ABSTRACT
The objective of the current study was to determine the phenolic composition and carotenoid contents of leaves, bark, and fruits of Malpighia umbellata, assess the changes in the phenolic composition and contents of carotenoids and vitamin C of its fruits in different stages of maturity, and evaluate the antioxidant properties of its leaves, bark, and fruits. Leaves accumulated the highest levels of flavonoids (10.55 mg/g dry extract), tannins (21.16 mg/g dry extract), and carotenoids (424 63 µg/g dry tissue); whereas, the highest level of total phenolics was found in bark (47.12 mg/g dry extract). The vitamin C content decreased with the maturity of fruits. Twenty-two phenolics were characterized by high performance liquid chromatography with diode array detection (HPLC-DAD). Apigenin-7-O-glycosides, phenolic acids, and flavonols were predominant in leaves, bark, and fruits, respectively. Important chemical variations were found during fruit ripening. The 2,2-diphenyl-1-picrylhydrazyl (DPPH•) scavenging activity and 2,2ʹ-azino-bis-(3-ethylbenzothiazoline-6-sulfonic acid) (ABTS) inhibition assays revealed important antioxidant properties for this acerola.
RESUMEN
El objetivo del presente estudio fue determinar la composición fenólica y contenidos de carotenoides de las hojas, corteza, y frutos de Malpighia umbellata; los cambios en la composición fenólica y contenidos de carotenoides y vitamina C de sus frutos durante la maduración; y las propiedades antioxidantes de sus hojas, corteza, y frutos. Las hojas acumularon los niveles más altos de flavonoides, taninos (10.55 y 21.16 mg/g extracto seco, respectivamente), y carotenoides (424 63 µg/g tejido seco); el nivel más alto de fenoles totales se encontró en corteza (47.12 mg/g extracto seco). El contenido de vitamina C disminuyó con la madurez de los frutos. Veintidós compuestos fenólicos se caracterizaron por HPLC-DAD. Apigenina-3-O-glicósidos fueron predominantes en hojas, ácidos fenólicos en corteza, y flavonoles en frutos. Se encontraron importantes variaciones químicas durante la madurez de los frutos. Los ensayos de 2,2-difenil-1-picrilhidracilo (DPPH) y 2,2ʹ-azino-bis-(3-etilbenzotiazolin-6-ácido sulfónico) (ABTS) revelaron importantes propiedades antioxidantes para esta acerola.
Introduction
The genus Malpighia (Malpighiaceae) comprises about 130 species, all naturally distributed in the tropical and subtropical regions of the American Continent (Meyer, Citation2000). Some species of the genus are economically important, such as Malpighia emarginata (Hanamura, Hagiwara, & Kawagishi, Citation2005; Marques et al., Citation2016; Mezadri, Fernández-Pachón, Villaño, García-Parrilla, & Troncoso, Citation2006), Malpighia glabra (Horta et al., Citation2016), and Malpighia punicifolia (Vendramini & Trugo, Citation2000), and their edible fruits have been extensively studied. Belwal et al. (Citation2018) made a review of the bioproducts and biological properties of fruits of M. emarginata and M. glabra, reporting the accumulation of benzoic acid derivatives, phenylpropanoid derivatives, flavonoids, anthocyanins, and several carotenoids as β-carotene, neochrome, neoxanthin, and violaxanthin, among others, as well as the antihyperglycemic, antihyperlipidemic, antioxidant, anti-inflammatory, hepatoprotective, antimutagenic, antimicrobial, antitumor, and antiobesity properties for these fruits.
Malpighia emarginata, M. glabra, and M. punicifolia are cultivated, rich in vitamin C, and indistinctly named acerola. Malpighia umbellata is another edible acerola, but unlike M. emarginata, M. glabra, and M. punicifolia, it is a wild species, which is endemic from the tropical regions of northeastern Mexico (Vega-Aviña, Aguilar-Hernández, Gutiérrez-García, & Hernández-Vizcarra, Citation2000).
The exploration of the abundance and diversity of bioactive compounds of wild edible and medicinal plants offers the opportunity to develop new compounds to improve human health, as well as to locate sources of valuable alleles to develop cultivars with enhanced accumulation of bioproducts. Despite the important studies already done, there are many wild species of which the bioproduct composition and biological properties are unknown, such as M. umbellata. Phenolic compounds and carotenoids are among the main bioproducts owing to their contribution to determine the organoleptic and antioxidant properties of plant foods, and their important and diverse beneficial effects on human health (Sevgi, Tepe, & Sarikurkcu, Citation2015; Yamagata, Citation2017).
To the best of our knowledge, the phytochemical composition and antioxidant properties of M. umbellata have not been studied. The objective of the current paper was to determine (1) the phenolic composition and carotenoid contents of leaves, bark, and fruits of M. umbellata, (2) the changes in the phenolic composition, as well as in the contents of carotenoid and vitamin C of its fruits at different stages of maturity, and (3) the antioxidant properties of its leaves, bark, and fruits.
Material and methods
Plant material
Samples were collected in San Ignacio Island, Navachiste Bay, Sinaloa, Mexico (25° 26ʹ 2.64ʺ N, 108° 54ʹ 42.03ʺ W). Leaves and bark were collected from 10 adult trees (1.5 kg/tree), in August 2016. Fruits at different maturity stages were collected from 10 trees (50 fruits per each maturity stage/tree) in October 2016. The analyzed maturity stages were S1: green fruits, 0.8–1.1 cm equatorial diameter (ED); S2: yellowish-green fruits, 1.2–1.3 cm ED; S3: yellow fruits, 1.2–1.3 cm ED; S4: yellowish red, 1.4–1.5 cm ED; S5: red fruits, 1.4–1.5 cm ED. Immediately after collected, samples of the same type were combined and for each, three subsamples (three replicas) were formed, dried to constant weight in a ventilated oven at 37°C (fruits were previously prepared in thin slices), ground and sieved through 250 µm mesh, and kept in plastic bags at room temperature until use (no more than three weeks). A voucher specimen was collected and deposited in the Herbarium CIIDIR (curatorial number CIIDIR-45464). Each subsample was separately analyzed.
