. (2008). Hypolipidimic and antioxidant activities of oleuropein and its hydrolysis derivative rich extracts from Chemlali olive leaves. Chemico-Biological Interactions, 176, 88–98.
- Kara, H. E., Sinan, S., & Turan, Y. (2011). Purification of beta-glucosidase from olive (Olea europaeaL.) fruit tissue with specifically designed hydrophobic interaction chromatography and characterization of the purified enzyme. Journal of Chromatography B, 879, 1507–1512.
- Karaagac, O., & Kockar, H. (2012). Effect of synthesis parameters on the properties of superparamagnetic iron oxide nanoparticles. Journal of Superconductivity and Novel Magnetism, 25(8), 2777–2781.
- Karaagac, O., Kockar, H., Beyaz, S., & Tanrisever, T. (2010). A simple way to synthesize superparamagnetic iron oxide nanoparticles in air atmosphere: Iron ion concentration effect. IEEE Transactions on Magnetics, 46(12), 3978–3983.
- Kockar, F., Beyaz S, Sinan, S., Kockar, H., Demir, D., Eryilmaz, S., Tanrisever, T., & Arslan, O. (2010). Paraoxonase 1-bound magnetic nanoparticles: Preparation and characterizations. Journal Of Nanoscience And Nanotechnology, 10(11), 7554–7559.
- Kubo, A., Lunde, C. S., & Kubo, I. (1995). Antimicrobial activity of the olive oil flavor compounds. Journal of Agricultural and Food Chemistry, 43, 1629–1633.
- Kumar, S., Jana, A. K., Dhamija, I., Singla, Y., & Maiti, M. (2013). Preparation, characterization and targeted delivery of serratiopeptidase immobilized on amino-functionalized magnetic nanoparticles. European Journal of Pharmaceutics and Biopharmaceutics, 85, 413–426.
- Lavermicocca, P., Valerio, F., Lonigro, S. L., Baruzzi, F., Morea, M., & Gobetti, M. (2002). Acta Horticulturae, 586, 621–624.
- Liburdi, K., Straniero, R., Benucci, I., Vittoria Garzillo, A. M., & Esti, M. (2012). Lysozyme immobilized on micro-sized magnetic particles: Kinetic parameters at wine pH. Applied Biochemistry and Biotechnology, 166, 1736.
- Lowry, O. H., Rosebrough, N. J., Farr, A. L., & Randall, R. J. (1951). Protein measurement with Folin phenol reagent. The Journal of Biological Chemistry, 193, 256–275. classics1977/A1977DM02300001.pdf
- Malik, N. S. A., & Bradford, J. M. (2006). Changes in oleuropein levels during differentiation and evelopment of floral buds in ‘Arbequina’ Olives. Scientia Horticulturae, 110, 274–278.
- Marsilio, V., Lanza, B., & Pozzi, N. (1996). Progress in table olive debittering: Degradationin vitro of oleuropein and its derivatives by Lactobacillus plantarum. Journal of the American Oil Chemists’ Society, 73, 593–597.
- Medina, E., Garcı´A, A., Romero, C., De Castro, A., & Brenes, M. (2009). Study of the anti-lactic acid bacteria compounds in table olives. International Journal of Food Science & Technology, 44, 1286–1291.
- Onem, H., Cicek, S., & Nadaroglu, H. (2016). Immobilization of a thermostable phytase from Pinar melkior (Lactarius piperatus) onto magnetite chitosan Nan particles. CyTA – Journal of Food, 14(1), 74–83.
- Ozel, F., & Kockar, H. (2015). Growth and characterizations of magnetic nanoparticles under hydrothermal conditions: Reaction time and temperature. Journal of Magnetism and Magnetic Materials, 373, 213–216.
- Ozel, F., Kockar, H., & Karaagac, O. (2015). Growth of iron oxide nanoparticles by hydrothermal process: Effect of reaction parameters on the nanoparticle size. Journal of Superconductivity and Novel Magnetism, 28(3), 823–829.
