ABSTRACT
Meat spoilage is a metabolic process where the change in sensory characteristics deems it to be unacceptable for human consumption. Meat spoilage typically occurs under conditions of optimal water availability, low oxygen, and low temperature conditions, where growth conditions are ideal for microbial growth. Technological advances in the food industry have been at high demand for the past decade, to ensure food safety and health regulations are met using earlier detection methods. Detection technologies such as polymerase chain reactions, spectroscopy, odor sensors devices such as the electric nose are just a few methods used to identify meat spoilage.
RESUMEN
El deterioro de la carne es un proceso metabólico en el que los cambios en las características sensoriales de la carne dan cuenta de que ésta es inaceptable para el consumo humano. Típicamente, dicho deterioro ocurre bajo condiciones de óptima disponibilidad de agua, bajo nivel de oxígeno y baja temperatura, es decir, condiciones ideales para el crecimiento bacteriano. Durante la última década, en la industria alimentaria se ha producido una fuerte demanda de avances tecnológicos en aras de garantizar la seguridad alimentaria y cumplir con las regulaciones sanitarias utilizando dichas tecnologías. Algunos métodos empleados para identificar el deterioro de la carne, entre otros, implican el uso de tecnologías de detección como reacciones en cadena de la polimerasa, espectroscopia y sensores de olor, por ejemplo, la nariz electrónica.
Introduction
Increasingly significant public concerns about sub-quality foods have been linked to increased morbidity, mortality, human suffering, and economic burden. These have become the drivers towards industry compliance for ensuring both high food quality that is compliant with internationally accepted food safety best practices (Cozzolino et al., Citation2018). Similarly, significant efforts within the meat industry to ensure reduced food wastage, poisoning incidences, and spoilage have been identified (Dou et al., Citation2016). Due to the exponential population growth, the economic importance of these technological advances has played a vital role as the quality of life and nutritional needs are becoming of higher demand among society (Kristensen, Støier, Würtz, & Hinrichsen, Citation2014).
Often perceived through changes in sensory characteristics, food spoilage can be defined as a metabolic process which causes food products to be unacceptable for human consumption (Rawat, Citation2015). Within every industry, there are set guidelines which dictate how the product is manufactured and stored to ensure safety all around. Meat is one of many food types that will perish rapidly if not refrigerated or kept in optimal conditions (Sarioglu, Celebioglu, Tekinay, & Uyar, Citation2016). Its composition allows for the ideal growth of a wide range of microorganisms by supplying a conducive environment for their nutritional and reproductive needs (Mayr et al., Citation2003). The process of meat spoilage is often rapid, especially when driven by bacteria, which can multiply to high densities within a short period of time; for example, the generation time for Escherichia coli is every 20 min (Roostalu, Jõers, Luidalepp, Kaldalu, & Tenson, Citation2008). The process of meat spoilage is highly dependent on a number of environmental factors and conditions, as each microorganism may require niche survival conditions to optimize its growth, source its nutrition and reproduce (Zinjarde, Citation2014). Altered conditions of nutrient availability, oxygen demand, temperature, and other competing organisms all affect microbial growth (Ayrapetyan, Williams, & Oliver, Citation2015; Cross, Hood, Benstead, Huryn, & Nelson, Citation2015).
Microorganisms require water, in any form, for growth and nutritional needs. Water present in food can be in two forms: either proportional or unavailable (Beuchat et al., Citation2013). Proportional water is where the water molecules are present in sufficient amounts to support growth whereas unavailable water is where the water molecules are occluded through binding to other solutes such as salt or sugar. This is the rationale for meat preservation using salt as this practice reduces the probability for microbial growth. Some foodborne molds, yeasts, and bacteria have developed a resilience to the lowered availability of water as they can adjust internal constitutes to compensate for external water activities (Bell, Jarvis, Ottesen, McFarland, & Brown, Citation2016). The water activity of a food can therefore be an important indicator of whether an environment is ideal for microbial growth (Syamaladevi et al., Citation2016). Oxygen is a critical requirement for survival of aerobic-preferring microorganisms, whereas detrimental to others (anaerobic). Some bacterium can survive in both oxic and anoxic conditions, and are referred to as facultative bacteria (Fu, Yuan, & Gao, Citation2015). In meat, for example, the spoilage-causing bacteria are most commonly yeasts and spore-forming bacteria, as they do not require oxygen for growth. Spore-forming bacteria are typically associated with meat spoilage incidences because their reproductive structures, spores, can remain unharmed during low oxygenated conditions (Clarke, Citation2014). These anaerobic bacteria are predominantly the cause of some meat spoilage incidences such as slime on meats because they can survive vacuum packaging, for example.
Similarly to oxygen, microorganisms also have an optimal temperature range for growth; for example, E.coli have an optimal growth temperature of 37°C (Gadgil, Kapur, & Hu, Citation2005). For a typical bacterial cell, the temperature range in which they can still maintain growth is 4–60°C. For this reason, food safety guidelines recommend refrigeration of meat products to be below the 4 oC limit to prevent microbial growth and meat spoilage (Ercolini et al., Citation2011).
Polymerase chain reactions
Most regulators have adopted zero tolerance for the presence of Salmonella in ready-to-eat foods sold or dispensed within their jurisdictions (Rodriguez-Lazaro et al., Citation2014). Until 2016, the presence of Salmonella detected within a 25 g sample of a food was considered to render it spoilt under ISO standards. However, subsequently, with the adoption of ISO 6579, a new, composite guideline for food characterization for bacterial loadings has since come into effect (Mooijman, Citation2018).
Polymerase chain reactions (PCR) are reactions that are used to rapidly replicate sections of DNA to allow for both detection and identification of species present (White et al., Citation2013). The process uses specific DNA polymerases to enable the replication of target sections of DNA, via three steps: denaturation, annealing and extension, all of which need three different temperatures (high-temperature denaturation: 90‒95°C, low temperature annealing: 55‒60°C and middle-temperature extension: 70‒72°C) (He, Huang, Huang, & Chen, Citation2016).
