ABSTRACT
This study aims to explore the preventive and therapeutic effects of Lycium barbarum pigment (LP) and polysaccharides (LBPs) on alcohol-induced fatty liver disease (AFLD) in mice, through the measurement of serum and hepatic biochemical indexes, morphologic pathological observation and expression of metabolism-related genes. The results showed that LP and LBPs were non-toxic. The body weight, liver index, and lipid metabolic indexes were significantly inhibited by LP and LBPs. Besides, LP and LBPs could protect liver from oxidative injury and allergy, as suggested by increased glutathione peroxidase (GSH-Px) and superoxide dismutase (SOD), reduced malondialdehyde (MDA) and inflammatory cytokine (TNF-α, IL-1β, IL-6). Furthermore, through RT-PCR, LP, and LBPs were found to regulate liver injury related metabolism by increasing expression of AMPKα2 mRNA and reducing expressions of SREBP-1c, CYP2E1, TLR4, and Myd88 mRNA. Therefore, Lycium barbarum may be developed as a potential functional food regarding preventing and treating AFLD.
RESUMEN
El presente estudio se propuso examinar los efectos preventivos y terapéuticos del pigmento y los polisacáridos de Lycium barbarum (LP y LBP, respectivamente) procedente de ratones con enfermedad de hígado graso inducida por el alcohol (AFLD). Para ello se realizó la medición de los índices hepáticos bioquímicos y de suero, la observación morfológica y patológica, y se examinó la expresión de los genes relacionados con el metabolismo. Los resultados dan cuenta de que el LP y los LBP no son tóxicos. Éstos inhibieron significativamente el peso corporal, el índice hepático y los índices de metabolismo de lípidos. Asimismo, protegieron al hígado del daño oxidativo y de alergias, como lo sugieren el aumento de glutatión peroxidasa (GSH-Px) y superóxido dismutasa (SOD), y la reducción de malonidialdehído (MDA) y citosinas inflamatorias (TNF-α, IL-1β, IL-6). Además, mediante pruebas de RT-PCR se constató que el LP y los LBP regulan el metabolismo vinculado con el daño hepático elevando la expresión de AMPKα2 mRNA y reduciendo la expresión de SREBP-1c, CYP2E1, TLR4 y Myd88 mRNA. Por lo tanto, se puede concluir que el Lycium barbarum puede ser desarrollado como posible alimento funcional destinado a prevenir y tratar la AFLD.
1. Introduction
It has been reported that increase in alcohol consumption augments the incidence of alcohol-induced liver injury that has gradually becoming the second largest liver disease in recent years (Chacko & Reinus, Citation2016). Long-term excessive drinking alcohol may cause a series of liver diseases (Masarone et al., Citation2016), of which alcohol-induced fatty liver disease (AFLD) is the initial stage. AFLD may cause more severe liver injuries, such as steatohepatitis, hepatic fibrosis, cirrhosis, and even hepatocellular carcinoma (Williams, Manley, & Ding, Citation2014). As AFLD is recognized as the initial stage of advanced severe liver diseases, the prevention and treatment of AFLD have increasingly obtained concern around the world. However, intake of synthetic medicine for AFLD treatment may increase kidney burden and may be not suitable for individuals widely. Therefore, it is necessary to find and develop natural anti-AFLD ingredients.
In recent years, plant extracts that have been reported to prevent or treat AFLD include polysaccharides (Zhang, Zhao, Wang, Zhao, & Zhao, Citation2017), polyphenols (Kim et al., Citation2017), polysaccharide peptides (Ren et al., Citation2018), etc. The indexes that have been used to characterize the preventive and therapeutic effects of synthetic or natural constituents on AFLD include body weight, liver index, total cholesterol (TC), triacylglycerol (TG), total bilirubin (TBil), alanine aminotransferase (ALT), aspartate aminotransferase (AST), malondialdehyde (MDA), glutathione (GSH), superoxide dismutase (SOD), inflammatory cytokine such as tumor necrosis factor-α (TNF-α) and interleukin (IL-1β and IL-6), etc. (Ren et al., Citation2018; Song et al., Citation2018; Zhang et al., Citation2017). In addition, the expression of genes related with lipid synthesis and metabolism such as AMP-activated protein kinase (AMPK), acetyl-CoA carboxylase (ACC), and peroxisome proliferator–activated receptor α (PPARα) (Ren et al., Citation2018), the expression of genes regarding inflammation responding to liver injury like toll-like receptor (TLR) (Li et al., Citation2018), as well as the expression of genes related with ethanol metabolism like cytochrome P450 2E1 (CYP2E1) (Yuan et al., Citation2018) have also been investigated through real-time PCR and western blot in previous studies.
Lycium barbarum is a kind of wild resource plant originally living in the northwest of China (Yao, Peng, Zhou, Xiao, & Sun, Citation2010), and it has been used as a medicine and food for at least 2000 years (Ulbricht et al., Citation2015). Recently, the production Lycium barbarum has increased, because it can be eaten as a fruit or a raw material for beverage that is described as an ‘exotic superfood’ by nutritionists (Jin et al., Citation2015). Lycium barbarum pigment (LP) is a kind of active component obtained from the berry and widely used as natural food colorants (Hu, Zheng, Li, & Suo, Citation2014). The main component in LP is carotenoids that have been reported to improve the functions of eye, liver, and kidney (Wang, Chang, Inbaraj, & Chen, Citation2010). Zeaxanthin and its palmitic derivative account for the most constituent of carotenoids (Amagase & Farnsworth, Citation2011), and they have been considered as antioxidants in many diseases caused by oxidative stress (Sen et al., Citation2014). Lycium barbarum polysaccharides (LPBs) is considered as one another active component in the fruit (Yang, Yang, Guo, Jiao, & Zhao, Citation2013). LPBs has been reported to consist of rhamnose, arabinose, xylose, mannose, glucose, glucuronic acid, galactose, and galacturonic acid in the glycan part (Masci et al., Citation2018). Modern pharmacological studies indicate that LPBs have been suggested some bioactivities, including antioxidant, hypoglycemic, anti-allergic properties, etc. (Garcia-Gonzalez, Alnaief, & Smirnova, Citation2011).
Although the hepatoprotective effects of LPBs on alcoholic liver disease in mice in terms of liver oxidative stress have been studied (Cheng & Kong, Citation2011; Wu et al., Citation2011), the detail preventive and therapeutic effects of LPBs on AFLD in mice need to be further shed light on. Additionally, pigment is another active constituent in Lycium barbarum, and the information about hepatoprotective effect of LP on AFLD is limited. Therefore, to synthetically evaluate the hepatoprotective effect of Lycium barbarum as a functional food, the preventive and therapeutic effects of LPBs and LP on AFLD in mice were investigated in this study through the determination of serum and hepatic biochemical indexes, morphologic pathological observation, and expression of metabolism-related genes. Notably, in the preventive trials (), normal mice were administrated with alcohol and the two compounds alternatively with 4 h intervals to avoid the direct interaction between alcohol and active compounds, while in the therapeutic trials (), AFLD model mice were developed with oral administration of alcohol in advance.