Preparation of phenolic extracts
Four grams of the sample was macerated in 40 mL of 80% methanol (v/v) for 24 h, in darkness, and at room temperature. After centrifugation (5 min, 6000 rpm), supernatants were separated, and pellets were re-extracted in 30 mL of 20% methanol (v/v) for 4 h. After centrifugation, both supernatants were combined, concentrated under vacuum to dryness, and then re-dissolved in 5 mL methanol. Aliquots were taken to determine the contents of diverse phenolic compounds, antioxidant properties, and to carry out the high performance liquid chromatography with diode array detection (HPLC-DAD) analyses.
Total phenolics (TF)
The phenolic contents were determined using the Folin–Ciocalteu reagent, according to Singleton and Rossi (Citation1995), and were calculated from a standard curve of gallic acid (slope = 0.0466, axis crossing point = −0.0455, r = 0.9983). TF was expressed as milligrams of gallic acid equivalents per gram of dry extract (mg GAE/g DE).
Condensed tannins (CT)
Condensed tannins were determined according to Julkunen-Tiitto (Citation1985), and were calculated from a standard curve of epicatechin (slope = 0.0113, axis crossing point = −0.0025, r = 0.9953). CT was expressed as milligrams of epicatechin equivalents per gram of dry extract (mg ECE/g DE).
Anthocyanins
Monomeric anthocyanins of fruits were determined with the differential pH method described by Giusti and Wrolstad (Citation2001), using the molecular weight (449.2) and the molar absorption (26,900) of cyanidin-3-O-glucoside as reference, due to this anthocyanin is predominant in other species of Malpighia (de Rosso et al., Citation2008). The values were expressed as milligrams per dry extract (mg/g DE).
Carotenoids
Carotenoids were extracted and analyzed according to Thompson et al. (Citation2000), using a standard curve of β-carotene (slope = 0.2785, axis crossing point = −0.0033, r = 0.999). Carotenoid contents were expressed as micrograms of β-carotene equivalents per gram of dry tissue (µg β-CE/g DT).
Vitamin C
Vitamin C contents of dry fruits were determined according to Bajaj and Kaur (Citation1981), using a standard curve of ascorbic acid (slope = 0.0966, axis crossing point = −0.0753, r = 0.981). The contents were expressed as micrograms of ascorbic acid equivalents per gram of dry tissue (µg AAE/g DT).
Phenolic profiles
The phenolic profiles of the different parts of M. umbellata were determined by HPLC-DAD, in a Perkin Elmer Series 200 HPLC system and a Perkin Elmer Brownlee Analytical C18 (4.6 × 250 mm, 5 µm) column, using the gradient method described by Campos and Markham (Citation2007). Water adjusted to pH 2.5 with orthophosphoric acid was the solvent A and acetonitrile was the solvent B. Both solvents were mixed according to the following gradient: starting with 100% A, decreasing to 91% over the next 12 min, to 87% over the next 8 min, to 67% over the next 10 min, holding this composition for 2 min, then A was decreased to 57% over the next 10 min. This last proportion was held until the end of the 60 min analysis. Injection volume was 50 µL. The flow rate was 0.8 mL/min at 25°C. Chromatograms were registered at 260 and 340 nm. Spectral data for all peaks were collected in the range of 200–400 nm by using DAD (Perkin Elmer Series 200). Structural information of resolved compounds was obtained taking into account the number and λmax of bands and shoulders, as well as the whole shape of the UV spectra according to the UV theory developed for flavonoids and phenolic acids by Campos and Markham (Citation2007), and also comparing the retention time (RT) and UV spectra with those of the following reference compounds: chlorogenic acid (TR: 27.76 min; λmax: 243sh, 296sh, 326), caffeic acid (TR: 53.13 min; λmax: 239sh, 295sh, 318), p-coumaric acid (TR: 37.2 min; λmax: 294sh, 308), quercetin (TR: 47.05 min; λmax: 255, 268sh, 299sh, 370), rutin (quercetin-3-O-rutinoside; TR: 33.74 min; λmax: 255, 264sh, 294sh, 355), quercitrin (quercetin-3-O-rhamnoside; TR: 38.54 min; λmax: 255, 264sh, 295sh, 348), apigenin (TR: 59.60 min, 267, 290sh, 335), and naringenin (TR: 52.25 min, λmax: 289, 335sh). The phenolic profile of each sample was formed by all compounds resolved in the respective chromatogram. The concentration of a single compound was estimated by area measurement from a standard curve of rutin (slope = 6564.40, axis crossing point = 127.33, r = 0.9965) for flavonols, apigenin (slope = 79,678, axis crossing point = −2564.6, r = 0.9949) for flavones, and chlorogenic acid (slope = 39,870, axis crossing point = 6372.9, r = 0.9985) for phenolic acids. The contents were expressed as milligrams per gram of dry extract (mg/g DE). The sum of individual flavonoid concentrations in a single sample represented the total flavonoid concentration.
DPPH• (2,2-diphenyl-1-picrylhydrazyl) scavenging activity
The DPPH• assay was carried out according to Medina-Medrano et al. (Citation2015). Absorbance diminutions (at 523 nm) of a DPPH• solution against increasing extract concentrations were plotted (slope = −67.581, axis crossing point = −0.2614, r = 0.9968) to calculate the antioxidant capacity as the amount of antioxidant needed to decrease by 50% the initial DPPH• concentration (EC50 (DPPH)). EC50 (DPPH) was expressed as micrograms per milliliter (µg/mL). Quercetin, epicatechin, and caffeic acid were analyzed as references.
ABTS∙+ reducing capacity
The ABTS assay was carried out according to Re et al. (Citation1999). The extract concentration providing 50% inhibition of ABTS•+ radical (EC50 (ABTS)) was calculated from a plot constructed with the values of percentage of activity against extract concentration (slope = −11.451, axis crossing point = 0.7292, r = 0.985). EC50 (ABTS) was expressed as micrograms per milliliter (µg/mL). Quercetin, epicatechin, and caffeic acid were analyzed as references.