The PCR method used is based on earlier studies which used known samples of Salmonella to develop a methodology for isolating and replicating the various Salmonella strains (Rodriguez-Lazaro, Hernández, Esteve, Hoorfar, & Pla, Citation2003). This method allowed for 100% differentiation of the pure Salmonella and other bacteria samples. When the sample preparation was combined with the PCR method, accurate identification of the presence of Salmonella was able to be found within 21 h compared to the seven days taken by the current standard (Rodriguez-Lazaro et al., Citation2014). This method has been validated by several laboratories across Europe who found that not only was its accuracy reliable, it represented an 83% decrease in costs compared to the current methods (Delibato et al., Citation2014). PCR has also found use in detecting bacteria that can spoil vacuum packaged meats. These bacteria are able to grow in temperatures of −1.5°C (Brightwell & Clemens, Citation2012; Gribble & Brightwell, Citation2013). As this temperature is the optimal storage temperature for the transport of red meat, the early screening of these bacteria in the meat is important. Of particular note are Brochothrix campestris and Brochothrix thermosphacta which can cause spoilage within 9 weeks of storage (Gribble & Brightwell, Citation2013). PCR has been used to identify and differentiate the two bacteria accurately for the first time. Previous methods did not differentiate between the two species and thus it is speculated that spoilage characteristics have been incorrectly attributed to both. Another key microorganism that can be detected via PCR is Clostridum estertheticum (Brightwell & Clemens, Citation2012). This bacterium causes the buildup of gas during the spoilage of vacuum-packed meats resulting in the bags bursting or blown pack spoilage. There are many species in the Clostridium strain, however, only C. estertheticum causes the blown pack spoilage, it is important to be able to accurately detect this strain so that unspoiled meat is not wasted. The previous method required 3–4 weeks of enrichment before testing was done so this method has been able to speed this detection up considerably and allow for cheaper and faster detections of the bacteria.
Reid and collaborators have developed a set of real-time PCR methods for the detection of various Clostridium species (C. estertheticum, C. gasigenes, and C. ruminantium, the causative agents of blown pack spoilage (BPS) in vacuum packaged beef. Robust validation of the sensitivity and specificity in three matrices (beef meat drip, wet environmental swabs and dry environmental swabs) as encountered in their testing laboratory and against Clostridium strains (n = 76) and non-Clostridium strains (n = 36) was undertaken. The authors reported successful detection or 4–5 spores per ml for C. estertheticum, 2 spores per ml for C. gasigenes and 8 spores per ml for C. ruminantium, without the need for sample enrichment (Reid et al., Citation2017). The team’s efforts in deriving optimized sensitivity and specificity parameters have translated in the detection of bacterial spores in extremely low numbers.
Very recently, Illikoud and collaborators have evaluated the genotypic and phenotypic diversity of Brochothrix thermosphacta, which is one of the major bacterial species involved in meat and seafood spoilage of meat and seafood. The authors collected 161 B. thermosphacta strains isolated from different foods, spoiled and otherwise, and from a slaughterhouse environment was collected completed with new isolates. Using the rpoB gene, a PCR test was thus developed for a fast screening of B. thermosphacta isolates. The strains were distinguished using Matrix Assisted Laser Desorption/Ionization Time of Flight Mass Spectrometry, mass spectrometry, Repetitive-element Palindromic PCR, and Pulsed-field Gel Electrophoresis. In this manner, the strains were put into distinct groups, revealing significant intra-species diversity. The authors stated that these classifications did not correlate with the ecological origin of strains. The ability to produce acetoin and diacetyl, two molecules associated with B. thermosphacta spoilage, was also evaluated in meat and shrimp juices. The production level was variable between strains and the spoilage ability on meat or shrimp juice did not correlate with the substrate origin of strains. The study concluded that despite the B. thermosphacta species encompassing ubiquitous strains, spoilage ability was both strain- and environment-dependent (Illikoud et al., Citationin press). The importance of this recent work is underscored by its affirmation that meat spoilage depends both on bacterial strain and the environment.
In another study, Stanborough and coworkers sequenced the genomes of 12 Pseudomona fragi and 7 Pseudomona lundensis isolates to understand the effect of both spoilage agents on meat and milk. The approach involved determining key volatile organic compounds (VOCs) produced or metabolized by the isolates during their growth on a beef paste and where possible, metabolic activity was associated with gene repertoire. Genome analyses showed that the isolates included in this work may belong to more than two Pseudomonas species with possible spoilage potential. Further, pan-genome analyses demonstrated a high degree of diversity among the P. fragi and genetic flexibility and diversity may be traits of both species. The authors also found that growth of the P. lundensis isolates was characterized by the production of large amounts of 1-undecene, 5-methyl-2-hexanone, and methyl-2-butenoic acid. On the other hand, P. fragi isolates were found to produce extensive amounts of methyl and ethyl acetate and the production of methyl esters predominated over ethyl esters. Some of the P. fragi also produced extremely low levels of VOCs, highlighting the importance of strain-specific studies in food matrices (Stanborough et al., Citation2018). This work highlights the variations in meat spoilage outcomes from within the same genus of bacteria, largely driven by the nature of the chemicals produced, which varies between the strains.