2. Materials and methods
2.1 Materials
Lycium barbarum pigment (LP) and polysaccharides (LBPs) were provided by Hebei Qianpin biotechnology Co. LTD. (Hebei, China). LBPs was extracted by hot water, the extraction yield of LBPs is about 48%. ELISA kits for the analyses of total bilirubin (TBil), alanine aminotransferase (ALT), aspartate aminotransferase (AST), triglyceride (TG), superoxide dismutase (SOD), malondialdehyde (MDA), glutathione peroxidase (GSH-Px), were obtained from R&D Systems Co. (Minneapolis, USA). All the other reagents used were of analytical grade.
2.2 Animals and treatments
2.2.1 Acute toxicity study
C57 mice (20–32 g body mass, n = 72) were obtained from The Fourth Army Medical University (Xi’an, China). All the experimental animals were housed individually in stainless steel cages under standard laboratory conditions at 25°C with 12 h of light and dark cycles, 40–70% humidity and free access to diet and water. All experiments were performed in accordance with the Regulations of Experimental Animal Administration issued by the State Committee of Science and Technology of the People’s Republic of China,and were approved by the institutional ethical guidelines. We tried our best to reduce the number of animals used and to minimize animals’ suffering.
After one week of acclimatization, mice were grouped based on the body weight (n = 8). Each group of mice was fasted for 16 h with free access to sterile water prior to treatment. For acute toxicity study, according to GB15193.3–2003, the mice were provided with LP and LBPs, respectively, at the dosage of 0 (physiological saline), 1.25, 2.50, 5.00, and 10.00 g/kg by gavage. In this study, LP was dissolved in 10% DMSO corn oil, and LBPs was prepared in physiological saline. Both the compounds were administrated four times in 24 h. Then, the treated mice were observed 14 days continuously, and the acute toxicity reaction including behavior activity, appearance, weight, and survival situation were recorded. On the 15th day, all the mice were dislocated and dissected. The main organs, including heart, liver, spleen, lung, and kidney were collected and prepared for paraffin sections, and then stained by hematoxylin and eosin (H&E). The pathological examination was conducted by use of a light microscope.
2.2.2 Preventive and therapeutic trials
The study for hepatoprotective effects of LP and LBPs on AFLD mice was divided into preventive and therapeutic trials. Specifically, for the preventive trial as shown in , the mice were divided into five groups. Group 1 (n = 8) was provided with physiological saline (PS) as the control, and group 2–5 (each group, n = 8) were provided with equal volume of 10 mL/kg alcohol (55%, v/v) by gavage. All the mice were allowed ad libitum access to diet and water. After 4 h, groups 1 and 2 were provided with physiological saline (PS), and group 3–5 were given 120 mg/kg bifendate, 1 g/kg LP and 1 g/kg LBPs, respectively. The body weight (BW) was measured every one week from the beginning. After 4 week treatment, the mice were anesthetized with sodium pentobarbital after overnight fasting. Then, the serum and hepatic samples were collected rapidly for further biochemical assays.
For the therapeutic trial as shown in , the AFLD model mice were developed as follows: the normal mice were fed with 10 mL/kg of alcohol (55%, v/v) by gavage for 4 weeks continuously. All the mice were allowed ad libitum access to diet and water during these 4 weeks. And then, a mouse was selected randomly, the blood biological indicators related to alcohol liver were detected comparing with the normal mice so as to confirm the success of modeling. Then, the mice were divided into five groups. The normal mice (n = 8) were applied for group 1 as the control. The AFLD model mice were applied for group 2–5 (each group, n = 8). All the mice were allowed ad libitum access to diet and water. Then, groups 1 and 2 were provided with physiological saline (PS), and group 3–5 were given 120 mg/kg bifendate, 1 g/kg LP and 1 g/kg LBPs, respectively. Four weeks later, the mice were anesthetized with sodium pentobarbital after overnight fasting. Then, the serum and hepatic samples were collected rapidly for further biochemical assays.
2.3 Determination of serum biochemical parameters
The serum levels of TG, TBil, ALT, and AST were determined by the respective ELISA kits according to the manufacturer’s instructions using a spectrophotometer.
2.4 Liver oxidative stress and inflammation analysis
10% (w/v) liver tissue homogenate was obtained from mice liver by use of a glass homogenizer for 6–8 min. Subsequently, the homogenate was centrifuged at 5000 × g for 15 min to obtain the supernatant that was then stored in a cryogenic refrigerator at −20°C. The levels of liver MDA, GSH-Px, SOD, TNF-α, IL-1β, and IL-6 were determined by the respective ELISA kits according to the manufacturer’s instructions using a spectrophotometer.
2.5 Histopathological observation
Hematoxylin and eosin (H&E) staining was performed on liver sections for histopathological examination. Specifically, the whole liver was fixed with 10% (v/v) formaldehyde solution for 48 h and then prepared for liver sections. The sections were stained with hematoxylin and eosin (H&E), followed by observation under a light microscope at 200× magnification to evaluate the histopathological changes.
2.6 mRNA level analyses
The total mRNA in mice liver was isolated with the Invitrogen® Trizol reagent according to the manufacturer’s instructions of mRNA isolation kit. cDNA was synthesized by reverse transcription, and then real-time PCR was performed using a Qiagen® PCR kit to determine six genes related with lipid and alcohol metabolism. All the quantitative PCR were performed in triplicate. PCR primers for CYP2E1, AMPKɑ2, PPARɑ, SREBP-1c, TLR4, and Myd88 are shown in .
Table 1. The primers used for RT-PCR.
Tabla 1. Iniciadores (primers) utilizados para el RT-PCR.
2.7 Statistical analysis
Data were expressed as the mean± standard error of replicate experiments and Origin® 8.0 software was used to perform the statistical analysis. The differences between the groups were defined as significant at P < 0.05.
3. Results
3.1 Acute toxicity study
It was found that there were no abnormalities observed for the hair color, respiration state and dietary status of the mice in two weeks, and the body weight of the mice was not significantly (P > 0.05) changed after two weeks treatment with LP and LBPs, respectively (, ). No death was observed for the treated mice. Therefore, the semi-lethal dosage (LD50) of LP and LBPs were both higher than 10.0 g/kg under the experiment conditions, which is a way to measure the short-term poisoning potential (acute toxicity) of a material, and defined as a statistically derived expression of a single dose of a substance that can be expected to kill 50% of animals.
Figure 2. The acute toxicity of LP and LBPs. The effects of LP (a) and LBPs (b) on body weight of normal mice; the effects of LP (c) and LBPs (d) on histopathological profiles of viscera of normal mice.