Data analysis
The analyses were carried out for three independent pools of each sample. Data were subjected to an analysis of variance (p < 0.05) and means separated by Duncan’s multiple range test. To assess the contribution of total phenolics, flavonoids, condensed tannins, anthocyanins, carotenoids, and vitamin C to distinguish fruits in different stages of maturity, data were subjected to a principal components analysis (PCA). Another PCA was carried out to determine the contribution of the concentration of each individual phenolic compound for the discrimination of fruits in different stages of maturity. PCAs were carried out using Past 3.0.
Results and discussion
Phenolic compounds contents
TF contents are displayed in . Leaves and bark accumulated the highest levels. The phenolic composition of leaves and bark of other species of Malpighia have been little explored. The few published studies reported only qualitative phytochemical screenings (Khatun et al., Citation2014) or expressed the contents differently from the present study (Venugopal, Mallikarjun, Somasundar, Nagaraj, & Prabhuswamy, Citation2016), so comparisons of our results with those of other species of the genus cannot be done. Leaves of M. umbellata accumulated comparable levels of TF to those of the leaves of Aloysia triphylla (L’Hér.) Britton (52 mg/g dry weight), which is considered as an important source of antioxidant phenolics (Galvez, Kwon, Apostolidis, & Shetty, Citation2010); whereas, the bark accumulated higher TF levels than those of Terminalia catappa (37.30 mg/g), which is a plant with antihypertensive and antioxidant properties (Oyeleye, Adebayo, Ogunsuyi, Dada, & Oboh, Citation2017).
Table 1. Content of bioactive secondary metabolites in Malpighia umbellata.
Tabla 1. Contenido de metabolitos secundarios bioactivos de Malpighia umbellata.
The stage of maturity is one of the most important factors determining the chemical composition of fruits (Vendramini & Trugo, Citation2000). TF concentration decreased with fruit maturity of M. umbellata (), in disagreement with Hanamura, Uchida, and Aoki (Citation2008), who reported the increase of TF according to fruit maturity of M. emarginata. This variation suggests that even between related species, genetically controlled fruit ripening programs have emerged. The TF value of the mature fruits of M. umbellata was higher than those reported for Hanamura, Uchida, and Aoki (Citation2008) for the mature fruits of M. emarginata (between 1 and 4 mg/g dry weight, depending on cultivar), also higher than those reported by Delva and Goodrich-Schneider (Citation2013) for the mature fruits of the same species (M. emarginata, 8340 mg/kg), and comparable to those of the mature fruits of M. punicifolia (11.8 mg/g dry weight) (Malinowska, Gliszczyńska-Świgło, & Szymusiak, Citation2014).
Significant differences in the flavonoid contents were observed (): those of leaves and bark were higher than those of the aerial parts of Hedeoma drummondii Benth. (3.2 mg/g), a medicinal plant (Viveros-Valdez, Rivas-Morales, Carranza-Rosales, Mendoza, & Schmeda-Hirschmann, Citation2008). Fruit flavonoid concentrations increased with maturity, suggesting important functions of these compounds in the fruits of M. umbellata. The mature fruits of M. umbellata accumulated higher flavonoid levels than the mature fruits of M. punicifolia (0.28 mg/g dry extract) (Malinowska et al., Citation2014).
Condensed tannins were significantly more abundant in leaves than in bark and fruits (). The tannin level in the M. umbellata leaves was higher than in the leaves of Leucaena leucocephala hybrid-Rendang (2.06 mg/g) (Zarin, Wan, Isha, & Armania, Citation2016). However, in the bark, the tannin level of M. umbellata was lower than that reported for the bark of several species of Senna (32.50–212.30 mg/g), for which relevant and diverse biological properties were reported (Maia, Trevisan, Silva, Breuer, & Owen, Citation2017). In fruits, the tannin amount diminished according to ripeness, and at any stage it was higher than those reported by Santos-Buelga and Scalbert (Citation2000) for apple (0.50 mg/g dry weight). High levels of tannins are considered as toxic and antinutrient owing to its capacity to precipitate proteins and reduce nutrient bioavailability (Naumann et al., Citation2014); however, these compounds also have beneficial biological activities for human health, as antioxidants, anti-inflammatory, and blood glucose regulators (Kumari & Jain, Citation2012). The current results revealed M. umbellata as an important source of these compounds.
Anthocyanins were found in fruits at all stages of maturity (). In any stage, the fruits of M. umbellata accumulated higher anthocyanin levels than the mature fruits of M. emarginata (0.0469–0.0503 mg/g) (Mezadri, Villaño, Fernández-Pachón, García-Parrilla, & Troncoso, Citation2008). Recently, it was reported that anthocyanins improve the glucose intake in human skeletal muscle cells (Ho, Kase, Wangensteen, & Barsett, Citation2017) and that their bioavailability is higher than it was supposed (Kay, Pereira-Caro, Ludwig, Clifford, & Crozier, Citation2017); these findings stimulate the search for new sources of anthocyanins. The current results revealed the fruits of M. umbellata as an important source of these phenolic compounds.
Phenolic profiles
The HPLC-DAD analysis revealed seven major foliar phenolic compounds (). Compounds 1–4, 6, and 7 were derivatives, 7-O-glycosides, of the flavone apigenin, and compound 5 was a derivative, 3-O-glycoside, of the flavonol quercetin. The most abundant compound was 4 (). Important antifungal and cytotoxic effects against colon cancer cells have been reported for apigenin-7-O-glycosides (Smiljkovic et al., Citation2017), which are abundant in the foliar tissues of M. umbellata.
Figure 1. HPLC chromatogram and DAD-obtained UV spectra of the foliar phenolic compounds from Malpighia umbellata leaves. HPLC chromatogram was registered at 340 nm with an acidified water–acetonitrile gradient method. DAD-obtained UV spectra were obtained between 200 and 400 nm. Retention time (min) and λmax (in italics) are indicated.
Figura 1. Cromatograma y espectros UV obtenidos por HPLC-DAD de los compuestos fenólicos de hojas de Malpighia umbellata. El cromatograma se registró a 340 nm con un método en gradiente de agua acidificada y acetonitrilo. Los espectros UV se obtuvieron entre 200 y 400 nm. Se indican el tiempo de retención (min) y λmax (en cursivas).