Don and coworkers have studied the effect of temperature in spoilage of the fresh shrimp, Litopenaeus vannamei, which were collected from tropical farm sites and stored at three different temperatures (30 ± 2°C room temperature, 4°C refrigerated and 1°C ice). The authors carried out sensory, biochemical, and microbial evaluation of the samples at frequent intervals and estimated the shelf life of the samples at 10 h, 12 days and 16 days at room temperature, refrigerated and ice storage, respectively. In ice storage, total volatile base nitrogen values increased at the initial days, followed by a decrease, and subsequently, an increase, attributed to the leaching out of ammonia by the melt water. These authors also examined the fitness of three Relative Rate of Spoilage (RRS) models to determine the shelf life of L. vannamei, and found the Arrhenius model to be more fitting. Spoilage potential evaluation was carried out by screening the bacterial isolates at the time of sensory rejection and the results revealed that many isolates possess high potential for spoilage. The 16SrRNA gene sequencing analysis identified the predominant potential spoilers as: Enterobacter and Acinetobacter at room temperature, Pseudomonas and Aeromonas at refrigerated storage and, Aeromonas and Enterococcus at ice storage (Don, Xavier, Devi, Nayak, & Kannuchamy, Citation2018). Similar to the findings of Stanborough and collaborators, Don and coworkers have identified the nitrogenous compound pathway to be ultimately associated with shrimp meat spoilage.
Spectroscopy
Many emerging techniques are designed to detect and quantify the spoilage as early as possible (Lohumi, Lee, Lee, & Cho, Citation2015; Pu, Wang, & Alfano, Citation2013). One of these emerging techniques is spectroscopy which is an advanced detection technique that functions on the interaction between matter and electromagnetic radiation of varying wavelengths (Nuernberger, Ruetzel, & Brixner, Citation2015; Pu et al., Citation2013). It is used to measure light that is emitted, absorbed, or scattered by materials and can be used to study, identify and quantify materials or substances of various biological complexity (Lohumi et al., Citation2015). Of the methods based on spectroscopy, several have been applied for a myriad of applications, too vast to cover here. As a summary, however, notable uses, often aided with robust statistical and chemometric analysis include laser-induced breakdown spectroscopy and Raman spectroscopy for food analysis and adulteration (Esteki, Shahsavari, & Simal-Gandara, Citation2018; Li & Church, Citation2014; Markiewicz-Keszycka et al., Citation2017; Sezer, Bilge, & Boyaci, Citation2017, Temiz, Sezer, Berkkan, Tamer, & Boyaci, Citation2018), fluorescence spectroscopy for beverage discrimination (Sádecká, Jakubíková, & Májek, Citation2018; Gordon, Cozzolino, Chandra, Power, Roberts, & Chapman, Citation2017), food authenticity testing (Dankowska, Citation2016), changes due to variable process-treatments (Liu, Zamora, Castillo, & Saldo, Citation2018) or even pungency in spicy food (Monago-Maraña, Guzmán-Becerra, Muñoz de la Peña, & Galeano-Díaz, Citation2018). Mid infrared (MIR) applications include MIR with chemometrics applied to food and beverage classification (Craig, Botelho, Oliveira, & Franca, Citation2018), monitoring shelf-life (Bureau, Georgé, Perrin, & Renard, Citation2017), and food value-adding (Coppa, Revello-Chion, Giaccone, Tabacco, & Borreani, Citation2017). Similarly, attenuated total reflectance (ATR)-IR spectroscopy has been useful for several applications, for example, in the detection and identification of food adulterants (Deconinck, Aouadi, Bothy, & Courselle, Citation2018), screening for food toxins (Jaiswal, Jha, Kaur, Borah, & Ramya, Citation2018) and quantification of food constituents (Anjos, Campos, Ruiz, & Antunes, Citation2015) among others. Both of these techniques are non-destructive, which is particularly advantageous in their application to food.
Oto and collaborators have investigated the potential of fluorescence spectroscopy for the non-destructive evaluation of adenosine triphosphate (ATP) content and plate count on pork meat surface stored aerobically at 15°C during three days. Excitation (Ex) Emission (Em) Matrix of fluorescence intensity was obtained and fluorescence from tryptophan (Ex = 295 nm and Em = 335 nm) and nicotinamide adenine dinucleotide phosphate (Ex = 335 nm and Em = 450 nm) was detected. The authors found that changing fluorescence behavior of both analytes arising from the growth of microorganisms could be an indicator of microbial spoilage on meat. By applying partial least squares (PLS) regression analysis, ATP content and plate count were predicted with good determination coefficient (0.94–0.97 in calibration and 0.84–0.88 in validation) (Oto et al., Citation2013). Shirai et al. have similarly applied excitation–emission matrix (EEM) spectroscopy in the rapid, non-destructive evaluation of cleanliness in meat processing plants using ATP as an indicator of microbial contamination. In their work, the fluorescent signal of ATP was detected at Ex = 286 nm and Em = 386 and 412 nm by applying two-dimensional Savitzky–Golay second-order differentiation of EEM obtained from the pork meat surface. The second derivative of the fluorescence intensity at Ex = 284 nm and Em = 412 nm, i.e. the wavelengths assigned to ATP, decreased with the ATP content. The ATP content and plate count were quantified using the second derivatives of EEMs by partial least squares regression in good agreement [coefficient correlation, 0.87; root mean square error of prediction, log10 (0.70, mol cm−2) for the predicted ATP content]. The model directly reflected the ATP fluorescent signal changes (Shirai, Oshita, & Makino, Citation2016). Recently, Han and collaborators have synthesized a class of positional isomers by attaching a carboxylic group in different sites (para-, meta- and ortho-position) of an aromatic core. These isomers underwent both aggregation-induced emission (AIE) and intramolecular charge transfer (ICT) mechanisms, verified by their photoluminescence behavior. The authors reported that by changing the position of the carboxylic group, the dipole–dipole direction is altered as well, which resulted in a transition from 1D nanowire to 2D microsheet, and even to 3D microcube. The benzoic acid moiety of the AIE isomers also showed strong affinity to amines, and adsorbing amines leads to spectral changes including hypochromatic shift and emission quenching. Additionally, the sensing mechanism was studied by density functional theory, which revealed that the amine-responsive fluorescence can be ascribed to the weakened ICT effect. Ultimately, a prototype of amine sensor based on m-DB self-assemblies was developed accordingly, showing a remarkable quenching efficiency as high as 85.4%. A linear relation, obtained from the quantitative determination of amine also offered a low detection limit of 2.02 Pa. These authors suggested that the prototype could also respond to other organic amines such as diisopropylamine, triethylamine, isopropylamine, and cyclohexylamine. Finally, the sensing film, when placed beside spoiled meat, yielded a spectrum showing a remarkable fluorescence quenching. In contrast, a much lower quenching efficiency was observed with fresher meat samples, indicating that the AIE isomers had the potential to serve as meat spoilage indicators (Han et al., Citation2018). Similarly, Sahar and Dufour have explored the potential of MIR spectroscopy to determine spoilage microorganism on the surface of chicken breast fillets that were kept aerobically at 5°C for 0, 1, 2, 3, 5 and 8 days, and at 15°C for 0, 0.5, 1, 2, 3, and 5 days. Their findings revealed that MIR spectroscopy (4000–1000 cm−1 range) coupled with ATR accessory can be used directly on the surface of meat samples to produce fingerprints. Culture-dependent methods were used to determine total viable count (TVC), Pseudomonas, Enterobacteriaceae and B. thermosphacta on chicken breast fillets at each step of the 2 kinetics. Based on the MIR spectra, with PLS discriminant analysis, the results showed 100% of good classifications for the six investigated storage times using 4 PLS factors. PLS regression was carried out to predict the microbial counts from the MIR spectral data and thus, a food microbial degradation index (Sahar & Dufour, Citation2014).