Figura 2. Toxicidad aguda del LP y los LBP. Efectos del LP (a) y los LBP (b) en el peso corporal de ratones normales; efectos del LP (c) y los LBP (d) en los perfiles histopatológicos de las vísceras de ratones normales.
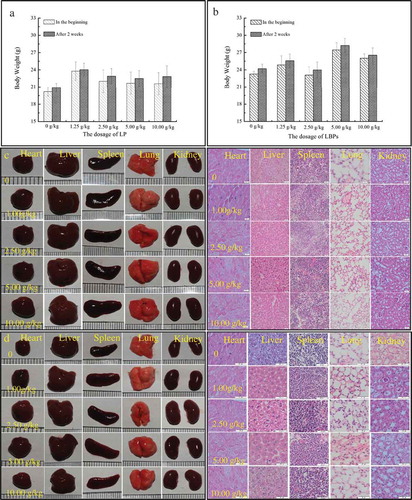
Also, the color, brightness, shape of heart, liver, spleen, lung, and kidney were found to have no obvious alternations (, ) after the mice were executed after two weeks. Then, the histopathologic profiles of heart, liver, spleen, lung, and kidney were observed by hematoxylin and eosin stains and shown in and . It was found that the internal tissue structures of the viscera arranged orderly, and the cell size and morphology were normal with the cell nuclei clearly observed (, ). Therefore, according to GB15193.3–2003 and the Organization for Economic Co-operation and Development (OECD, Citation2001), LP and LBPs were non-toxicity.
3.2 Effects of LP and LBPs on body weight and liver index
The effects of LP and LBPs on the body weight of alcohol-treated mice in the preventive and therapeutic trials are shown in and . As shown, the body weight of the alcohol-treated mice was significantly (P < 0.05) lower than the control group () after four weeks. However, LP was found to significantly (P < 0.05) reduce the body weight loss in the preventive trial. Additionally, in the therapeutic trial, the growth of body weight was significantly (P < 0.05) reduced for the AFLD model mice group, while LP, LBPs and the positive control (bifendate) were all found to resume the growth to some extent ().
Figure 3. The effects of LP and LBPs on the body weight and liver index during the growth process in the preventive trial (a and b) and therapeutic trial (c and d). The meaning of each group is shown in and . In the respective trial, a P < 0.05 when compared to group 1, b P < 0.05 when compared to group 2, c P < 0.05 when compared to group 3, d P < 0.05 when compared to group 4.
Figura 3. Efectos del LP y los LBP en el peso corporal y el índice hepático durante el proceso de crecimiento en el ensayo preventivo (a y b) y el ensayo terapéutico (c y d). El significado de cada grupo se muestra en las y 1b. En cada ensayo respectivo, a P < 0.05 comparado con el grupo 1, b P < 0.05 comparado con el grupo 2, c P < 0.05 comparado con el grupo 3, d P < 0.05 comparado con el grupo 4.
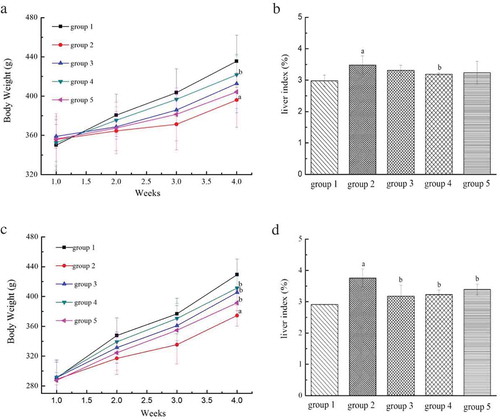
The preventive and therapeutic effects of LP and LBPs on the liver index of alcohol-treated mice are suggested in and . It was found that alcohol treatment could significantly (P < 0.05) increase the liver index, while LP could inhibit the increasing tendency in the preventive trial (). Besides, in the therapeutic trial, the liver index of the AFLD model mice was significantly (P < 0.05) higher than that of the control mice, while LP, LBPs and bifendate were all found to control the index increase for the AFLD model mice ().
3.3 Effects of LP and LBPs on lipid metabolism and liver injury indexes
To evaluate the preventive and therapeutic effects of LP and LBPs on AFLD in mice regarding lipid metabolism, the levels of serum and liver triglyceride (TG) were studied and shown in and . It was found that the contents of serum and liver TG in the alcohol-treated mice were significantly higher than that in the normal mice in the preventive trial, indicating fat accumulation in the alcohol-treated mice. However, both LP and LBPs treatments could significantly (P < 0.05) reduce the values in the treated mice (, ). The results in the therapeutic trial were shown a similar tendency.
Figure 4. The effects of LP and LBPs on the level of TG in serum (a) and liver (b), TBil (c), ALT (d) and AST (e) in serum in the preventive and therapeutic trials. The meaning of each group is shown in and . In the respective trial, aP < 0.05 when compared to group 1, bP < 0.05 when compared to group 2, cP < 0.05 when compared to group 3, dP < 0.05 when compared to group 4.
Figura 4. Efectos del LP y los LBP en el nivel de TG en el suero (a) e hígado (b), TBil (c), ALT (d) y AST (e) en el suero durante las pruebas preventivas y terapéuticas. El significado de cada grupo se muestra en las y 1b. En cada ensayo respectivo, a P < 0.05 comparado con el grupo 1, b P < 0.05 comparado con el grupo 2, c P < 0.05 comparado con el grupo 3, d P < 0.05 comparado con el grupo 4.
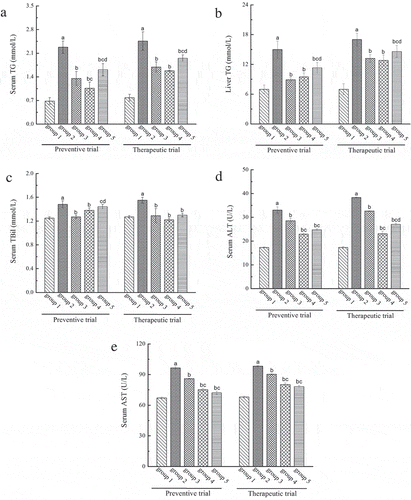
The effects of LP and LBPs on the liver function of alcohol-treated mice were studied in terms of functional indexes, including serum total bilirubin (TBil), alanine aminotransferase (ALT) and aspartate aminotransferase (AST). As shown in , alcohol resulted in significant increase in serum TBil content, indicating that the mice liver injury occurred. LP was found to decrease the TBil content that had been elevated by alcohol. Besides, in the therapeutic trial, the TBil contents of LP and LBPs treated groups were significantly lower than that of the AFLD model mice, suggesting the good therapeutic effect of both compounds on AFLD. Furthermore, as shown in and , both the activities of serum ALT and AST were significantly increased in the alcohol-treated mice, indicating the liver injury and thus the liver function loss in the mice. However, both LP and LBPs were shown to relieve the liver injury as suggested by the lower values of the enzyme activities in serum. It should be noted that the preventive effect of LP and LBPs on liver injury caused by alcohol was significantly (P < 0.05) better than the commercial medicine, bifendate. Similarly, LP and LBPs were also shown better therapeutic effect on liver injury for the AFLD model mice than bifendate.