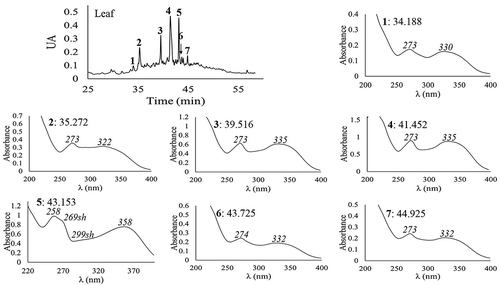
Figure 2. Concentration of the individual phenolic compounds of the leaves (a), bark (b), and fruits on five maturity stages (c) of Malpighia umbellata. Concentrations were estimated by the peak area measurements from the respective HPLC chromatograms and using a standard curve of rutin (slope = 6564.40, axis crossing point = 127.33, r = 0.9965) for flavonols, apigenin (slope = 79,678, axis crossing point = −2564.6, r = 0.9949) for flavones, and chlorogenic acid (slope = 39,870, axis crossing point = 6372.9, r = 0.9985) for phenolic acids.
Figura 2. Concentración de los compuestos fenólicos individuales de las hojas (a), corteza (b) y frutos en cinco estados de madurez (c) de Malpighia umbellata. Las concentraciones se estimaron midiendo las áreas de los picos de los respectivos cromatogramas y usando una curva estándar de rutina (pendiente = 6564.40, ordenada al origen = 127.33, r = 0.9965) para flavonoles, de apigenina (pendiente = 79,678, ordenada al origen = −2564.6, r = 0.9949) para flavonas, y de ácido clorogénico (pendiente = 39,870, ordenada al origen = 6372.9, r = 0.9985) para ácidos fenóliocos.
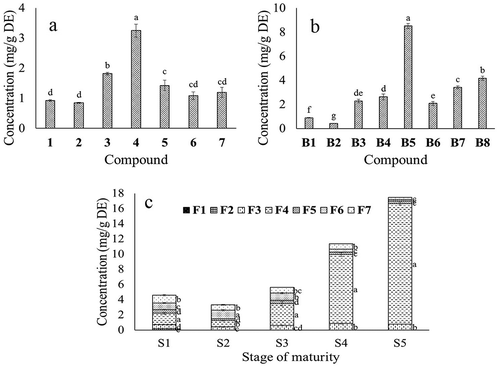
In bark, eight major phenolic compounds were found (). Six were phenolic acids (B1–B6). B7 and B8 were flavonols. The phenolic acid B5 was the most abundant (). The sum of the individual concentrations of all the phenolic acids found in the bark of M. umbellata (16.818 mg/g DE) was higher than those reported for the bark of other tropical species, Uncaria tomentosa L. (cat´s claw) (312.8–2411.2 µg/g extract depending on the geographical origin), for which relevant pharmacological activities have been reported (Hoyos et al., Citation2015). Our results indicate that M. umbellata bark is an important source of phenolic acids; this is relevant because this type of phenolics has highlighting antioxidant activities (Sevgi et al., Citation2015).
Figure 3. HPLC chromatogram and DAD-obtained UV spectra of the bark phenolic compounds from Malpighia umbellata. HPLC chromatogram was registered at 340 nm with an acidified water–acetonitrile gradient method. DAD-obtained UV spectra were obtained between 200 and 400 nm. Retention time (min) and λmax (in italics) are indicated.
Figura 3. Cromatograma y espectros UV obtenidos con HPLC-DAD de los compuestos fenólicos de la corteza de Malpighia umbellata. El cromatograma se registró a 340 nm con un método en gradiente de agua acidificada y acetonitrilo. Los espectros UV se obtuvieron entre 200 y 400 nm. Se indican el tiempo de retención (min) y λmax (en cursivas).
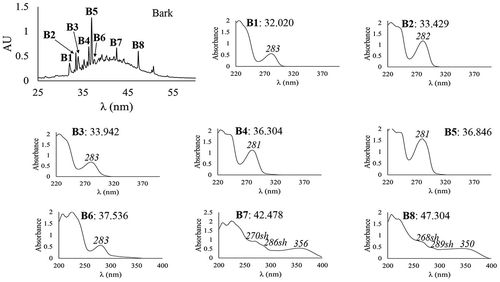
The HPLC chromatograms of the fruit extracts of M. umbellata are displayed in . A total of seven major compounds were found. F1 and F3 were two 3-O-glycosides of quercetin, F2 and F4 were two 3-O-glycosides of kaempferol, and F5 was a flavone (). F1 and F6 were identified as rutin and quercetin, respectively, because their spectral data and retention time () coincided with those of rutin and quercetin analyzed as references. F7 was identified as kaempferol because its spectral data coincided with those of this compound reported by Campos and Markham (Citation2007). Vendramini and Trugo (Citation2004) also found quercetin and kaempferol in fruits of M. punicifolia, in addition to the acids p-coumaric, ferulic, chlorogenic, y caffeic, which were not found here for the fruits of M. umbellata. In contrast, the 3-O-glycosides of quercetin (F1 and F3) and kaempferol (F2 and F4), as well as the flavone (F5) found in the fruits of M. umbellata were not found in the fruits of M. punicifolia (Vendramini & Trugo, Citation2004). In M. emarginata fruits, Mezadri et al. (Citation2008) found chlorogenic acid and rutin, but not flavonol aglycones; whereas, Nascimento et al. (Citation2018) reported rutin, quercetin, and kaempferol (all these found in the current study for M. umbellata fruits) besides catechin, epicatechin, quercitrin, and the acids gallic, caffeic, and ellagic, which were not found here for the fruits of M. umbellata. These variations in the phenolic composition of fruits of different species of acerola could have resulted from some variations in the extraction methods used in each study; however, they also may reflect genetic differences, commanding a defined sequential order in the biosynthesis pathway, which was reported to be specific for each plant species (Heller & Forkmann, Citation1994). This defined sequential order supports the species-specific tendency found for the phenolic profiles of plant tissues (Medina-Medrano et al., Citation2015).
Figure 4. HPLC chromatograms of phenolic compounds of fruits in five maturity stages (S1–S5) of Malpighia umbellata. HPLC chromatograms were registered at 340 nm with an acidified water–acetonitrile gradient method.