Another spectroscopic method finding increasing appeal in the meat and food industries is near infrared (NIR), which encompasses the further defined technique Fourier transform near-infrared (FT-NIR) spectroscopy. Together, FT-NIR spectroscopy analysis applies the spectral absorption of the overtone and combination of molecule-level vibrations of a functional group in the food matrix. Like MIR and ATR, its notable advantages include high specificity, ease of operation, rapid analysis time, low cost, and non-destructive and non-invasive properties (Chen, Liu, Cai, Xu, & Chen, Citation2018). Similarly to fluorescence spectroscopy, NIR and FT-NIR measure food spoilage through the presence of biological molecules and chemicals in the food sample. By testing for elevated or decreased levels of compounds such as proteins, lipids, collagen, and metabolic waste, one can predict the nature of microbial organisms at the time of measurement, and consequently predict the remaining ‘shelf-life’ of the sample (Lohumi et al., Citation2015; Shapaval et al., Citation2012). FT-NIR has also been shown the capacity for measuring specific fungal species within aqueous food samples. These fungal species were some of the most common in terms of meat spoilage causes, and were identified with a rate of 94.0% (Shapaval et al., Citation2012). Following this, total viable counts of bacteria have been performed to estimate food spoilage, as well bacterial identification processes in assessing harmful microorganisms through chemometrics have been conducted (Lohumi et al., Citation2015). Although FT-NIR spectroscopy has many applications and advantages, the process itself still is very recent, and with it comes disadvantages. The process involves much calibration, and the resolution of the scans taken plays a role in the amount of time needed to complete analysis, making FT-NIR a time-consuming process (Lohumi et al., Citation2015). As well as this, the computer programs and instrumentation needed to perform the analysis are not readily available to the broader community (Zheng & He, Citation2014).
Spectroscopy has shown great precision with identifying specific molecules [such as NADH] as well varying fungal and bacterial species. In relation to meat spoilage and food safety, the future of spectroscopy is an open field were constant innovations and improvements are being made to refine methods for use in houses and businesses.
Odour sensors and electronic nose technology
These type of sensors are based on human olfaction, the electronic nose (or e-nose) is modeled after the chemical interactions between odor compounds and primary neurons found in the human nasal cavity (Ghasemi-Varnamkhastim, Mohtasebi, Siadat, & Balasubramanian, Citation2009). The e-nose technology consists of many electrochemical sensors and pattern classification algorithms which can detect odors, resembling the correspondence between the primary neurons of the nasal cavity and the chemical sensors of the e-nose (Hasan, Ejaz, Ejaz, & Kim, Citation2012). There are many advancements in sensor technology and many types are chemosensors readily available. Of these products, metal oxide semiconductor (MOS) and conducting organic polymer (CP) will be briefly reviewed in this section (Hasan et al., Citation2012).
Conducting organic polymers
Conducting polymers (CP) are a set of active mechanisms which detect odors and convert chemical vapors into electrical signals (Casalinuovo, Di Pierro, Coletta, & Di Francesco, Citation2006). Furthermore, CP have vital mechanical properties that allow for construction of food spoilage sensors (Bai & Shi, Citation2007). Conducting polymer characteristics depend on doping levels, ion size of the dopant, water content, and protonation levels. Use of diagnostic medical reagents and distinguishable chemical memory are the primary materials of industrial sensors. These sensors may be classified into mode of transduction and application (Gupta et al., Citation2006).
Despite these advantages, the research outputs involving CP-based e-noses has been relatively limited. As early as 2001, work by Neely and collaborators has looked at developing semiconductor-based e-noses to distinguish between alpaca and llama meats in Peru and Bolivia (Neely, Taylor, Prosser, & Hamlyn, Citation2001). With the aid of linear discriminant analysis of data generated, the authors were able to apply their device to the examination of meat and reported success, as well as the problems experienced in analyzing the data relating to sample size (Neely et al., Citation2001). Since this work, little reports of e-noses based on CPs applied towards meat examination has been emerging, with the bulk of the e-nose sensors based on MOS-based modifications.