3.4 Effects of LP and LBPs on liver oxidative stress and inflammation
Chronic alcohol administration has been reported to induce liver oxidative stress that can be evaluated by the contents of malondialdehyde (MDA), glutathione peroxidase (GSH-Px) and superoxide dismutase (SOD) in liver tissues (Masarone et al., Citation2016; Zeng et al., Citation2013), and inflammation that can be detected by the contents of inflammatory cytokine such as tumor necrosis factor- α (TNF- α) and interleukin (IL- 1β and IL- 6) (Li et al., Citation2015; Shankar, Klein, & Klein, Citation2006). The effects of LP and LBPs on the oxidative stress and inflammation in the mice treated with alcohol were studied for both the preventive and therapeutic trials, and the results are shown in . As shown, a significant increase in liver MDA content responding to oxidative stress was found for the alcohol-treated mice, but LP and LBPs were found to inhibit the increasing trend to a large extent (). Similar effects were observed in the therapeutic trial. Besides, alcohol treatment was displayed to reduce the contents of liver GSH-Px and SOD significantly (P < 0.05) (, ). However, LP and LBPs could increase both the contents that had been reduced, indicating that the two compounds relieved the liver oxidative stress caused by alcohol. Similar observation obtained in the therapeutic trial suggests good treating effects of LP and LBPs on oxidative stress injury in AFLD model mice. In addition, Chronic alcohol administration obviously increased (P < 0.05) the contents of inflammatory cytokine TNF-α, IL-1β and IL-6, and significantly decreased the increase by treatment with LP and LBPs both in preventive and therapeutic experiments, as shown in – , showing that LP and LBPs could attenuate the inflammation of liver caused by alcohol.
Figure 5. The effects of LP and LBPs on the level of MDA (a), GSH-Px (b), SOD (c), TNF- α (d), IL- 1β (e) and IL- 6 (f) in liver in the preventive and therapeutic trials. The meaning of each group is shown in and . In the respective trial, a P < 0.05 when compared to group 1, b P < 0.05 when compared to group 2, c P < 0.05 when compared to group 3, d P < 0.05 when compared to group 4.
Figura 5. Efectos del LP y los LBP en el nivel de MDA (a), GSH-Px (b), SOD (c), TNF- α (d), IL- 1β (e) Y IL- 6 (f) en el hígado durante los ensayos preventivos y terapéuticos. El significado de cada grupo se muestra en las y 1b. En cada ensayo respectivo, a P < 0.05 comparado con el grupo 1, b P < 0.05 comparado con el grupo 2, c P < 0.05 comparado con el grupo 3, d P < 0.05 comparado con el grupo 4.
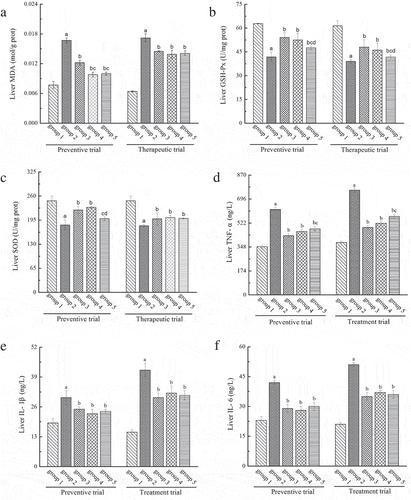
3.5 Effects of LP and LBPs on liver morphology and histopathology
To directly describe the protective effects of LP and LBPs on liver injury caused by alcohol, the morphological and histopathological images of mice liver tissues were observed by hematoxylin and eosin (H&E) stains. As shown in , the fat droplets were clearly observed on the liver surface of the alcohol-treated mice, while the surface fat droplets were not obvious for the bifendate-alcohol, LP-alcohol, and LBPs-alcohol groups. Consistent with the surface observation, the histopathologic images also suggested that the liver tissue cells treated with alcohol-LP and alcohol-LBPs arranged more orderly with less fat droplets than the tissue cells treated with alcohol-PS. In addition, the hepatic repairing effects of LP and LBPs on the AFLD model mice were also observed in the therapeutic trial ().
Figure 6. The effects of LP and LBPs on the histopathological profiles of mice livers in the preventive (a) and therapeutic (b) trials. The meaning of each group in both the trials was the same as that in .
Figura 6. Efectos del LP y los LBP en los perfiles histopatológicos de los hígados de ratones durante los ensayos preventivo (a) y terapéutico (b). El significado de cada grupo en ambos ensayos es el mismo que el mostrado en la .
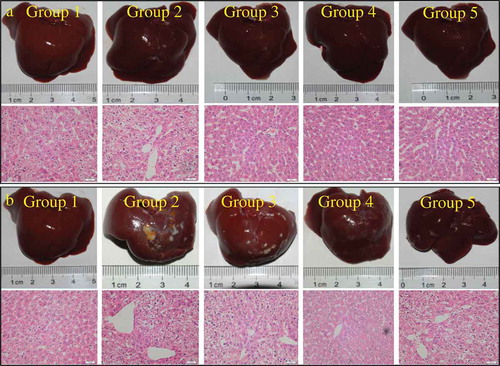
3.6 Effects of LP and LBPs on expression of metabolism-related genes
The effects of LP and LBPs on the transcription of metabolism-related mRNA in the alcohol-treated and AFLD mice are shown in . As shown, the expression of CYP2E1, related with ethanol metabolism and liver oxidative stress was significantly increased in the alcohol-treated mice, while both LP and LBPs were found to decrease the mRNA expression (). Besides, the expression of SREBP-1c, related with fatty acid transformation and triglyceride formation, was significantly higher in the alcohol-treated mice than that in the control ones. However, LP and LBPs could inhibit the increasing tendency caused by alcohol treatment (). Another two mRNA transcription responding to lipid (fatty acid) β-oxidation, including AMPKα2 and PPARα were also investigated. As shown in , both LP and LBPs were found to recover the expression of AMPKα2 that was inhibited by alcohol. However, the two active compounds had no effects on the inhibited expression of PPARα. In addition, the expression of TLR4 mRNA and its ligand (Myd88 mRNA) responding to intracellular inflammation were studied. As shown in , both the gene expressions in liver were activated by alcohol, suggesting the liver inflammation caused by alcohol. Because of the preventing and repairing effects of LP and LBPs on liver injury as shown in , the expression of the two mRNA was decreased by the two compounds. Similar effects of LP and LBPs on the expression of the above metabolism-related genes were observed in the therapeutic trial as well.