Figura 4. Cromatogramas obtenidos por HPLC de los compuestos fenólicos de frutos en cinco estados de madurez (S1–S5) de Malpighia umbellata. Los cromatogramas se registraron a 340 nm con un método en gradiente de agua acidificada y acetonitrilo.
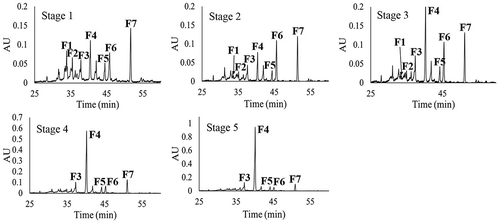
Figure 5. DAD-obtained UV spectra of the phenolic compounds of fruits of Malpighia umbellata. DAD-obtained UV spectra were registered between 200 and 400 nm. Retention time (min) and λmax (in italics) are indicated.
Figura 5. Espectros UV obtenidos por DAD de los compuestos fenólicos de los frutos de Malpighia umbellata. Los espectros UV se registraron entre 200 y 400 nm. Se indica el tiempo de retención (min) y λmax (en cursivas).
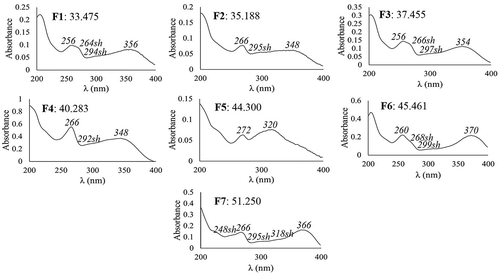
The fruits of M. umbellata displayed different quantitative phenolic compositions according to the stage of ripening (), mainly F4, which represented 90.80% of the flavonoid concentration at S5. F6 and F7 were among the major compounds from S1 to S3, F6 reaching its highest concentration at S2, whereas F7 at S1. The concentration of F4 in the mature fruits was 4.8 and 1.8 fold higher than those of the major foliar compound (4) and the major compound of bark (B5), respectively. This reveals F4 as the most relevant phenolic compound accumulated by M. umbellata. The sum of the individual concentrations of all the flavonols found in the mature fruits of M. umbellata (17.114 mg/g DE) was higher than that reported for the dry fruits of Myrciaria dubia (camu-camu) (3.05 mg/100 g) by Fracassetti, Costa, Moulay, and Tomás-Barberán (Citation2013). Recently, important anti-obesity and anti-diabetic properties were reported for kaempferol glycosides (Zang, Zhang, Igarashi, & Yu, Citation2015); whereas, potent anti-inflammatory activities were informed for kaempferol (Devi et al., Citation2015). According to the current results, the fruits of M. umbellata are a relevant source of kaempferol when immature, of quercetin when reaching the S2, and of a kaempferol glycoside when mature.
Carotenoid contents
Among the most important and most studied terpene derivatives are carotenoids. However, the foliar carotenoid composition of Malpighia species has been little explored. Instead, other terpene derivatives than carotenoids, such as norfriedelins and acerolanins, which are pentacyclic terpenes and tetranoditerpenes, respectively, have been analyzed for M. emarginata. For the first ones, acetylcholinesterase inhibitory effects were reported by Liu et al. (Citation2013); whereas, for the second ones, a moderate cytotoxicity against some human tumor cell lines was informed by Liu et al. (Citation2014). The leaves of M. umbellata accumulated the highest level of carotenoids (). At any stage of maturity, fruits of M. umbellata accumulated considerably higher contents of carotenoids than those reported by De Rosso and Mercadante (Citation2005) for the ripe fruits of M. punicifolia (between 3.70 and 18.81 µg/g). The presence of carotenoids in fruits of M. umbellata at any maturity stage and the fact that they are precursors of abscisic acid (Milborrow, Citation2001), which is a phytohormone involved in the ripening of fleshy fruits (Pilati et al., Citation2017), suggest that carotenoids play relevant roles in the fruit development of this acerola. Carotenoids also are light harvesters in the photosynthesis process and photoprotectors (Mirkovic et al., Citation2017). These roles could account for the high carotenoid content found in the leaves of M. umbellata, which must withstand high incidences of sunlight in its natural habitat. Carotenoids have multiple bioactivities of interest for humans, as they contribute to maintain the normal functions of endothelial cells, helping to reduce the risk of cardiovascular diseases (Yamagata, Citation2017), among others.
Vitamin C contents
The vitamin C contents of M. umbellata fruits showed a tendency to decrease with maturity (). A similar diminution was found for the fruits of M. punicifolia (Vendramini & Trugo, Citation2000) and for the fruits of M. glabra (Nunes et al., Citation2011). For M. punicifolia, Vendramini and Trugo (Citation2000) reported between 1074 and 2164 mg/kg, depending on maturity. For three cultivars of M. emarginata, Hanamura et al. (Citation2008) found between 5 and 25 g/kg. In addition, for M. emarginata, Mezadri et al. (Citation2008) reported between 9.52 and 17.7 g/kg. For the same species (M. emarginata), Rufino et al. (Citation2010) found 1357 mg/100 g. In the current study, the vitamin C contents were assessed for dry fruits of M. umbellata and a lower concentration than those reported for M. punicifolia and M. emarginata was found. Drying of fruits is one of the most common strategies for the long preservation of fleshy fruits; however, the heat sensibility of this vitamin may cause degradation (Araújo et al., Citation2017), which can explain the lower vitamin C contents found in M. umbellata dry fruits. Nevertheless, the contents in S2–S5 were comparable to those of the dry fruits of Myrciaria dubia (camu-camu) (3.51 mg/100 g) (Fracassetti et al., Citation2013), being at S1 even higher.
Antioxidant properties
Comparisons of the antioxidant properties found for M. umbellata with those reported for other species of Malpighia are difficult to make mainly because of the different extraction methods and expression of concentrations used in other studies. As DPPH• scavenger, the leaves of M. umbellata displayed a relevant activity (). Mature fruits showed a not significant higher DPPH• scavenging activity than immature fruits. Leaves, bark, and fruits showed higher DPPH• scavenging activities than that reported for the aerial parts of Hedeoma drummondii (EC50 (DPPH) = 25.12 µg/mL) (Viveros-Valdez et al., Citation2008).