Metal oxide semiconductor
The metal oxide semiconductor MOS is the most commonly used in e-noses (Majchrzak, Wojnowski, Dymerski, Gębicki, & Namieśnik, Citation2018). It is a form of a gas sensor which can detect early signs of meat spoilage. For example, Timsorn and coworkers have proposed an e-nose based on eight MOS sensors that was used for evaluation of chicken meat freshness and bacterial population on chicken meat stored at 4.0°C and 30.0°C for up to 5 days (Timsorn, Thoopboochagorn, Lertwattanasakul, & Wongchoosuk, Citation2016). Based on principal component analysis (PCA) for pattern recognition and classification, the authors demonstrated their results to illustrate the classification of chicken meat freshness corresponding to different storage days and temperatures. Furthermore, the e-nose exhibited good correlation (0.94) of bacterial population on chicken, suggesting that the developed e-nose system can be used as a rapid and alternative way for evaluation of bacterial population on meats and offers several advantages including fast, portable, low cost, and non-destructive measurement with high relative accuracy (Timsorn et al., Citation2016). Kachele and colleagues evaluated the chemical changes, microbial load and sensory attributes of silver carp fillets when packaged at two vacuum levels (30 and 50 kPa) and stored at 4°C for 14 days, using an e-nose (Kachele, Zhang, Gao, & Adhikari, Citation2017). Their detection system was reported to be based on many metal oxide semiconductor sensors combined with pattern recognition algorithms were used to construct the intelligent bionic olfactory e-nose system. The findings provided valuable points on improving aspects of vacuum packaging, for example, headspace and refrigeration conditions as well as duration. Zhang and collaborators have similarly developed an e-nose based on MOS-sensors for characterizing microbial colonies developed during refrigeration of vacuum-packed Yao meat (Zhang, Yao, Gao, Wang, & Xu, Citation2018). Their findings provided new insights on packaging methods and areas for improvements.
Zou and coworkers assessed the effects of ultrasonic assisted cooking on the chemical profiles of spiced beef taste and flavor using an e-nose system equipped with 18 metal oxide semiconductor sensors (Zou et al., Citation2018). The authors found that ultrasonic treatment could significantly increase the content of sodium, and some essential amino acid content and the essential amino acid/non-essential ratios in beef with the ultrasound treatment. They concluded that the application of ultrasound during cooking has a positive effect on chemical profiles of spiced beef taste and flavor (Zou et al., Citation2018). A similar e-nose system to Zou and collaborators has been employed by Wang and coworkers who have examined the flavor qualities of three edible parts of three types of Chinese mitten crab from different areas (Wang et al., Citation2016). According to the authors, the flavor profiles detected by the e-nose (and an e-tongue) showed that differences existed in tastes and odors among wild-caught crabs, while the total free amino acids contents of wild crabs were all at the highest level in meat, gonads, and hepatopancreas. The ovaries had the highest nucleotides content and equivalent umami concentration (EUC) than other tissues in both female and male. The EUC was the highest in all parts of wild crabs as well (Wang et al., Citation2016). Elsewhere, Wang and coworkers evaluated the sensory qualities of the fresh chilled pork (artificially contaminated with Salmonella) using a portable e-nose containing a detector unit composed of an array of 10 different MOS-type chemical sensors (Wang et al., Citation2017). The authors found that the e-nose was able to assist in detection and minimization of odor and therefore, improve the sensory outlook of chilled pork (Wang et al., Citation2017).
Future and applications of spoilage technologies
In , we present a summary of technologies discussed in this review. From the emerging trends in the literature, PCR will continue to see use into the future of meat spoilage detection as the library of known bacterial sequences increases as well as the development of specific primers and DNA esterases used to amplify the target DNA sequences. Due to its inherent specificity, it will find use proving conclusively the presence or lack thereof of bacterial specimens.
Figure 1. Summarized depiction of technologies for meat spoilage detection as discussed in this review. Note: LIBS represents laser-induced breakdown spectroscopy.
Figura 1. Representación resumida de las tecnologías para la detección del deterioro de la carne abordadas en esta revisión. Nota: LIBS significa espectroscopia de plasma inducido por láser.
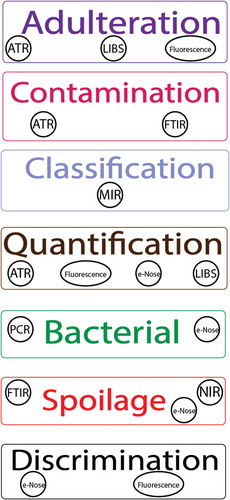
Technological evolution has seen spectroscopy from the 20th century being very different to that if the 21st century. With the advancement of computing and digital programming, researchers can more accurately measure and quantify data collected from spectroscopic methods. The field of spectroscopy itself is moving towards more advanced methods of spectral imaging, such as surfaced-enhanced Raman spectroscopy [SERS] and hyperspectral imaging [HIS] (Hagen & Kudenov, Citation2014; Wu & Sun, Citation2013). These techniques are still relatively new, but can provide greater precision, accuracy, clarity, and identification of sampled materials (Schlücker, Citation2014). Through these new techniques, the advancement of computing software and the minimization of technology through branches of science [such as nanoscience], spectroscopy machines are predicted to become more readily available to businesses and households in the foreseeable future (Ramsden, Citation2011; Sun, Citation2016).
Odor sensors and e-nose are modeled after odor compounds and primary neurons, an interaction found during the process of human olfaction (Ghasemi-Varnamkhastim et al., Citation2009). This technology is used in an industrial setting to identify spoilage in food, particular advancements are being made in the area of meat spoilage. These technologies are still relatively new, but many improvements are being made to increase accuracy and efficiency. With more advancements being made in technology, it can be said that odor sensory and e-nose technology will become more readily available to smaller companies and businesses, in order to prevent meat spoilage and promote early detection. This may be the integral steps towards making sure that the final product is at sufficient quality when presented for human consumption (Wilson, Citation2013).