Figure 7. The effects of LP and LBPs on the mRNA levels of CYP2E1, AMPLα2, PPARα, SREBP-1c, TLR4, and Myd88 in the preventive and therapeutic trials. The meaning of each group in both the trials was the same as that in . In the respective trial, a P < 0.05 when compared to group 1, b P < 0.05 when compared to group 2, c P < 0.05 when compared to group 3, d P < 0.05 when compared to group 4.
Figura 7. Efectos del LP y los LBP en los niveles de mRNA de CYP2E1, AMPLα2, PPARα, SREBP-1c, TLR4 y Myd88 durante los ensayos preventivo y terapéutico. El significado de cada grupo se muestra en las y 1b. En cada ensayo respectivo, a P < 0.05 comparado con el grupo 1, b P < 0.05 comparado con el grupo 2, c P < 0.05 comparado con el grupo 3, d P < 0.05 comparado con el grupo 4.
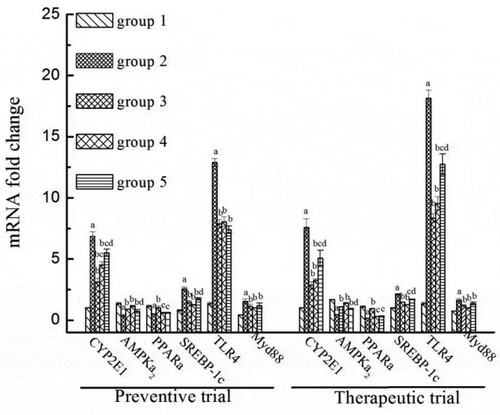
4. Discussion
Alcoholic fatty liver disease (AFLD) has been increasingly attracting concern. Though several drugs including bifendate, tiopronin, and bicyclol have been reported to have protective effect against AFLD (Hirayama, Kishimoto, Wakushima, & Murawaki, Citation1983; Zhao, Chen, & Li, Citation2008), the definite treatment strategies remain undefined (Hirayama et al., Citation1983; Mato & Lu, Citation2007; Zhao et al., Citation2008). Developing a range of safe and efficient agents possessing hepatoprotective effects from natural products has been increasingly concerned (Chandan et al., Citation2008; Muriel & Rivera-Espinoza, Citation2008). Pigment and polysaccharides are two kinds of bioactive compounds in Lycium barbarum that has been regarded as a functional food (Potterat, Citation2010), and bifendate is used for the treatment of hepatitis as well as a positive control for researching other hepatic protectors because of minimal observable side effects at the prescribed dosage (Cui, Wang, & Fan, Citation2002; Guan et al., Citation2005; Gui et al., Citation2005; Pan, Yang, Dong, Yu, & Ko, Citation2006). Therefore, the bifendate was used in the study as a positive control to evaluate the preventive and therapeutic effects of LP and LBPs on AFLD through intragastric administration of alcohol-treated mice and AFLD model mice.
The acute toxicity study suggested the safety of the two compounds (LD50 > 10 g/kg body weight). Normal intake of LP and LBPs would not induce acute toxicity, visceral injury and significant body weight change (). Alcohol consumption was found to negatively affect the growth of body weight of mice in this study (, ), which is consistent with one previous study (Ren et al., Citation2018), and this may result from the disordered lipid metabolism and oxidative stress in alcohol injured liver (Chen et al., Citation2015). It was found that LP and LBPs were effective in both the alcohol-treated and AFLD model mice regarding maintaining body weight growth.
The fat accumulation was obviously observed in the liver tissues of both the alcohol-treated and AFLD model mice (), which is in accordance with the significant increased TG content in serum and liver caused by alcohol (, ), as TG is one lipid component of fat substances. Because LP and LBPs could decrease the levels of TG in serum and liver (, ), they were supposed to reduce the fat accumulation in liver tissues caused by alcohol treatment.
Bilirubin is excreted in bile and urine, and its elevated level in serum indicates certain liver diseases (Hamoud, Weaver, Stec, & Hinds, Citation2018). AST and ALT are two important enzymes exist in liver tissues. When liver is injured, the permeability of liver cellular membrane is increased. Thus, the two enzymes are released from cytoplasm to blood, leading to the increase in the enzymic activities in serum. Therefore, serum ASL and ALT levels are commonly measured clinically as biomarkers for liver health. In our study, alcohol induced the significant increase in the levels of TBil, AST, and ALT, indicating the liver injury in mice in a certain degree. Generally, LP and LBPs were shown to decrease the levels of the three indexes that were elevated by alcohol treatment in both the preventive and therapeutic trials. This suggests the preventive and therapeutic effects of LP and LPBs on the alcohol-induced liver injury in mice.
Liver MDA results from lipid peroxidation of fatty acids, and the increase in MDA level is usually regarded as a marker for oxidative stress of liver injury (Weismann & Binder, Citation2012). Therefore, both LP and LBPs were suggested to decrease the liver oxidative stress caused by alcohol in the preventive and therapeutic trials. In addition, GSH-Px and SOD are two important enzymes, which play the main biological role in protecting organism from oxidative damage (Sultan et al., Citation2018). After treatment with alcohol, the activities of liver GSH-Px and SOD in mice were both significantly reduced. As LP and LBPs were able to recover the reduced activities of both enzymes to some extent (, ), the partially recovered GSH-Px and SOD may develop the ability of capturing and eliminating free oxidative radicals, reducing liver oxidative stress. Furthermore, liver diseases can be stimulated by alcohol-induced inflammation, hence inflammatory cytokines (TNF-α, IL-1β, and IL-6) were determined in the study, which can act as an index of neutrophil infiltration that could enlarge the reaction of hydrogen peroxide through hypochlorous acid and free radicals generations (Leung & Nieto, Citation2013; Shankar et al., Citation2006). TNF-α could accelerate the transfer of neutrophils, and the increased reactive oxygen species (ROS) can be generated as the increased proteolytic enzymes from neutrophils, which can further lead to liver injury (Mouzaoui, Rahim, & Djerdjouri, Citation2012). IL-1β and IL-6 are mainly used to detect accumulative liver damage, and the elevation of these two indexes means severe liver disease even malignancies (Gao et al., Citation2017). After the LP and LBPs treatment, the increased TNF-α, IL-1β and IL-6 levels in liver induced by alcohol were decreased, suggesting that the LP and LBPs from Lycium barbarum could protect the liver from alcohol injury.