Table 2. DPPH* scavenging activity (EC50 (DPPH))) and ABTS*+ scavenging activity (EC50 (ABTS)) of Malpighia umbellata.
Tabla 2. Capacidad bloqueadora del radical libre DPPH* (EC50 (DPPH)) y de ABTS*+ (EC50 (ABTS)) de Malpighia umbellata.
Bark had the highest ABTS•+ reducing capacity (); however, the ABTS•+ reducing capacities of leaves, bark, and fruits of M. umbellata were higher than that reported by Raghavendra, Reddy, Yadav, Raju, and Kumar (Citation2013) for Amaranthus viridis, an edible plant (EC50 (ABTS) = 500 µg/mL).
Principal components analysis
The differences in contents of total phenolics, flavonoids, tannins, anthocyanins, carotenoids, and vitamin C allowed to distinguish the fruits of M. umbellata by their stage of maturity ()). The principal component (PC) 1 accounted for 73.95% of the variance, was correlated with the contents of vitamin C and total phenolics, and had the highest relative discriminative power (166.48).
Figure 6. Results of two principal components analysis comparing total phenolics, flavonoids, tannins, anthocyanins, carotenoids, and vitamin C for fruits of Malpighia umbellata in five stages of maturity (S1–S5) (a), and comparing the concentration of each individual phenolic compound found in the fruits of M. umbellata in the same five stages of maturity (b). The results were generated from variance–covariance matrixes.
Figura 6. Resultados de dos análisis de componentes principales comparando los contenidos de fenoles totales, flavonoides, taninos, antocianinas, carotenoides, y vitamina C de los frutos de Malpighia umbellata en cinco estados de madurez (S1–S5) (a), y comparando la concentración de cada compuesto fenólico individual encontrado en los frutos de M. umbellata en los mismos cinco estados de madurez (b). Los resultados se generaron a partir de matrices de varianza-covarianza.
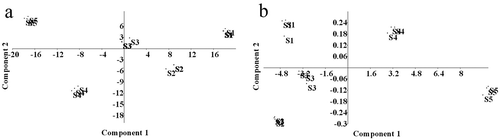
The differences in contents of the individual phenolic compounds also allowed to distinguish the fruits of M. umbellata by their stage of maturity ()). The PC1 had the highest relative discriminative power (35.13), accounted for 99.83% of the variance, and was correlated with the concentration of F4.
Chemical typifications, as this is done here for M. umbellata, are required to develop strategies of rational exploitation and guarantee the quality of plant foods. Chemical fingerprinting of edible plant parts at a different stage of maturity must be a required issue to use all the potential of plants and develop a type of passport/ID with commercial, research, and safety purposes.
Conclusions
The leaves, bark, and fruits of M. umbellata are relevant sources of bioproducts and have important antioxidant properties. 7-O-glycosides of the flavone apigenin were predominant in leaves, phenolic acids in the bark; whereas, 3-O-glycosides of the flavonols kaempferol and quercetin were predominant in fruits. Dry fruits also are an excellent source of vitamin C. A kaempferol-3-O-glycoside accumulated in the red mature fruits was the main phenolic compound of this species of acerola. Chemical differences allow discriminating the fruits, in particular stages of maturity, of Malpighia umbellate. This species of acerola represents an alternative crop for its edible fruits and its diversity and contents of bioproducts. The collected data are a contribution to the knowledge of the diversity and abundance of bioproducts of tropical wild species and can contribute to develop a chemical fingerprinting for the integral use of this species of acerola.
Acknowledgments
AVS acknowledges CONACYT México and IPN for a doctoral fellowship.
Disclosure statement
No potential conflict of interest was reported by the authors.
Additional information
Funding
References
- Araújo, A. C., Oliveira, S. M., Ramos, I. N., Brandão, T. R. S., Monteiro, M. J., & Silva, C. L. M. (2017). Evaluation of drying and storage conditions on nutritional and sensory properties of dried galega kale (Brassica oleracea L. var. Acephala). Journal of Food Quality, 2017, 1–9.
- Bajaj, K. L., & Kaur, G. (1981). Spectrophotometric determination of L-ascorbic acid in vegetables and fruits. Analyst, 106, 117–120.
- Belwal, T., Devkota, H. P., Hassan, H. A., Ahluwalia, S., Ramadan, M. F., Mocan, A., & Atanasov, A. G. (2018). Phytopharmacology of Acerola (Malpighia spp.) and its potential as functional food. Trends in Food Science & Technology, 74, 99–106.
- Campos, M. G., & Markham, K. R. (2007). Structure information from HPLC and on-line measured absorption spectra-flavone, flavonols and phenolic acids. Coimbra: Coimbra University Press.
- De Rosso, V., Hillebrand, V., Montilla, S., Bobbio, E. C., Winterhalter, F. O., & Mercadante, A. Z. (2008). Determination of anthocyanins from acerola (Malpighia emarginata DC.) and acai (Euterpe oleracea Mart.) by HPLC-PDA-MS/MS. Journal of Food Composition and Analysis, 21, 291–299.
- De Rosso, V. V., & Mercadante, A. Z. (2005). Carotenoid composition of two Brazilian genotypes of acerola (Malpighia punicifolia L.) from two harvests. Food Research International, 38, 1073–1077.
- Delva, L., & Goodrich-Schneider, R. (2013). Antioxidant activity and antimicrobial properties of phenolic extracts from acerola (Malpighia emarginata DC) fruit. International Journal of Food Science and Technology, 48, 1048–1056.
- Devi, K. P., Malar, D. S., Nabavi, S. F., Sureda, A., Xiao, J., Nabavi, S. M., & Daglia, M. (2015). Kaempferol and inflammation: From chemistry to medicine. Pharmacological Research, 99, 1–10.
- Fracassetti, D., Costa, C., Moulay, L., & Tomás-Barberán, F. A. (2013). Ellagic acid derivatives, ellagitannins, proanthocyanidins and other phenolics, vitamin C and antioxidant capacity of two powder products from camu-camu fruit (Myrciaria dubia). Food Chemistry, 139, 578–588.