Highlights
PCR technology is often the industry standard for food studies
Spectroscopic and electrochemical detection platforms are emerging new alternatives
Chemometrics has become an invaluable discriminate tool for data analysis
Disclosure statement
No potential conflict of interest was reported by the authors.
References
- Anjos, O., Campos, M. G., Ruiz, P. C., & Antunes, P. (2015). Application of FTIR-ATR spectroscopy to the quantification of sugar in honey. Food Chemistry, 169, 218–223.
- Ayrapetyan, M., Williams, T. C., & Oliver, J. D. (2015). Bridging the gap between viable but non-culturable and antibiotic persistent bacteria. Trends in Microbiology, 23(1), 7–13.
- Bai, H., & Shi, G. (2007). Gas sensors based on conducting polymers. Sensors, 7, 267–307.
- Bell, R. L., Jarvis, K. G., Ottesen, A. R., McFarland, M. A., & Brown, E. W. (2016). Recent and emerging innovations in Salmonella detection: A food and environmental perspective. Microbial Biotechnology, 9(3), 279–292.
- Beuchat, L. R., Komitopoulou, E., Beckers, H., Betts, R. P., Bourdichon, F., Fanning, S., … Ter Kuile, B. H. (2013). Low–Water activity foods: Increased concern as vehicles of foodborne pathogens. Journal of Food Protection, 76(1), 150–172.
- Brightwell, G., & Clemens, R. (2012). Development and validation of a real-time PCR assay specific for Clostridium estertheticum and C. estertheticum-like psychrotolerant bacteria. Meat Science, 92(4), 697–703.
- Bureau, S., Georgé, S., Perrin, A., & Renard, C. M. G. C. (2017). Use of mid-infrared spectroscopy to monitor shelf-life of ready-made meals. LWT - Food Science and Technology, 85, 474–478.
- Casalinuovo, I. A., Di Pierro, D., Coletta, M., & Di Francesco, P. (2006). Application of electronic noses for disease diagnosis and food spoilage detection. Sensors, 6, 1428–1439.
- Chen, H., Liu, Z., Cai, K., Xu, L., & Chen, A. (2018). Grid search parametric optimization for FT-NIR quantitative analysis of solid soluble content in strawberry samples. Vibrational Spectroscopy, 94, 7–15.
- Clarke, W. S., System for cultivation and processing of microorganisms, processing of products therefrom, and processing in drillhole reactors. 2014, Google Patents.
- Coppa, M., Revello-Chion, A., Giaccone, D., Tabacco, E., & Borreani, G. (2017). Could predicting fatty acid profile by mid-infrared reflectance spectroscopy be used as a method to increase the value added by milk production chains? Journal of Dairy Science, 100(11), 8705–8721.
- Cozzolino, D., Chandra, S., Roberts, J., Power, A., Rajapaksha, P., Ball, N., … Chapman, J. (2018). There is gold in them hills: Predicting potential acid mine drainage events through the use of chemometrics. Science of the Total Environment, 619–620, 1464–1472.
- Craig, A. P., Botelho, B. G., Oliveira, L. S., & Franca, A. S. (2018). Mid infrared spectroscopy and chemometrics as tools for the classification of roasted coffees by cup quality. Food Chemistry, 245, 1052–1061.
- Cross, W. F., Hood, J. M., Benstead, J. P., Huryn, A. D., & Nelson, D. (2015). Interactions between temperature and nutrients across levels of ecological organization. Global Change Biology, 21(3), 1025–1040.
- Dankowska, A. (2016). 5 - advances in fluorescence emission spectroscopy for food authenticity testing A2 - Downey, Gerard, in advances in food authenticity testing (pp. 117–145). Duxford, UK: Woodhead Publishing.
- Deconinck, E., Aouadi, C., Bothy, J. L., & Courselle, P. (2018). Detection and identification of multiple adulterants in plant food supplements using attenuated total reflectance—Infrared spectroscopy. Journal of Pharmaceutical and Biomedical Analysis, 152, 111–119.
- Delibato, E., Rodriguez-Lazaro, D., Gianfranceschi, M., De Cesare, A., Comin, D., Gattuso, A., … De Medici, D. (2014). European validation of real-time PCR method for detection of Salmonella spp. in pork meat. International Journal of Food Microbiology, 184, 134–138.
- Don, S., Xavier, K. A. M., Devi, S. T., Nayak, B. B., & Kannuchamy, N. (2018). Identification of potential spoilage bacteria in farmed shrimp (Litopenaeus vannamei): Application of relative rate of spoilage models in shelf life-prediction. LWT, 97, 295–301.
- Dou, Z., Ferguson, J. D., Galligan, D. T., Kelly, A. M., Finn, S. M., & Giegengack, R. (2016). Assessing U.S. food wastage and opportunities for reduction. Global Food Security, 8, 19–26.
- Ercolini, D., Ferrocino, I., Nasi, A., Ndagijimana, M., Vernocchi, P., La Storia, A., … Villani, F. (2011). Monitoring of microbial metabolites and bacterial diversity in beef stored under different packaging conditions. Applied and Environmental Microbiology, 77(20), 7372–7381.
- Esteki, M., Shahsavari, Z., & Simal-Gandara, J. (2018). Use of spectroscopic methods in combination with linear discriminant analysis for authentication of food products. Food Control, 91, 100–112.
- Fu, H., Yuan, J., & Gao, H. (2015). Microbial oxidative stress response: Novel insights from environmental facultative anaerobic bacteria. Archives of Biochemistry and Biophysics, 584, 28–35.
- Gadgil, M., Kapur, V., & Hu, W.-S. (2005). Transcriptional response of escherichia coli to temperature shift. Biotechnology Progress, 21(3), 689–699.