- Paiva-Martins, F., & Gordon, M. H. (2001). Isolation and characterization of the antioxidant component 3,4-dihydroxyphenylethyl 4-formyl-3-formylmethyl-4-hexenoate from olive (Olea europaea) leaves. Journal of Agricultural and Food Chemistry, 49, 4214–4219.
- Pistarino, E., Aliakbarian, B., Casazza, A. A., Paini, M., Cosulich, M. E., & Perego, P. (2013). Combined effect of starter culture and temperature on phenolic compounds during fermentation of Taggiasca black olives. Food Chemistry, 138, 2043–2049.
- Restuccia, C., Muccilli, S., Palmeri, R., Randazzo, C. L., Caggia, C., & Spagna, G. (2011). An alkaline β-glucosidase isolated from an olive brine strain of Wickerhamomyces anomalus. FEMS Yeast Research, 11(6), 487–493.
- Romani, A., Mulinacci, N., Pinelli, P., Vincieri, F. F., & Cimato, A. (1999). Polyphenolic content in five tuscany cultivars of Olea europaea L. Journal of Agricultural and Food Chemistry, 47, 964–967.
- Romero, C., Brenes, M., Garcia, P., Garcia, A., & Garrido, A. (2004a). Polyphenol changes during fermentation of naturally black olives. Journal of Agricultural and Food Chemistry, 52, 1973–1979.
- Romero, C., Brenes, M., Yousfi, K., Garcia, P., Garcia, A., & Garrido, A. (2004b). Effect of cultivar and processing method on the contents of polyphenols in table olives. Journal of Agricultural and Food Chemistry, 52, 479–484.
- Romero, C., Garcia, P., Brenes, M., Garcia, A., & Garrido, A. (2002). Phenolic compounds in natural black Spanish olive varieties. European Food Research and Technology, 215, 489–496.
- Romero-Segura, C., Sanz, C., & Perez, A. G. (2009). Purification and characterization of an olive fruit beta-glucosidase involved in the biosynthesis of virgin olive oil phenolics. Journal of Agricultural and Food Chemistry, 57, 7983.
- Ruiz-Barba, J. L., Brenes-Balbuena, M., Jiménez-Díaz, R., García-García, P., & Garrido Fernández, A. (1993). Inhibition of Lactobacillus plantarum by polyphenols extracted from two different kinds of olive brine. Journal of Applied Microbiology, 74(1), 15–19.
- Savas, E., & Uylaser, V. (2013). Quality improvement of green table olive cv. ‘Domat’ (Olea europaea L.) grown in Turkey using different de-bittering methods. Notulae Botanicae Horti Agrobotanici Cluj-Napoca, 41(1), 269–275.
- Soler-Rivas, C., Espin, J. C., & Wichers, H. J. (2000). Oleuropein and related compounds. Journal of the Science of Food and Agriculture, 80, 1013–1023.
- Webb, G. A., Belton, P. S., Gil, A. M., Delgadillo, I., Capozzi, F., Cremonini, M. A., … Uccella, N. (2001). Oleuropein biomimetic conformations by magnetic resonance experiments and molecular mechanics and dynamics. Magnetic Resonance in Food Science: A View to the Future, 2001, 129–135.
- Yorulmaz, A., Poyrazoglu, E. S., Özcan, M. M., & Tekin, A. (2012). Phenolic profiles of Turkish olives and olive oils. European Journal of Lipid Science and Technology, 114, 1083–1093.
- Yu, C. C., Kuo, Y. Y., Liang, C. F., Chien, W. T., Wu, H. T., Chang, T. C., … Lin, C. C. (2012). Site-specific immobilization of enzymes on magnetic nanoparticles and their use in organic synthesis. Bioconjugate Chemistry, 23, 714−724.
- Zhu, X., Ma, Y., Zhao, C., Lin, Z., Zhang, L., Chen, R., & Yang, W. A. (2014). Mild strategy to encapsulate enzyme into hydrogel layer grafted on polymeric substrate. Langmuir, 30, 15229−15237.