CYP2E1 is involved in the breakdown of a large number of toxic chemicals and carcinogens entering the body, as well as the basic metabolic reactions such as fatty acids (Zeng et al., Citation2018). On the other hand, CYP2E1 can be induced by ethanol and thus takes part in ethanol metabolism, generating more reactive oxygen species (ROS), such as superoxide anion radical and hydrogen peroxide (Lu & Cederbaum, Citation2008). By this way, the ethanol metabolism through CYP2E1 pathway may cause liver oxidative stress, which can be suggested by the increased MDA. This is supported by the findings in our study that the CYP2E1 mRNA level was increased by alcohol induction () with the liver MDA content being elevated (). As LP and LBPs were able to reduce the transcription of CYP2E1 mRNA in the alcohol treated and AFLD model mice (), the expression of CYP2E1 was supposed to be inhibited. Therefore, the level of ethanol metabolism through the CYP2E1 pathway was reduced, leading to the decrease in the level of liver oxidative stress (Ikarashi et al., Citation2011). This is consistent with the decreased liver MDA content by LP and LBPs in the alcohol treated and AFLD model mice ().
SREBP-1c is a key lipogenic transcription factor and dedicate to fatty acid uptake and triglyceride synthesis (Ikarashi et al., Citation2011). AMPKα2 is a key regulator of intracellular fatty acid metabolism. Stresses, like exercise, hypoxemia or prolonged starvation could activate AMPK via increasing intracellular AMP/ATP ratio, finally facilitating fatty acids entry into mitochondria for β-oxidation (Rutter, Da Silva Xavier, & Leclerc, Citation2003). Additionally, PPARα plays a key role in the regulation of fatty acid β-oxidation, ketogenesis, and triglyceride turnover (Cave et al., Citation2016). Therefore, according to the above previous studies, SREBP-1c is crucial in fat accumulation, while both AMPKα2 and PPARα are important in fat utilization. Based on these mRNA levels observed in our study, the fat accumulation () and increased TG level in liver tissues of alcohol treated and AFLD model mice () may be due to both the lipid synthesis increase caused by activated SREBP-1c level (suggested by SREBP-1c mRNA) and lipid utilization decrease resulted from the inactivated AMPKα2 and PPARα levels (suggested by AMPKα2 and PPARα mRNA) (). As LP and LBPs were able to positively-regulate the expression of SREBP-1c and AMPKα2 mRNA (), the fat droplet formation and TG level in the livers of alcohol-treated and AFLD model mice were decreased by the two compounds (, ). However, the positive effects did not involve the PPARα pathway ().
TLR4 with the independent Myd88 is a transmembrane protein, belonging to pattern recognition receptor, and inflammatory cytokine production which is responsible for activating the innate immune system that can relieve organ injury (Li et al., Citation2018). The significant increase in the TLR4 and Myd88 mRNA levels in the livers of the alcohol-treated and AFLD model mice () indicate the potential high expression of TLR4 and Myd88, which further indicates the liver injury. Therefore, the reduced TLR4 and Myd88 mRNA levels by LP and LBPs in the treated mice indicate that the two compounds may prevent and treat the liver injury in mice, which can be suggested by the decreased inflammatory cytokine ( – ). Meanwhile, this result is consistent with the decreased liver injury indexes, including TBil, AST, and ALT by LP and LBPs, as suggested in .
LBPs has been reported to be mainly composed of rhamnose, arabinose, xylose, mannose, galactose, glucuronic acid, and galacturonic acid (Table S1) (Masci et al., Citation2018; Zou, Zhang, Yao, Niu, & Gao, Citation2010). ROS can cause damage to a wide range of biomolecules, and LBPs was supposed to prevent ROS injury due to its antioxidant activity in vitro (Gong et al., Citation2018). Our results in this study demonstrate the antioxidant activity of LBPs in vivo by decreasing the liver MDA and CYP2E1 mRNA levels, both of which are intracellular oxidation stress indexes. Recently, some polysaccharides rich in uronic acids have been shown high biological effects including hepatoprotective effect, because uronic acid residues could modify the polysaccharide solubility, enhancing the contacting chance of polysaccharide with ROS (Yang et al., Citation2013). Therefore, the potential hepatoprotective effect of LBPs in the preventive and therapeutic trials in this study may also be attributed to the uronic acids, including glucuronic acid and galacturonic acid in the polysaccharides (Table S1) (Masci et al., Citation2018; Zou et al., Citation2010). However, the detail hepatoprotective effect and its mechanism of uronic acids in LBPs need to be further studied. In addition, LP has been reported to mainly contain carotenoids, in which zeaxanthin dipalmitate accounts for the largest amount (Table S1) (Amagase & Farnsworth, Citation2011). Therefore, the preventive and therapeutic effects of LP on ALFD in mice may be attributed to zeaxanthin dipalmitate that has been reported to therapeutically improve hepatic functions in AFLD model mice (Xiao et al., Citation2014). It should be noted that LP and LBPs were provided 4 h after the mice was treated with alcohol in the preventive trial (), by which most alcohol was metabolized within 4 h according to drug metabolism and pharmacokinetics (Naritomi, Sanoh, & Ohta, Citation2018). By doing this, we intended to avoid the direct interaction between the two compounds and alcohol. Therefore, the hepatoprotective effects of LP and LPBs imply that after drinking alcohol, intake of Lycium barbarum may be recommended as an effective way in preventing AFLD for human beings.
5. Conclusions
The preventive and therapeutic effects of pigment and polysaccharides in Lycium barbarum on AFLD in mice were investigated in this study. It was found that both LP and LPBs could reduce the liver oxidative injury, as suggested by the decreased liver MDA content. This may arise from the decreased CYP2E1 mRNA level and the increased SOD and GSH-Px levels by the two compounds. LP and LPBs were also found to decrease the fat accumulation and TG level in liver through activating AMPKα2 and inactivating SREBP-1c of alcohol-treated mice. Furthermore, the anti-inflammatory activity of the two compounds responding to alcoholic liver injury was suggested by the reduction in inflammatory cytokines TNF- α, IL- 1β, IL- 6, and TLR4, Myd88 mRNA level. This is consistent with the liver injury indexes, including TBil, AST, and ALT in both the preventive and therapeutic trials. As both pigment and polysaccharides are two important components in Lycium barbarum, and Lycium barbarum may be developed as a functional food in preventing and treating AFLD for human beings.
Abbreviations
AFLD: alcohol-induced fatty liver disease;
LP: Lycium barbarum pigment;
LBPs: Lycium barbarum polysaccharides;
TG: triglyceride;
TBil: total bilirubin;
ALT: alanine aminotransferase;
AST: aspartate aminotransferase;
SOD: superoxide dismutase;
MDA: malondialdehyde;
GSH-Px: glutathione peroxidase;
H&E: Hematoxylin and eosin.
Supplemental Material
Download MS Word (14.8 KB)Supplemental data
Supplemental data for this article can be accessed here.
Disclosure statement
No potential conflict of interest was reported by the authors.
Additional information
Funding
References
- Amagase, H., & Farnsworth, N. R. (2011). A review of botanical characteristics, phytochemistry, clinical relevance in efficacy and safety of Lycium barbarum fruit (Goji). Food Research International, 44(7), 1702–1717.