- Galvez, R. L., Kwon, Y. I., Apostolidis, E., & Shetty, K. (2010). Phenolic compounds, antioxidant activity and in vitro inhibitory potential against key enzymes relevant for hyperglycemia and hypertension of commonly used medicinal plants, herbs and spices in Latin America. Bioresource Technology, 101, 4676–4689.
- Giusti, M. M., & Wrolstad, R. E. (2001). Characterization and measurement of anthocyanins by UV-visible spectroscopy. In R. E. Wrolstad (Ed.), Currents protocols in food analytical chemistry (pp. F1: F1.2.1–F1.2.13). New York, NY: Wiley.
- Hanamura, T., Hagiwara, T., & Kawagishi, H. (2005). Structural and functional characterization of polyphenols isolated from acerola (Malpighia emarginata DC.) fruit. Bioscience, Biotechnology, and Biochemistry, 69, 280–286.
- Hanamura, T., Uchida, E., & Aoki, H. (2008). Changes of the composition in acerola (Malpighia emarginata DC.) fruit in relation to cultivar, growing region and maturity. Journal of the Science of Food and Agriculture, 88, 1813–1820.
- Heller, W., & Forkmann, G. (1994). Biosynthesis of flavonoids. In J. B. Harborne (Ed.), The flavonoids. Advances in research since 1986 (pp. 499–535). London: Chapman and Hall.
- Ho, G. T. T., Kase, E. T., Wangensteen, H., & Barsett, H. (2017). Phenolic elderberry extracts, anthocyanins, procyanidins, and metabolites influence glucose and fatty acid uptake in human skeletal muscle cells. Journal of Agricultural and Food Chemistry, 65, 2677–2685.
- Horta, N. R., Khal, V. F. S., Sarmento, M. S., Nunes, M. F. S., Porto, C. R. M., de Andrade, V. M., … Da Silva, J. (2016). Protective effects of acerola juice on genotoxicity induced by iron in vivo. Genetics and Molecular Biology, 39, 122–128.
- Hoyos, M. N., Sánchez-Patán, F., Masis, R. M., Martín-Álvarez, P. J., Ramírez, W. Z., Monagas, M. J., & Bartolomé, B. (2015). Phenolic assessment of Uncaria tomentosa L. (Cat’s Claw): Leaves, stem, bark and wood extracts. Molecules, 20, 22703–22717.
- Julkunen-Tiitto, R. (1985). Phenolic constituents in the leaves of northern willows: Methods for the analysis of certain phenolics. Journal of Agricultural and Food Chemistry, 33, 213–217.
- Kay, C. D., Pereira-Caro, G., Ludwig, I. A., Clifford, M. N., & Crozier, A. (2017). Anthocyanins and flavanones are more bioavailable than previously perceived: A review of recent evidence. Annual Review of Food Science and Technology, 8, 155–180.
- Khatun, A., Rahman, M., Haque, T., Rahman, M. M., Akter, M., Akter, S., & Jhumur, A. (2014). Cytotoxicity potentials of eleven Bangladeshi medicinal plants. The Scientific World Journal, 2014, 1–7.
- Kumari, M., & Jain, S. (2012). Tannins: An antinutrient with positive effect to manage diabetes. Research Journal of Recent Sciences, 1, 10–73.
- Liu, J.-Q., Deng, -Y.-Y., Li, T.-Z., Han, Q., Li, Y., & Qiu, M.-H. (2014). Three new tetranorditerpenes from aerial parts of Acerola cherry (Malpighia emarginata). Molecules, 19, 2629–2636.
- Liu, J.-Q., Peng, X.-R., Li, X.-Y., Li, T.-Z., Zhang, W.-M., Shi, L., … Qiu, M.-H. (2013). Norfriedelins A–C with acetylcholinesterase inhibitory activity from acerola tree (Malpighia emarginata). Organic Letters, 15, 1580–1583.
- Maia, I. R. D. O., Trevisan, M. T. S., Silva, M. G. D. V., Breuer, A., & Owen, R. W. (2017). Content of total phenolic compounds, flavonoids and tannins in methanol extracts of the genus Senna Mill. from the northeast of Brazil and evaluation of antioxidant capacity. Journal of Pharmacognosy and Phytochemistry, 6, 1321–1325.
- Malinowska, P., Gliszczyńska-Świgło, A., & Szymusiak, H. (2014). Protective effect of commercial acerola, willow, and rose extracts against oxidation of cosmetic emulsions containing wheat germ oil. European Journal of Lipid Science and Technology, 116, 1553–1562.
- Marques, T. R., Caetano, A. A., Alves, D. S., Ramos, V. O., Simão, A. A., Carvalho, G. A., & Corrêa, A. D. (2016). Malpighia emarginata DC. bagasse acetone extract on Spodoptera frugiperda (J. E. Smith) (Lepidoptera: Noctuidae). Chilean Journal of Agricultural Research, 76, 55–61.
- Medina-Medrano, J. R., Almaraz-Abarca, N., González-Elizondo, M. S., Uribe-Soto, J. N., González-Valdez, L. S., & Herrera-Arrieta, Y. (2015). Phenolic constituents and antioxidant properties of five wild species of Physalis (Solanaceae). Botanical Studies, 56(24), 1–13.
- Meyer, F. K. (2000). Revision der gattung Malpighia L. (Malpighiaceae). Berlin: Phanerogamarum Monographie XXIII.
- Mezadri, T., Fernández-Pachón, M. S., Villaño, D., García-Parrilla, M. C., & Troncoso, A. M. (2006). The acerola fruit: Composition, productive characteristics and economic importance. Archivos Latinoamericanos de Nutrición, 56(2), 101–109.
- Mezadri, T. D., Villaño, D., Fernández-Pachón, M. S., García-Parrilla, M. C., & Troncoso, A. M. (2008). Antioxidant compounds and antioxidant activity in acerola (Malpighia emarginata DC.) fruits and derivatives. Journal of Food Composition and Analysis, 21, 282–290.
- Milborrow, B. V. (2001). The pathway of biosynthesis of abscisic acid in vascular plants: A review of the present state of knowledge of ABA biosynthesis. Journal of Experimental Botany, 52(359), 1145–1164.