- Ghasemi-Varnamkhastim, M., Mohtasebi, S. S., Siadat, M., & Balasubramanian, S. (2009). Meat quality assessment by electronic nose (Machine olfactory technology). Sensors, 9, 6058–6083.
- Gordon, R., Cozzolino, D., Chandra, S. , Power, A., Roberts, J. J., & Chapman, J. (2017). Analysis of Australian beers using fluorescence spectroscopy. Beverages, 3(4), 57.
- Gribble, A., & Brightwell, G. (2013). Spoilage characteristics of Brochothrix thermosphacta and campestris in chilled vacuum packaged lamb, and their detection and identification by real time PCR. Meat Science, 94(3), 361–368.
- Gupta, N., Sharma S, Mir IA, Kumar D. (2006). Advances in sensors based on conducting polymers. Journal of Scientific & Industrial Research, 65, 549–557.
- Hagen, N. K., & Kudenov, M. W. (2014). Review of snapshot spectral imaging technologies. Optical Engineering, 52(9), 1–23.
- Han, J., Li, Y., Yuan, J., Li, Z., Zhao, R., Han, T., & Han, T. (2018). To direct the self-assembly of AIEgens by three-gear switch: Morphology study, amine sensing and assessment of meat spoilage. Sensors and Actuators B: Chemical, 258, 373–380.
- Hasan, N. U., Ejaz, N., Ejaz, W., & Kim, H. S. (2012). Meat and fish freshness inspection system based on odor sensing. Sensors, 12, 15542–15557.
- He, Q.-D., Huang, D.-P., Huang, G., & Chen, Z.-G. (2016). Advance in research of microfluidic polymerase chain reaction chip. Chinese Journal of Analytical Chemistry, 44(4), 542–550.
- Illikoud, N., Rossero, A., Chauvet, R., Courcoux, P., Pilet M.-F., Charrier, T., … Zagorec, M. (in press). Genotypic and phenotypic characterization of the food spoilage bacterium Brochothrix thermosphacta. Food Microbiology, 2018, 1–10. doi:10.1016/j.fm.2018.01.015
- Jaiswal, P., Jha, S. N., Kaur, J., Borah, A., & Ramya, H. G. (2018). Detection of aflatoxin M1 in milk using spectroscopy and multivariate analyses. Food Chemistry, 238, 209–214.
- Kachele, R., Zhang, M., Gao, Z., & Adhikari, B. (2017). Effect of vacuum packaging on the shelf-life of silver carp (Hypophthalmichthys molitrix) fillets stored at 4°C. LWT, 80, 163–168.
- Kristensen, L., Støier, S., Würtz, J., & Hinrichsen, L. (2014). Trends in meat science and technology: The future looks bright, but the journey will be long. Meat Science, 98(3), 322–329.
- Li, Y.-S., & Church, J. S. (2014). Raman spectroscopy in the analysis of food and pharmaceutical nanomaterials. Journal of Food and Drug Analysis, 22(1), 29–48.
- Liu, J., Zamora, A., Castillo, M., & Saldo, J. (2018). Modeling of the changes in bovine milk caused by ultra-high pressure homogenization using front-face fluorescence spectroscopy. Journal of Food Engineering. doi:10.1016/j.jfoodeng.2018.04.010
- Lohumi, S., Lee, S., Lee, H., & Cho, B. K. (2015). A review of vibrational spectroscopic techniques for the detection of food authenticity and adulteration. Trends in Food Science & Technology, 1(46), 85–98.
- Majchrzak, T., Wojnowski, W., Dymerski, T., Gębicki, J., & Namieśnik, J. (2018). Electronic noses in classification and quality control of edible oils: A review. Food Chemistry, 246, 192–201.
- Markiewicz-Keszycka, M., Cama-Moncunill, X., Casado-Gavalda, M. P., Dixit, Y., Cama-Moncunill, R., Cullen, P. J., & Sullivan, C. (2017). Laser-induced breakdown spectroscopy (LIBS) for food analysis: A review. Trends in Food Science & Technology, 65, 80–93.
- Mayr, D., Margesin, R., Klingsbichel, E., Hartungen, E., Jenewein, D., Schinner, F., & Märk, T. D. (2003). Rapid detection of meat spoilage by measuring volatile organic compounds by using proton transfer reaction mass spectrometry. Applied and Environmental Microbiology, 69(8), 4697–4705.
- Monago-Maraña, O., Guzmán-Becerra, M., Muñoz de la Peña, A., & Galeano-Díaz, T. (2018). Determination of pungency in spicy food by means of excitation-emission fluorescence coupled with second-order chemometric calibration. Journal of Food Composition and Analysis, 67, 10–18.
- Mooijman, K. A. (2018). The new ISO 6579-1: A real horizontal standard for detection of Salmonella, at last! Food Microbiology, 71, 2–7.
- Neely, K., Taylor, C., Prosser, O., & Hamlyn, P. F. (2001). Assessment of cooked alpaca and llama meats from the statistical analysis of data collected using an ‘electronic nose’. Meat Science, 58(1), 53–58.
- Nuernberger, P., Ruetzel, S., & Brixner, T. (2015). Multidimensional electronic spectroscopy of photochemical reactions. Angewandte Chemie International Edition, 54(39), 11368–11386.
- Oto, N., Oshita, S., Makino, Y., Kawagoe, Y. (2013). Non-destructive evaluation of ATP content and plate count on pork meat surface by fluorescence spectroscopy. Meat Science, 93(3), 579–585.
- Pu, Y., Wang, W., & Alfano, R. R. (2013). Optical detection of meat spoilage using fluorescence spectroscopy with selective excitation wavelength. Applied Spectroscopy, 67(2), 210–213.
- Ramsden, J. J. (2011). Nanotechnology; An Introduction (1st ed.). Waltham: Elsevier Inc.
- Rawat, S. (2015). Food spoilage: microorganisms and their prevention. Asian Journal of Plant Science and Research, 5(4), 47–56.