- Cave, M. C., Clair, H. B., Hardesty, J. E., Falkner, K. C., Feng, W. K., Clark, B. J., … Prough, R. A. (2016). Nuclear receptors and nonalcoholic fatty liver disease. Biochimica et Biophysica Acta, 1859(9), 1083–1099.
- Chacko, K. R., & Reinus, J. (2016). Spectrum alcoholic liver disease. Clinics in Liver Disease, 20(3), 419–427.
- Chandan, B. K., Saxena, A. K., Shukla, S., Sharma, N., Gupta, D. K., Singh, K., … Qazi, G. N. (2008). Hepatoprotective activity of Woodfordia fruticosa Kurz flowers against carbon tetrachloride induced hepatotoxicity. Journal of Ethnopharmacology, 119(2), 218–224.
- Chen, P., Miyamoto, Y., Mazagova, M., Lee, K. C., Eckmann, L., & Schnabl, B. (2015). Microbiota Protects Mice Against Acute Alcohol-Induced Liver Injury. Alcoholism-Clinical and Experimental Research, 39(12), 2313–2323.
- Cheng, D., & Kong, H. (2011). The Effect of Lycium barbarum polysaccharide on alcohol-induced oxidative stress in rats. Molecules, 16(3), 2542–2550.
- Cui, S., Wang, M., & Fan, G. (2002). Anti-HBV efficacy of bifendate in treatment of chronic hepatitis B, a primary study. Zhonghua Yi Xue Za Zhi, 82(8), 538–540.
- Gao, Z., Li, J., Song, X., Zhang, J., Wang, X., Jing, H., … Jia, L. (2017). Antioxidative, anti-inflammation and lung-protective effects of mycelia selenium polysaccharides from Oudemansiella radicata. International Journal of Biological Macromolecules, 104(Pt A), 1158–1164.
- Garcia-Gonzalez, C. A., Alnaief, M., & Smirnova, I. (2011). Polysaccharide-based aerogels-promising biodegradable carriers for drug delivery systems. Carbohydrate Polymers, 86(4), 1425–1438.
- Gong, G., Dang, T., Deng, Y., Han, J., Zou, Z., Jing, S., … Wang, Z. (2018). Physicochemical properties and biological activities of polysaccharides from Lycium barbarum prepared by fractional precipitation. International Journal of Biological Macromolecules, 109, 611–618.
- Guan, L. P., Nan, J. X., Jin, X. J., Jin, Q. H., Kwak, K. C., Chai, K. Y., & Quan, Z. S. (2005). Protective effects of chalcone derivatives for acute liver injury in mice. Archives of Pharmacal Research, 28(1), 81–86.
- Gui, S. Y., Wei, W., Wang, H., Wu, L., Sun, W. Y., & Wu, C. Y. (2005). Protective effect of fufanghuangqiduogan against acute liver injury in mice. World J Gastroenterol, 11(19), 2984–2989.
- Hamoud, A. R., Weaver, L., Stec, D. E., & Hinds, T. D. (2018). Bilirubin in the liver- gut signaling axis. Trends in Endocrinology and Metabolism, 29(3), 140–150.
- Hirayama, C., Kishimoto, Y., Wakushima, T., & Murawaki, Y. (1983). Mechanism of the protective action of thiol compounds in alcohol-induced liver injury. Biochemical Pharmacology, 32(2), 321–325.
- Hu, N., Zheng, J., Li, W. C., & Suo, Y. R. (2014). Isolation, stability and antioxidant activity ofanthocyanins from Lycium ruthenicum murray and nitraria tangutorum bobr. of qinghai-tibetan plateau. Separation Science and Technology, 49(18), 2897–2906.
- Ikarashi, N., Toda, T., Okaniwa, T., Ito, K., Ochiai, W., & Sugiyama, K. (2011). Anti-obesity and anti-diabetic effects of acacia polyphenol in obese diabetic KKAy mice fed high-fat diet. Evidence-Based Complementary and Alternative Medicine, (2011, 952031.
- Jin, H. L., Liu, Y. F., Yang, F., Wang, J. X., Fu, D. M., Zhang, X. L., … Liang, X. M. (2015). Characterization of anthocyanins in wild Lycium ruthenicum Murray by HPLC-DAD/QTOF-MS/MS. Analytical Methods, 7(12), 4947–4956.
- Kim, H., Pan, J. H., Kim, J. K., Kim, Y. J., Lee, J. H., & Park, J. W. (2017). Chlorogenic acid ameliorates alcoholic liver disease through MiRNA-mediated restoration of mitochondrial redox system. Faseb Journal, 31(1).
- Leung, T. M., & Nieto, N. (2013). CYP2E1 and oxidant stress in alcoholic and non-alcoholic fatty liver disease. Journal of Hepatology, 58(2), 395–398.
- Li, J., Sasaki, G. Y., Dey, P., Chitchumroonchokchai, C., Labyk, A. N., McDonald, J. D., … Bruno, R. S. (2018). Green tea extract protects against hepatic NF kappa B activation along the gut-liver axis in diet-induced obese mice with nonalcoholic steatohepatitis by reducing endotoxin and TLR4/MyD88 signaling. Journal of Nutritional Biochemistry, 53, 58–65.
- Li, X. J., Mu, Y. M., Li, T. T., Yang, Y. L., Zhang, M. T., Li, Y. S., … Shang, H. C. (2015). Gynura procumbens Reverses Acute and Chronic Alcohol-Induced Liver Steatosis through MAPK/SREBP-1c -Dependent and -Independent Pathways. Journal of Agricultural and Food Chemistry, 63(38), 8460–8471.
- Lu, Y., & Cederbaum, A. I. (2008). CYP2E1 and oxidative liver injury by alcohol. Free Radical Biology & Medicine, 44(5), 723–738.
- Masarone, M., Rosato, V., Dallio, M., Abenavoli, L., Federico, A., Loguercio, C., & Persico, M. (2016). Epidemiology and natural history of alcoholic liver disease. Reviews on Recent Clinical Trials, 11(3), 167–174.
- Masci, A., Carradori, S., Casadei, M. A., Paolicelli, P., Petralito, S., Ragno, R., & Cesa, S. (2018). Lycium barbarum polysaccharides: Extraction, purification, structural characterisation and evidence about hypoglycaemic and hypolipidaemic effects. A review. Food Chemistry, 254, 377–389.
- Mato, J. M., & Lu, S. C. (2007). Role of S-adenosyl-L-methionine in liver health and injury. Hepatology, 45(5), 1306–1312.
- Mouzaoui, S., Rahim, I., & Djerdjouri, B. (2012). Aminoguanidine and curcumin attenuated tumor necrosis factor (TNF)-alpha-induced oxidative stress, colitis and hepatotoxicity in mice. International Immunopharmacology, 12(1), 302–311.