- Mirkovic, T., Ostroumov, E. E., Anna, J. M., Van Grondelle, R., Govindjee, & Scholes, G. D. (2017). Light absorption and energy transfer in the antenna complexes of photosynthetic organisms. Chemical Reviews, 117, 249–293.
- Nascimento, E. M. M., Rodrigues, F. F. G., Costa, W. D., Boligon, A. A., Sousa, E. O., Rodrigues, F. F. G., … da Costa, J. G. M. (2018). HPLC and in vitro evaluation of antioxidant properties of fruit from Malpighia glabra (Malpighiaceae) at different stages of maturation. Food and Chemical Toxicology, accepted manuscript. doi:10.1016/j.fct.2017.11.042
- Naumann, H. D., Hagerman, A. E., Lambert, B. D., Muir, J. P., Tedeschi, L. O., & Kothmann, M. M. (2014). Molecular weight and protein-precipitating ability of condensed tannins from warm-season perennial legumes. Journal of Plant Interactions, 9, 212–219.
- Nunes, R. S., Kahl, V. F. S., Sarmento, M. S., Richter, M. F., Costa-Latufo, L. V., Rodrigues, F. A. R., … da Silva, J. (2011). Antigenotoxicity and antioxidant activity of acerola fruit (Malpighia glabra L.) at two stages of ripeness. Plant Foods for Human Nutrition, 66, 129–135.
- Oyeleye, S. I., Adebayo, A. A., Ogunsuyi, O. B., Dada, F. A., & Oboh, G. (2017). Phenolic profile and enzyme inhibitory activities of almond (Terminalia catappa) leaf and stem bark. International Journal of Food Properties, 20, S2810–S2821.
- Pilati, S., Bagagli, G., Sonego, P., Moretto, M., Brazzale, D., Castorina, G., … Mozer, C. (2017). Abscisic acid is a major regulator of grape berry ripening onset: New insight with ABA signaling network. Frontiers in Plant Science, 8, 1–16.
- Raghavendra, M., Reddy, A. M., Yadav, P. R., Raju, A. S., & Kumar, L. S. (2013). Comparative studies on the in vitro antioxidant properties of methanolic leafy extracts from six edible leafy vegetables of India. Asian Journal of Pharmaceutical and Clinical Research, 6, 96–99.
- Re, R., Pellegrini, N., Proteggente, A., Pannala, A., Yang, M., & Rice-Evans, C. (1999). Antioxidant activity applying an improved ABTS radical cation decolorization assay. Free Radical Biology & Medicine, 26, 1231–1237.
- Rufino, M. S. M., Alves, R. E., De Brito, E. S., Perez, J. J., Suara, C. F., & Mancini, F. J. (2010). Bioactive compounds and antioxidant capacities of 18 non-traditional tropical fruits from Brasil. Food Chemistry, 121, 996–1002.
- Santos-Buelga, C., & Scalbert, A. (2000). Proanthocyanidins and tannin-like compounds-nature, occurrence, dietary intake and effects on nutrition and health. Journal of the Science of Food and Agriculture, 80, 1094–1117.
- Sevgi, K., Tepe, B., & Sarikurkcu, C. (2015). Antioxidant and DNA damage protection potentials of selected phenolic acids. Food and Chemical Toxicology, 77, 12–21.
- Singleton, V. L., & Rossi, J. A. (1995). Colorimetry of total phenolics with phosphomolybdic-phosphotungstic acid reagents. American Journal of Enology and Viticulture, 16, 144–158.
- Smiljkovic, M., Stanisavljevic, D., Stojkovic, D., Petrovic, I., Marjanovic, J. V., Popovic, J., … Sokovic, M. (2017). Apigenin-7-O-glucoside versus apigenin: Insight into the modes of anticandidal and cytotoxic actions. EXCLI Journal, 16, 795–807.
- Thompson, K. A., Marshall, M. R., Sims, C. A., Wei, C. I., Sargent, S. A., & Scott, J. W. (2000). Cultivar, maturity, and heat treatment on lycopene content in tomatoes. Journal of Food Science, 65(5), 791–795.
- Vega-Aviña, R., Aguilar-Hernández, H., Gutiérrez-García, J. A., & Hernández-Vizcarra, J. A. (2000). Endemismo regional presente en la flora del municipio de Culiacán, Sinaloa, México. Acta Botánica Mexicana, 53, 1–15.
- Vendramini, A. L., & Trugo, L. C. (2000). Chemical composition of acerola fruit (Malpighia punicifolia L.) at three stages of maturity. Food Chemistry, 71, 195–198.
- Vendramini, A. L. A., & Trugo, L. C. (2004). Phenolic compounds in acerola fruit (Malpighia punicifolia, L.). Journal of the Brazilian Chemical Society, 15, 664–668.
- Venugopal, T. M., Mallikarjun, N., Somasundar, K., Nagaraj, K., & Prabhuswamy, B. (2016). Antioxidant and antibacterial activity of methanol extract of Malpighia glabra against Viridans group of Streptococci involved in dental caries. Advances in Bioresearch, 7, 173–180.
- Viveros-Valdez, E., Rivas-Morales, C., Carranza-Rosales, P., Mendoza, S., & Schmeda-Hirschmann, G. (2008). Free radical scavengers from the Mexican herbal tea “Poleo” (Hedeoma drummondii). Zeitschrift für Naturforschung, 63, 341–346.
- Yamagata, K. (2017). Carotenoids regulate endothelial functions and reduce the risk of cardiovascular disease. In D. J. Cvetkovic & G. S. Nikolic (Eds.), Carotenoids (pp. 106–121). Leskovac: InTech.
- Zang, Y., Zhang, L., Igarashi, K., & Yu, C. (2015). The anti-obesity and anti-diabetic effects of kaempferol glycosides from unripe soybean leaves in high-fat-diet mice. Food and Function, 6, 834–841.
- Zarin, M. A., Wan, H. Y., Isha, A., & Armania, N. (2016). Antioxidant, antimicrobial and cytotoxic potential of condensed tannins from Leucaena leucocephala hybrid-Rendang. Food Science and Human Wellness, 5, 65–75.