- Reid, R., Burgess, C. M., McCabe, E., Fanning, S., Whyte, P., Kerry, J., & Bolton, D. (2017). Real-time PCR methods for the detection of blown pack spoilage causing Clostridium species; C. estertheticum, C. gasigenes and C. ruminantium. Meat Science, 133, 56–60.
- Rodriguez-Lazaro, D., Gonzalez-García, P., Delibato, E., De Medici, D., García-Gimeno, R., Valero, A., & Hernandez, M. (2014). Next day Salmonella spp. detection method based on real-time PCR for meat, dairy and vegetable food products. International Journal of Food Microbiology, 13(8), 113–120.
- Rodriguez-Lazaro, D., Hernández, M., Esteve, T., Hoorfar, J., & Pla, M. (2003). A rapid and direct real time PCR-based method for identification of Salmonella spp. Journal of Microbiological Methods, 54(3), 381–390.
- Roostalu, J., Jõers, A., Luidalepp, H., Kaldalu, N., & Tenson, T. (2008). Cell division in Escherichia colicultures monitored at single cell resolution. BMC Microbiology, 8(1), 68.
- Sádecká, J., Jakubíková, M., & Májek, P. (2018). Fluorescence spectroscopy for discrimination of botrytized wines. Food Control, 88, 75–84.
- Sahar, A., & Dufour, É. (2014). Use of Fourier transform-infrared spectroscopy to predict spoilage bacteria on aerobically stored chicken breast fillets. LWT - Food Science and Technology, 56(2), 315–320.
- Sarioglu, O., Celebioglu, A., Tekinay, T., & Uyar, T. (2016). Bacteria-immobilized electrospun fibrous polymeric webs for hexavalent chromium remediation in water. International Journal of Environmental Science and Technology, 13(8), 2057–2066.
- Schlücker, S. (2014). Surface-enhanced raman spectroscopy: concepts and chemical applications. Angewandte Chemie, 53(9), 4756–4795.
- Sezer, B., Bilge, G., & Boyaci, I. H. (2017). Capabilities and limitations of LIBS in food analysis. TrAC Trends in Analytical Chemistry, 97, 345–353.
- Shapaval, V. S., Møretrø, J., Suso, T., Skaar, H. P., Asli, I., Lillehaug, A. W., & Kohler A, D. (2012). Characterization of food spoilage fungi by FTIR spectroscopy. Journal of Applied Microbiology, 114(3), 788–796.
- Shirai, H., Oshita, S., & Makino, Y. (2016). Detection of fluorescence signals from ATP in the second derivative excitation–Emission matrix of a pork meat surface for cleanliness evaluation. Journal of Food Engineering, 168, 173–179.
- Stanborough, T., Fegan, N., Powell, S. M., Singh, T., Tamplin, M., & Chandry, P. S. (2018). Genomic and metabolic characterization of spoilage-associated Pseudomonas species. International Journal of Food Microbiology, 268, 61–72.
- Sun, D.-W. (2016). Computer vision technology for food quality evaluation (2nd ed.). San Diego, USA: Academic Press.
- Syamaladevi, R. M., Tang, J., Villa-Rojas, R., Sablani, S., Carter, B., & Campbell, G. (2016). Influence of water activity on thermal resistance of microorganisms in low‐moisture foods: A review. Comprehensive Reviews in Food Science and Food Safety, 15(2), 353–370.
- Temiz, H. T., Sezer, B., Berkkan, A., Tamer, U., & Boyaci, I. H. (2018). Assessment of laser induced breakdown spectroscopy as a tool for analysis of butter adulteration. Journal of Food Composition and Analysis, 67, 48–54.
- Timsorn, K., Thoopboochagorn, T., Lertwattanasakul, N., & Wongchoosuk, C. (2016). Evaluation of bacterial population on chicken meats using a briefcase electronic nose. Biosystems Engineering, 151, 116–125.
- Wang, C., Yang, J., Zhu, X., Lu, Y., Xue, Y., & Lu, Z. (2017). Effects of Salmonella bacteriophage, nisin and potassium sorbate and their combination on safety and shelf life of fresh chilled pork. Food Control, 73, 869–877.
- Wang, S., He, Y., Wang, Y., Tao, N., Wu, X., Wang, X., … Ma, M. (2016). Comparison of flavour qualities of three sourced Eriocheir sinensis. Food Chemistry, 200, 24–31.
- White, A. K., Heyries KA, Doolin C, Vaninsberghe M (2013). High-throughput microfluidic single-cell digital polymerase chain reaction. Anal Chem, 85(15), 7182–7190.
- Wilson, A. D. (2013). Diverse applications of electronic-nose technologies in agriculture and forestry. Sensors, 13, 2295–2348.
- Wu, D., & Sun, D.-W. (2013). Advanced applications of hyperspectral imaging technology for food quality and safety analysis and assessment: A review — Part II: Applications. Innovative Food Science & Emerging Technologies, 19, 15–28.
- Zhang, Y., Yao, Y., Gao, L., Wang, Z., & Xu, B. (2018). Characterization of a microbial community developing during refrigerated storage of vacuum packed Yao meat, a Chinese traditional food. LWT, 90, 562–569.
- Zheng, J. H., & He, L. (2014). Surface-enhanced raman spectroscopy for the chemical analysis of food. Comprehensive Reviews in Food Science and Food Safety, 13(3), 317–328.
- Zinjarde, S. S. (2014). Food-related applications of Yarrowia lipolytica. Food Chemistry, 152, 1–10.
- Zou, Y., Kang, D., Liu, R., Qi, J., Zhou, G., & Zhang, W. (2018). Effects of ultrasonic assisted cooking on the chemical profiles of taste and flavor of spiced beef. Ultrasonics Sonochemistry, 46, 36–45.