- Muriel, P., & Rivera-Espinoza, Y. (2008). Beneficial drugs for liver diseases. Journal of Applied Toxicology, 28(2), 93–103.
- Naritomi, Y., Sanoh, S., & Ohta, S. (2018). Chimeric mice with humanized liver: Application in drug metabolism and pharmacokinetics studies for drug discovery. Drug Metab Pharmacokinet, 33(1), 31–39.
- OECD. (2001). OECD/OCDE 420 Guideline Document. In OECD Guideline for testing of chemicals. Acute oral toxicity- fixed dose procedure. 1–14.
- Pan, S. Y., Yang, R., Dong, H., Yu, Z. L., & Ko, K. M. (2006). Bifendate treatment attenuates hepatic steatosis in cholesterol/bile salt- and high-fat diet-induced hypercholesterolemia in mice. European Journal of Pharmacology, 552(1–3), 170–175.
- Potterat, O. (2010). Goji (Lycium barbarum and L. chinense): Phytochemistry, pharmacology and safety in the perspective of traditional uses and recent popularity. Planta Medica, 76(1), 7–19.
- Ren, Y. L., Geng, Y., Chen, H. D., Lu, Z. M., Shi, J. S., & Xu, Z. H. (2018). Polysaccharide peptides from Coriolus versicolor: A multi-targeted approach for the protection or prevention of alcoholic liver disease. Journal of Functional Foods, 40, 769–777.
- Rutter, G. A., Da Silva Xavier, G., & Leclerc, I. (2003). Roles of 5ʹ-AMP-activated protein kinase (AMPK) in mammalian glucose homoeostasis. The Biochemical Journal, 375(Pt 1), 1–16.
- Sen, A., Marsche, G., Freudenberger, P., Schallert, M., Toeglhofer, A. M., Nagl, C., … Schmidt, H. (2014). Association between higher plasma lutein, zeaxanthin, and vitamin C concentrations and longer telomere length: Results of the Austrian stroke prevention study. Journal of the American Geriatrics Society, 62(2), 222–229.
- Shankar, A., Klein, R., & Klein, B. E. (2006). The association among smoking, heavy drinking, and chronic kidney disease. American Journal of Epidemiology, 164(3), 263–271.
- Song, X., Shen, Q., Liu, M., Zhang, C., Zhang, L., Ren, Z., … Jia, L. (2018). Antioxidant and hepatoprotective effects of intracellular mycelium polysaccharides from Pleurotus geesteranus against alcoholic liver diseases. International Journal of Biological Macromolecules, 114, 979–988.
- Sultan, C. S., Saackel, A., Stank, A., Fleming, T., Fedorova, M., Hoffmann, R., … Wagner, A. H. (2018). Impact of carbonylation on glutathione peroxidase-1 activity in human hyperglycemic endothelial cells. Redox Biology, 16, 113–122.
- Ulbricht, C., Bryan, J. K., Costa, D., Culwell, S., Giese, N., Isaac, R., … Zhou, S. (2015). An evidence-base systematic review of Goji (Lycium spp.) by the Natural Standard Research Collaboration. Journal of Dietary Supplements, 12(2), 184–240.
- Wang, C. C., Chang, S. C., Inbaraj, B. S., & Chen, B. H. (2010). Isolation of carotenoids, flavonoids and polysaccharides from Lycium barbarum L. and evaluation of antioxidant activity. Food Chemistry, 120(1), 184–192.
- Weismann, D., & Binder, C. J. (2012). The innate immune response to products of phospholipid peroxidation. Biochimica Et Biophysica Acta-Biomembranes, 1818(10), 2465–2475.
- Williams, J. A., Manley, S., & Ding, W. X. (2014). New advances in molecular mechanisms and emerging therapeutic targets in alcoholic liver diseases. World Journal of Gastroenterology, 20(36), 12908–12933.
- Wu, H. T., He, X. J., Hong, Y. K., Ma, T., Xu, Y. P., & Li, H. H. (2011). Chemical characterization of lycium barbarum polysaccharides and its inhibition against liver oxidative injury of high-fat mice. International Journal of Biological Macromolecules, 46(5), 540–543.
- Xiao, J., Wang, J. T., Xing, F. Y., Han, T., Jiao, R., Liong, E. C., … Tipoe, G. L. (2014). Zeaxanthin dipalmitate therapeutically improves hepatic functions in an alcoholic fatty liver disease model through modulating MAPK pathway. Plos One, 9, 4.
- Yang, X. B., Yang, S., Guo, Y. R., Jiao, Y. D., & Zhao, Y. (2013). Compositional characterisation of soluble apple polysaccharides, and their antioxidant and hepatoprotective effects on acute CCl4-caused liver damage in mice. Food Chemistry, 138(2–3), 1256–1264.
- Yao, X., Peng, Y., Zhou, Q., Xiao, P. G., & Sun, S. Q. (2010). Distinction of eight Lycium species by Fourier-transform infrared spectroscopy and two-dimensional correlation IR spectroscopy. Journal of Molecular Structure, 974(1–3), 161–164.
- Yuan, R., Tao, X., Liang, S., Pan, Y., He, L., Sun, J., … Wang, C. (2018). Protective effect of acidic polysaccharide from Schisandra chinensis on acute alcohol-induced liver injury through reducing CYP2E1-dependent oxidative stress. Biomedicine & Pharmacotherapy, 99, 537–542.
- Zeng, T., Zhang, C. L., Song, F. Y., Zhao, X. L., Yu, L. H., Zhu, Z. P., & Xie, K. Q. (2013). The activation of HO-1/Nrf-2 contributes to the protective effects of diallyl disulfide (DADS)against alcohol-induced oxidative stress. Biochimica Et Biophysica Acta-General Subjects, 1830(10), 4848–4859.
- Zeng, T., Zhang, C. L., Zhao, N., Guan, M. J., Xiao, M., Yang, R., … Xie, K. Q. (2018). Impairment of Akt activity by CYP2E1 mediated oxidative stress is involved in chronic alcohol-induced fatty live. Redox Biology, 14, 295–304.
- Zhang, L. J., Zhao, Q. S., Wang, L. W., Zhao, M. X., & Zhao, B. (2017). Protective effect of polysaccharide from maca (Lepidium meyenii) on Hep-G2 cells and alcoholic liver oxidative injury in mice. International Journal of Biological Macromolecules, 99, 63–70.
- Zhao, J., Chen, H., & Li, Y. (2008). Protective effect of bicyclol on acute alcohol-induced liver injury in mice. European Journal of Pharmacology, 586(1–3), 322–331.
- Zou, S., Zhang, X., Yao, W. B., Niu, Y. G., & Gao, X. D. (2010). Structure characterization and hypoglycemic activity of a polysaccharide isolated from the fruit of Lycium barbarum L. Carbohydrate Polymers, 80(4), 1161–1167.